TaqMan probe-based one-step multiplex real-time RT-PCR assay for the diagnosis of foot-and-mouth disease
- ICAR-Directorate of Foot-and-Mouth Disease, Internal Centre for Foot-and-Mouth Disease (ICFMD), Bhubaneswar, Odisha, India
Effective control and monitoring the spread of foot-and-mouth disease (FMD) relies upon rapid and accurate laboratory detection of FMD virus (FMDV). Therefore, in this report, a multiplex TaqMan probe-based one-step RT-qPCR assay simultaneously targeting FMDV 5′UTR and 3Dpol regions, and 18S rRNA housekeeping gene (as an internal control) in a single reaction tube was developed and evaluated. The multiplex one-step RT-qPCR assay specifically detected viral genome in both FMDV-infected cell culture suspensions and clinical samples collected from known-FMD infected animals. The assay could detect FMDV RNA in the archived FMDV cell culture isolates (n = 120) collected during the last two decades in India. In addition, the new assay could also detect viral RNA in the FMD suspected clinical samples (n = 740) collected from various field outbreaks. At a cut-off Ct-value of <38, the assay could detect at least 20 and 10 copies of FMDV 3Dpol and 5′UTR genes, respectively. Further, the multiplex RT-qPCR assay proved to be robust, showing an inter-assay co-efficient of variations ranging from 1.3% to 3.03% for FMDV-3Dpol gene target, and from 1.44% to 4.69% for 5′UTR gene target. In addition, it was found that the new assay could be used to detect viral genome in a variety of samples (epithelium, saliva, OPF, milk and blood) without any significance difference in the detection limit of the assay. Hence, the multiplex one-step RT-qPCR assay could be considered a valuable tool for the detection of FMDV in India.
Introduction
Foot-and-mouth disease (FMD) is a contagious, vesicular-viral disease of both domesticated and certain wild-life species. Although the mortality due to FMD is generally low, the disease affects a large number of animals of different species leading to a heavy economic loss and reduced livestock productivity in the endemic countries of Asia and African continents (Biswal et al., 2012). Since, collectively three-quarters of world’s livestock population is concentrated in the FMD-endemic areas (Knight-Jones and Rushton, 2013), the disease can seriously impact the world’s food and nutritional security. In addition, the transboundary spread of FMD from the endemic to the disease-free countries can pose significant risk to the livestock industries. The etiological agent of the disease, FMD virus (FMDV), has a single-stranded, positive-sense genomic RNA of about 8.4 kb size. FMDV belongs to the genus Aphthovirus within the Picornaviridae family, and there are seven immunologically distinct serotypes such as O,A,C, Asia1, SAT-1, SAT-2 and SAT-3 (Belsham, 1993). Each serotype of FMDV contains multiple variants and the variants are often restricted to specific geographic regions, however, the global distribution of FMDV serotypes is uneven (Jamal and Belsham, 2013). In India, serotypes O, A and Asia1 are currently circulating with a dominance of FMDV serotype O (Subramaniam et al., 2013; Subramaniam et al., 2022).
Clinical diagnosis of FMD can be made based on symptoms of fever, lameness, ropy-salivation, anorexia, and formation of vesicles and epithelial erosions in the tongue, oral-cavity, foot, udder and coronary band of the affected animals (Grubman and Baxt, 2004). However, since FMD is highly contagious and has clinical signs similar to the other vesicular and look-alike diseases, a quick confirmatory laboratory diagnosis is extremely important (Biswal et al., 2022). A definitive diagnosis of FMD is based on the detection of the virus in the clinical samples from the affected animals. Although virus isolation was considered longtime as the gold standard to detect FMD virus, it is labor-intensive, time-consuming and requires high-containment laboratory facility (Sharma et al., 2015). Detection of FMDV antigen through antigen-ELISA is a faster approach to virus-isolation, however, the assay suffers from low sensitivity (Remond et al., 2002). Therefore, faster diagnostic methods based on the amplification of the specific sequence of the FMDV-genome by RT-PCR assay have been developed by several researchers (Reid et al., 1999; Reid et al., 2001; Giridharan et al., 2005). The RT-PCR assays can be applied to different kind of clinical samples, and the assay can identify FMDV-infected animals even before the manifestation of the clinical signs. However, the conventional agarose-gel electrophoresis-based RT-PCR does not have optimal results in terms of specificity and sensitivity (Alexandersen et al., 2003). In addition, the viral RNA amplified by the conventional RT-PCR is usually analyzed by agarose-gel electrophoresis which is laborious, time-consuming, and assay interpretation is subjective in nature (Biswal et al., 2022). Therefore, fluorogenic TaqMan probe-based real-time RT-PCRs (RT-qPCR) targeting the conserved 5′UTR-internal ribosome entry site (IRES) and viral RNA-polymerase (3Dpol) coding sequences have been developed and evaluated for pan-serotype detection of FMDV in a variety of sample matrices (Callahan et al., 2002; Reid et al., 2002; Shaw et al., 2007; Reid et al., 2009).
Due to the high level of nucleotide mutations generated during the replication of FMDV, nucleotide substitution(s) either in the primer or probe binding regions may result in negative signal in one RT-qPCR assay, while the other RT-qPCR assay could still gives the positive result. However, an RT-qPCR assay targeting multiple genetic regions could enhance the overall sensitivity of nucleic acid detection for the diagnosis of FMDV (King et al., 2006). Therefore, we have developed a multiplex TaqMan probe-based one-step RT-qPCR assay simultaneously targeting the 5′UTR and 3Dpol regions, and endogenous 18S rRNA gene as internal control (IC). The newly developed multiplex RT-qPCR assay was also evaluated for the detection of FMDV serotypes O, A and Asia1 strains circulating in India in a variety of clinical sample matrices from the FMDV affected animals.
Materials and methods
Virus isolates and clinical samples
FMDV serotypes O, A, and Asia1 isolates available in the virus repository of ICAR-Directorate of Foot-and-Mouth Disease (ICAR-DFMD) as infected cell-culture supernatant (n = 120) were used in this study. A 10% PBS-suspension of tongue/feet epithelial samples (n = 740) from suspected FMDV infected animals from various parts of India were also used in this study. In addition, oro-pharyngeal fluids (n = 210) (OPF), oral-swabs/nasal-swabs (n = 75), and milk samples (n = 48) from FMDV-suspected animals were also used in developing the assay.
RNA extraction
The viral nucleic acid from the above samples was extracted using QIAamp Viral RNA Mini Kit (Qiagen, Germany) as per the manufacturer’s instructions. Following the extraction, the viral RNA was stored at −80°C until further analyses.
RT-qPCR
The primers and probes sequences used in two earlier reported pan-FMDV serotype RT-qPCR assays targeting the FMDV 3D-polymerase (3Dpol) coding sequence (Callahan et al., 2002) and FMDV 5′UTR (Reid et al., 2002) were used in this study. The details of the primers and probes used in this study has been mentioned in the Table 1. Initially, the two RT-qPCR assays were used in parallel for the amplification of FMDV genomic region. Subsequently, the primers and probes of 3Dpol and 5′UTR region were combined together in a single tube along with primer and probe of 18S rRNA housekeeping gene to develop the multiplex pan-FMDV serotype RT-qPCR. The conserved region in18S rRNA housekeeping gene-sequence shared among multiple FMDV-susceptible animal species (cattle, sheep, goat, and pigs) was used as an internal control for amplification in the multiplex RT-qPCR to determine the quality of clinical samples (Bai et al., 2018; Wang et al., 2020).
The multiplex RT-qPCR assay was performed in a one-step format using the AgPath-IDTM One-step RT-PCR reagents (Applied Biosystems). Briefly, 5 µL of extracted RNA was amplified in a reaction mixture consisting of 12.5 µL 2X RT-PCR buffer, 1 µL of 25X RT-PCR enzyme mix, 400 nM each of 3Dpol forward and reverse primers, and 120 nM of 3Dpol-probe, 200 nM each of 5′UTR forward and reverse primers, and 60 nM of 5′UTR-probe, 100 nM each of 18S specific forward and reverse primers, and 50 nm of 18S-probe. The final volume of the reaction mixture was adjusted up to 25 µL with nuclease-free water. The probe targeting the 3Dpol was labelled with 5′HEX and 3′BHQ-1, the probe targeting the 5′UTR was labelled with 5′-FAM and 3′-TAMRA, and the probe targeting the 18S rRNA gene was labelled with 5′Cy5 and 3′BHQ-2 (Eurofins Scientific). The parameters for RT-qPCR reaction condition start with reverse transcription (RT) step at 45°C for 10 min, a RT-inactivation step at 95°C for 10 min, followed by 45 cycles of denaturation at 95°C for 15 s, and annealing/extension at 60°C for 45 s. The multiplex RT-qPCR assay was performed with CFX96 Touch Real-Time PCR detection system (Bio-Rad). The fluorescence emitted by the HEX, FAM and Cy5 dyes was measured simultaneously and independently at the end of annealing/extension step. The result was expressed as cycle threshold (Ct-value), which is defined as the cycle number at which statistically significant fluorescent intensity was generated by the qPCR assay. The Ct-value was determined under the default settings for the real-time PCR detection software.
Analytical sensitivity of one-step multiplex RT-qPCR
To determine the analytical sensitivity of the multiplex RT-qPCR assay, full-length FMDV RNA was synthesized from FMDV serotype O full-genome length cDNA plasmid (Biswal et al., 2015) using the HiScribe T7 High Yield RNA synthesis kit (NEB). Following DNase treatment and column purification, the concentration of resuspended full-genome length viral RNA was measured using the nano-spectrophotometer (NanoDropTM 2000). The in vitro transcribed full-length FMDV RNA was diluted in Tris-EDTA buffer as 10-fold serial dilutions, and triplicates of each serial dilution of viral RNA was analyzed in the multiplex RT-qPCR. The obtained Ct-values were plotted against each serial dilution of viral RNA to determine the standard curves. The amplification efficiency of the new assay was determined from the standard curve using the formula, E% = 10−1/slope×100. The concentration of synthetic FMDV RNA-standard in each serial dilution was converted into RNA-copy number as described earlier (Huang et al., 2009) to determine detection limit of the assay.
Analytical specificity and reproducibility
The specificity of the assay was evaluated using FMDV serotypes O, A and Asia1infected cell culture supernatants (n = 120). Total RNA extracted from saliva (n = 25), nasal swab (n = 30), blood (n = 20) and milk samples (n = 40) from apparently healthy animals with no history of recent FMD outbreak, and found negative for FMDV-specific NSP-antibodies (Mohapatra et al., 2011), were analyzed using the one-step multiplex RT-qPCR. In addition, nucleic acids extracted from the vaccine strain of PPR virus and Goatpox virus were also analyzed.
The variability of the multiplex RT-qPCR was determined using five-different ten-fold dilution series of viral RNA extracted from known positive FMDV-clinical sample. For intra-assay repeatability, each dilution of viral RNA was analyzed in triplicate within a single plate, while for inter-assay repeatability, each dilution of viral RNA was analyzed in three different reactions performed on three different days. The mean, standard deviation (SD) and co-efficient of variance (CV) of the Ct-value from the intra- and inter-assay runs were calculated separately.
Sample matrix effect
To determine the effect of sample matrices on the detection limit of the one-step multiplex RT-qPCR, six different FMDV-negative sample matrices were used. These includes BHK-21 cell culture sample, bovine EDTA-blood samples, healthy goat-tongue (from abattoir) epithelial suspension, saliva samples, milk samples, bovine oro-pharyngeal fluid (OPF) samples. These samples were collected from animals Which Are found negative for FMDV NSP-antibodies. Each sample matrix was spiked with 1:10 serial dilution series of FMDV serotype O field isolate (FMDV IC18/2019). Viral RNA was extracted from spiked sample matrices and multiplex RT-qPCR was conducted on the extracted RNA for three times. The mean Ct-value was calculated.
Detection of FMDV RNA in the field samples by one-step multiplex RT-qPCR
RNA extracted from FMDV-suspected clinical (tongue/feet epithelial) samples (n = 740), bovine OPF (n = 210), milk-samples (n = 48) from FMDV-infected animals, and oral/nasal swab-samples (n = 75) which included before on-set of clinical signs of FMD (n = 20), during FMDV infection (n = 40), and after the completion of clinical course of FMD (n = 15) were tested by the new multiplex RT-qPCR assay.
Results
Analytical sensitivity of one-step multiplex RT-qPCR (RT-qPCR) assay
Analytical sensitivity of the multiplex RT-qPCR assay which simultaneously amplify the 5′UTR and 3Dpol genes of FMDV was analyzed using standard curve generated using three replicates of ten-fold serial dilutions of in vitro transcribed FMDV RNA and by plotting the Ct-value against the viral copies number. In addition, the same dilution series of in vitro transcribed RNA was also analyzed in the uniplex RT-qPCR format (targeting either 5′UTR or 3D pol genes) to determine the comparative analytical sensitivity between the uniplex and multiplex RT-qPCR assays. For the 3Dpol gene, the efficiencies were 103.09% and 101% for the multiplex and uniplex RT-qPCR respectively, (Figure 1A). Similarly, for the 5′UTR gene, the PCR efficiencies were 91.35% and 89% for the multiplex and uniplex formats respectively (Figure 1B). From the standard curve analyses, at the pre-selected cut-off Ct-value of <38, the limit of detection of the assay was 20 copies/PCR reaction and 10 copies/PCR reaction for the 3Dpol and 5′UTR genes, respectively.
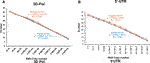
FIGURE 1. Standard curves of probe-based RT-qPCR assay targeting (A) 3Dpol coding sequence of FMDV, and (B) 5′UTR coding sequence of FMDV, either in uniplex (blue-colored) or multiplex (orange-colored) format generated by serial dilutions of FMDV in vitro transcribed viral RNA. The in vitro transcribed viral RNA concentration has been converted into genome copies number. The linear trend lines were depicted as blue-colored and orange-colored dotted lines for uniplex- and multiplex-format RT-qPCR assays respectively.
To determine the analytical sensitivity of the multiplex RT-qPCR assay at the TCID50 level, viral RNA extracted from serial ten-fold dilutions of FMDV serotypes O, A and Asia1 was used in the assay. The standard curves were generated by plotting the Ct-value against the virus titre in terms of TCID50/ml. The analyses showed that at the cut-off Ct-value of <38, the detection limits of viral RNA for serotypes O, A and Asia1 were 0.3 TCID50/ml, 0.8 TCID50/ml, and 1.05 TCID50/ml, respectively (Figure 2).
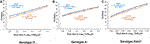
FIGURE 2. Standard curves of one-step multiplex RT-qPCR assay targeting FMDV 3Dpol (orange-colored) and 5′UTR (blue-colored) coding regions generated from the amplification of tenfold serial dilutions of FMDV (A) serotype O, (B) serotype A, and (C) serotype Asia1. The linear trend lines were depicted as blue-colored and orange-colored dotted lines for 5′UTR and 3Dpol targets respectively.
Specificity and reproducibility of one-step multiplex RT-qPCR
The specificity of multiplex RT-qPCR assay was experimentally tested and confirmed with the archived FMDV cell culture isolates (n = 120) belonging to the serotypes O, A and Asia1 circulating in India during the last 20 years (Supplementary Table S1). The specificity of the assay was also determined by testing the saliva (n = 25), nasal swab (n = 30), blood (n = 20) and milk samples (n = 40) from apparently healthy animals that were found negative for FMDV-NSP antibodies. In addition, viral genome extracted from other diseases to be differentially diagnosed such as PPR virus and Goatpox virus were also analyzed using the new assay. The results from the study demonstrated that the assay specifically detects FMDV genome from infected cell-culture supernatant. However, while testing the oro-pharyngeal fluid (OPF) samples from FMDV negative cattle (OPF samples those were found negative for FMD virus isolation during three consecutive rounds of probang collections), it was observed that 4 out of the 102 OPF samples had Ct-value between 38 and 41 in the multiplex RT-qPCR assay for both 3Dpol and 5′UTR targets, while no-signal (no Ct-value) was detected for rest of the OPF samples (98 out of the 102). Even repeating assay after following a very stringent decontamination protocols for pipettes, centrifuges and bio-safety cabinets, we could observe the linear increase in fluorescence in those OPF samples as detected in the earlier runs. Since neither the presence of FMD virus through virus isolation in cell culture, nor any other virus-associated indicators such as infection specific antibodies (NSP-antibodies) could be conclusively demonstrated in these animals (data not shown), a conservative cut-off Ct-value of <38 was used to define the positive samples in the one-step multiplex RT-qPCR assay.
The reproducibility of the multiplex RT-qPCR assay was determined using serial five 10-fold dilutions of FMDV-RNA in triplicate by the intra-and inter-assay assessment. The standard deviation and co-efficient of variance were calculated based on the Ct-values obtained from the replicates. As shown in Table 2, the intra- or inter-assay CV is <5%, indicating the assay is reliable and repeatable, since the CV value < 5% is suggested to be acceptable (Campbell et al., 2010).
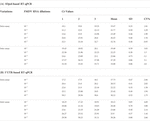
TABLE 2. Repeatability of intra-and inter-assay with different FMDV RNA dilutions by the one-step multiplex RT-qPCR.
Effect of different sample matrices on the detection of FMDV RNA by multiplex RT-qPCR
The effect of different sample matrices on the detection limit of multiplex RT-qPCR for the 3Dpol and 5′UTR gene targets has been depicted in the Figures 3A, B. Compared to the BHK-21 cell-culture-spiked virus control samples, little or no matrix effect of tongue epithelium, saliva, milk, and oro-pharyngeal fluid samples on the detection limit of multiplex RT-qPCR was observed. However, one-log10 reduction in the detection limit was noted for the EDTA-blood sample.
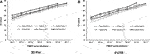
FIGURE 3. The effect different sample matrices on the detection limit of the FMDV as determined by multiplex RT-qPCR assay targeting (A) 3Dpol and (B) 5′UTR. The standard curves were generated by amplification of FMDV from the tenfold serial dilution of virus-spiked sample (BHK-21 cell culture supernatant, tongue epithelium, saliva, milk, OPF and blood) matrices.
Application of one-step multiplex RT-qPCR assay to the FMD suspected field samples
In order to evaluate the performance of the one-step multiplex RT-qPCR assay on the field samples, RNA extracted from a variety of clinical samples were tested (Figure 4). Out of 740 tongue/feet epithelial (TE/FE) samples collected from the FMD-suspected animals, 704 samples were detected positive in the multiplex RT-qPCR. Amongst these epithelial samples, FMDV RNA was detected in 172/172, 298/310 and 234/258 samples collected from infected animals at 1–5 days, 5–10 days, and 10–15 days, respectively post-manifestation of clinical symptoms (Figure 4A). Similarly, out of 210 OPF samples, viral RNA was detected in 26/35, 52/98, and 32/77 samples collected at 1–3 months, 3–6 months, and 6–9 months, respectively post-FMD outbreaks (Figure 4B). Likewise, FMDV RNA was detected in 8/20, 40/40, and 9/15 oral/nasal swab samples collected before manifestation of FMDV-signs, during the clinical course of the disease, and after clinical-recovery from FMD, respectively (Figure 4C). Milk samples collected sequentially from 10 FMDV naturally infected cows were also analyzed by multiplex RT-qPCR, and it was determined that 10/10,10/10, 7/10, 5/9, and 3/9 cattle were found positive for viral RNA in the milk samples collected at 2 days-post manifestation of infection (DPI), 7DPI, 14 DPI, 21 DPI, and 28 DPI, respectively (Figure 4D). Furthermore, in all the field samples tested, both the 3Dpol and 5′UTR targets were amplified with comparable Ct-values.
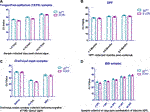
FIGURE 4. Detection of FMDV RNA in various FMD-suspected field samples, (A) tongue/feet epithelium (TE/FE), (B) oro-pharyngeal fluids (OPF) (C) oral/nasal swabs, and (D) milk samples, by one-step multiplex RT-qPCR assay.
Discussion
Nucleic acid recognition techniques such as agarose gel-based RT-PCR and real-time RT-PCRs, are replacing conventional techniques such as virus-isolation and antigen-ELISA for diagnosis of FMDV owing to the ease of automation and rapidity of results determination through these assays (Belak, 2007a; Goris et al., 2009). It has been reported that the analytical sensitivity of real-time RT-PCR exceeds that of the virus-isolation (Moonen et al., 2003), antigen-ELISA (Reid et al., 2002), and conventional agarose-gel-based RT-PCR(Hearps et al., 2002) without compromising the analytical specificity to differentially diagnose FMDV from other look-alike diseases (Callahan et al., 2002; Oem et al., 2005). In addition, earlier investigators have also observed that, if necessary precautionary measures were adopted to avoid cross-contamination, 100% diagnostic specificity could be obtained while using the real-time RT-PCR for the diagnosis of FMDV (Moonen et al., 2003). Compared to the single-plex real-time RT-PCR, the multiplex RT-PCR assays have the advantage of detecting multiple targets in a single assay platform thereby reducing the cost, time and sample resources (Laamiri et al., 2018). Therefore, in this study, a multiplex real-time RT-PCR was developed by simultaneously targeting two conserved genetic regions of FMD virus along with an endogenous house-keeping gene (18S rRNA) for the pan-serotype detection of FMDV circulating in India.
The World Organization for Animal Health (WOAH)-approved primers and 5′-nuclease probes targeting the inter-serotype conserved 3Dpol and IRES-regions of FMDV were used for the development of multiplex real-time RT-PCR. In addition, sequence of the 18S rRNA housekeeping gene that was conserved and can be amplified from multiple cloven-hoofed animal species was used as an internal control in the multiplex PCR. Since the quality of clinical samples received at the FMDV-diagnostic laboratories varies, and the detection of FMD virus/genome in the clinical samples is impacted to a great extent by the sample quality, inclusion of the 18S rRNA detecting primers and probes as an internal control would ensure no false negative reporting due to the deterioration of clinical samples rather than absence of viral genome. In our study it was observed that multiplexing the 3Dpol and IRES primers/probes did not affect the amplification efficiency of the viral genome, since a good correlation was observed between the uniplex and multiplex formats. In fact, the PCR-amplification efficiencies of 3Dpol and IRES coding sequences were marginally higher in the multiplex format compared to their respective uniplex format. Such kind of increased amplification efficiency for FMDV 3Dpol region when multiplexed with the 5′UTR region of Seneca Valley virus (SVV-1) has already been reported earlier (Wang et al., 2020). Although the possible reason for the increased amplification efficiency under multiplexed condition is not known, denaturation and stabilization of the secondary structural complex formed by interaction between viral genome and PCR-primers may explain the increased efficiency to some extent (Vandenbussche et al., 2017; Wang et al., 2020). At the selected cut-off Ct-value of<38, up to 20 and 10 copies of FMDV RNA were detected by 3Dpol and 5′UTR-based primer-probes respectively, in the multiplex RT-qPCR format. Furthermore, the detection limit of multiplex RT-qPCR varied from 1.05 to 1.8 log10 TCID50/ml per reaction for different FMDV serotypes. Therefore, the assay can be expected to accurately detect over a wide concentration range of FMDV in the suspected clinical samples. In addition, the low co-efficient of variation as determined from the inter-and intra-assay comparison suggested the multiplex RT-qPCR to be repeatable and reproducible with low day-to-day or operator-to-operator variations.
Since the viral genome detection through the RT-qPCR assay relies mainly on the oligonucleotides primers and probes that target the conserved region of the viral genome, the assay is prone to produce false negative result in the event of nucleotide substitutions in the complementary target region (Belak, 2007b; Tam et al., 2009). Therefore, to determine the diagnostic specificity and robustness of the one-step multiplex RT-qPCR assay, the viral RNA extracted from different lineages/genotypes of FMDV serotype O, A and Asia1 that have been prevalent in India during the last 20-years were analyzed. All these archival isolates could be detected positive by both the 3D pol and 5′UTR specific RT-qPCR assay in the multiplex format. Therefore, the multiplex RT-qPCR assay could be used for the pan-serotype specific detection of FMDV even in the event of emergence and re-emergence of various lineages of FMDV in India. In addition, owing to the pan-serotypic nature, the assay may add value in detection of FMDV serotypes which are not present in this region.
From the data obtained using five different FMDV-spiked sample matrices, a one-log10 reduction in the detection limit was noted for the EDTA-blood sample. The comparative lower sensitivity of multiplex RT-qPCR for the detection of FMDV genome in the EDTA-blood samples could be due the presence of PCR-inhibitors in the blood samples (Akane et al., 1994; Abu Al-Soud and Radstrom, 2000). Nevertheless, the effect of sample matrices on the detection of viral genome through RT-qPCR assay should be ideally performed using the known field samples or experimental samples, instead of using FMDV-spiked materials. However, both in cases of the experimental and field samples, it would be difficult to ensure the equal concentration of virus in various clinical sample matrices in order to determine a comparative analytical sensitivity. Through the analyses of the effect of sample matrices on the detection of viral genome, it could be suggested that the multiplex RT-qPCR could be used for the detection of FMDV genome in a variety of samples (epithelial, saliva, OPF, milk and blood) collected from the infected/FMD-suspected animals.
Subsequent to success with the virus-spiked various sample-matrices, the multiplex RT-qPCR was implemented on a variety of FMD suspected field samples. While analyzing tongue epithelial/feet epithelial field samples (n = 740) collected on various days after the appearance of gross lesion, it was observed that the assay was 100% sensitive in detection of viral genome in the samples collected during early phase of infection, and the sensitivity was decreased to 90.69% for samples collected approximately after 2-weeks post appearance of clinical symptoms. This could be due to the non-viability or clearance of FMDV in the old lesions. It is worthy to mention that the likelihood of virus isolation can be extremely reduced in the samples collected after 7–10 days after the appearance of clinical lesions (Alexandersen et al., 2003). Nevertheless, the analyses suggested that the new assay could be used for the detection of viral genome in the field up to 3 weeks of FMDV outbreak and could be used for active surveillance purpose as well with a probability of environmental surveillance. Similarly, 8 out of the 20 oral/nasal swab samples collected from animals before the manifestation of clinical Signs of FMD were found positive for the viral genome in the multiplex RT-qPCR. Therefore, the assay could be used for the screening of the sick animals before the manifestation of FMDV-clinical symptoms so that necessary quarantine and biosecurity measures could be undertaken to prevent the spread of FMD in any dairy herd. While analyzing the milk samples collected sequentially from the FMDV-infected cattle, it was observed that 3 out of the 9 milk samples were detected positive for viral genome at 28 days post manifestation of clinical symptoms. Hence, the assay could be used in active surveillance of viral genome through the pooled bulk or individual milk sampling approach as suggested by earlier investigators (Armson et al., 2018; Armson et al., 2020). Similarly, analyses of OPF samples from the naturally infected animals suggest that the multiplex RT-qPCR assay could also be used for the identification of FMDV-persistently infected animals through the detection of viral genome in the OPF samples. Altogether, analyses of various clinical samples collected from the field condition suggested that the multiplex RT-qPCR assay could provide several advantages over the traditional agarose gel-based PCR such as increased laboratory throughput, real-time detection of viral genome without post-PCR processing, and the ease and speed of the virus diagnosis. Furthermore, the assay is less prone to carry-over contamination, since it can be performed as a one-step format in a closed tube. It is worthy to mention that while analyzing FMD-suspected clinical samples, some of the samples produced poor amplification curve lacking the sigmoidal pattern, and those samples were considered negative.
In conclusion, a TaqMan-probe-based one-step multiplex RT-qPCR targeting the 3Dpol and 5′UTR-region of FMDV for the detection of viral genome was developed in this study. The assay was proven to be rapid, efficient, specific and reproducible for the detection of FMDV genome in a variety of clinical samples collected from the FMDV-affected/suspected species. Furthermore, the addition of the 18S rRNA target in the multiplex RT-qPCR, would confirm false negative results owing to the RNA degradation due to badly preserved samples, operator mistakes, reagent quality and flaws with the PCR-instrument. Although, in our current endeavor we have validated the assay by analyzing the samples from Indian origin, owing to the pan-serotypic nature of the primers/probes encoding the 3Dpol/IRES region, we are confident that this assay would also be useful for the detection of FMDV genome in the samples from international origin. Therefore, the new multiplex pan-serotypic RT-qPCR would be helpful for the effective management of FMDV by providing rapid and accurate diagnosis of the virus, and can enhance the diagnostic efficacy in case of low prevalence of the disease owing to the on-going FMD control programme in India.
Data availability statement
The original contributions presented in the study are included in the article/Supplementary Material, further inquiries can be directed to the corresponding author.
Author contributions
JB, JM, and RS: Wrote the Manuscript; JB, JM, and RS: Concept development and experimental design; JB, RR, MR, and SD: Sample processed and Experiment conducted; All authors contributed to the article and approved the submitted version.
Funding
This work is supported by ICAR-Directorate of Foot-and-mouth disease under the institutional project ANSC/DFMD/S/I/L/2021/001/00144.
Acknowledgments
The authors thank the Director, ICAR-DFMD for facilitating the work.
Conflict of interest
The authors declare that the research was conducted in the absence of any commercial or financial relationships that could be construed as a potential conflict of interest.
Supplementary Material
The Supplementary Material for this article can be found online at: https://www.frontierspartnerships.org/articles/10.3389/av.2023.12075/full#supplementary-material
References
Abu Al-Soud, W., and Radstrom, P. (2000). Effects of amplification facilitators on diagnostic PCR in the presence of blood, feces, and meat. J. Clin. Microbiol. 38, 4463–4470. doi:10.1128/jcm.38.12.4463-4470.2000
Akane, A., Matsubara, K., Nakamura, H., Takahashi, S., and Kimura, K. (1994). Identification of the heme compound copurified with deoxyribonucleic acid (DNA) from bloodstains, a major inhibitor of polymerase chain reaction (PCR) amplification. J. Forensic Sci. 39, 13607J–13672J. doi:10.1520/jfs13607j
Alexandersen, S., Zhang, Z., Donaldson, A. I., and Garland, A. J. (2003). The pathogenesis and diagnosis of foot-and-mouth disease. J. Comp. Pathol. 129, 1–36. doi:10.1016/s0021-9975(03)00041-0
Armson, B., Gubbins, S., Mioulet, V., Qasim, I. A., King, D. P., and Lyons, N. A. (2020). Foot-and-Mouth disease surveillance using pooled milk on a large-scale dairy farm in an endemic setting. Front. Vet. Sci. 7, 264. doi:10.3389/fvets.2020.00264
Armson, B., Mioulet, V., Doel, C., Madi, M., Parida, S., Lemire, K. A., et al. (2018). Detection of foot-and-mouth disease virus in milk samples by real-time reverse transcription polymerase chain reaction: optimisation and evaluation of a high-throughput screening method with potential for disease surveillance. Vet. Microbiol. 223, 189–194. doi:10.1016/j.vetmic.2018.07.024
Bai, J., Trinetta, V., Shi, X., Noll, L. W., Magossi, G., Zheng, W., et al. (2018). A multiplex real-time PCR assay, based on invA and pagC genes, for the detection and quantification of Salmonella enterica from cattle lymph nodes. J. Microbiol. Methods 148, 110–116. doi:10.1016/j.mimet.2018.03.019
Belak, S. (2007a). Experiences of an OIE collaborating centre in molecular diagnosis of transboundary animal diseases: A review. Dev. Biol. (Basel) 128, 103–112.
Belak, S. (2007b). Molecular diagnosis of viral diseases, present trends and future aspects A view from the OIE collaborating centre for the application of polymerase chain reaction methods for diagnosis of viral diseases in veterinary medicine. Vaccine 25, 5444–5452. doi:10.1016/j.vaccine.2006.11.068
Belsham, G. J. (1993). Distinctive features of foot-and-mouth disease virus, a member of the picornavirus family; aspects of virus protein synthesis, protein processing and structure. Prog. Biophys. Mol. Biol. 60, 241–260. doi:10.1016/0079-6107(93)90016-d
Biswal, J. K., Bisht, P., Subramaniam, S., Ranjan, R., Sharma, G. K., and Pattnaik, B. (2015). Engineering foot-and-mouth disease virus serotype O IND R2/1975 for one-step purification by immobilized metal affinity chromatography. Biologicals 43, 390–398. doi:10.1016/j.biologicals.2015.06.001
Biswal, J. K., Jena, B. R., Ali, S. Z., Ranjan, R., Mohapatra, J. K., and Singh, R. P. (2022). One-step SYBR green-based real-time RT-PCR assay for detection of foot-and-mouth disease virus circulating in India. Virus Genes 58, 113–121. doi:10.1007/s11262-021-01884-3
Biswal, J. K., Sanyal, A., Rodriguez, L. L., Subramaniam, S., Arzt, J., Sharma, G. K., et al. (2012). Foot-and-mouth disease: global status and indian perspective. Indian J. Animal Sci. 82, 109–131.
Callahan, J. D., Brown, F., Osorio, F. A., Sur, J. H., Kramer, E., Long, G. W., et al. (2002). Use of a portable real-time reverse transcriptase-polymerase chain reaction assay for rapid detection of foot-and-mouth disease virus. J. Am. Vet. Med. Assoc. 220, 1636–1642. doi:10.2460/javma.2002.220.1636
Campbell, M. J., Machin, D., and Walters, S. J. (2010). Medical statistics: a textbook for the health sciences. New York: Wiley.
Giridharan, P., Hemadri, D., Tosh, C., Sanyal, A., and Bandyopadhyay, S. K. (2005). Development and evaluation of a multiplex PCR for differentiation of foot-and-mouth disease virus strains native to India. J. Virol. Methods 126, 1–11. doi:10.1016/j.jviromet.2005.01.015
Goris, N., Vandenbussche, F., Herr, C., Villers, J., Van der Stede, Y., and De Clercq, K. (2009). Validation of two real-time RT-PCR methods for foot-and-mouth disease diagnosis: RNA-extraction, matrix effect, uncertainty of measurement and precision. J. Virol. Methods 160, 157–162. doi:10.1016/j.jviromet.2009.05.005
Grubman, M. J., and Baxt, B. (2004). Foot-and-mouth disease. Clin. Microbiol. Rev. 17, 465–493. doi:10.1128/cmr.17.2.465-493.2004
Hearps, A., Zhang, Z., and Alexandersen, S. (2002). Evaluation of the portable Cepheid SmartCycler real-time PCR machine for the rapid diagnosis of foot-and-mouth disease. Vet. Rec. 150, 625–628. doi:10.1136/vr.150.20.625
Huang, Y. L., Pang, V. F., Pan, C. H., Chen, T. H., Jong, M. H., Huang, T. S., et al. (2009). Development of a reverse transcription multiplex real-time PCR for the detection and genotyping of classical swine fever virus. J. Virol. Methods 160, 111–118. doi:10.1016/j.jviromet.2009.04.029
Jamal, S. M., and Belsham, G. J. (2013). Foot-and-mouth disease: past, present and future. Vet. Res. 44, 116. doi:10.1186/1297-9716-44-116
King, D. P., Ferris, N. P., Shaw, A. E., Reid, S. M., Hutchings, G. H., Giuffre, A. C., et al. (2006). Detection of foot-and-mouth disease virus: comparative diagnostic sensitivity of two independent real-time reverse transcription-polymerase chain reaction assays. J. Vet. Diagn Invest. 18, 93–97. doi:10.1177/104063870601800114
Knight-Jones, T. J., and Rushton, J. (2013). The economic impacts of foot and mouth disease - what are they, how big are they and where do they occur? Prev. Vet. Med. 112, 161–173. doi:10.1016/j.prevetmed.2013.07.013
Laamiri, N., Aouini, R., Marnissi, B., Ghram, A., and Hmila, I. (2018). A multiplex real-time RT-PCR for simultaneous detection of four most common avian respiratory viruses. Virology 515, 29–37. doi:10.1016/j.virol.2017.11.021
Mohapatra, J. K., Pandey, L. K., Sanyal, A., and Pattnaik, B. (2011). Recombinant non-structural polyprotein 3AB-based serodiagnostic strategy for FMD surveillance in bovines irrespective of vaccination. J. Virol. Methods 177, 184–192. doi:10.1016/j.jviromet.2011.08.006
Moonen, P., Boonstra, J., van der Honing, R. H., Leendertse, C. B., Jacobs, L., and Dekker, A. (2003). Validation of a LightCycler-based reverse transcription polymerase chain reaction for the detection of foot-and-mouth disease virus. J. Virol. Methods 113, 35–41. doi:10.1016/s0166-0934(03)00220-9
Oem, J. K., Kye, S. J., Lee, K. N., Kim, Y. J., Park, J. Y., Park, J. H., et al. (2005). Development of a Lightcycler-based reverse transcription polymerase chain reaction for the detection of foot-and-mouth disease virus. J. Vet. Sci. 6, 207–212. doi:10.4142/jvs.2005.6.3.207
Reid, S. M., Ebert, K., Bachanek-Bankowska, K., Batten, C., Sanders, A., Wright, C., et al. (2009). Performance of real-time reverse transcription polymerase chain reaction for the detection of foot-and-mouth disease virus during field outbreaks in the United Kingdom in 2007. J. Vet. Diagn Invest. 21, 321–330. doi:10.1177/104063870902100303
Reid, S. M., Ferris, N. P., Hutchings, G. H., De Clercq, K., Newman, B. J., Knowle, N. J., et al. (2001). Diagnosis of foot-and-mouth disease by RT-PCR: use of phylogenetic data to evaluate primers for the typing of viral RNA in clinical samples. Arch. Virol. 146, 2421–2434. doi:10.1007/s007050170012
Reid, S. M., Ferris, N. P., Hutchings, G. H., Zhang, Z., Belsham, G. J., and Alexandersen, S. (2002). Detection of all seven serotypes of foot-and-mouth disease virus by real-time, fluorogenic reverse transcription polymerase chain reaction assay. J. Virol. Methods 105, 67–80. doi:10.1016/s0166-0934(02)00081-2
Reid, S. M., Hutchings, G. H., Ferris, N. P., and De Clercq, K. (1999). Diagnosis of foot-and-mouth disease by RT-PCR: Evaluation of primers for serotypic characterisation of viral RNA in clinical samples. J. Virol. Methods 83, 113–123. doi:10.1016/s0166-0934(99)00113-5
Remond, M., Kaiser, C., and Lebreton, F. (2002). Diagnosis and screening of foot-and-mouth disease. Comp. Immunol. Microbiol. Infect. Dis. 25, 309–320. doi:10.1016/s0147-9571(02)00028-0
Sharma, G. K., Mahajan, S., Matura, R., Subramaniam, S., Ranjan, R., Biswal, J., et al. (2015). Diagnostic assays developed for the control of foot-and-mouth disease in India. World J. Virol. 4, 295–302. doi:10.5501/wjv.v4.i3.295
Shaw, A. E., Reid, S. M., Ebert, K., Hutchings, G. H., Ferris, N. P., and King, D. P. (2007). Implementation of a one-step real-time RT-PCR protocol for diagnosis of foot-and-mouth disease. J. Virol. Methods 143, 81–85. doi:10.1016/j.jviromet.2007.02.009
Subramaniam, S., Mohapatra, J. K., Sahoo, N. R., Sahoo, A. P., Dahiya, S. S., Rout, M., et al. (2022). Foot-and-mouth disease status in India during the second decade of the twenty-first century (2011-2020). Vet. Res. Commun. 46, 1011–1022. doi:10.1007/s11259-022-10010-z
Subramaniam, S., Pattnaik, B., Sanyal, A., Mohapatra, J. K., Pawar, S. S., Sharma, G. K., et al. (2013). Status of foot-and-mouth disease in India. Transbound. Emerg. Dis. 60, 197–203. doi:10.1111/j.1865-1682.2012.01332.x
Tam, S., Clavijo, A., Engelhard, E. K., and Thurmond, M. C. (2009). Fluorescence-based multiplex real-time RT-PCR arrays for the detection and serotype determination of foot-and-mouth disease virus. J. Virol. Methods 161, 183–191. doi:10.1016/j.jviromet.2009.04.033
Vandenbussche, F., Lefebvre, D. J., De Leeuw, I., Van Borm, S., and De Clercq, K. (2017). Laboratory validation of two real-time RT-PCR methods with 5'-tailed primers for an enhanced detection of foot-and-mouth disease virus. J. Virol. Methods 246, 90–94. doi:10.1016/j.jviromet.2017.04.014
Keywords: FMDV, TaqMan probe, multiplex RT-qPCR, 3D polymerase, 5′UTR
Citation: Biswal JK, Mohapatra JK, Ranjan R, Rout M, Dahiya SS and Singh RP (2023) TaqMan probe-based one-step multiplex real-time RT-PCR assay for the diagnosis of foot-and-mouth disease. Acta Virol. 67:12075. doi: 10.3389/av.2023.12075
Received: 01 March 2023; Accepted: 11 September 2023;
Published: 21 November 2023.
Edited by:
Katarina Polcicova, Slovak Academy of Sciences, SlovakiaCopyright © 2023 Biswal, Mohapatra, Ranjan, Rout, Dahiya and Singh. This is an open-access article distributed under the terms of the Creative Commons Attribution License (CC BY). The use, distribution or reproduction in other forums is permitted, provided the original author(s) and the copyright owner(s) are credited and that the original publication in this journal is cited, in accordance with accepted academic practice. No use, distribution or reproduction is permitted which does not comply with these terms.
*Correspondence: Jitendra K. Biswal, jkubiswal@gmail.com