- 1Clinical Institute of Clinical Chemistry and Biochemistry, University Medical Centre Ljubljana, Ljubljana, Slovenia
- 2Faculty of Pharmacy, University of Ljubljana, Ljubljana, Slovenia
- 3Faculty of Health Sciences, Biomedicine in Healthcare, University of Ljubljana, Ljubljana, Slovenia
- 4Nutrition Institute, Ljubljana, Slovenia
- 5Biotechnical Faculty, Department of Food Science and Technology, Group for Nutrition, Ljubljana, Slovenia
- 6Division of Paediatrics, University Medical Centre Ljubljana, Ljubljana, Slovenia
- 7Division of Internal Medicine, Department of Gastroenterology, University Medical Centre Ljubljana, Ljubljana, Slovenia
- 8Faculty of Applied Sciences (VIST), Ljubljana, Slovenia
Objective: The aim of our study was to compare the total 25(OH)D fraction, the bioavailable vitamin fraction, and the free vitamin D fraction in spring and fall in a group of healthy individuals.
Methods: In our study, we collected blood samples from healthy participants at the end of both summer and winter, and measured serum levels of albumin, DBP, and 25(OH)D. Utilizing these data, we calculated the percentage of free and bioavailable vitamin D. Our cohort comprised 87 participants, with a male-to-female ratio of 14:73, aged 35.95 ± 12.55 years, ranging from 19 to 70 years. We employed the chemiluminescence method to determine the vitamin 25(OH)D levels, the ELISA method was utilized to determine DBP levels, the albumin BCP Assay was performed using the ADVIA biochemical analyzer (Siemens) and an online calculator was used to determine the free and bioavailable 25(OH)D levels.
Results: Our findings indicate significantly lower 25(OH)D levels in winter (44.13 ± 17.82 nmol/L) compared to summer (74.97 ± 22.75 nmol/L; p < 0.001). For vitamin D binding protein there was no significant difference from summer (236.2 ± 164.39 mg/L) to winter (239.86 ± 141.9 mg/L; p = 0.77), albumin levels were significantly higher in summer (49.37 ± 4.15 g/L vs. 47.97 ± 3.91 g/L, p = 0.01), but the magnitude of the change may not be large enough to be solely responsible for the stability of vitamin D levels throughout the year. In the winter season a significantly lower calculated bioavailable 25(OH)D vitamin (7.45 ± 5.66 nmol/L against 13.11 ± 8.27 nmol/L; p < 0.001) was observed, and the free fraction also showed a significant decrease (17.3 ± 12.9 pmol/L versus 29.7 ± 19.1 pmol/L; p < 0.0001). We observed a moderately positive correlation between 25(OH)D and bioavailable percentage in winter (r = 0.680; p < 0.001), in contrast with a lower positive association in summer (r = 0.343; p < 0.001).
Conclusion: Our data suggest a positive correlation between total and bioavailable 25(OH)D levels. In addition to the statistically significant variation in 25(OH)D between the two observation periods, there was an additional variation in the free vitamin D percentage. The summertime synthesis of vitamin D in the skin could contribute directly to the free fraction of vitamin D. Standardizing the measurement of free 25(OH)D and clinical studies is necessary to establish reference values before these methods can be implemented in clinical practice.
Introduction
Physiological functions
Vitamin D is a pro-hormone that plays a crucial role in phosphorus-calcium metabolism and is therefore essential for the maintenance of healthy bones and teeth (Holick, 2008a).
Vitamin D is also involved in several other important physiological functions, including: 1) maintaining immune function by stimulating white blood cell production, 2) regulating cell growth and differentiation, which is important for maintaining healthy tissues and organs, 3) reducing the risk of depression and improving cognitive function, and 4) regulating gene expression through vitamin D’s involvement in the regulation of several genes important for maintaining health and preventing disease (Holick, 2008a; Haussler et al., 2008; Wacker and Holick, 2013; Bouillon and Carmeliet, 2018; Carlberg and Haq, 2018). In recent years, the status of vitamin D has received much attention in the context of COVID-19, which has also contributed to a significant increase in vitamin D supplementation compared to pre-pandemic levels (Žmitek et al., 2021; Vičič and Pandel Mikuš, 2023).
Vitamin D insufficiency and deficiency
Vitamin D deficiency is associated with bone diseases such as rickets and osteoporosis. Low vitamin D levels are also associated with an increased risk of other chronic diseases such as cardiovascular disease, cancer and autoimmune diseases (Calvo et al., 2004; Holick, 2008b; Heaney et al., 2017).
Based on the data that are now available and clinical considerations, various associations and organizations employ slightly different criteria for vitamin D insufficiency, deficiency, and sufficiency.
The Endocrine Society recommends that vitamin D status be assessed in patients who are at risk for vitamin D deficiency by measuring the circulating serum 25(OH)D level using an accurate test. A 25(OH)D level of less than 20 ng/mL (50 nmol/L) is considered to be vitamin D insufficiency, whereas a 25(OH)D of 21–29 ng/mL (52.5–72.5 nmol/L) is considered to be vitamin D sufficiency (Holick et al., 2011).
A multidisciplinary Polish panel developed concerns regarding the guidelines for treating and preventing vitamin D insufficiency in both the general population and in high-risk patient groups. The ranges of total blood 25-hydroxyvitamin D concentration indicating suboptimal status (>20 ng/mL, <50 nmol/L), ideal concentration (>30–50 ng/mL, <75 nmol/L), and vitamin D insufficiency (<20 ng/mL, <50 nmol/L) were confirmed on the basis of network discussions (Płudowski et al., 2023).
Vitamin D metabolism and fractions
Vitamin D sources, metabolism and bioavailability are schematically presented in Figure 1. Vitamin D is mainly obtained through diet and skin synthesis when exposed to UVB rays. Sun exposure is the primary source for most people, but without enough UVB exposure, dietary vitamin D becomes essential (Calvo et al., 2004; Spiro and Buttriss, 2014).
Vitamin D3 is produced from 7-dehydrocholesterol (7-DHC) in the skin through UVB radiation. It is converted to previtamin D3, which reaches peak concentration within hours (Holick, 2008a). This previtamin D3 is then transported to the liver via extracellular fluid and dermal capillaries, bound to vitamin D binding protein (DBP) (Holick et al., 2011).
Dietary sources of vitamin D include vitamin D2 (ergocalciferol), D3 (cholecalciferol), and 25-hydroxycholecalciferol [25(OH)D] (Taylor et al., 2014). These forms are transported to the liver in chylomicrons via lymph and blood plasma.
In the blood, two main fractions of vitamin D are measured. Total 25-hydroxyvitamin D (25(OH)D) is the primary circulating form and is often used as a marker for vitamin D status. It binds to DBP and is taken up by the kidneys through receptor-mediated endocytosis involving megalin and cubilin (Nykjaer et al., 2001). The active form, 1,25-dihydroxyvitamin D [1,25(OH)2D], is produced in the kidneys from 25(OH)D under the influence of parathyroid hormone (Lips, 2006; Holick, 2008b). This active form binds to vitamin D receptors, influencing calcium and phosphorus metabolism (Holick, 2008a; Bikle, 2014).
Vitamin D status typically refers to serum 25(OH)D concentration, which does not include vitamin D stored in fat or other tissues (Prentice et al., 2008; Bolland et al., 2018). This status is influenced by factors like body fat, muscle mass, and genetic variation (Cashman and Kiely, 2011; Ross et al., 2011).
Free 25(OH)D is the fraction that is not bound to DBP and can enter cells directly (Powe et al., 2013a). Bioavailable 25(OH)D includes free and albumin-bound 25(OH)D, making it more readily available for tissue uptake (Bikle and Schwartz, 2019).
DBP transports and stores vitamin D, increasing its stability and protecting it from degradation (Bikle, 2014; Speeckaert et al., 2006). Higher DBP concentrations increase vitamin D binding capacity, forming a larger reservoir of bound vitamin D (Smith and Goodman, 1971).
Measuring free and bioavailable vitamin D is complex and costly, making these measurements uncommon in clinical practice (Chun and Nielson, 2018). These can be calculated using total 25(OH)D, DBP, and albumin levels, although accuracy varies (Vičič et al., 2022).
Vitamin D status fluctuates with the seasons, especially at latitudes above 40°N where UVB exposure is insufficient during autumn and winter (Spiro and Buttriss, 2014; O’Neill et al., 2016).
Seasonal variation
Sunlight-induced vitamin D synthesis in lighter-skinned populations begins at the end of March, with serum total 25(OH)D levels peaking after the summer months and then declining from October onwards. Therefore, a significant proportion of the European population relies on vitamin D from food and endogenous stores to maintain an adequate vitamin D status, especially during the winter season. A rather high prevalence of vitamin D deficiency has been reported in several countries (Spiro and Buttriss, 2014; O’Neill et al., 2016; Zittermann et al., 2016), and was also found in our previous studies (Hribar et al., 2020; Hribar et al., 2023). In addition to geographic location and season, there are other important factors that influence vitamin D status, such as age, sex, body mass index, constitutional skin color and various lifestyle factors (Webb et al., 2018; 1988; Hribar et al., 2020; Žmitek et al., 2020; Hribar et al., 2023).
The amount of solar radiation reaching an area is affected by the amount of time spent in the sun and factors that affect the angle of the sun: season, latitude, altitude and time of the day. Before sun rays reach the skin they can be blocked by particles in the air (PM2.5, PM10) and later by sunscreen use, cosmetics with SPF, clothing and obstacles such as buildings (Neville et al., 2021). When sun rays reach the skin they are partially blocked by melanin, which is dependent on skin pigmentation (Webb et al., 2018; Neville et al., 2021). In our previous study we showed that constitutive skin color, as well as tanning, hours spent in the sun and protective behaviors against the sun affect individual vitamin D levels (Hribar et al., 2023). Older age is associated with decreased cholecalciferol synthesis in the skin (MacLaughlin and Holick, 1985).
In some cases, taking vitamin D supplements may be recommended to maintain adequate levels of this important nutrient, especially during the winter months or in people who are at higher risk of deficiency (Lips, 2001; Kimball et al., 2007).
Studies have shown that levels of both bioavailable vitamin D and free vitamin D vary seasonally, with higher levels generally observed in the summer months and lower levels observed in the winter months (Zerwekh, 2008; Lehmann et al., 2013).
Aims
The aim of the study was to determine and evaluate the relationship between the total 25(OH)D concentration, the amount of free and bioavailable vitamin D and the level of vitamin D binding protein (DBP) in Slovenian adults at the end of winter and at the end of summer.
Materials and methods
Study participants
Our study included 87 healthy volunteers aged 35.95 ± 12.55 years in the range (19–70 years), and the male-to-female ratio was 14:73. General information is presented in Table 1. The participants were part of the Nutri-D study: “Challenges in achieving adequate Vitamin D status in the adult population.” For this study a sub-sample of the Nutri-D study participants recruited in 2020 (the second year of the study) was used. The study was conducted with two observation periods, one in winter (January–February 2020) and one after the end of summer (September 2020). The invitation to participate in the study was posted on social media and the website of the Nutrition Institute (Slovenia).
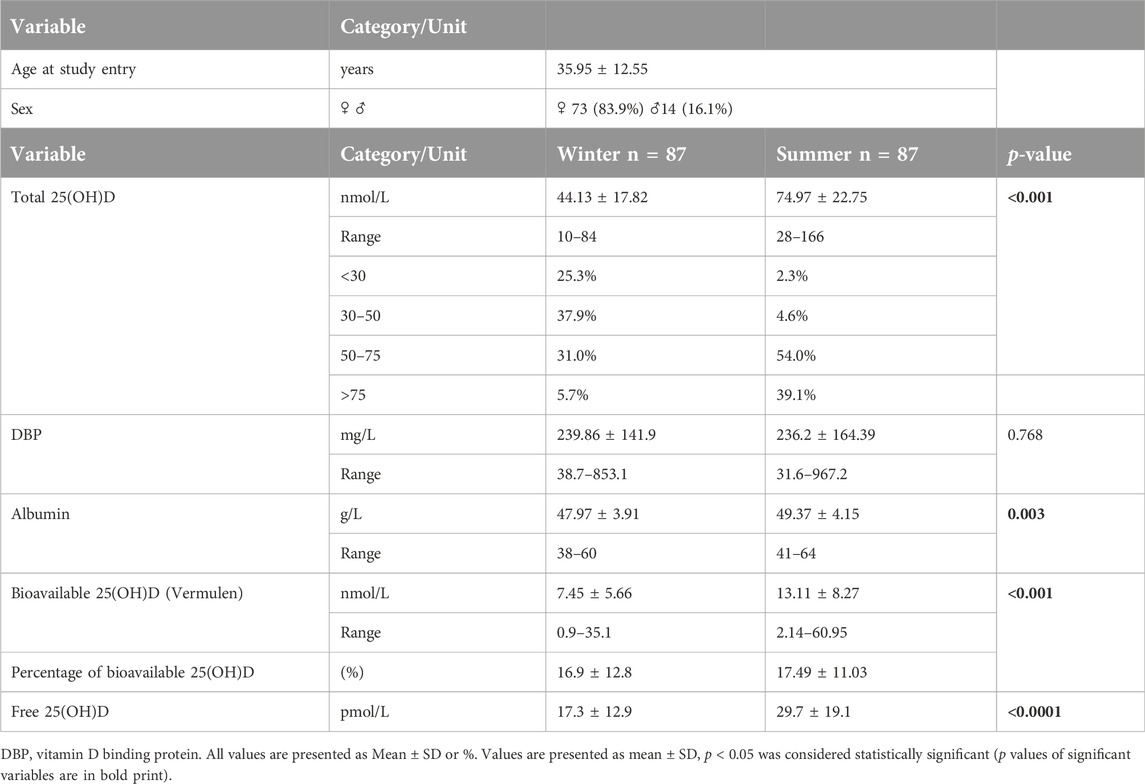
Table 1. Population characteristics, vitamin D status of healthy Slovenian adults aged 19–70 years. The study was conducted in winter (January–February 2020) and after the end of summer (September 2020).
Caucasian race (Fitzpatrick skin types I–IV), age over 18 years, desire to abstain from artificial UVB sources, and willingness to adhere to all study protocols were the inclusion criteria. Pregnancy or breastfeeding, severe sun avoidance (e.g., sun allergy), use of sunbeds, use of supplements or medications containing vitamin D, fish oil, or omega-3 fatty acids in the three months prior to study enrolment, regular (daily) consumption of foods enriched with vitamin D (e.g., fortified margarine or plant-based milk alternatives), adherence to a diet prescribed by a dietitian or medical staff, adherence to special diets (e.g., vegetarianism, low-carbohydrate, high-fat, and calorie restriction); current diseases of the kidneys, thyroid, digestive tract, osteoporosis and other bone diseases, skin diseases and other conditions that interfere with the absorption and synthesis of vitamin D, were the exclusion criteria for the study.
The study protocol was carried out in compliance with institutional and local regulations and was approved by the Ethics Committee of the Faculty of Applied Sciences (VIST), SI-1000 Ljubljana, Slovenia (Approval No. 2018/4-ET-SK). It was also registered at ClinicalTrials.gov (ID: NCT03818594). To take part, each subject had to complete an informed consent form.
Methods of determination
In winter (January-February 2020) and after the end of summer (September 2020) a biochemical blood tube (4 mL) was collected. Albumin and total 25(OH)D were analyzed from fresh serum after blood collection, while the aliquot for DBP was stored at minus 80°C until analysis and all samples were analyzed simultaneously. Special care was taken to ensure that samples were taken from the same subject in the same batch on the same plate.
Measurements were performed at the Clinical Institute of Clinical Chemistry and Biochemistry (University Medical Centre, Ljubljana). Serum 25(OH)D, albumin and DBP, were measured in all participants using the following methods: vitamin 25(OH)D concentration was measured using a competitive luminescence immunoassay with a limit of quantification of 6 nmol/L (Architect analyzer, Abbott Diagnostics, Lake Forest, United States), Human Vitamin D Binding Protein was measured with ELISA (MyBioSource, Inc., San Diego, CA, United States); the limit of quantification was 31 mg/L. The concentration of albumin was measured with an automated Albumin Assay (ADVIA analyzer, Siemens, New York, United States). The assay is based on the reaction of albumin with bromcresol green followed by spectrophotometric detection; the limit of quantification was 10 g/L.
Specific formulas that use the amounts of total 25-hydroxyvitamin D [25(OH)D], vitamin D-binding protein (DBP), and albumin are used to calculate the bioavailable and free fractions of vitamin D. Predictive equations derived from equilibrium dialysis or ultrafiltration procedures may also be used to approximate these formulas.
Calculation of free vitamin D
Free vitamin D can be calculated using the following formula:
Where:
• [Total [25(OH)D] is the total concentration of 25-hydroxyvitamin D.
• [DBP] is the concentration of vitamin D binding protein.
• [Alb] is the concentration of albumin.
• KDBP is the affinity constant of 25(OH)D for DBP (approximately 5.98 × 108 M−1).
• KAlb is the affinity constant of 25(OH)D for albumin (approximately 6 × 105 M−1).
Calculation of bioavailable vitamin D
Bioavailable vitamin D includes both the free and albumin-bound fractions. It can be calculated as follows:
Simplified, the bioavailable vitamin D formula can be written as:
Free and bioavailable 25(OH)D were calculated using an online calculator (Vičič et al., 2022) based on a modified Vermeulen equation (Vermeulen et al., 1999; Powe et al., 2013a; Bikle et al., 2017).
Statistical methods
The observed variables in the statistical analysis were total 25(OH)D, DBP, albumin, calculated bioavailable 25(OH)D and calculated free 25(OH)D. The explanatory variables were season (winter/summer), sex, and age.
The Endocrine Society cut-off values were used to assess total serum 25(OH)D levels target concentration for the optimal vitamin D effect: 75–125 nmol/L, insufficiency: 50–75 nmol/L and deficiency: <50 nmol/L (Holick et al., 2011; Holick et al., 2012; Vieth and Holick, 2018).
A paired sample t-test was performed to analyze differences between groups.
Values are expressed as mean ± SD or percentage (%) in the case of categorical variables. Statistical analysis was performed using SPSS, version 27 and MS Excel 2019.
A Pearson correlation coefficient was calculated to assess the linear relationship (Mukaka, 2012).
Odds ratios (OR) were calculated using binary logistic regression. For OR, 95% confidence intervals were calculated. The significance level was set at p < 0.05.
Results and discussion
Our results show that vitamin D levels are lower in the winter months than in the summer months, that serum albumin concentrations are stable, and that DBP remains almost unchanged. Free vitamin D and bioavailable vitamin D levels are higher in the summer compared to the winter.
Total vitamin D levels are significantly lower in winter 44.13 ± 17.82 nmol/L compared to summer 74.97 ± 22.75 nmol/L (p < 0.001) (This is likely due to reduced sun exposure and lower cutaneous vitamin D synthesis during the winter, as supported by previous studies (Hribar et al., 2020; Hribar et al., 2023).
Although there is a small but significant increase in serum albumin concentration from winter 47.97 ± 3.91 g/L to summer 49.37 ± 4.15 g/L (p < 0.01), this change is not large enough to explain the year-round stability of vitamin D levels.
There was no significant difference in DBP levels from winter 239.86 ± 141.9 mg/L to summer 236.2 ± 164.39 mg/L (p = 0.77). This suggests that DBP is not a major factor in the seasonal variation of vitamin D levels.
There is a noticeable and significant increase in the free fraction of vitamin D from winter 17.3 ± 12.9 pmol/L to summer 29.7 ± 19.1 pmol/L (p < 0.0001), indicating higher availability of unbound vitamin D during the summer months.
The calculated bioavailable 25(OH)D is significantly lower in winter 7.45 ± 5.66 nmol/L compared to summer 13.11 ± 8.27 nmol/L (p < 0.001). This highlights the reduced availability of vitamin D for physiological use during the winter months.
The proportion of bioavailable 25(OH)D as a percentage of the total does not show a statistically significant difference between winter 16.9% ± 12.8% and summer 17.5% ± 11.0%. This suggests that while the absolute levels of bioavailable vitamin D vary seasonally, the relative proportion of bioavailable vitamin D to total vitamin D remains stable throughout the year.
These findings underscore the significant seasonal variation in vitamin D status, which is primarily driven by changes in sun exposure and subsequent synthesis of vitamin D. The stability of the proportion of bioavailable vitamin D relative to total vitamin D suggests that the body’s mechanisms for maintaining vitamin D homeostasis are robust, even though absolute levels fluctuate with the seasons.
A box plot of the winter and summer total 25(OH)D data and the estimated values of the bioavailable percentage are displayed in Figure 2.
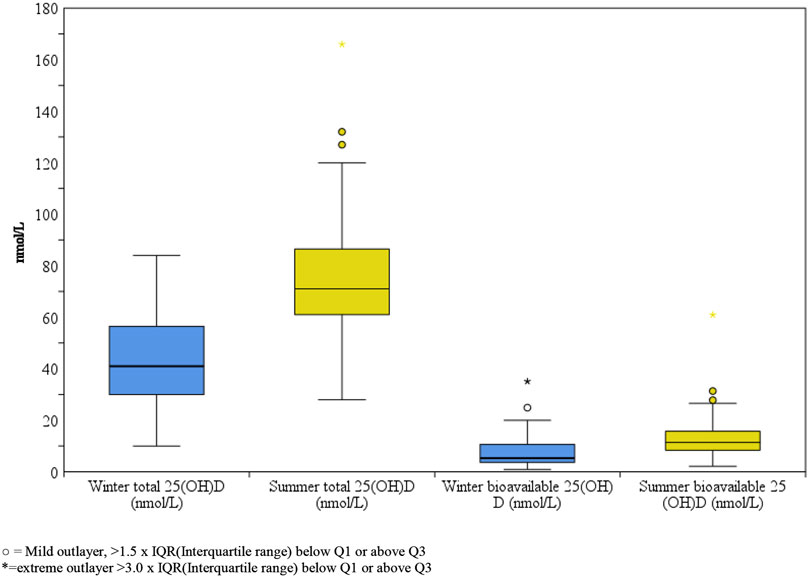
Figure 2. Box-plot comparison of winter and summer total and bioavailable 25(OH)D of healthy Slovenian adults aged 19–70 years. Study was carried out in winter (January-February 2020) and after the end of summer (September 2020).
Correlation analysis
As part of our study, we also calculated the correlation between summer and winter total 25(OH)D and bioavailable vitamin results. The results are shown in Table 2.
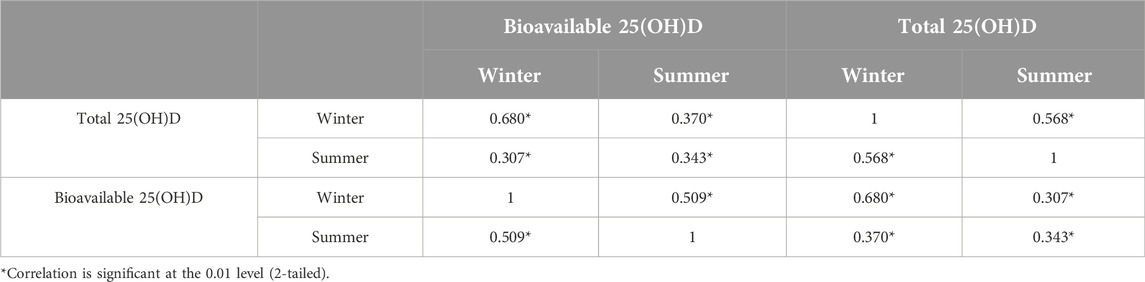
Table 2. Pearson correlation coefficients (r) of blood biomarkers of healthy Slovenian adults aged 19–70 years. The study was conducted in winter (January–February 2020) and after the end of summer (September 2020).
Wintertime Correlation of Total and Bioavailable 25(OH)D (p = 0.680): The robust association suggests that the total 25(OH)D levels are a reliable indicator of the bioavailable 25(OH)D levels during the winter. This suggests that in the absence of considerable sunlight the body’s regulatory systems may be at work to maintain a stable percentage of bioavailable vitamin D from the total amount available.
Winter total 25(OH)D and summer bioavailable 25(OH)D are correlated (p = 0.370): The fact that the association is smaller suggests that winter total 25(OH)D levels do not predict summer bioavailable 25(OH)D levels as well. Prediction based solely on winter levels is reduced because of seasonal variations and greater sun exposure in the summer, which probably result in additional factors influencing vitamin D bioavailability.
Summertime Correlation of Total and Bioavailable 25(OH)D (p = 0.343): Summertime correlations are similar to those observed in winter in that they are weak, meaning that although total 25(OH)D levels do affect bioavailable 25(OH)D levels, the effect is not as great. This decreased correlation may be caused by both increased sun exposure and perhaps greater diversity in the sun exposure habits of each subject.
Summer total 25(OH)D and winter bioavailable 25(OH)D are correlated (p = 0.307): Summer total 25(OH)D levels appear to be a poor predictor of winter bioavailable 25(OH)D levels, based on this weak association. This is to be expected given the stark variations in sun exposure, which cause the amounts of total and bioavailable vitamin D to change dramatically.
In addition, we looked at how the concentrations of total 25(OH)D and bioavailable vitamin D varied with the seasons. The results are shown in Figure 3.
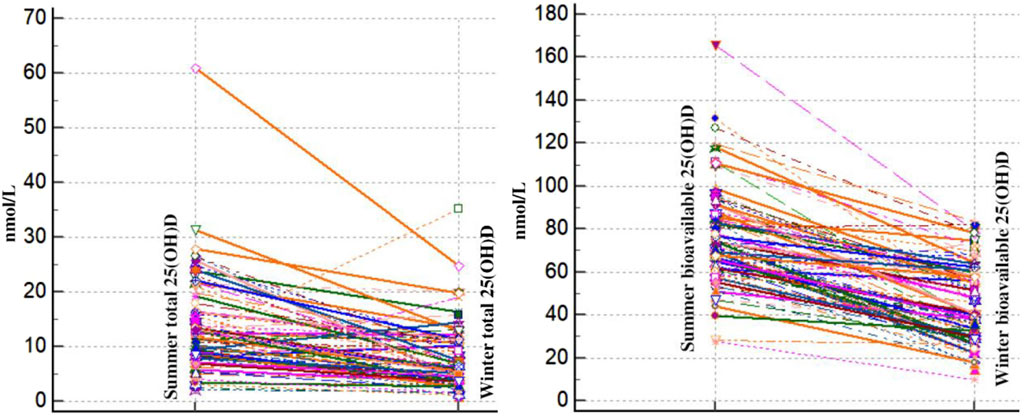
Figure 3. Spaghetti plot comparison of winter and summer total and bioavailable 25(OH)D of healthy Slovenian adults aged 19–70 years. Study was carried out in winter (January–February 2020) and after the end of summer (September 2020).
From winter to summer, there is a noticeable and constant increase in total and bioavailable 25(OH)D levels in all individuals. This seasonal change makes sense given the well-documented consequences of increased sun exposure in the summer. There is observable individual diversity in the absolute levels of total and bioavailable 25(OH)D as well as the degree of growth, despite the general trend. This diversity can be explained by variations in the amount of time that each person spends in the sun, skin type, food consumption, and even hereditary variables that affect the metabolism of vitamin D. Across all the individuals, the proportionate increase in bioavailable 25(OH)D relative to total 25(OH)D appears to remain fairly constant. This implies that the mechanisms controlling the conversion and availability of bioavailable vitamin D are operating consistently in response to the elevated total vitamin D levels from summer sun exposure.
Seasonal variation of total 25(OH)D levels
According to our research, the concentration of vitamin 25(OH)D is considerably lower in the winter than it is in the summer (Hribar et al., 2023). This significant seasonal variation is in line with earlier studies showing that seasonal variations in sunlight exposure have an impact on vitamin D levels. Several studies (Hine and Roberts, 1994; Kull et al., 2009; Hribar et al., 2023) have shown comparable trends and linked lower winter levels to decreased ultraviolet B (UVB) radiation. UVB light is necessary for the production of vitamin D in the skin.
Serum albumin concentrations
Serum albumin concentrations varied significantly between the winter and summer (p = 0.003), but the magnitude of the variation was small. Higher summer levels of free and accessible vitamin D may be due in part to albumin, a key protein that binds to vitamin D. The small magnitude of variation, however, suggests that albumin alone has a limited effect on the seasonal variations in vitamin D levels.
Vitamin D binding protein (DBP) levels
According to our research, summertime vitamin D binding protein levels are slightly lower than wintertime levels, but the difference is not statistically significant (p = 0.77). This is consistent with other research, including the findings of Powe et al. (2013b) that DBP levels do not significantly fluctuate with the seasons. The stability of DBP levels indicates that variations in total 25(OH)D levels are more likely to be the cause of changes in free and bioavailable vitamin D than fluctuations in DBP.
Free fraction of vitamin D
There was a noticeable increase in the percentage of free vitamin D from winter to summer (p < 0.0001). There may be more unbound vitamin D available throughout the summer due to this significant seasonal increase in free vitamin D. This result is in line with recent studies showing that summertime increases in free vitamin D levels are caused by increased cutaneous production and decreased binding to albumin and DBP.
Bioavailable 25(OH)D levels
With a p-value <0.001, bioavailable 25(OH)D was considerably lower in the winter than in the summer. This notable seasonal variation highlights the influence of a lack of UVB exposure on vitamin D bioavailability. The decrease in bioavailable vitamin D during the winter months may have effects on the immune system and bone health. Bioavailable vitamin D is essential for physiological processes and includes both free and albumin-bound fractions. These findings support research by several researchers (Bikle et al., 1986; Bouillon and Van Assche, 1995; O’Mahony et al., 2011; Charoenngam and Holick, 2020), highlighting the importance of bioavailable vitamin D in maintaining health.
Proportion of bioavailable 25(OH)D as a percentage of total
Research such as that of Chun et al. (2010), which addresses the homeostatic control of vitamin D bioavailability, supports the relatively stable proportion of bioavailable 25(OH)D as a percentage of total vitamin D (± standard deviation), with 16.9% ± 12.8% in winter and 17.5% ± 11.0% in summer, which is not a statistically significant difference. This stability suggests that while absolute levels of bioavailable vitamin D vary seasonally, the body’s regulatory mechanisms maintain a consistent proportion of bioavailable to total vitamin D.
Correlation between total 25-hydroxyvitamin D and bioavailable 25-hydroxyvitamin D levels in winter and summer
Because there are fewer daylight hours and less direct sunlight during the winter, there is less exposure to UVB irradiation of sufficient intensity to induce endogenous vitamin D synthesis. As a result, in order to maintain their vitamin D levels, humans rely primarily on food sources and stored vitamin D (from summer sun exposure). Both newly synthesized and stored vitamin D from food sources are included in total 25(OH)D. Conversely, vitamin D that is attached to albumin and the free fraction of vitamin D are known as bioavailable vitamin D, and these are readily absorbed by cells. Consequently, it is expected that a higher level of bioavailable 25(OH)D would correlate with a higher level of total 25(OH)D. This may be because, in the absence of substantial vitamin D deficiency, part of the stored vitamin D becomes accessible.
Summertime is when humans in our latitudes can produce extra vitamin D in their skin due to increased sun exposure and a higher intensity of UVB irradiation. When more freshly generated vitamin D from sunlight is available in the summer, the weak correlation seen in the winter may become a slightly positive correlation. The amount of stored vitamin D that contributes to total 25(OH)D levels decreases as more vitamin D is produced in the skin during the summer. The association with bioavailable vitamin D may be reduced as a result.
The higher correlation in winter indicates that the body is more dependent on its stored vitamin D at times when vitamin D availability is reduced. On the other hand, the reduced summer correlation may be a result of higher synthesis rates and distinct binding dynamics during periods of increased vitamin D availability.
A study of healthy adults in Switzerland, for example, found that both total and free vitamin D levels were significantly lower in winter than in summer, while there were no seasonal differences in bioavailable vitamin D levels (Merlo et al., 2015). In contrast, a study of older adults in the United Kingdom found that while total vitamin D levels were lower in winter, there was no significant difference in free or bioavailable vitamin D between summer and winter months (Cashman et al., 2012; Hilger et al., 2014), Vitamin D supplementation may increase total vitamin D levels, including the bioavailable fraction. However, the extent to which supplementation affects the bioavailable fraction may depend on several factors, including the form and dose of vitamin D, individual differences in vitamin D metabolism and absorption, and the presence of underlying medical conditions.
A study conducted on postmenopausal women with low vitamin D levels found that supplementation with vitamin D3 significantly increased both total and bioavailable vitamin D levels (Hassanein et al., 2023). Another study of overweight and obese adults with vitamin D deficiency showed that daily supplementation with high-dose vitamin D3 for 16 weeks increased bioavailable vitamin D levels (Entezari et al., 2018).
However, some studies have also reported that vitamin D supplementation does not increase bioavailable levels equally in all individuals. For example, one study of healthy adults found that 12 weeks of vitamin D3 supplementation did not significantly alter bioavailable vitamin D levels (Jorde and Grimnes, 2011; Chandler et al., 2015; Flueck et al., 2016).
Food fortification with vitamin D can be an effective strategy for increasing vitamin D intake in populations with low sun exposure or inadequate dietary intake.
Possibilities for food fortification with vitamin D are numerous Examples include but are not limited to: milk, fat spreads (Jääskeläinen et al., 2017), milk alternatives such as soy beverages (Vatanparast et al., 2020), orange juice (Tangpricha et al., 2003), flour and cereals (Allen et al., 2015), UV irradiated mushrooms (Cardwell et al., 2018), UV-irradiated yeast for the preparation of bread (EFSA Panel on Dietetic Products Nutrition and Allergies NDA, 2014), and biofortification of chicken eggs (Browning and Cowieson, 2014; Vičič et al., 2023). The media for fortification should be consumed regularly by the majority of the population and should minimally increase production costs and prices for consumers (Pilz et al., 2018).
Reliable total 25(OH)D, DBP, and albumin levels are necessary for calculation accuracy. Laboratory procedures and assays can differ, which can impact the outcomes.
Genetic variants and individual variations in DBP isoforms can affect binding affinities and concentrations, which in turn can affect the estimated levels of free and bioavailable 25(OH)D (Fernando et al., 2020).
Although helpful, the formulas for determining the free and available fractions of vitamin D have some drawbacks. These include assumptions regarding individual biological differences, measurement variability in proteins, and binding constants.
Immunoassays made specifically to measure free 25-hydroxyvitamin D [25(OH)D], equilibrium dialysis, and ultrafiltration are direct methods for determining levels of free and bioavailable vitamin D. These techniques directly measure the unbound and albumin-bound fractions of vitamin D, with the goal of giving a more realistic picture of the physiologically active forms of the vitamin but their complexity and resource needs prevent frequent use, and are useful in research contexts and for detailed clinical assessments.
Study limitations and future research
The use of bioavailable 25(OH)D or free 25(OH)D may be useful in physiological and pathological conditions that affect DBP, such as pregnancy, genetic polymorphisms, liver disease, and kidney disease (Tsuprykov et al., 2018a), when comparing vitamin D status in premenopausal and postmenopausal women (Vičič et al., 2022) and in ethnically diverse populations (Aloia et al., 2015; Tsuprykov et al., 2018a; Abidin and Mitra, 2020). There is also a discrepancy between calculated free 25(OH)D and directly measured free 25(OH)D. This is more pronounced in African Americans and has been attributed to differences in DBP binding capacity between ethnic groups (Schwartz et al., 2014). Currently there are no generally accepted reference values for calculated or directly measured free and bioavailable 25(OH)D. Zeng et al. (2021) established reference values for free 25(OH)D through linear regression models but emphasized the need for clinical studies to validate their recommendations. Tsuprykov et al. (2018b) established different reference values in pregnant women for both calculated and measured free 25(OH)D. They concluded that both methods may be appropriate.
We did not evaluate bone health or markers of other significant diseases to determine whether bioavailable/free 25(OH)D is a better predictor of low bone mass density and other conditions. We are currently focusing on this assessment in patients with liver disease.
Conclusion
Calculating vitamin D fractions using formulas is a cost-effective and convenient alternative when direct measurement of individual fractions is not available or feasible. However, it is important to consider the assumptions, accuracy and variability associated with the formulas used and recognize that direct measurement methods may provide more accurate and reliable results.
Our study provides important insights into seasonal variations in vitamin D status and bioavailability. We found that vitamin 25(OH)D concentrations are significantly lower in winter compared to summer, with a slight, non-significant difference in vitamin D binding protein from summer to winter, and significantly higher albumin concentrations in summer. Both bioavailable and free vitamin D levels are significantly lower in winter.
The primary cause of the winter decrease in total 25(OH)D levels is reduced sunlight exposure, which limits vitamin D synthesis. This also leads to reduced bioavailable and free vitamin D, indicating that not only is less vitamin D produced, but the amount available for use by the body is also reduced. The stable DBP levels suggest that DBP is not the main factor in the seasonal variation of free and bioavailable vitamin D. The seasonal increase in albumin concentration during summer may contribute to a greater amount of bioavailable vitamin D. Albumin’s weaker binding affinity for vitamin D compared to DBP allows for easier dissociation of vitamin D, shifting the balance toward more free and albumin-bound vitamin D available for cellular uptake.
There is a moderately positive correlation between total and bioavailable 25(OH)D in winter and a lower positive correlation in summer. This stronger winter correlation suggests that during low vitamin D availability, the body relies more on existing stores, while the weaker summer correlation indicates that with higher vitamin D synthesis, bioavailability dynamics are less dependent on total vitamin D levels.
Data availability statement
The raw data supporting the conclusions of this article will be made available by the authors, without undue reservation.
Ethics statement
The study protocol was approved by the Ethics Committee of the Faculty of Applied Sciences (Approval No. 2018/4-ET-SK). The studies were conducted in accordance with the local legislation and institutional requirements. All participants signed an informed consent form to participate. Study was registered at ClinicalTrials.gov (ID: NCT03818594).
Author contributions
Conceptualization, JO; formal analysis, JO, DS, KK, and MH; investigation, AJ, UČ, and TF; writing—original draft, JO and VV; writing—review and editing, JO, VV, EB, IP, and KŽ. All authors contributed to the article and approved the submitted version.
Funding
The authors declare financial support was received for the research, authorship, and/or publication of this article. We acknowledge that the study was conducted within the national research project Nutri-D: “Challenges in achieving adequate vitamin D status in the adult population” (L7-1849), co-funded by the Slovenian Research and Innovation Agency and the Ministry of Health of the Republic of Slovenia. We also acknowledge the support of the national research programs “Nutrition and Public Health” (P3-0395), and “Metabolic and hereditary factors of reproductive health-Labour III” (P3-0124), funded by the Slovenian Research and Innovation Agency, as well as internal research funding of the University Medical Centre Ljubljana, Slovenia (Record IDs 20220017).
Acknowledgments
We would like to thank all the participants who participated in this study. The authors would like to thank Vera Troha Poljančič for her help in processing the serum samples.
Conflict of interest
The authors declare that the research was conducted in the absence of any commercial or financial relationships that could be construed as a potential conflict of interest.
Abbreviations
BMI, body mass index; UV, ultraviolet; D3, cholecalciferol; D2, ergocalciferol; Total 25(OH)D, 25-hydroxycholecalciferol, calcifediol, calcidiol, 25-hydroxyvitamin D3; 1,25-(OH)D, 1,25-dihydroxyvitamin D; DBP, vitamin D binding protein; OR, Odds ratio.
References
Abidin, N. Z., and Mitra, S. R. (2020). Total vs. bioavailable: determining a better 25(OH)D index in association with bone density and muscle mass in postmenopausal women. Metabolites 11, 23. doi:10.3390/metabo11010023
Allen, R. E., Dangour, A. D., Tedstone, A. E., and Chalabi, Z. (2015). Does fortification of staple foods improve vitamin D intakes and status of groups at risk of deficiency? A United Kingdom modeling study. Am. J. Clin. Nutr. 102, 338–344. doi:10.3945/ajcn.115.107409
Aloia, J., Mikhail, M., Dhaliwal, R., Shieh, A., Usera, G., Stolberg, A., et al. (2015). Free 25(OH)D and the vitamin D paradox in african Americans. J. Clin. Endocrinol. Metab. 100, 3356–3363. doi:10.1210/JC.2015-2066
Bikle, D. D. (2014). Vitamin D metabolism, mechanism of action, and clinical applications. Chem. Biol. 21, 319–329. doi:10.1016/j.chembiol.2013.12.016
Bikle, D. D., Gee, E., Halloran, B., Kowalski, M. A., Ryzen, E., and Haddad, J. G. (1986). Assessment of the free fraction of 25-hydroxyvitamin D in serum and its regulation by albumin and the vitamin D-binding protein. J. Clin. Endocrinol. Metab. 63, 954–959. doi:10.1210/jcem-63-4-954
Bikle, D. D., Malmstroem, S., and Schwartz, J. (2017). Current controversies: are free vitamin metabolite levels a more accurate assessment of vitamin D status than total levels? Endocrinol. Metab. Clin. N. Am 46, 901–918. doi:10.1016/j.ecl.2017.07.013
Bikle, D. D., and Schwartz, J. (2019). Vitamin D binding protein, total and free vitamin D levels in different physiological and pathophysiological conditions. Front. Endocrinol. (Lausanne) 10, 317. doi:10.3389/fendo.2019.00317
Bolland, M. J., Grey, A., and Avenell, A. (2018). Effects of vitamin D supplementation on musculoskeletal health: a systematic review, meta-analysis, and trial sequential analysis. Lancet Diabetes Endocrinol. 6, 847–858. doi:10.1016/S2213-8587(18)30265-1
Bouillon, R., and Carmeliet, G. (2018). Vitamin D insufficiency: definition, diagnosis and management. Best Pract. Res. Clin. Endocrinol. Metab. 32, 669–684. doi:10.1016/j.beem.2018.09.014
Bouillon, R., and Van Assche, F. A. (1995). The free fraction of 25-hydroxyvitamin D. Clin. Chem. 41, 1279–1280. doi:10.1210/edrv-16-2-200
Browning, L. C., and Cowieson, A. J. (2014). Vitamin D fortification of eggs for human health. J. Sci. Food Agric. 94, 1389–1396. doi:10.1002/jsfa.6425
Calvo, M. S., Whiting, S. J., and Barton, C. N. (2004). Vitamin D fortification in the United States and Canada: current status and data needs. Am. J. Clin. Nutr. 80, 1710s–6s. doi:10.1093/ajcn/80.6.1710S
Cardwell, G., Bornman, J. F., James, A. P., and Black, L. J. (2018). A review of mushrooms as a potential source of dietary vitamin D. Nutrients 10, 1498. doi:10.3390/NU10101498
Carlberg, C., and Haq, A. (2018). The concept of the personal vitamin D response index. J. Steroid Biochem. Mol. Biol. 175, 12–17. doi:10.1016/j.jsbmb.2016.12.011
Cashman, K. D., and Kiely, M. (2011). Towards prevention of vitamin D deficiency and beyond: knowledge gaps and research needs in vitamin D nutrition and public health. Br. J. Nutr. 106, 1617–1627. doi:10.1017/S0007114511004995
Cashman, K. D., Seamans, K. M., Lucey, A. J., Stöcklin, E., Weber, P., Kiely, M., et al. (2012). Relative effectiveness of oral 25-hydroxyvitamin D3 and vitamin D3 in raising wintertime serum 25-hydroxyvitamin D in older adults. Am. J. Clin. Nutr. 95, 1350–1356. doi:10.3945/ajcn.111.031427
Chandler, P. D., Scott, J. B., Drake, B. F., Ng, K., Chan, A. T., Hollis, B. W., et al. (2015). Impact of vitamin D supplementation on adiposity in African-Americans. Nutr. Diabetes 5, e147. doi:10.1038/nutd.2014.44
Charoenngam, N., and Holick, M. F. (2020). Immunologic effects of vitamin D on human health and disease. Nutrients 12, 2097. doi:10.3390/nu12072097
Chun, R. F., Lauridsen, A. L., Suon, L., Zella, L. A., Pike, J. W., Modlin, R. L., et al. (2010). Vitamin D-binding protein directs monocyte responses to 25-hydroxy- and 1,25-dihydroxyvitamin D. J. Clin. Endocrinol. Metab. 95, 3368–3376. doi:10.1210/jc.2010-0195
Chun, R. F., and Nielson, C. M. (2018). Chapter 51—free Vitamin D: concepts, assays, outcomes, and prospects, in Feldman DBT-VD. Fourth E ed. Academic Press), 925–937.
EFSA Panel on Animal Health and Welfare AHAW (2014). Scientific Opinion on porcine epidemic diarrhoea and emerging porcine deltacoronavirus. J. Eur. Food Saf. Auth. 12, 3877. doi:10.2903/j.efsa.2014.3877
Entezari, M., Khosravi, Z., Kafeshani, M., Tavasoli, P., and Zadeh, A. (2018). Effect of vitamin D supplementation on weight loss, glycemic indices, and lipid profile in obese and overweight women: a clinical trial study. Int. J. Prev. Med. 9, 63. doi:10.4103/ijpvm.IJPVM_329_15
Fernando, M., Ellery, S. J., Marquina, C., Lim, S., Naderpoor, N., and Mousa, A. (2020). Vitamin D-binding protein in pregnancy and reproductive health. Nutrients 12, 1489. doi:10.3390/nu12051489
Flueck, J. L., Schlaepfer, M. W., and Perret, C. (2016). Effect of 12-week vitamin D supplementation on 25[OH]D status and performance in athletes with a spinal cord injury. Nutrients 8, 586. doi:10.3390/nu8100586
Hassanein, M. M., Huri, H. Z., Baig, K., and Abduelkarem, A. R. (2023). Determinants and effects of vitamin D supplementation in postmenopausal women: a systematic review. Nutrients 15, 685. doi:10.3390/nu15030685
Haussler, M. R., Haussler, C. A., Bartik, L., Whitfield, G. K., Hsieh, J.-C., Slater, S., et al. (2008). Vitamin D receptor: molecular signaling and actions of nutritional ligands in disease prevention. Nutr. Rev. 66, S98–S112. doi:10.1111/j.1753-4887.2008.00093.x
Heaney, R. P., Holick, M. F., and Rafferty, K. (2017). The vitamin D nutrient reference value: back to the future? J. Bone Min. Res. 32, 1317–1319. doi:10.1016/j.jsbmb.2016.12.021
Hilger, J., Friedel, A., Herr, R., Rausch, T., Roos, F., Wahl, D. A., et al. (2014). A systematic review of vitamin D status in populations worldwide. Br. J. Nutr. 111, 23–45. doi:10.1017/S0007114513001840
Hine, T. J., and Roberts, N. B. (1994). Seasonal variation in serum 25-hydroxy vitamin D3 does not affect 1,25-dihydroxy vitamin D. Ann. Clin. Biochem. 31 (Pt 1), 31–34. doi:10.1177/000456329403100105
Holick, M. F. (2008a). The vitamin D deficiency pandemic and consequences for non-skeletal health: mechanisms of action. Mol. Aspects Med. 29, 361–368. doi:10.1016/j.mam.2008.08.008
Holick, M. F. (2008b). Vitamin D: a D-lightful health perspective. Nutr. Rev. 66, S182–S194. doi:10.1111/j.1753-4887.2008.00104.x
Holick, M. F., Binkley, N. C., Bischoff-Ferrari, H. A., Gordon, C. M., Hanley, D. A., Heaney, R. P., et al. (2011). Evaluation, treatment, and prevention of vitamin D deficiency: an endocrine society clinical practice guideline. J. Clin. Endocrinol. Metabol. 96, 1911–1930. doi:10.1210/jc.2011-0385
Holick, M. F., Binkley, N. C., Bischoff-Ferrari, H. A., Gordon, C. M., Hanley, D. A., Heaney, R. P., et al. (2012). Guidelines for preventing and treating vitamin D deficiency and insufficiency revisited. J. Clin. Endocrinol. and Metabolism 97, 1153–1158. doi:10.1210/jc.2011-2601
Hribar, M., Hristov, H., Gregorič, M., Blaznik, U., Zaletel, K., Oblak, A., et al. (2020). Nutrihealth study: seasonal variation in vitamin D status among the slovenian adult and elderly population. Nutrients 12, 1–18. doi:10.3390/NU12061838
Hribar, M., Pravst, I., Pogačnik, T., and Žmitek, K. (2023). Results of longitudinal nutri-D study: factors influencing winter and summer vitamin D status in a caucasian population. Front. Nutr. 10, 1253341. doi:10.3389/fnut.2023.1253341
Jääskeläinen, T., Itkonen, S. T., Lundqvist, A., Erkkola, M., Koskela, T., Lakkala, K., et al. (2017). The positive impact of general Vitamin D food fortification policy on Vitamin D status in a representative adult Finnish population: evidence from an 11-y follow-up based on standardized 25-hydroxyVitamin D data. Am. J. Clin. Nutr. 105, 1512–1520. doi:10.3945/ajcn.116.151415
Jorde, R., and Grimnes, G. (2011). Vitamin D and metabolic health with special reference to the effect of vitamin D on serum lipids. Prog. Lipid Res. 50, 303–312. doi:10.1016/j.plipres.2011.05.001
Kimball, S. M., Ursell, M. R., O’Connor, P., and Vieth, R. (2007). Safety of vitamin D3 in adults with multiple sclerosis. Am. J. Clin. Nutr. 86, 645–651. doi:10.1093/ajcn/86.3.645
Kull, M. J., Kallikorm, R., Tamm, A., and Lember, M. (2009). Seasonal variance of 25-(OH) vitamin D in the general population of Estonia, a Northern European country. BMC Public Health 9, 22. doi:10.1186/1471-2458-9-22
Lehmann, U., Hirche, F., Stangl, G. I., Hinz, K., Westphal, S., and Dierkes, J. (2013). Bioavailability of vitamin D(2) and D(3) in healthy volunteers, a randomized placebo-controlled trial. J. Clin. Endocrinol. Metabol. 98, 4339–4345. doi:10.1210/jc.2012-4287
Lips, P. (2001). Vitamin D deficiency and secondary hyperparathyroidism in the elderly: consequences for bone loss and fractures and therapeutic implications. Endocr. Rev. 22, 477–501. doi:10.1210/er.22.4.477
Lips, P. (2006). Vitamin D physiology. Prog. Biophys. Mol. Biol. 92, 4–8. doi:10.1016/j.pbiomolbio.2006.02.016
MacLaughlin, J., and Holick, M. F. (1985). Aging decreases the capacity of human skin to produce vitamin D3. J. Clin. Invest. 76, 1536–1538. doi:10.1172/JCI112134
Merlo, C., Trummler, M., Essig, S., and Zeller, A. (2015). Vitamin D deficiency in unselected patients from Swiss primary care: a cross-sectional study in two seasons. PLoS One 10, e0138613. doi:10.1371/journal.pone.0138613
Mukaka, M. M. (2012). Statistics corner: a guide to appropriate use of correlation coefficient in medical research. Malawi Med. J. 24, 69–71.
Neville, J. J., Palmieri, T., and Young, A. R. (2021). Physical determinants of vitamin D photosynthesis: a review. JBMR plus 5, e10460. doi:10.1002/jbm4.10460
Nykjaer, A., Fyfe, J. C., Kozyraki, R., Leheste, J. R., Jacobsen, C., Nielsen, M. S., et al. (2001). Cubilin dysfunction causes abnormal metabolism of the steroid hormone 25(OH) vitamin D(3). Proc. Natl. Acad. Sci. U. S. A. 98, 13895–13900. doi:10.1073/pnas.241516998
O’Mahony, L., Stepien, M., Gibney, M. J., Nugent, A. P., and Brennan, L. (2011). The potential role of vitamin D enhanced foods in improving vitamin D status. Nutrients 3, 1023–1041. doi:10.3390/nu3121023
O’Neill, C. M., Kazantzidis, A., Ryan, M. J., Barber, N., Sempos, C. T., Durazo-Arvizu, R. A., et al. (2016). Seasonal changes in vitamin D-effective UVB availability in europe and associations with population serum 25-hydroxyvitamin D. Nutrients 8, 533. doi:10.3390/nu8090533
Pilz, S., März, W., Cashman, K. D., Kiely, M. E., Whiting, S. J., Holick, M. F., et al. (2018). Rationale and plan for vitamin D food fortification: a review and guidance paper. Front. Endocrinol. (Lausanne) 9, 373. doi:10.3389/fendo.2018.00373
Płudowski, P., Kos-Kudła, B., Walczak, M., Fal, A., Zozulińska-Ziółkiewicz, D., Sieroszewski, P., et al. (2023). Guidelines for preventing and treating vitamin D deficiency: a 2023 update in Poland. Nutrients 15, 695. doi:10.3390/nu15030695
Powe, C. E., Evans, M. K., Wenger, J., and Tr, Z. (2013b). Seasonal variations in plasma 25-hydroxyvitamin D and vitamin D-binding protein in African American and white women. Am. J. Clin. Nutr. 97, 470–476. doi:10.1056/NEJMoa1306357
Powe, C. E., Evans, M. K., Wenger, J., Zonderman, A. B., Berg, A. H., Nalls, M., et al. (2013a). Vitamin D-binding protein and vitamin D status of black Americans and white Americans. N. Engl. J. Med. 369, 1991–2000. doi:10.1056/NEJMoa1306357
Prentice, A., Goldberg, G. R., and Schoenmakers, I. (2008). Vitamin D across the lifecycle: physiology and biomarkers. Am. J. Clin. Nutr. 88, 500S–506S. doi:10.1093/ajcn/88.2.500S
Ross, A. C., Manson, J. E., Abrams, S. A., Aloia, J. F., Brannon, P. M., Clinton, S. K., et al. (2011). The 2011 report on dietary reference intakes for calcium and vitamin D from the Institute of Medicine: what clinicians need to know. J. Clin. Endocrinol. Metab. 96, 53–58. doi:10.1210/jc.2010-2704
Schwartz, J. B., Lai, J., Lizaola, B., Kane, L., Markova, S., Weyland, P., et al. (2014). A comparison of measured and calculated free 25(OH) vitamin D levels in clinical populations. J. Clin. Endocrinol. Metabol. 99, 1631–1637. doi:10.1210/jc.2013-3874
Speeckaert, M., Huang, G., Delanghe, J. R., and Taes, Y. E. C. (2006). Biological and clinical aspects of the vitamin D binding protein (Gc-globulin) and its polymorphism. Clin. Chim. Acta. 372, 33–42. doi:10.1016/j.cca.2006.03.011
Spiro, A., and Buttriss, J. L. (2014). Vitamin D: an overview of vitamin D status and intake in Europe. Nutr. Bull. 39, 322–350. doi:10.1111/nbu.12108
Smith, J. E., and Goodman, D. S. (1971). The turnover and transport of vitamin D and of a polar metabolite with the properties of 25-hydroxycholecalciferol in human plasma. J. Clin. Invest. 50, 2159–2167. doi:10.1172/JCI106710
Tangpricha, V., Koutkia, P., Rieke, S. M., Chen, T. C., Perez, A. A., and Holick, M. F. (2003). Fortification of orange juice with vitamin D: a novel approach for enhancing vitamin D nutritional health. Am. J. Clin. Nutr. 77, 1478–1483. doi:10.1093/ajcn/77.6.1478
Taylor, C. L., Patterson, K. Y., Roseland, J. M., Wise, S. A., Merkel, J. M., Pehrsson, P. R., et al. (2014). Including food 25-hydroxyvitamin D in intake estimates may reduce the discrepancy between dietary and serum measures of vitamin D status. J. Nutr. 144, 654–659. doi:10.3945/jn.113.189811
Tsuprykov, O., Buse, C., Skoblo, R., Haq, A., and Hocher, B. (2018b). Reference intervals for measured and calculated free 25-hydroxyvitamin D in normal pregnancy. J. Steroid Biochem. Mol. Biol. 181, 80–87. doi:10.1016/j.jsbmb.2018.03.005
Tsuprykov, O., Chen, X., Hocher, C.-F., Skoblo, R., Yin, L., and Hocher, B. (2018a). Why should we measure free 25(OH) vitamin D? J. Steroid Biochem. Mol. Biol. 180, 87–104. doi:10.1016/j.jsbmb.2017.11.014
Vatanparast, H., Patil, R. P., Islam, N., Shafiee, M., and Whiting, S. J. (2020). Vitamin D intake from supplemental sources but not from food sources has increased in the Canadian population over time. J. Nutr. 150, 526–535. doi:10.1093/jn/nxz291
Vermeulen, A., Verdonck, L., and Kaufman, J. M. (1999). A critical evaluation of simple methods for the estimation of free testosterone in serum. J. Clin. Endocrinol. and Metabolism 84, 3666–3672. doi:10.1210/jcem.84.10.6079
Vičič, V., Kukec, A., Kugler, S., Geršak, K., Osredkar, J., and Pandel Mikuš, R. (2022). Assessment of vitamin D status in Slovenian premenopausal and postmenopausal women, using total, free, and bioavailable 25-hydroxyvitamin D (25(OH)D). Nutrients 14, 1–3. doi:10.3390/nu14245349
Vičič, V., Mikuš, R. P., Kugler, S., Geršak, K., Osredkar, J., and Kukec, A. (2023). Vitamin D fortification of eggs alone and in combination with milk in women aged 44-65 years: fortification model and economic evaluation. Slovenian J. Public Health 62, 30–38. doi:10.2478/sjph-2023-0005
Vičič, V., and Pandel Mikuš, R. (2023). Vitamin D supplementation during COVID-19 lockdown and after 20 months: follow-up study on Slovenian women aged between 44 and 66. Slovenian J. Public Health 62, 182–189. doi:10.2478/sjph-2023-0026
Vieth, R., and Holick, M. F. (2018). “The IOM—endocrine society controversy on recommended vitamin D targets: In support of the endocrine society position,” in Vitamin D. Editor D. Feldman 4th ed. (Academic Press), 1091–1107. doi:10.1016/B978-0-12-809965-0.00059-8
Wacker, M., and Holick, M. F. (2013). Vitamin D - effects on skeletal and extraskeletal health and the need for supplementation. Nutrients 5, 111–148. doi:10.3390/nu5010111
Webb, A. R., Kazantzidis, A., Kift, R. C., Farrar, M. D., Wilkinson, J., and Rhodes, L. E. (2018). Colour counts: sunlight and skin type as drivers of vitamin D deficiency at UK latitudes. Nutrients 10, 457. doi:10.3390/nu10040457
Webb, A. R., Kline, L., and Holick, M. F. (1988). Influence of season and latitude on the cutaneous synthesis of vitamin D3: exposure to winter sunlight in Boston and edmonton will not promote vitamin D3 synthesis in human skin. J. Clin. Endocrinol. and Metabolism 67, 373–378. doi:10.1210/jcem-67-2-373
Zeng, S., Chu, C., Doebis, C., von Baehr, V., and Hocher, B. (2021). Reference values for free 25-hydroxy-vitamin D based on established total 25-hydroxy-vitamin D reference values. J. Steroid Biochem. Mol. Biol. 210, 105877. doi:10.1016/j.jsbmb.2021.105877
Zerwekh, J. E. (2008). Blood biomarkers of vitamin D status. Am. J. Clin. Nutr. 87, 1087S–91S. doi:10.1093/ajcn/87.4.1087S
Zittermann, A., Pilz, S., Hoffmann, H., and März, W. (2016). Vitamin D and airway infections: a European perspective. Eur. J. Med. Res. 21, 14. doi:10.1186/s40001-016-0208-y
Žmitek, K., Hribar, M., Hristov, H., and Pravst, I. (2020). Efficiency of vitamin D supplementation in healthy adults is associated with body mass index and baseline serum 25-hydroxyvitamin D level. Nutrients 12, 1268. doi:10.3390/nu12051268
Žmitek, K., Hribar, M., Lavriša, Ž., Hristov, H., Kušar, A., and Pravst, I. (2021). Socio-demographic and knowledge-related determinants of vitamin D supplementation in the context of the COVID-19 pandemic: assessment of an educational intervention. Front. Nutr. 8, 648450. doi:10.3389/fnut.2021.648450
Keywords: vitamin 25(OH)D, free fraction of vitamin D, bioavailable fraction of vitamin D, seasonal variation, calculated fractions
Citation: Osredkar J, Vičič V, Hribar M, Benedik E, Siuka D, Jerin A, Čegovnik Primožič U, Fabjan T, Kumer K, Pravst I and Žmitek K (2024) Seasonal variation of total and bioavailable 25-hydroxyvitamin D [25(OH)D] in the healthy adult Slovenian population. Acta Biochim. Pol 71:13108. doi: 10.3389/abp.2024.13108
Received: 08 April 2024; Accepted: 29 July 2024;
Published: 11 September 2024.
Edited by:
Grzegorz Wegrzyn, University of Gdansk, PolandReviewed by:
Michal A. Zmijewski, Medical University of Gdansk, PolandMarta Karaźniewicz-Łada, Poznan University of Medical Sciences, Poland
Copyright © 2024 Osredkar, Vičič, Hribar, Benedik, Siuka, Jerin, Čegovnik Primožič, Fabjan, Kumer, Pravst and Žmitek. This is an open-access article distributed under the terms of the Creative Commons Attribution License (CC BY). The use, distribution or reproduction in other forums is permitted, provided the original author(s) and the copyright owner(s) are credited and that the original publication in this journal is cited, in accordance with accepted academic practice. No use, distribution or reproduction is permitted which does not comply with these terms.
*Correspondence: Joško Osredkar, josko.osredkar@kclj.si
†These authors have contributed equally to this work