- 1Division of Clinical Review, Office of Safety and Clinical Evaluation, Office of Generic Drugs, Center for Drug Evaluation and Research, U.S. Food and Drug Administration, Silver Spring, MD, United States
- 2York College, The City University of New York, Jamaica, NY, United States
- 3Office of Research and Standards, Office of Generic Drugs, Center for Drug Evaluation and Research, U.S. Food and Drug Administration, Silver Spring, MD, United States
Purpose: This study aims to investigate the potential of Oregon grape root extracts to modulate the activity of P-glycoprotein.
Methods: We performed 3H-CsA or 3H-digoxin transport experiments in the absence or presence of two sources of Oregon grape root extracts (E1 and E2), berberine or berbamine in Caco-2 and MDCKII-MDR1 cells. In addition, real time quantitative polymerase chain reaction (RT-PCR) was performed in Caco-2 and LS-180 cells to investigate the mechanism of modulating P-glycoprotein.
Results: Our results showed that in Caco-2 cells, Oregon grape root extracts (E1 and E2) (0.1–1 mg/mL) inhibited the efflux of CsA and digoxin in a dose-dependent manner. However, 0.05 mg/mL E1 significantly increased the absorption of digoxin. Ten µM berberine and 30 µM berbamine significantly reduced the efflux of CsA, while no measurable effect of berberine was observed with digoxin. In the MDCKII-MDR1 cells, 10 µM berberine and 30 µM berbamine inhibited the efflux of CsA and digoxin. Lastly, in real time RT-PCR study, Oregon grape root extract (0.1 mg/mL) up-regulated mRNA levels of human MDR1 in Caco-2 and LS-180 cells at 24 h.
Conclusion: Our study showed that Oregon grape root extracts modulated P-glycoprotein, thereby may affect the bioavailability of drugs that are substrates of P-glycoprotein.
Introduction
Oregon grape root (Mahonia aquifolia), has synergistic antibacterial, anti-inflammatory, and bile-stimulating properties and is used for chronic eruptions, rashes associated with pustules, and rashes associated with eating fatty foods [1]. Recently, Oregon grape root extract has been shown to effectively treat inflammatory skin diseases, such as psoriasis and eczema [2, 3]. The Oregon grape root extracts are available in both oral and topical dosage forms.
Berberine, a bisbenzylisoquinoline alkaloid, is found in Oregon grape root and has been used for many years in traditional Eastern medicine as an effective treatment of gastroenteritis and diarrhea [4]. Since then, other pharmacological effects have been reported such as antimicrobial [5], antiarrhythmic [6], anticancer [7], anti-inflammatory [8] and antiproliferative effects [9]. Studies have shown that berberine is a weak to moderate inhibitor of CYP1As, CYP2C8, and CYP2E1 [10]. Additionally, berberine not only exhibited time-dependent inhibition of CYP2D6 and CYP3A4 but also showed strong inactivation of CYP2D6 and modest inactivation of CYP3A [10, 11]. Berbamine, another bisbenzylisoquinoline alkaloid, although was reported to be present in Oregon grape root, is not a typical component of Oregon grape root. It is found in other plant species such as Amur barberry (Berberis amurensis). Berbamine is widely used in traditional Chinese medicine as an antiarrhythmic, antihypertensive, anti-inflammatory and anticancer agent [12, 13]. Particularly, berbamine showed antiproliferative effects for melanoma, chronic myeloid leukemia and breast cancer [13]. Previous phenotyping studies indicate that CYP3A4 is the primary enzyme involved in the metabolism of berbamine [13].
In vivo experiments using P-glycoprotein (P-gp)-knock out mice and in vitro studies using both Caco-2 cells and MDR1-transfected MDCK cells suggested berberine is a P-gp substrate [14–17]. P-gp is a member of the ATP-binding cassette (ABC) transporter family, also known as ABCB1 or MDR1 (encoded by ABCB1 or MDR1 gene). In Caco-2 cells, P-gp inhibitors such as verapamil (1 mM), daunomycin (1 mM) and rhodamine123 (1 mM) were able to inhibit the efflux of 20 µM berberine by 100% [18]. Similarly, in the presence of the P-gp inhibitors cyclosporin A (10 µM), the efflux ratios of berberine (10 µM) were significantly reduced to 0.79 and 1.34, respectively [17]. In addition to P-gp, previous studies showed that human organic cation transporter 2 (OCT2, SLC22A2), OCT3 (SLC22A3) and multidrug and toxin extrusion protein 1 (MATE1) mediated the transport of berberine in the kidney [19–22], and OCT1, MATE1, organic anion-transporting polypeptide 1B3 (OATP1B3) mediated transport of berberine in the liver [23, 24]. Meanwhile, berberine is not an OATP1B1 and multidrug resistance-associated protein 2 (MRP2) substrate [17, 23]. Conversely, no significant transport of berberine was observed by bile salt export pump (BSEP), MRP2, MRP3, and sodium/taurocholate co-transporting polypeptide (NTCP) in vitro [25–27].
As an inhibitor for transporters, berberine inhibits breast cancer resistance protein (BCRP), MATE1, MATE2-K, organic anion transporter 1 (OAT1), OAT3, OCT1, OCT2, and OCT3 In vitro [19, 27–30]. There are inconsistent data about whether berberine can inhibit P-gp, OATP1B1, OTAP1B3, or OATP2B1 [17, 26, 27, 31]. Studies have shown that berberine can induce P-gp. Twenty-four hours exposure of berberine up-regulated P-gp expression in human and murine hepatoma cells [32] as well as up-regulated P-gp expression in cultured bovine brain capillary endothelial cells [33]. Jing et al. indicated that P-gp protein was upregulated by berberine treatment (0.1, 0.5 or 2.5 µM up to 48 h) in a dose- and time-dependent manner in Caco-2 cells [34]. Seven day pretreatment of 30 µM berberine increased the efflux of daunomycin in Caco-2 cells by 1.4 fold [18].
Berbamine has, on the other hand, been shown to down-regulate the expression of MDR1 mRNA after 72 h treatment in human erythroleukemic cells [35] as well as modulating multi-drug resistance (MDR) in breast cancer cells [36]. In vitro studies with berbamine have shown an increase in the intracellular concentration of adriamycin and down-regulation of MDR1 mRNA and P-gp levels in a human leukemic cell line, K562/A02 [35] as well as in a human breast cancer cell line, MCF7 [37].
To our best knowledge, there are no studies examine the effects of Oregon grape root extracts on P-gp. Because Oregon grape root extract is known as a source of berberine and not berbamine, we hypothesized that Oregon grape root extracts (e.g., E1 and E2) will have similar effects as berberine in the modulation of P-gp (inhibition or upregulation). Herein, we have conducted studies: i) to confirm the presence of berberine and but not beramine in Oregon grape root extracts; ii) to evaluate the effect of the Oregon grape root extracts on the transport of two well known P-gp substrates, CsA and digoxin, using the Caco-2 drug transport models; and iii) to evaluate the effect of the Oregon grape root extracts on the gene expression of human MDR1 in Caco-2 and LS-180 cells to elucidate the role of PXR in the mechanism of P-gp induction. Additionally, we included berberine and berbamine in our in vitro studies to confirm their interactions with P-gp as a substrate and inhibitor in Caco-2 and MDCK II-MDR1 cells.
Materials and methods
Chemicals
Radiolabeled 3H-cyclosporin A (CsA, 7.0 Ci/mmol, 96.7% purity) and 3H-digoxin (23.5 Ci/mmol, 97% purity) were obtained from Amersham, Inc. (Piscataway, NJ, USA). Berberine chloride, berbamine dihydrochloride, CsA, digoxin, verapamil, levothyroxine, rifampin, sodium pyruvate, L-lactic dehydrogenase (LDH), β-nicotinamide adenine dinucleotide, reduced form (β-NADH), thiazolyl blue tetrazolium bromide and triethylamine (TEA) were purchased from Sigma, Inc. (St. Louis, MO, USA). Fetal bovine serum (FBS) was purchased from Hyclone (Logan, UT, USA). Dulbecco’s Modified Eagle Medium (DMEM) and non-essential amino acids were obtained from Invitrogen Corporation (Grand Island, NY, USA). Penicillin/streptomycin solution was purchased from Mediatech, Inc. (Herndone, VA, USA). The 18s internal standard and MDR1 primers were purchased from Applied Biosystems (Foster City, CA, USA). Solvent acetonitrile was HPLC grade and was purchased from Fisher Scientific (Fair Lawn, NJ, USA). Ammonium acetate was purchased from Mallinckroft Baker Inc. (Paris, KY, USA). Oregon grape root extract 1 was a gift from Oregon’s Wild Harvest (Sandy, OR, USA). Oregon grape root extract 2 was purchased as a root powder from Health Herbs (Philomath, OR, USA). All other reagents were analytical grade.
Oregon grape root extract preparation for the experiments
See the details on the preparation of Oregon grape root extract 1 (E1) (liquid) and Oregon grape root extract 2 (E2) (root powder) for the experiments in the Supplementary Material.
Cell culture
The human intestinal epithelial cell line, LS-180 was purchased from American Type Culture Collection (ATCC) (Manassas, VA, USA) and grown in Minimum Essential Medium supplemented with 10% FBS. The Caco-2 cells were obtained from ATCC. MDCKII-MDR1 and MDCKII wild-type cell lines were a generous gift from Dr. Piet Borst (The Netherlands Cancer Research Institute). Both Caro-2 and MDCKII cells were grown in DMEM, 10% FBS, 0.1 mM non-essential amino acids, and 0.01% penicillin/streptomycin [38,39]. All cells were maintained at 37°C with 5% CO2, and 95% relative humidity. For the transport experiments, cells were seeded, 3.0 × 105cells/well, onto 6-well Transwell® inserts 4.71 cm2 (Corning Costar, Cambridge, MA, USA) and maintained until use on Days 21–25 (Caco-2, passages 23–30) and Days 6–7 (MDCKII-MDR1 and MDCKII wild-type). The integrity of the monolayers was assessed by measuring transepithelial electrical resistance (TEER) using a World Precision Instrument, EVOM (Sarasota, FL, USA) and evaluating 14C-mannitol transport. The average TEER readings minus the background were 625.94 ± 75.47 Ωcm2 (Caco-2), 476.91 ± 101.21 Ωcm2 (MDCKII-MDR1) and 211.52 ± 22.33 Ωcm2 (MDCKII wild-type). Also, permeability studies with 14C-mannitol were performed with the various treatment conditions and the transport rate was less than 1% per hour throughout the entire experiment. The apparent permeability (Papp) of mannitol was 2.09 ± 0.40 × 10-6 cm/s, 2.38 ± 0.13 × 10−6 cm/s, and 2.16 ± 0.72 × 10−6 cm/s for Caco-2, MDCKII-MDR1, and MDCKII wild-type cells, respectively. These data indicate that the cell monolayer was not compromised. For the real time RT-PCR, cells were seeded at 6 × 105 cells/well (Caco-2) and 1 × 106 cells/well (LS-180) onto 6-well Transwell® inserts 4.71 cm2 and grown for 7 (Caco-2) and 6 (LS-180) days.
Chemical exposure
We initiated cytotoxicity experiments with the Caco-2, MDCKII-MDR1 and MDCKII-wild type cells on Day 7 (Caco-2) and Day 4 (MDCKII-MDR1 and MDCKII-wild type) after the initial plating of the cells at 2.5 × 103 cells/well in 48-well plates (Becton Dickinson, Franklin Lakes, NJ, USA). See details of preparation for the cytotoxicity experiments in Supplementary Material.
For the transport experiments, cells grown on Transwell® inserts were exposed to test compounds on 21–25 days (Caco-2) or 6–7 days (MDCKII-MDR1 and MDCKII-wild type). Stock solutions of 10 mM berberine or berbamine were prepared as described above. Stock solutions of 100 mg/mL E1 or E2 were prepared by dissolving the 100 mg E1 or E2 in 25% ethanol. The stock solutions of berberine, berbamine, E1 and E2 were further diluted with HBSS at a pH of 6.8 for the apical (A) compartment and 7.4 for the basolateral (B) compartment to yield final concentrations of 3, 10, 30, and 100 µM berberine and berbamine and 0.05, 0.1, 0.25, 0.5 and 1 mg/mL for E1 and E2. Ethanol (0.15%v/v) was used as the solvent vehicle for CsA and verapamil and DMSO (0.15%v/v) were used for digoxin and berberine. The MDR1 transport inhibitor, 100 µM verapamil, was used as a positive control and no treatment was used as a negative control.
For the real time RT-PCR studies, 6 × 105 cells/well (Caco-2) or 1 × 106 cells/well (LS-180) were grown on Transwell® inserts and exposed to test compounds on Day 7 (Caco-2) or Day 6 (LS-180). Stock solutions of 10 mM levothyroxine or 10 µM rifampin were prepared by dissolving compounds in 50% DMSO with 50% distilled water. The stock solutions of E1, E2, levothyroxine or rifampin were further diluted with serum-free and antibiotic-free medium to yield final concentrations of 0.1 mg/mL and 0.25 mg/mL for E1 and E2 and 100 µM levothyroxine or 10 µM rifampin. The final treatment concentration of ethanol or DMSO was 0.15% (v/v) ethanol or 0.5% (v/v) DMSO/culture medium. Levothyroxine and rifampin were used as positive controls for Caco-2 and LS-180 cells, respectively.
Cytotoxicity assays
Lactate dehydrogenase (LDH) assay and MTT assay
See details of LDH assay and MTT assay methods in the Supplementary Material.
Transport studies
Transport of 3H-CsA and 3H-digoxin (0.5 µM and 1.0 µCi), MDR1 substrates, were performed using transport buffer consisting of HBSS with 10 mM HEPES and 25 mM D-glucose at a pH of 6.8 for the apical (A) compartment and 7.4 for the basolateral (B) compartment. Experiments were performed at 37°C, 5% CO2, and 95% relative humidity. Cells in the Transwell® inserts were washed 3 times with a transport buffer and allowed to equilibrate for 30 min prior to the addition of test compounds. Berberine, berbamine, E1, E2 and verapamil were prepared in a transport buffer at the appropriate pH (pH 6.8 for the A compartment and 7.4 for the B compartment) and allowed to incubate at 37°C for 30 min prior to the start of the experiment. Berberine, berbamine, E1, E2 and verapamil were present in both the A and B chambers during the transport of the MDR1 substrates. To evaluate the efflux mechanism involved with berberine and berbamine, 100 µM of berberine and berbamine were placed in the donor chamber and 100 µL samples were collected in the receiver chamber at 0, 0.5, 1.0, 1.5, 2.0, 2.5, and 3.0 h and analyzed by HPLC. Each experiment was performed in duplicate and repeated for each condition tested. For experiments performed in “non-sink” conditions, 100 µL aliquots were taken from the donor and receiver chambers at the beginning and from the receiver chamber at 0.5, 1.0, 1.5, 2.0, 2.5, and 3.0 h. An equal volume of 100 µL was replaced in the chamber at each sample time point. “Non-sink” conditions were performed when less than 10% of compound is transported across cells during the transport experiment such that a concentration gradient was always present. For the “sink” condition experiments, the entire receiver volume was replaced at each time interval and a 100 µL aliquot was taken from the receiver volume for scintillation counting. A 100 µL sample was taken from the donor chamber at 3 h in order to calculate the mass balance of radioactivity to determine if adsorption to the cell culture transport apparatus had occurred. Each sample was placed in 0.9 mL of scintillation fluor (Cytoscint ES, ICN, Cosa Mesa, CA, USA) and read on a Beckman LS 6500 (Palo Alto, CA, USA) scintillation counter for 3H activity. For berberine and berbamine analysis, 100 µL aliquot was assayed by HPLC.
For the transport of radiolabeled CsA and digoxin, a 100 µL sample was taken from the receiver chamber over the 3 h period as well as the donor chamber at 3 h to determine the mass balance of radioactivity to assess if adsorption to the cell culture transport apparatus occurred. Each radiolabeled sample was placed in 0.9 mL of scintillation fluor (Cytoscint ES, ICN, Cosa Mesa, CA, USA) and read on a Beckman LS 6500 (Palo Alto, CA, USA) scintillation counter for 3H activity.
The apparent permeability (Papp) was calculated using the following equation [40]:
Where A is the surface area of the monolayer (4.71 cm2) and C0 is the initial concentration of radiolabeled probe substrate in the donor compartment. dQ/dt is the slope of the steady-state rate constant. The efflux ratio was determined by dividing the Papp in the B to A direction by the Papp in the A to B direction [41]:
Real time quantitative PCR analysis of MDR1 mRNA
Caco-2 or LS-180 cells were grown on Transwell® inserts, 4.71 cm2 for 7 (Caco-2) and 6 (LS-180) days. The cells were exposed to E1, E2, levothyroxine or rifampin for 24 h and 48 h. Total RNA was isolated by adding 1 mL Trizol® reagent (Gibco-BRL, Carlsbad, CA, USA) to the cells and processed according to the manufacturer’s instructions. The concentration and purity of isolated RNA samples were measured using Quant-iTTM RiboGreen® RNA assay kit (Invitrogen, Eugene, OR, USA). The RNA sample (0.06 µg) was reverse transcribed by an iScriptTM cDNA synthesis kit (Bio-Rad Laboratories, Hercules, CA, USA). Relative quantification of gene expression was performed by iTaqTM SYBR® Green supermix with ROX (Bio-Rad Laboratories) using an ABI Prism 7500 Real Time PCR system (Applied Biosystems, Foster City, CA, USA). Each experiment was performed in duplicate and repeated for each condition tested. The mRNA levels of all genes were normalized using 18s as an internal control. Results are expressed as ratios of MDR1 to 18s expression.
High performance liquid chromatography (HPLC) analysis
See details of the HPLC analysis method in the Supplementary Material.
Liquid chromatography/mass spectrometry (LC/MS) analysis
See details of the LC/MS analysis method in the Supplementary Material.
Statistical analysis
All values are presented as a mean ± standard deviation (SD). The percent of drug transported during the experiment for the various treatments and the Papp values within treatment groups were performed with the one-way analysis of variance (ANOVA) followed by a Dunnett’s multiple comparison post-test which compare all the treatments with the control. Differences between A and B transport were compared using a t-test. A probability of difference less than 0.05 (p < 0.05) was considered to be statistically significant.
Results
Cytotoxicity of berberine, berbamine, Oregon grape root extracts
The results of the cytotoxic data indicate that 150 and 300 µM berberine, 300 µM berbamine, significantly decreased MTT reduction and significantly increased LDH leakage in Caco-2 cells. In the MDCKII-MDR1 and MDCKII wild-type cells, none of the berberine and berbamine concentrations were toxic, except for the 300 µM berberine and berbamine in the MDCKII wild-type cells. Thus, concentrations of 100 µM or less of berberine and berbamine were used in these studies.
For Oregon grape root extract 1 (E1) and Oregon grape root extract 2 (E2) only the 2 mg/mL E1 and E2 concentrations were toxic in the Caco-2 cells. Thus, the highest concentration used for the transport studies was 1 mg/mL for E1 and E2. For the real time RT-PCR pretreatment studies in the Caco-2 and LS-180 cells, 0.25 mg/mL or less was used for the 24 and 48 h exposure conditions. See additional details of the results in the Supplementary Material.
Transepithelial transport of berberine and berbamine
In order to investigate whether carrier-mediated transport is involved in the transepithelial transport of berberine and berbamine, transport of 100 µM berberine and berbamine was evaluated as a function of time in the Caco-2 cells and MDCKII-MDR1cells (Figure 1). Because berberine has been shown to be actively effluxed by the Caco-2 cells [19], the efflux mechanism speculated to be involved in berberine and berbamine transport was evaluated by measuring the permeability and the amount transported across the Caco-2 and MDCKII-MDR1 cells from the B to A and the A to B direction. Figures 1A, B show that the transport of berberine and berbamine was faster from the B to A direction (berbine: 1.45 nmol/min; berbamine: 5.94 nmol/min) than that in the A to B direction (berberine: 0.18 nmol/min; berbamine: 0.39 nmol/min) in Caco-2 cells. Similar results were seen with the MDCKII-MDR1 cells (Figures 1C, D). The Papp of berberine and berbamine from the B to A direction was also significantly greater than the A to B direction in Caco-2 and MDCKII-MDR1 cells (Figure 1). There was approximately a 4-fold increase of Papp observed in the B to A direction (10.4 × 10−6 cm/s) compared with the A to B direction (2.35 × 10−6 cm/s) for 100 µM berberine (Figure 1A) and ∼30-fold increase of Papp in the B to A direction (60.3 × 10−6 cm/s) compared with the A to B direction (2.49 × 10−6 cm/s) for 100 µM berbamine (Figure 1B). This suggests that there is an efflux mechanism involved in the transport of both berberine and berbamine in the Caco-2 cells. In MDCKII-MDR1 cells, there was a greater efflux seen for berberine than in the Caco-2 cells. An approximately 12-fold increase of Papp was observed in the B to A direction (15.6 × 10−6 cm/s) compared with the A to B direction (1.3 × 10−6 cm/s) (Figure 1C). For berbamine, less efflux was seen in MDCKII-MDR1 cells compared with the Caco-2 cells (Figure 1D).
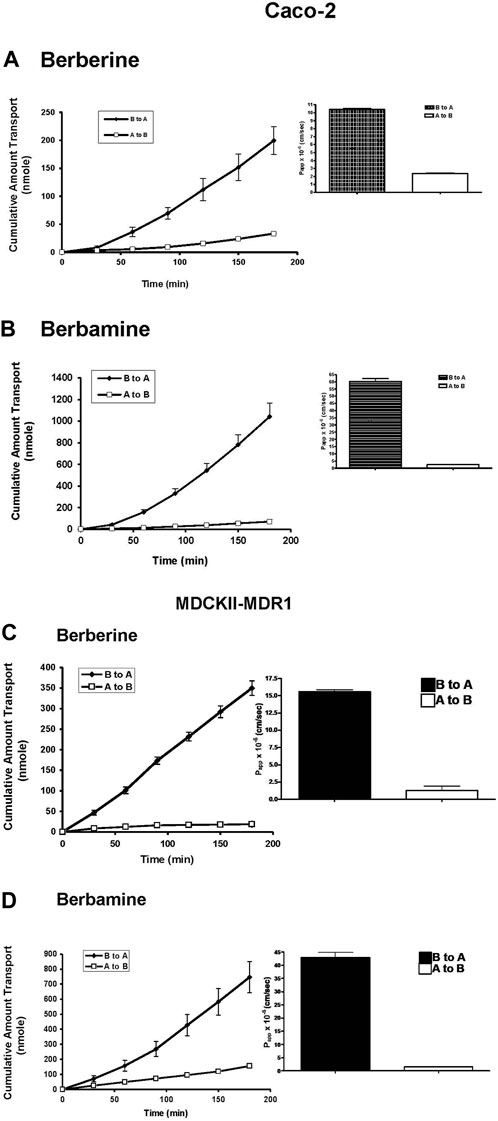
FIGURE 1. Transport of 100 µM berberine (A) or 100 µM berbamine (B) from the apical to the basolateral (A to B, □) and the B to A (♦) across Caco-2 cells, and Transport of 100 µM berberine (C) or 100 µM berbamine (D) from the apical to basolateral (A to B, □) and the B to A (♦) across MDCKII-MDR1 cells. The inset shows the apparent permeability, Papp, of berberine and berbamine from both transport directions in Caco-2 and MDCKII-MDR cells. Data are represented as mean ± standard deviation, n = 4.
Effect of berberine and berbamine on the transport of 3H-CsA and 3H-digoxin
In the Caco-2 cells, the B to A transport of 0.5 µM 3H-CsA was decreased by berberine and berbamine with the significance seen as low as 10 µM for berberine and 30 µM for berbamine (Papp B to A of CsA from 2.83 × 10−6 cm/s to 2.18 × 10−6 cm/s, p < 0.0001) (Figure 2A; Table 1). For digoxin, berbamine significantly inhibited efflux of 0.5 µM 3H-digoxin (Papp B to A of digoxin from 2.78 × 10−6 cm/s to 1.79 × 10−6 cm/s for 30 µM berbamine, p = 0.0253, and to 1.23 × 10−6 cm/s for 100 µM berbamine, p = 0.0021), whereas there were no significant effects of berberine (up to 30 μM) on digoxin transport (Figure 2B; Table 1). In the MDCKII-MDR1 cells, berberine and berbamine significantly decreased the efflux of 0.5 µM 3H-CsA (Papp B to A of CsA from 13.10 × 10−6 cm/s to 5.34 × 10−6 cm/s for 10 µM berberine, p < 0.0001, and to 2.55 × 10−6 cm/s for 30 µM berbamine, p < 0.0001) and the efflux of 0.5 µM 3H-digoxin (Papp B to A of digoxin from 3.30 × 10−6 cm/s to 1.56 × 10−6 cm/s for 10 µM berberine, p = 0.0232, and to 1.71 × 10−6 cm/s for 30 µM berbamine, p = 0.0248) (Table 1). Similar results were seen with the MDCKII wild-type cells compared with that in Caco-2 cells (Table 1).
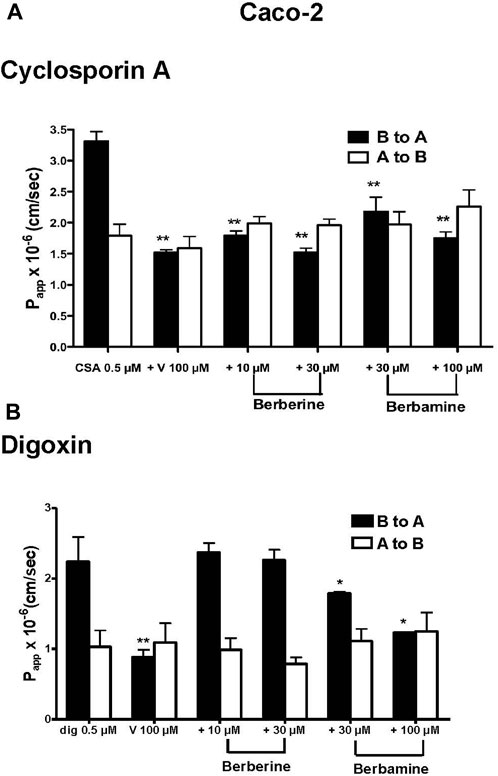
FIGURE 2. Effects of berberine and berbamine on the transport of 0.5 µM 3H-cyclosporin A (CsA, 1 µCi) and 0.5 µM 3H-digoxin (dig, 1 µCi) in Caco-2 cells. Panel (A) Effects of berberine (10 and 30 µM), berbamine (30 and 100 µM) and 100 µM verapamil (V) on the transport of CsA from basolateral the (B) to apical (A) direction and A to B direction. Panel (B) Effects of berberine (10 and 30 µM), berbamine (30 and 100 µM) and 100 µM verapamil on the transport of digoxin from both the B to A and A to B directions. All data represents mean ± standard deviation, n = 4. Statistical significance from 0.5 µM CsA or 0.5 µM digoxin is shown with asterisks, *p < 0.05, **p < 0.01.
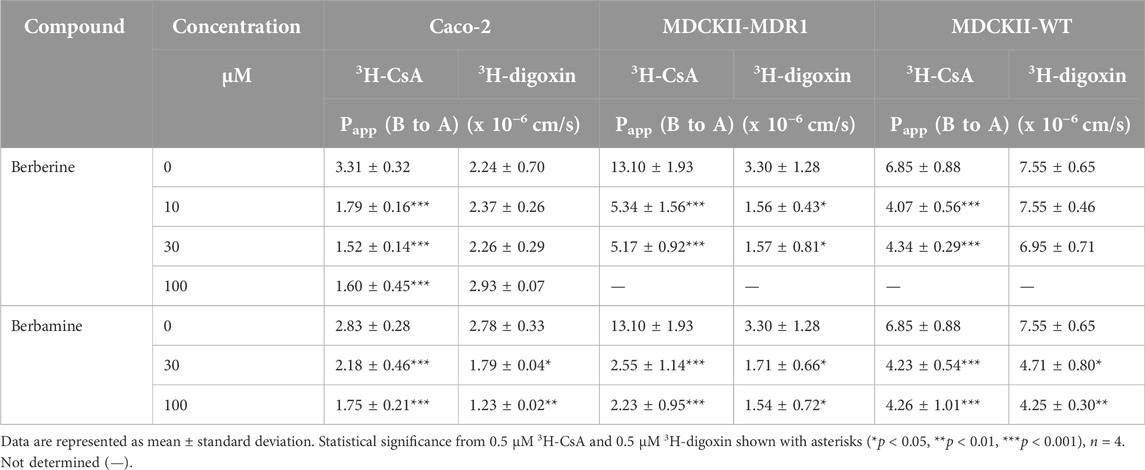
TABLE 1. Effect of berberine and berbamine on apparent permeability (Papp) of 3H-cyclosporine A (CsA) and 3H-digoxin in Caco-2, MDCKII-MDR1 and MDCKII wild-type (MDCKII-WT) cells from basolateral to apical direction.
Effect of Oregon grape root extracts on the transport of 3H- CsA and 3H-digoxin
Table 2 shows the effect of 0.05, 0.1, 0.25, 0.5 and 1 mg/mL E1 or E2 on the transport of 0.5 µM 3H-CsA and 0.5 µM 3H-digoxin in the Caco-2 cells. The B to A transport of 3H- CsA was inhibited by E1 in a dose-response manner with significance effect seen as low as 0.1 mg/mL, p = 0.0439, (Table 2). E2 also inhibited the efflux of 3H-CsA similarly with significance seen at 0.05 mg/mL (p = 0.0007, Table 2). For digoxin, E1 and E2 also inhibited the efflux of 3H-digoxin with significance seen at 0.1 mg/mL for E1 (p < 0.0001), and 0.05 mg/mL for E2 (p < 0.0001). In addition, 0.05 mg/mL E1 significantly increased the absorption of digoxin (p = 0.0005). To compare the effect of E1 and E2 modulating the efflux of CsA and digoxin, efflux ratios of the Papp of B to A and the A to B transport in Caco-2 cells were determined (Table 2). E1 and E2 significantly decreased the efflux ratios of CsA and digoxin. The effect was seen at 0.1 mg/mL for E1 (p = 0.0349) and 0.05 mg/mL for E2 (p < 0.0001) for CsA. For digoxin, significance also occurred at 0.1 mg/mL for E1 (p = 0.0002) and 0.05 mg/mL for E2 (p = 0.0134).
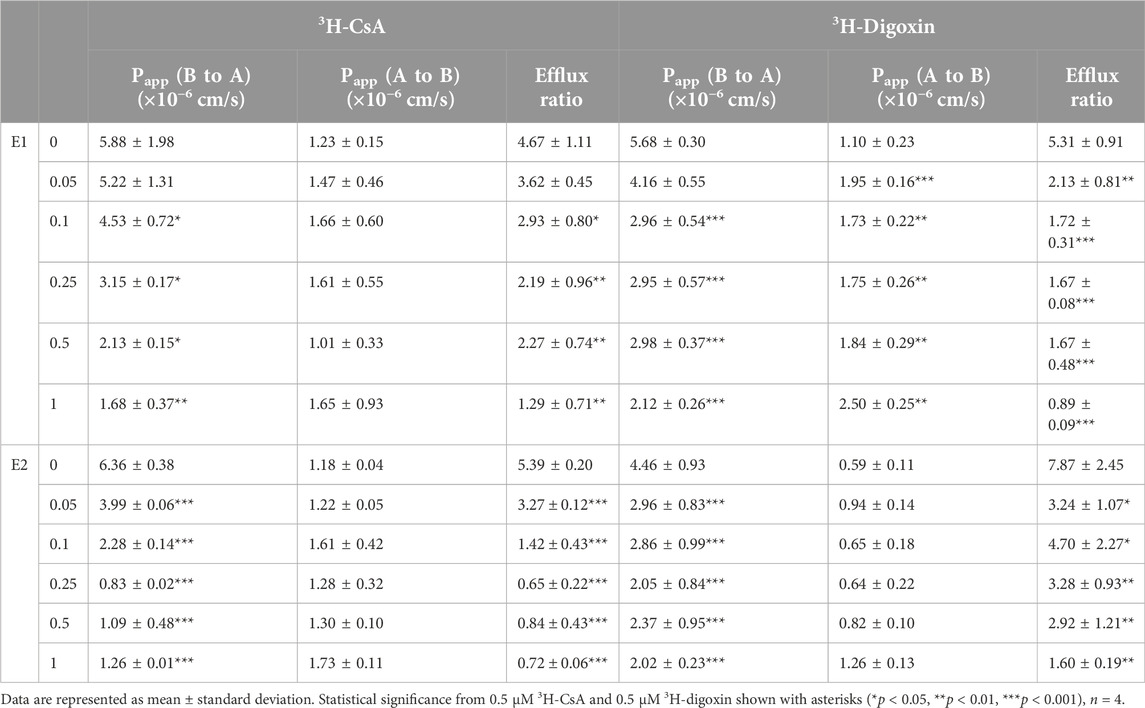
TABLE 2. Comparison of apparent permeability (Papp), and efflux ratio of 3H-cyclosporin A (CsA) and 3H-digoxin with various concentrations of Oregon grape root extract 1 (E1) and Oregon grape root extract 2 (E2) in Caco-2 cells.
HPLC chromatogram and mass spectrometry (MS) of berberine, berbamine and Oregon grape root extracts
It has been reported that Oregon grape root contains berberine. On the other hand, although berbamine was reported to be present in Oregon grape root, it is not a typical component. In order to confirm if it is the case, HPLC was conducted with the Oregon grape root extracts. Berberine was detected in Oregon grape root extracts E1 and E2, but berbamine was not detected in either E1 or E2. See details of the HPLC results in the Supplementary Material.
Induction of P-gp by Oregon grape root extracts
In order to investigate the mechanism of induction of P-gp, real time RT-PCR was used in our study. Real time RT-PCR is routinely used to study low abundance gene expression as well as to elucidate the mechanism of modulation of efflux transport proteins [42]. Exposure of Oregon grape root extracts to Caco-2 and LS-180 cells upregulated the mRNA level of the human MDR1 gene in both cell lines (Figure 3). Levothyroxine, a positive control, upregulated the human MDR1 gene through a PXR-independent manner in the Caco-2 cells [43]. In Figure 3A, 0.25 mg/mL E1 significantly increased the mRNA level of human MDR1 at 48 h in the Caco-2 cells (p = 0.0312) whereas significance was seen at 0.1 mg/mL E2 at 24 h (p < 0.0001). Rifampin was used as the positive control to upregulate the human MDR1 gene through the PXR-dependent pathway in the LS-180 cells [43]. In Figure 3B, 0.1 mg/mL E1 significantly increased the mRNA level of human MDR1 in the LS-180 cells at 48 h (p = 0.0015) while significance was seen with 0.1 mg/mL E2 at 24 h (p = 0.0017) and ∼11-fold increase at 48 h (p < 0.0001). This data suggest that E1 and E2 probably upregulate the MDR1 gene through both the PXR-dependent and PXR-independent pathways.
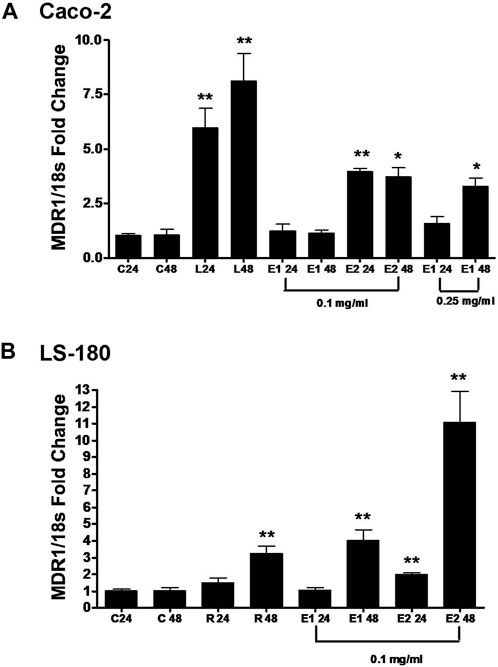
FIGURE 3. Effect of Oregon grape root extract 1 (E1) and Oregon grape root extract 2 (E2) on the mRNA levels of human MDR1 (ABCB1) in Caco-2 cells (Panel (A)) and LS-180 cells (Panel (B)). C: control; 24: 24 h; 48: 48 h; L: 100 µM levothyroxine; and R: 10 µM rifampin. Data are represented as mean ± standard deviation, n = 4. Statistical significance from control is shown with asterisks (treatments at 24 h were compared with the control at 24 h, treatments at 48 h were compared with control at 48 h), *p < 0.05; **p < 0.01.
Discussion
P-gp, encoded by MDR1 gene, has been found in both tumor cells as well as epithelial cells of normal tissues which is responsible for the efflux of a large number of structurally and pharmacologically unrelated lipophilic and amphipathic xenobiotics [44], and it also plays a role in drug resistance of cancer chemotherapy [45]. Clinical herb-drug interactions such as St. John’s wort and sertraline have been reported which alter the efficacy or level of adverse effects of the drug [46]. Herbal products have been shown to interact with drug efflux transporters such as P-gp. Examples of interactions are St. John’s Wort [47, 48], garlic [49, 50], ginko biloba extract [51] and milk thistle [52, 53] as P-gp inhibitors or inducers. These herbal products altered the bioavailability of the drugs which are substrates of P-gp, such as CsA and St. John’s Wort [28], saquinavir and garlic [23], and indinavir and milk thistle [54]. It was hypothesized that inhibition of P-gp by herbal constituents may provide a novel approach for reversing multi-drug resistance in tumor cells whereas increased P-gp expression may have therapeutic implications for chemoprotection by herbal medicines.
Bisbenzylisoquinoline alkaloids, which are found in herbal products like goldenseal (Hydrastis canadensis), berberies and Oregon grape root (Mahonia aquifolia), have been shown to interact with P-gp and have the potential for drug-diet interactions [55, 56]. Fu et al. (2001) screened potential MDR modifiers from naturally occurring bisbenzylisoquinoline alkaloids using MCF-7/ADR and KBv200 tumor cells. Many of the natural compounds tested decreased the resistance of tumor cells to doxorubicin and vincristine, P-gp substrates. In addition, these compounds also increased intracellular accumulation of 3H-vincristine by 3.3-fold [55]. Moreover, PK11195, an isoquinoline carboxamide ligand, has been shown to increase drug accumulation and facilitate drug-induced apoptosis in human multidrug-resistant leukemia cells [56]. This suggests that the mechanism to reverse MDR is probably linked to increased intracellular drug accumulation by inhibition of P-gp. Also, in adriamycin-resistant mouse leukemia P388/ADR cells and human myelogenous leukemia K562/ADR cells, isoquinoline derivatives effectively reversed the resistance to vinblastine by inhibiting 3H-vinblastine efflux by inhibiting the binding of drugs to P-gp [57].
Our study confirms a previous report that berberine is effluxed by the Caco-2 cells and that Papp from B to A is greater than A to B direction [18] (Figure 1A). Moreover, the present study shows that berberine is effluxed by the MDCK-MDR1 cells suggesting that berberine is a P-gp substrate, and efflux observed in the Caco-2 is likely mediated by P-gp (Figure 1C). We also found that berberine can inhibit the P-gp-mediated transport of CsA and digoxin (Figure 2). Berberine (10 µM) significantly inhibited the efflux of 0.5 µM CsA in Caco-2 and MDCK-wild type cells with no effect seen on digoxin transport in these two cell systems (Figure 2; Table 1). Similar transport experiments performed with cells overexpressing P-gp, MDCKII-MDR1, demonstrated that berberine significantly inhibits the Papp efflux of both CsA and digoxin. The differential effect of berberine observed on the transport of digoxin in the Caco-2 and MDCK-wild type cells vs. MDCKII-MDR1 cells are probably due to multiple mechanisms that are differentially expressed in these cell systems. In vivo studies have shown that berberine can alter the bioavailability of P-gp substrates. For instance, the mean area under the curve of CsA was increased 34.5% (p < 0.05) after co-administration of 200 mg berberine three times a day for 12 days in renal-transplant patients [58]). In rats, the intestinal absorption of 50 µM berberine was increased with co-exposure of 5 µM CsA or 200 µM verapamil by 12.3% and 14.7%, respectively [59]. In vitro studies have shown that berberine can up-regulate P-gp expression in gastric (SC-M1 and NUGC-3) and colon (COLO205 and CT26) cancer cell lines after 24 h exposure [32] as well as increase the intracellular accumulation of rhodamine 123, another P-gp substrate, in bovine brain capillary endothelial cells [33].
Our study provides new evidence that berbamine is effluxed in both Caco-2 and MDCKII-MDR1 cells to a greater extent, ∼5-fold, than berberine (Figures 1B, D). The efflux ratio of berbamine is similar in both the Caco-2 cells (24.2 ± 4.5) and MDCKII-MDR1 cells (27.4 ± 5.1) which indicates that berbamine may be transported by additional efflux transporters in Caco-2 cells. Berbamine was able to significantly inhibit the efflux of CsA and digoxin transport in the Caco-2, MDCKII-MDR1 and MDCKII wild-type cells (Table 1). The effect of berbamine on modulating the transport of CsA and digoxin in the B to A direction was not as potent as berberine in the MDCKII-MDR1 cells.
Oregon grape root extracts are commonly used by Native Americans as a dietary supplement for treating skin disease, gall bladder disease and gastro-intestinal disorder [2, 3, 60]. Currently, there are only two studies of identification of compounds present in the Oregon grape root [61, 62]. One analytical study shows the separation of the Oregon grape root extracts by HPLC but does not quantify any of the components within the extract [61]. Another study used capillary-HPLC coupled with ESI MS/MS for the characterization of alkaloids extracted from Oregon grape root [62]. No publication reports any interactions of Oregon grape root with drugs, metabolic pathways or transporters. Our study provides evidence that berberine was present in both of the Oregon grape root extracts (E1 and E2) and that berberine had similar retention times as the berberine standard with 15% berberine in E1 and 17% berberine in E2. As for berbamine, it was not present in either of the extracts which is inconsistent with a previous study [62]. This could probably be due to the difference in the source of the Oregon grape root extracts and the difference of the analytical method. Further confirmation of these compounds needs to be performed by MS/MS and nuclear magnetic resonance spectroscopy analysis. Unlike berberine, berbamine is not a typical component of Oregon grape root. Our results confirmed that berberine was present in Oregon grape root extracts but not berbamine.
The data from our study supported the hypothesis that Organ grape root extracts would have similar effects as berberine in the modulation of P-gp. Our data show that both E1 and E2 inhibited the efflux transport of CsA and digoxin in Caco-2 cells with E2 being slightly more potent than E1 (Table 2). Because we did not detect berbamine in either E1 or E2, the effect observed may mainly reflect the berberine component in the extracts. The different effect of E1, E2 compared to that of berberine on digoxin transport in Caco-2 cells implies that there may be additional components in E1 or E2 (yet to be determined) that may affect digoxin transport.
We further studied if Oregon grape root extracts can induce P-gp. P-gp expression has been shown to be inducible by many xenobiotics such as rifampin [63], dexamethasone [64], and St. John’s wort [65]. In this study, we used both Caco-2 and LS-180 cells as appropriate in vitro models to investigate the mechanism of P-gp induction. Because rifampin can increase P-gp mRNA in LS-180 cells [66], it was used as a positive control in our study. In Caco-2 cells, the positive control, levothyroxine, significantly increase the P-gp mRNA and it is consistent with a previous study [43]. The real time RT-PCR analysis shows that both E1 and E2 up-regulate mRNA levels of human MDR1 in both Caco-2 and LS-180 cell lines (Figure 3). Because Caco-2 cells lack the PXR receptor while LS-180 cells have it, E1 and E2 probably modulate P-gp through both a PXR-independent and a PXR-dependent manner. Because there are other components in either E1 or E2, whether berberine modulate P-gp in both PXR-independent and PXR-dependent manner needs to be further investigated with the purified berberine component. Based on the recommended dosing regimen for Oregon grape root extracts, 750 mg three times a day, intestinal concentrations of Oregon grape root extracts are approximately 3 mg/mL (dose/250 mL) [65]. Thus, the concentrations evaluated in the study (0.1–1 mg/mL) are clinically relevant assuming berberine levels are similar across extracts. This finding is also consistent with previous findings that berberine induces P-gp. Future clinical studies to understand the potential effect of Oregon grape root extract on the PK of P-gp substrates will help determine the in vivo applicability of our in vitro findings.
The limitation of our research is that E1 and E2 were from different resources (see Material and Method section), therefore, it is not clear what the exact components are and what different components were between these two extracts. From our analytical results, it is only confirmed that berberine exists in both extracts. Further investigation is warranted to determine all major components and understand the possible differences between the two extracts.
In summary, to the best of our knowledge, this is the first study to show that Oregon grape root extracts can modulate P-gp by inhibiting P-gp-mediated transport and up-regulating mRNA levels of MDR1, likely caused by berberine component in the extracts. Thus, dietary/herbal supplements, such as Oregon grape root extracts (that contain berberine), may affect the drug efflux or absorption in the intestine which should be taken into consideration when taking drugs that are substrates for P-gp.
Data availability statement
The original contributions presented in the study are included in the article/Supplementary Material, further inquiries can be directed to the corresponding author.
Ethics statement
Ethical approval was not required for the studies on humans in accordance with the local legislation and institutional requirements because only commercially available established cell lines were used.
Author contributions
Conceptualization: YF; Methodology: YF; Conducted experiments: YF; Data analysis and/or interpretation of data for the work: YF, ZZ, and LZ; Wrote or contributed to the writing of the manuscript: YF, ZZ, and LZ; Project administration: YF and ZZ; Funding acquisition: ZZ. All authors have read and agreed to the published version of the manuscript.
Funding
This research was funded by the National Institute of Diabetes and Digestive and Kidney Diseases of the National Institutes of Health under Award Number R21 AT00853A and National Institute of Environmental Health Sciences of the National Institutes of Health under Award Number T35 ES07316, National Institute Of General Medical Sciences of the National Institutes of Health under Award Number R16GM146679 and Medical Research Foundation of Oregon.
Conflict of interest
The authors declare that the research was conducted in the absence of any commercial or financial relationships that could be construed as a potential conflict of interest.
Acknowledgments
We acknowledge Dr. Rosita R. Proteau for her funding support, guidance and supervision (Rosita R. Proteau is currently retired from the Oregon State University and chooses not to be listed as an author); Dr. Ling Zhang for her technical assistance with the LS-MS; Dr. Bingnan Han, Dr. Philip Proteau, Dr. Cristobal Miranda and Dr. Taifo Mahmud for their helpful comments and suggestions. We also gratefully acknowledge Dr. Virginia Lesser for the statistical advice as well as Dr. Piet Borst from The Netherlands Cancer Research Institute for the MDCKII-MDR1 and MDCKII-wild type cells. Part of this work was presented at the Society of Toxicology’s 46th Annual Meeting, Charlotte, NC in March 2007. It is also part of YF’s Dissertation (YF 2007, Effect of pharmaceuticals and natural products on multidrug resistance mediated transport in Caco-2 and MDCKII-MDR1 drug transport models: https://ir.library.oregonstate.edu/concern/graduate_thesis_or_dissertations/8910jx39j).
Author disclaimer
This article reflects the views of the authors and should not be construed to represent FDA’s views and policies. YF and LZ are employed by the U.S. Food and Drug Administration. Their contributions to the article were based on their outside research work that does not reflect any position of the U.S. Food and Drug Administration. The content is solely the responsibility of the authors and does not necessarily represent the official views of the National Institutes of Health either.
Supplementary material
The Supplementary Material for this article can be found online at: https://www.frontierspartnerships.org/articles/10.3389/jpps.2023.11927/full#supplementary-material
References
1. Dattner, AM. From medical herbalism to phytotherapy in dermatology: back to the future. Dermatol Ther (2003) 16(2):106–13. doi:10.1046/j.1529-8019.2003.01618.x
2. Bernstein, S, Donsky, H, Gulliver, W, Hamilton, D, Nobel, S, and Norman, R. Treatment of mild to moderate psoriasis with Relieva, a Mahonia aquifolium extract--a double-blind, placebo-controlled study. Am J Ther (2006) 13(2):121–6. doi:10.1097/00045391-200603000-00007
3. Donsky, H, and Clarke, D. Relieva, a Mahonia aquifolium extract for the treatment of adult patients with atopic dermatitis. Am J Ther (2007) 14(5):442–6. doi:10.1097/mjt.0b013e31814002c1
4. Chopra, BDR, and Chowan, J. Pharmacological action of berberine. Indian J Med Res (1932) 19:1193–203.
5. Kaneda, Y, Torii, M, Tanaka, T, and Aikawa, M. In vitro effects of berberine sulphate on the growth and structure of Entamoeba histolytica, Giardia lamblia and Trichomonas vaginalis. Ann Trop Med Parasitol (1991) 85(4):417–25. doi:10.1080/00034983.1991.11812586
6. Sanchez-Chapula, J. Increase in action potential duration and inhibition of the delayed rectifier outward current Ik by berberine in cat ventricular myocytes. Br J Pharmacol (1996) 117(7):1427–34. doi:10.1111/j.1476-5381.1996.tb15302.x
7. Iizuka, N, Miyamoto, K, Okita, K, Tangoku, A, Hayashi, H, Yosino, S, et al. Inhibitory effect of Coptidis Rhizoma and berberine on the proliferation of human esophageal cancer cell lines. Cancer Lett (2000) 148(1):19–25. doi:10.1016/s0304-3835(99)00264-5
8. Kuo, CL, Chi, CW, and Liu, TY. The anti-inflammatory potential of berberine in vitro and in vivo. Cancer Lett (2004) 203(2):127–37. doi:10.1016/j.canlet.2003.09.002
9. Jantova, S, Cipak, L, and Letasiova, S. Berberine induces apoptosis through a mitochondrial/caspase pathway in human promonocytic U937 cells. Toxicol Vitro (2007) 21(1):25–31. doi:10.1016/j.tiv.2006.07.015
10. McDonald, MG, Tian, DD, Thummel, KE, Paine, MF, and Rettie, AE. Modulation of major human liver microsomal cytochromes P450 by component alkaloids of goldenseal: time-dependent inhibition and allosteric effects. Drug Metab Dispos (2020) 48(10):1018–27. doi:10.1124/dmd.120.091041
11. Li, Y, Li, J, Yan, D, Wang, Q, Jin, J, Tan, B, et al. Influence of zuojin pill on the metabolism of venlafaxine in vitro and in rats and associated herb-drug interaction. Drug Metab Dispos (2020) 48(10):1044–52. doi:10.1124/dmd.120.000048
12. Li Lhl, SY, Teh, BS, Teh, B, Seow, W, and Thong, Y. Anti-inflammatory and immunosuppressive properties of the bis-benzylisoquinolines: in vitro comparisons of tetrandrine and berbamine. Int J Immunopharmacology (1989) 11:395–401. doi:10.1016/0192-0561(89)90086-6
13. Sun, Y, Yao, T, Li, H, Peng, Y, and Zheng, J. In vitro and in vivo metabolic activation of berbamine to quinone methide intermediate. J Biochem Mol Toxicol (2017) 31(4):e21876. doi:10.1002/jbt.21876
14. Zhang, YT, Yu, YQ, Yan, XX, Wang, WJ, Tian, XT, Wang, L, et al. Different structures of berberine and five other protoberberine alkaloids that affect P-glycoprotein-mediated efflux capacity. Acta Pharmacol Sin (2019) 40(1):133–42. doi:10.1038/s41401-018-0183-7
15. Li, J, Wang, Y, and Hidalgo, IJ. Kinetic analysis of human and canine P-glycoprotein-mediated drug transport in MDR1-MDCK cell model: approaches to reduce false-negative substrate classification. J Pharm Sci (2013) 102(9):3436–46. doi:10.1002/jps.23523
16. Zhang, H, Wang, X, Wang, T, Chen, K, Wang, H, Jia, Q, et al. Enhancement of berberine hypoglycemic activity by oligomeric proanthocyanidins. Molecules (2018) 23(12):3318. doi:10.3390/molecules23123318
17. Zhang, X, Qiu, F, Jiang, J, Gao, C, and Tan, Y. Intestinal absorption mechanisms of berberine, palmatine, jateorhizine, and coptisine: involvement of P-glycoprotein. Xenobiotica (2011) 41(4):290–6. doi:10.3109/00498254.2010.529180
18. Maeng, HJ, Yoo, HJ, Kim, IW, Song, IS, Chung, SJ, and Shim, CK. P-glycoprotein-mediated transport of berberine across Caco-2 cell monolayers. J Pharm Sci (2002) 91(12):2614–21. doi:10.1002/jps.10268
19. Nies, AT, Herrmann, E, Brom, M, and Keppler, D. Vectorial transport of the plant alkaloid berberine by double-transfected cells expressing the human organic cation transporter 1 (OCT1, SLC22A1) and the efflux pump MDR1 P-glycoprotein (ABCB1). Naunyn-Schmiedeberg's Arch Pharmacol (2008) 376(6):449–61. doi:10.1007/s00210-007-0219-x
20. Shi, R, Yang, Y, Xu, Z, Dai, Y, Zheng, M, Wang, T, et al. Renal vectorial transport of berberine mediated by organic cation transporter 2 (OCT2) and multidrug and toxin extrusion proteins 1 (MATE1) in rats. Biopharmaceutics & Drug Disposition (2018) 39(1):47–58. doi:10.1002/bdd.2112
21. Shi, R, Xu, Z, Xu, X, Jin, J, Zhao, Y, Wang, T, et al. Organic cation transporter and multidrug and toxin extrusion 1 co-mediated interaction between metformin and berberine. Eur J Pharm Sci (2019) 127:282–90. doi:10.1016/j.ejps.2018.11.010
22. Sun, S, Wang, K, Lei, H, Li, L, Tu, M, Zeng, S, et al. Inhibition of organic cation transporter 2 and 3 may be involved in the mechanism of the antidepressant-like action of berberine. Prog Neuro-Psychopharmacology Biol Psychiatry (2014) 49:1–6. doi:10.1016/j.pnpbp.2013.11.005
23. Chen, C, Wu, ZT, Ma, LL, Ni, X, Lin, YF, Wang, L, et al. Organic anion-transporting polypeptides contribute to the hepatic uptake of berberine. Xenobiotica (2015) 45(12):1138–46. doi:10.3109/00498254.2015.1042537
24. Wang, GFJJ, Jin, J, Zeng, J, Shi, R, Dai, Y, Wu, J, et al. Involvement of P-glycoprotein and multidrug and toxin extrusion protein 1 in hepatic and renal berberine efflux in mice. RSC Adv (2017) 7(55):34801–9. doi:10.1039/c7ra01643c
25. Pedersen, JM, Matsson, P, Bergstrom, CA, Hoogstraate, J, Noren, A, LeCluyse, EL, et al. Early identification of clinically relevant drug interactions with the human bile salt export pump (BSEP/ABCB11). Toxicol Sci (2013) 136(2):328–43. doi:10.1093/toxsci/kft197
26. Karlgren, M, Vildhede, A, Norinder, U, Wisniewski, JR, Kimoto, E, Lai, Y, et al. Classification of inhibitors of hepatic organic anion transporting polypeptides (OATPs): influence of protein expression on drug-drug interactions. J Med Chem (2012) 55(10):4740–63. doi:10.1021/jm300212s
27. Nguyen, JT, Tian, DD, Tanna, RS, Hadi, DL, Bansal, S, Calamia, JC, et al. Assessing transporter-mediated natural product-drug interactions via in vitro-in vivo extrapolation: clinical evaluation with a probe cocktail. Clin Pharmacol Ther (2021) 109(5):1342–52. doi:10.1002/cpt.2107
28. Tan, KW, Li, Y, Paxton, JW, Birch, NP, and Scheepens, A. Identification of novel dietary phytochemicals inhibiting the efflux transporter breast cancer resistance protein (BCRP/ABCG2). Food Chem (2013) 138(4):2267–74. doi:10.1016/j.foodchem.2012.12.021
29. Xiao, L, Xue, Y, Zhang, C, Wang, L, Lin, Y, and Pan, G. The involvement of multidrug and toxin extrusion protein 1 in the distribution and excretion of berberine. Xenobiotica (2018) 48(3):314–23. doi:10.1080/00498254.2017.1300707
30. Kwon, M, Choi, YA, Choi, MK, and Song, IS. Organic cation transporter-mediated drug-drug interaction potential between berberine and metformin. Arch Pharm Res (2015) 38(5):849–56. doi:10.1007/s12272-014-0510-6
31. Xin, HW, Wu, XC, Li, Q, Yu, AR, and Klotz, U. Effects of berberine on the transport of P-gp substrates across Caco-2 and L-MDR1 cell monolayers. Chin Pharmacol Bull (2007)(23) 799–803.
32. Lin, HL, Liu, TY, Lui, WY, and Chi, CW. Up-regulation of multidrug resistance transporter expression by berberine in human and murine hepatoma cells. Cancer (1999) 85(9):1937–42. doi:10.1002/(sici)1097-0142(19990501)85:9<1937::aid-cncr9>3.0.co;2-f
33. He, L, and Liu, GQ. Effects of various principles from Chinese herbal medicine on rhodamine123 accumulation in brain capillary endothelial cells. Acta Pharmacol Sin (2002) 23(7):591–6.
34. Jing, W, Safarpour, Y, Zhang, T, Guo, P, Chen, G, Wu, X, et al. Berberine upregulates P-glycoprotein in human caco-2 cells and in an experimental model of colitis in the rat via activation of nrf2-dependent mechanisms. J Pharmacol Exp Ther (2018) 366(2):332–40. doi:10.1124/jpet.118.249615
35. Han, YQYJ, Yuan, JY, Shi, YJ, Zhu, Y, and Wu, SL. Reversal effect of berbamine on multidrug resistance of K562/A02 cells and its mechanism. Zhongguo Shi Yan Xue Ye Xue Za Zhi (2003) 11(11):604–8.
36. Tian, H, and Pan, QC. A comparative study on effect of two bisbenzylisoquinolines, tetrandrine and berbamine, on reversal of multidrug resistance. Yao Xue Xue Bao (1997) 32(4):245–50.
37. Han, YQSY, Yuan, JY, Zhu, Y, and Wu, SL. The study of reversal resistance effect and its mechanism of berbamine in MCF7/ADR cells. Acta Anatomica Sinica (2004)(35) 161–4.
38. Evers, R, Zaman, GJ, van Deemter, L, Jansen, H, Calafat, J, Oomen, LC, et al. Basolateral localization and export activity of the human multidrug resistance-associated protein in polarized pig kidney cells. J Clin Invest (1996) 97(5):1211–8. doi:10.1172/jci118535
39. Evers, R, Kool, M, van Deemter, L, Janssen, H, Calafat, J, Oomen, LC, et al. Drug export activity of the human canalicular multispecific organic anion transporter in polarized kidney MDCK cells expressing cMOAT (MRP2) cDNA. J Clin Invest (1998) 101(7):1310–9. doi:10.1172/jci119886
40. Sun, J, He, ZG, Cheng, G, Wang, SJ, Hao, XH, and Zou, MJ. Multidrug resistance P-glycoprotein: crucial significance in drug disposition and interaction. Med Sci Monit (2004) 10(1):RA5–14.
41. Crivori, P, Reinach, B, Pezzetta, D, and Poggesi, I. Computational models for identifying potential P-glycoprotein substrates and inhibitors. Mol Pharmaceutics (2006) 3(1):33–44. doi:10.1021/mp050071a
42. Haslam, IS, Jones, K, Coleman, T, and Simmons, NL. Induction of P-glycoprotein expression and function in human intestinal epithelial cells (T84). Biochem Pharmacol (2008) 76(7):850–61. doi:10.1016/j.bcp.2008.07.020
43. Mitin, T, Von Moltke, LL, Court, MH, and Greenblatt, DJ. Levothyroxine up-regulates P-glycoprotein independent of the pregnane X receptor. Drug Metab Dispos (2004) 32(8):779–82. doi:10.1124/dmd.32.8.779
44. Bodo, A, Bakos, E, Szeri, F, Varadi, A, and Sarkadi, B. The role of multidrug transporters in drug availability, metabolism and toxicity. Toxicol Lett (2003) 140-141:133–43. doi:10.1016/s0378-4274(02)00497-6
45. Guo, A, Marinaro, W, Hu, P, and Sinko, PJ. Delineating the contribution of secretory transporters in the efflux of etoposide using Madin-Darby canine kidney (MDCK) cells overexpressing P-glycoprotein (Pgp), multidrug resistance-associated protein (MRP1), and canalicular multispecific organic anion transporter (cMOAT). Drug Metab Dispos (2002) 30(4):457–63. doi:10.1124/dmd.30.4.457
46. Fugh-Berman, A, and Ernst, E. Herb-drug interactions: review and assessment of report reliability. Br J Clin Pharmacol (2001) 52(5):587–95. doi:10.1046/j.0306-5251.2001.01469.x
47. Wang, Z, Hamman, MA, Huang, SM, Lesko, LJ, and Hall, SD. Effect of St John's wort on the pharmacokinetics of fexofenadine. Clin Pharmacol Ther (2002) 71(6):414–20. doi:10.1067/mcp.2002.124080
48. Dresser, GK, Schwarz, UI, Wilkinson, GR, and Kim, RB. Coordinate induction of both cytochrome P4503A and MDR1 by St John's wort in healthy subjects. Clin Pharmacol Ther (2003) 73(1):41–50. doi:10.1067/mcp.2003.10
49. Kaye, AD, Clarke, RC, Sabar, R, Vig, S, Dhawan, KP, Hofbauer, R, et al. Herbal medicines: current trends in anesthesiology practice--a hospital survey. J Clin Anesth (2000) 12(6):468–71. doi:10.1016/s0952-8180(00)00195-1
50. Piscitelli, SC, Burstein, AH, Welden, N, Gallicano, KD, and Falloon, J. The effect of garlic supplements on the pharmacokinetics of saquinavir. Clin Infect Dis (2002) 34(2):234–8. doi:10.1086/324351
51. Rosenblatt, M, and Mindel, J. Spontaneous hyphema associated with ingestion of Ginkgo biloba extract. N Engl J Med (1997) 336(15):1108. doi:10.1056/nejm199704103361518
52. Gaedeke, J, Fels, LM, Bokemeyer, C, Mengs, U, Stolte, H, and Lentzen, H. Cisplatin nephrotoxicity and protection by silibinin. Nephrol Dial Transplant (1996) 11(1):55–62. doi:10.1093/oxfordjournals.ndt.a027066
53. Schönfeld, J, Weisbrod, B, and Muller, MK. Silibinin, a plant extract with antioxidant and membrane stabilizing properties, protects exocrine pancreas from cyclosporin A toxicity. Cell Mol Life Sci (1997) 53(11-12):917–20. doi:10.1007/s000180050111
54. Pfaffl, MW. A new mathematical model for relative quantification in real-time RT-PCR. Nucleic Acids Res (2001) 29(9):e45–45. doi:10.1093/nar/29.9.e45
55. Fu, LW, Deng, ZA, Pan, QC, and Fan, W. Screening and discovery of novel MDR modifiers from naturally occurring bisbenzylisoquinoline alkaloids. Anticancer Res (2001) 21(4A):2273–80.
56. Jakubikova, J, Duraj, J, Hunakova, L, Chorvath, B, and Sedlak, J. PK11195, an isoquinoline carboxamide ligand of the mitochondrial benzodiazepine receptor, increased drug uptake and facilitated drug-induced apoptosis in human multidrug-resistant leukemia cells in vitro. Neoplasma (2002) 49(4):231–6.
57. Wakusawa, S, Nakamura, S, Tajima, K, Miyamoto, K, Hagiwara, M, and Hidaka, H. Overcoming of vinblastine resistance by isoquinolinesulfonamide compounds in adriamycin-resistant leukemia cells. Mol Pharmacol (1992) 41(6):1034–8.
58. Wu, LT, Tsou, MF, Ho, CC, Chuang, JY, Kuo, HM, and Chung, JG. Berberine inhibits arylamine N-acetyltransferase activity and gene expression in Salmonella typhi. Curr Microbiol (2005) 51(4):255–61. doi:10.1007/s00284-005-4569-7
59. Pan, GY, Wang, GJ, Liu, XD, Fawcett, JP, and Xie, YY. The involvement of P-glycoprotein in berberine absorption. Pharmacol Toxicol (2002) 91(4):193–7. doi:10.1034/j.1600-0773.2002.t01-1-910403.x
60. Moga, MM. Alternative treatment of gallbladder disease. Med Hypotheses (2003) 60(1):143–7. doi:10.1016/s0306-9877(02)00351-1
61. Weber, HA, Zart, MK, Hodges, AE, White, KD, Barnes, SM, Moody, LA, et al. Method validation for determination of alkaloid content in goldenseal root powder. J AOAC INTERNATIONAL (2003) 86(3):476–83. doi:10.1093/jaoac/86.3.476
62. Dabrowski, D, Lech, K, and Jarosz, M. Capillary-HPLC with tandem mass spectrometry in analysis of alkaloid dyestuffs - a new approach. Electrophoresis (2018) 39(9-10):1276–83. doi:10.1002/elps.201700349
63. Westphal, K, Weinbrenner, A, Zschiesche, M, Franke, G, Knoke, M, Oertel, R, et al. Induction of P-glycoprotein by rifampin increases intestinal secretion of talinolol in human beings: a new type of drug/drug interaction. Clin Pharmacol Ther (2000) 68(4):345–55. doi:10.1067/mcp.2000.109797
64. Fardel, O, Lecureur, V, and Guillouzo, A. Regulation by dexamethasone of P-glycoprotein expression in cultured rat hepatocytes. FEBS Lett (1993) 327(2):189–93. doi:10.1016/0014-5793(93)80167-s
65. Tian, R, Koyabu, N, Morimoto, S, Shoyama, Y, Ohtani, H, and Sawada, Y. Functional induction and de-induction of P-glycoprotein by St. John's wort and its ingredients in a human colon adenocarcinoma cell line. Drug Metab Dispos (2005) 33(4):547–54. doi:10.1124/dmd.104.002485
66. Schuetz, EG, Beck, WT, and Schuetz, JD. Modulators and substrates of P-glycoprotein and cytochrome P4503A coordinately up-regulate these proteins in human colon carcinoma cells. Mol Pharmacol (1996) 49(2):311–8.
Glossary
Keywords: Oregon grape root, berberine, berbamine, P-glycoprotein (P-gp), cyclosporin A (CsA), digoxin, inhibition, induction
Citation: Fan Y, Zhou Z and Zhang L (2024) Effect of Oregon grape root extracts on P-glycoprotein mediated transport in in vitro cell lines. J. Pharm. Pharm. Sci 26:11927. doi: 10.3389/jpps.2023.11927
Received: 16 August 2023; Accepted: 22 December 2023;
Published: 18 January 2024.
Edited by:
Reza Mehvar, Chapman University, United StatesCopyright © 2024 Fan, Zhou and Zhang. This is an open-access article distributed under the terms of the Creative Commons Attribution License (CC BY). The use, distribution or reproduction in other forums is permitted, provided the original author(s) and the copyright owner(s) are credited and that the original publication in this journal is cited, in accordance with accepted academic practice. No use, distribution or reproduction is permitted which does not comply with these terms.
*Correspondence: Ying Fan, ZmFueWluZzEyMzQ1QGdtYWlsLmNvbQ==
†These authors have contributed equally to this work