- Department of Environment and Soil Sciences, Universitat de Lleida, Lleida, Spain
Gypsum is widely found in soils under arid and semi-arid climates due to its semi-soluble nature. In spite of that, they are less known than other soils, and this has generated some misunderstandings in some initial pedological concepts and in soil classification systems. In addition, the quantification of gypsum, and in particular of its secondary accumulations is affected by the sampling procedures and sample handling in the lab; besides by the methods used for the determination of gypsum themselves, since they differ on the accuracy, cost, and expertise needed. The objective of our research is to improve some laboratory procedures in order to determine and quantify gypsum in the soil, especially secondary accumulations. We applied several methods of sample handling and gypsum analysis to a loess profile in the Ebro Valley (NE Iberia), consisting of 10 horizons containing gypsum in varying amounts (0 to about 50%); of different sizes and morphologies. We propose a protocol considering procedures (sieving or not), qualitative determinations and two methods (turbidimetry and dehydration of crystallization water) for an optimal determination of gypsum depending on the characteristics of the sample and compared them with the acetone method (US Salinity Laboratory Staff, Agric. Handb., 1954, 60, 175; Nelson, 1978, 181), as it is the reference method in the main Classification Systems. The results obtained after applying the different methods for the analysis of gypsum in bulk samples have allowed us to propose a decision tree procedure for the determination of gypsum in soil materials. This procedure includes, determination of gypsum in all fractions, coarse and fine, the estimated amount of gypsum in the field (as a major or minor component) and the presence of other components that may interfere with the results. The most accurate results are obtained with those methods based on the loss of gypsum water upon heating when gypsum content is >4%, and with the turbidimetric method in case of lower amounts of gypsum. Finally, we discuss the implications of these analyses when a soil is classified according to the main soil classification systems (WRB 2014; Soil Survey Staff, SSS- NRCS, 2014).
Introduction
In comparison with other types of soils, those containing gypsum have not been widely studied. Due to its semi-soluble nature they are mainly found in arid and semi-arid areas of the world (FAO 1990), which have been traditionally less surveyed than, e.g., other soils in temperate areas. This lack of research created misunderstandings in some initial pedological concepts. For instance, in the past, gypsum soils were classified either as saline or as calcareous soils (as in the 7th approximation of Soil Taxonomy, Soil Survey Staff, 1990).
The Soil Map of the World (FAO 1988) defines “gypsiferous soil material” as that containing at least 5% gypsum. Herrero et al. (2009) distinguish gypsiferous from gypseous soils on the basis of gypsum content: the first term would be applied when gypsum content is small and the main soil properties are not controlled by it, and the second to soils in which gypsum is the major component (reaching 90% or more). In other cases, gypsiferous soils have been defined according to land use, as those containing a sufficient amount of gypsum to interfere with plant growth (FAO 1990). Casby-Horton et al. (2015) indicate that gypsum contents higher than 30% have strong implications for physical and chemical properties relevant for agronomic purposes.
Numerous authors (Eswaran et al., 1981; Ilaiwi, 1983; Herrero et al., 1987; Herrero and Port, 1991; Herrero et al., 1992) have contributed over the years to the improvement of the knowledge of soils with gypsum. Due to its relatively high solubility (2.4 g L-1 at 25°C) gypsum is seldom found as inherited from the parent material in arid and semiarid soils but as secondary gypsum accumulations after several dissolution/reprecipitation cycles. The main morphological types of gypsum accumulations as described in the literature are presented in Supplementary Table S1.
Some of these accumulations are cemented, either completely or partly. This is due to the growth of loose gypsum crystals within a confined pore space until they join together forming a xenotopic fabric as seen in thin section (Poch et al., 2018). The concept of cementation has been misused in literature, due to the fact that many gypseous materials become very hard when dry, but disintegrate when submerged in water or become friable when wet (Watson 1985; Porta and Herrero 1988), as it happens with some gypsum “crusts” or “encroûttements” (Supplementary Table S1). Another possible explanation for this confusion may be the presence of cements other than gypsum (Dekkiche, 1974; Kulkc, 1974). However, this origin seems unlikely since it has been experimentally demonstrated that when these cements are eliminated, the material does not lose cohesion, and also, as Halitim (1985) indicates, a high gypsum content makes the behavior of a minority compound impossible as cement.
Current methodologies for describing and defining soils in the two main classification systems “Soil Taxonomy” (S.S.S. 2014) and “World Reference Base for Soil Resource” (WRB 2014–2015) are not very precise when applied to gypsum soils, since they do not they take into account all the characteristics related to their composition, genesis and behavior. In addition, the basic requirements are ambiguous when describing them in the field (definitions of Gypsic and Petrogypsic horizons are intertwined when they should be defined individually), and other criteria are difficult to observe, require additional chemical analysis, and are very difficult to determine (Herrero ey al, 2004).
Another limitation when applying the classification systems is related to the definitions of diagnostic horizons and materials. For instance, both classifications include the Gypsic horizon as a diagnostic horizon, but the definitions are not identical. In both systems a minimum of 5% gypsum (by weight) is required in the fine Earth, but depending on the diagnostic feature or horizon, other gypsum contents or estimates of volume percentages are required, whose precision is not very high. Several qualifiers are used in these systems for gypsum soils. Soil Taxonomy (SSS-NRCS 2014) defines the terms Gypsic, Gypsifactic, and Gypseous while the WRB (2014–15) includes the qualifiers Gypsiric, Artzic, Gypsifractic, and Hypogypsic. The qualifier Hypergypsic is also defined differently in both systems: the fine Earth must contain a minimum of 40% gypsum in Soil Survey Staff, 2014), and 50% gypsum in the World Reference Base (2014–15). These differences can lead to misunderstandings and make the use of the classifications difficult.
The determination of gypsum content in soils is affected on the one hand, by the management of the sample prior to the analysis, and on the other hand, by the analytical methods themselves.
A qualitative test to detect the presence of sulphates using a BaCl2 solution can be used in the field (Porta and López-Acevedo 2005). This test is especially useful when trying to distinguish calcium carbonate from gypsum when it is found as small crystals, as both of them are white.
The standard procedures in soil science laboratories comprise a preliminary sieving to obtain the fine Earth (mineral particles less than 2 mm), before carrying out many of the analyses. Soils with gypsum often contain accumulations of gypsum (either hard or discontinuously cemented) larger than 2 mm, that will not pass through the sieve and therefore will not be included in the sample, which can lead to an erroneous estimate of the actual amount of gypsum. Moreover, the gypsum-containing coarse elements are often fragile and therefore will vary depending on the intensity of the grinding and sieving treatment.
There are several methods to determine the gypsum content in the soil, although not all are equally accurate. In addition, the costs and the time required vary remarkably among them.
The acetone method is the reference wet chemical method for gypsum analysis (US Salinity Laboratory Staff, 1954). It is based on the complete dissolution of the gypsum in the sample, followed by its reprecipitation by adding acetone. The precipitate must be further completely dissolved again and sulphates measured in the solution. There are several ways to quantify gypsum when applying this method: either measuring the electrical conductivity (EC), using a correlation between EC and dissolved salts (US Salinity Laboratory Staff, 1954); or analyzing either SO42− (Loeppert and Suarez 1996) or Ca2+ (Van Reeuwijk 1987) ions. Some of the methods based on the determination of SO42− ions are very precise and can even determine very small amounts of sulphates, on the order of micrograms (Horton and Thomason 1951), but they are time consuming and are difficult to apply. Furthermore, the results may be erroneous when other sulphate minerals are present, since acetone can react with them, as well as with anhydrite (Soil Survey Staff 2014). In these cases, corrections are necessary due to exchange error (reactivity with other sulphates that do not come from gypsum) and occlusion effects of acetone (Lagerwerff et al., 1965). On the other hand, the methods based on the measurement of the EC present the same limitations as the previous method and, in addition, they consume a lot of time, since they require the complete dissolution of the gypsum, a very slow step due to the slow kinetic dissolution of the gypsum (Hirst and Greaves 1922; Lagerwerff et al., 1965). Therefore, it is not a very suitable method for gypsum-rich samples, which require very large amounts of water.
Thermogravimetric methods, based on the loss of crystallization water at low temperatures, allow a faster determination, although they require a minimum amount of gypsum (about 4%) in the sample, to be able to measure it with enough accuracy (Nelson et al., 1978; Lebron et al., 2009). The differential water loss method (Artieda et al., 2006) is based in the same principle. It estimates the amount of gypsum water that is lost when the sample is heated at different fixed temperatures (normally 70–90°C), with an accuracy of approximately 2% gypsum (Artieda et al., 2006). Another method, also based on the water loss when the sample is heated in a temperature range of 70°C to 135–150°C, is OMRAN GypSim method (Omram, 2016) which allows to determine the gypsum content within 1% accuracy by heating the sample to 150°C (temperature at which gypsum becomes anhydrite). Other non-destructive methods based on loss of mass due to the release of water from gypsum use X-ray fluorescence (XRF) (Weindorf et al., 2013) and reflectance; and when combined, the precision increases considerably (Herrero et al., 2020). However, these methods are more expensive and are not easily accessible.
Gypsum rocks are frequent in the Ebro valley (NE Iberia), which have their origin in a Miocene evaporitic basin. This parent material is the origin of the generalized occurrence of soils with varying contents, sizes and degrees of cementation of gypsum (Poch et al., 2021), which poses problems of analysis and classification.
The objectives of this research are to establish the best approaches to characterize gypsum concentrations in soils, taking into account the forms, sizes and amounts of gypsum, and using different methods in order to make recommendations of sample handling and gypsum analysis for genesis and classification purposes.
Materials and Methods
Field Description, Sample Handling and Physical-Chemical Analysis
The profile chosen to develop this methodology is Mas de Caspolí (Mequinenza, Zaragoza, coordinates UTM: X: 265134; Y:4,580,885) located in the upper section of a slope (>3%), at an altitude of 375 m asl and with a depth of 5.5 m. The profile was described in the field following CBDSA, 1983) and sampled for mineralogical, chemical and physical analyses. The samples were taken in bags and air-dried in the lab at room temperature.
Between 300 and 500 g of the air-dried samples were manually sieved to separate the fine Earth (less than 2 mm) from the coarse particles (larger than 2 mm), in order to preserve the gypsum accumulations (nodules, crystals and gypsum intergrowths) observed in the field (Figure 1). As a result, three subsamples were obtained from each horizon for subsequent gypsum content determination: fine Earth (<2 mm), coarse elements (>2 mm) and the unsieved sample (total fraction). All samples and subsamples were weighed before and after sieving (Figure 1). All fractions were crushed in an agate mortar before analysis.
Routine physico-chemical analyzes as described by Porta, 1986) were carried out on the fine Earth fraction. Calcium carbonate equivalent (CaCO3 eq.) was determined by gasometry with the Bernard´s Calcimeter. The organic carbon (OC) was obtained by wet oxidation following Walkley-Black method. pH was measured with a pH-meter in a 1:2.5 soil:water suspension and the electrical conductivity (EC1:5) with a conductimeter in a 1:5 soil:water extract.
The mineralogy of the clay fraction (both coarse and fine clay) was determined through XR diffraction using a Bruker model D8 Advance powder diffractometer at the Facultad de Geología of the Universidad Complutense de Madrid. This equipment has a Cu-anode and a SOL-X energy dispersion detector. The diffractometer works with Bragg-Brentano measurements. The software used for the acquisition, treatment and evaluation of diffractometric data was DIFFRACplus. The preparation of the clay mineral samples included treatments to remove carbonates, organic matter and sulphates using the acetic acid attack method (Porta, 1998). After separating the clays and the preparation of oriented aggregates, a dry air (NT), a solvation with ethylene glycol and a heat treatment at 550°C were performed before reflection analysis in the 060 plane.
Analyses and Morphoscopy of the Coarse Fraction
Some representative gypsum crystals and intergrowths from the coarse fraction were carefully cleaned with a brush and a small chisel prior to their observation and description with the help of a binocular microscope (OLYMPUS-SZX16, objective: SD PLAPO 1XPF). Their colors, cleavage, crystalline habit and type of crystal aggregation were described, as well as other properties that could help to understand their genesis.
Gypsum Analysis Methods
Qualitative and Prospective Tests
The presence of sulphates can be detected in the field by the formation of a precipitate after adding a drop of BaCl2 10% to a soil:water suspension and subsequent filtration (Porta & López-Acevedo 2005). It indicates the presence of gypsum, as well as of other sulphates in salt-affected soils (as mirabilite, burkeite, bloedite or jarosite). In the lab a 1:5 soil:water suspension (volume ratio) was used (Porta & López-Acevedo 2005). This test cannot detect gypsum concentrations below 0.26% (Porta et al., 1986).
The electrical conductivity of a 1:5 suspension (EC1:5) also indicates the presence of gypsum, since the EC of a solution saturated with gypsum is 2.2 dS/m at 25°C. This value remains fairly constant when analyzing gypsum-containing soils regardless of the soil:water ratio, due to the relatively low solubility of gypsum (2.4 g L−1at 25°C) that saturates any solution very fast in absence of other salts.
Artieda Method
This method measures the difference in weight resulting from the loss of crystalline water from the gypsum molecule, when the sample is heated to fixed temperatures, which determines the transformation of gypsum into bassanite and anhydrite. Since not all the water is lost when the sample is heated, it is necessary to apply a recovery factor (Burns et al., 2002).
We used the method proposed by Artieda (1993), the refined method proposed by these authors (Artieda et al., 2006), and the method proposed by Lebron et al. (2009) following the recommendations of Herrero et al. (2020).
The steps followed have been the same as those proposed by Artieda et al. (2006), applying the following intervals (40–105°C; 70–105°C):
(1) Weigh about 15 g of the air dried sample,
(2) Place it on an oven container in a hot air oven at 40°C for 48 h,
(3) Let the sample cool down in a desiccator to prevent it from absorbing moisture,
(4) Weigh the sample again,
(5) Place it again into the oven, at 70°C, for further 48 h,
(6) Leave the sample again in a desiccator and weigh it again once cooled.
(7) Repeat the process (steps 5–6), now at 105°C.
(8) Calculate the amount of gypsum in the sample, applying the following expression:
ws: sample weight at the initial temperature (40°C or 70°C); wf: sample weight at the final temperature (105°C); wc: container weight; rf: recovery factor according to the temperature interval.
The recovery factor is 19.1% when the interval between 70 and 105°C is used (Artieda et al., 2006), and 19.39% for the interval 40–105°C (Artieda 1993).
This method loses accuracy when gypsum contents are lower than 8% for the temperature ranges 40–105°C (Artieda et al., 2006). Furthermore, it is not recommended for gypsum contents lower than 2% when calculated for temperatures higher than 70°C (Artieda et al., 2006). Moreover, previous experiences in our lab (unpublished) seem to indicate that the precision is already lower when there is less than 5% gypsum.
Three replications were carried out for each sample and the standard deviation was calculated, in order to quantify the variation between the gypsum results obtained, in the different fractions (total, coarse and fine).
Finally, to determine the precision of the method, three replications have been carried out for each fraction of the Artieda method, in the two temperature ranges indicated (40–105°C; 70–105°C).
The results obtained from the replications have been assessed by taking into account the weight of each sample fraction (fine and coarse), to obtain the total gypsum content in each horizon, and compare them with the one obtained in the total sample. The expression used to calculate the total gypsum content is as follows:
%A: % gypsum in the fine fraction; weight FF: grams of fine fraction; %B: % gypsum in the coarse fraction (contribution of the coarse fraction to total gypsum (B)); weight CF: grams of coarse fraction; weight TF: grams of total fraction.
Turbidimetry Method
Gypsum content can be determined by turbidimetry in absence of other sulphates (Porta et al., 1986). This method is based on the precipitation of BaSO4 and other sulphate salts after the addition of Ba2+ (as BaCl2) in a sulphate-containing solution, and the estimation of the turbidity of the resulting solution by spectrometry. All gypsum in the sample must be dissolved before the addition of BaCl2, and therefore it is necessary to have a prior rough estimate of the gypsum content, since it will affect the dilution factor, which varies between 1:50 to 1:1000 (soil: water) for gypsum contents between 5 and 90% respectively (Porta et al., 1986). It is also necessary that the precipitate remains in suspension during the measurement with the spectrometer, and that gypsum standards are prepared and measured to obtain a calibration curve. This technique seems to give better results when analyzing non-saline samples with low gypsum contents (<5%).
The detailed turbidimetry method procedure is as follows:
(1) Weigh the required pulverized sample quantity to prepare a 250 ml of solution by adding deionized water, and stir for 8 h on a shaker.
(2) Put 200 ml of the extract in 250 ml-centrifuge bottles, and centrifuge at 8,000 rpm for 10 min (In this study, a Beckman Coulter centrifuge, model Allegra 25 R was used).
(3) In 50 ml flasks, add in the following order: the amount of extract required (ml) (It is advisable to previously shake the prepared extract to homogenize it), deionized water, 10 ml of stabilizer solution (135 g of CaCl2·2H2O+ 50 ml of concentrated HCl +500 ml ethylene glycol in 1 L of solution), deionized water, 0.2 g of BaCl2·2H2O powder and deionized water up to 50 ml.
(4) In addition, five standards of 0, 10, 20, 30, 40 ppm of SO42- are prepared with 0, 0.5, 1, 2, 3 ml, respectively, of a solution of SO42--1000 ppm.
(5) Allow the reagents to act for 8 min. After this time, the readings must be carried out in the next 15 min. Due to this time constraint, it is important to choose an adequate number of samples in each run to allow time to read.
(6) Read the turbidity with a spectrophotometer at 420 nm (we used a Unicamp UV-VIS ultraviolet spectrometer, model 9423).
Thermogravimetry Method
The Thermogravimetric Analysis (TGA) consists in the continuous measurement of the mass of a substance, which is monitored as a function of temperature (T) or time (t), since the sample specimen is subjected to a constant temperature increase (T/t) in a controlled atmosphere. Its application to gypsum determination is based on the loss of crystallization water of gypsum molecules at certain temperature ranges. This technique has been successfully used in gypsum analysis (Al-Muktar, 1987; Poch 1992). It was applied to the samples of the 2Cy1 and 4Bym horizons of profile Mas de Caspolí, in order to compare the results obtained with those from the other analyses.
The equipment used was an SDT Q600 V8.3 Build 10.1 Thermogravimetry with ±2% precision, which allows simultaneous measurements of weight change due to heating (TGA) and differential heat flow (DSC). The measurements were carried out on approximately 10 mg of sample, therefore a thorough homogenization of the sample -mixing with a spatula- was required prior to weighing. The temperature range applied was from room temperature (aprox. 25°C) to 1000°C with an increase of 10°C/min and with an air flow system of 100 ml/min.
Acetone Method
The Acetone method is a wet chemical method based on the measurement of the electrical conductivity of the solution resulting from the dissolution of the gypsum in the sample and its subsequent reprecipitation by adding pure acetone (99%). This method has been applied to all the sample fractions (coarse and fine fraction) which has allowed estimating the amount of real gypsum in each fraction.
The analysis protocol followed has consisted of the following steps, based on the protocol proposed by the US Salinity Laboratory Staff. (1954).
(1) Weighing of the soil sample between 2–20 g (the amount will depend on the EC of the previously measured sample, taking >850 dS/m as the limit).
(2) Dissolution of the samples with 100 ml of milliQ water, shaking the sample for 30 min and subsequent filtering (Millipore filter, 185 mm, Whatman No.2V).
(3) Addition of 5 ml of pure acetone (99%), mix manually and allow to flocculate
(4) Centrifuge without stopper, at 5,000 rpm for 5 min (Centrifuge: Beckman Coulter/Allegra 25R)
(5) Decant the remainder and add a further 5 ml of acetone. Mix with a laboratory Vortex mixer for approximately 20 s
(6) The centrifugation is repeated at 5,000 rpm for 5 min, to re-precipitate the gypsum and the remaining acetone is decanted.
(7) Finally, add 10 ml of miliQ water and mix the samples in a laboratory Vortex mixer for 20 s
(8) Reading of the electrical conductivity in a conductivity meter (Crison GLP31) and the pertinent calculations are made to obtain the % of real gypsum, following the equations proposed by the US Salinity Laboratory Staff. (1954).
Results
Profile Description and Analyses
The studied profile has been developed on loess, deposited over the underlying material (below 5.5 m) consisting of Miocene shales, limestones, calcareous sandstones and gypsum. It has been classified as a Typic Haploxerept (Soil Survey Staff, 2014). It contains several types of gypsum accumulations which generate crystals of different sizes and morphologies. Supplementary Table S2, shows a summary of its field description and its main chemical properties.
The results of clay mineralogy analysis, through X-ray diffraction (Figure 2), have given peaks that correspond to interstratified illite-montmorillonite (peaks >1–1.4 nm, in air dry, 1.6–1.8 nm with glycol and decay to 1 nm at 550°C), illite (very sharp peak at 1 nm), kaolinite (peaks at 1.25, 0.43 and 0.33 nm), quartz (very sharp peaks at 0.43 and 0.33 nm, especially in the coarse fraction) and chlorite (peaks at 1.4, 0.76, 0.47, 0.35 nm).
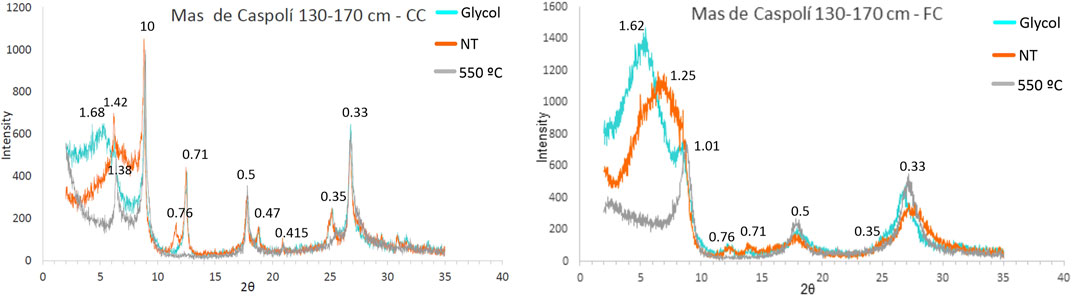
FIGURE 2. Diffractograms of Coarse Clays (CC) and Fine Clays (FC) of the 3Bwy1 horizon (130–170 cm). The three lines correspond to the three treatments used (Glycol, dry air (NT) and 550°C). Peak values (nm) observed to identify clay mineralogy are indicated.
Partition of Fine Earth/Coarse Fractions
The different fractions obtained from the sieving have been subdivided into three representative parts, trying to make an equal division that would represent a similar percentage of each fraction.
The fine Earth includes 61%–83% of the mass of the sample, with a relatively small deviation among the replicates measured. The Supplementary Table S3, shows the partition, as a percentage by weight of each fraction, coarse fine, and total sample, of the manually sieved profile horizons. The upper horizons do not appear because they consisted only of fine Earth.
Morphoscopy of the Coarse Gypsum Fragments
The observation and description of the morphologies of the secondary gypsum accumulations has made it possible to know which morphologies are the most abundant and thus help to understand their formation (Table 1). Some of the accumulations observed are presented in Figure 3, with the aim of showing the range of sizes and the variability of morphologies that can be found.
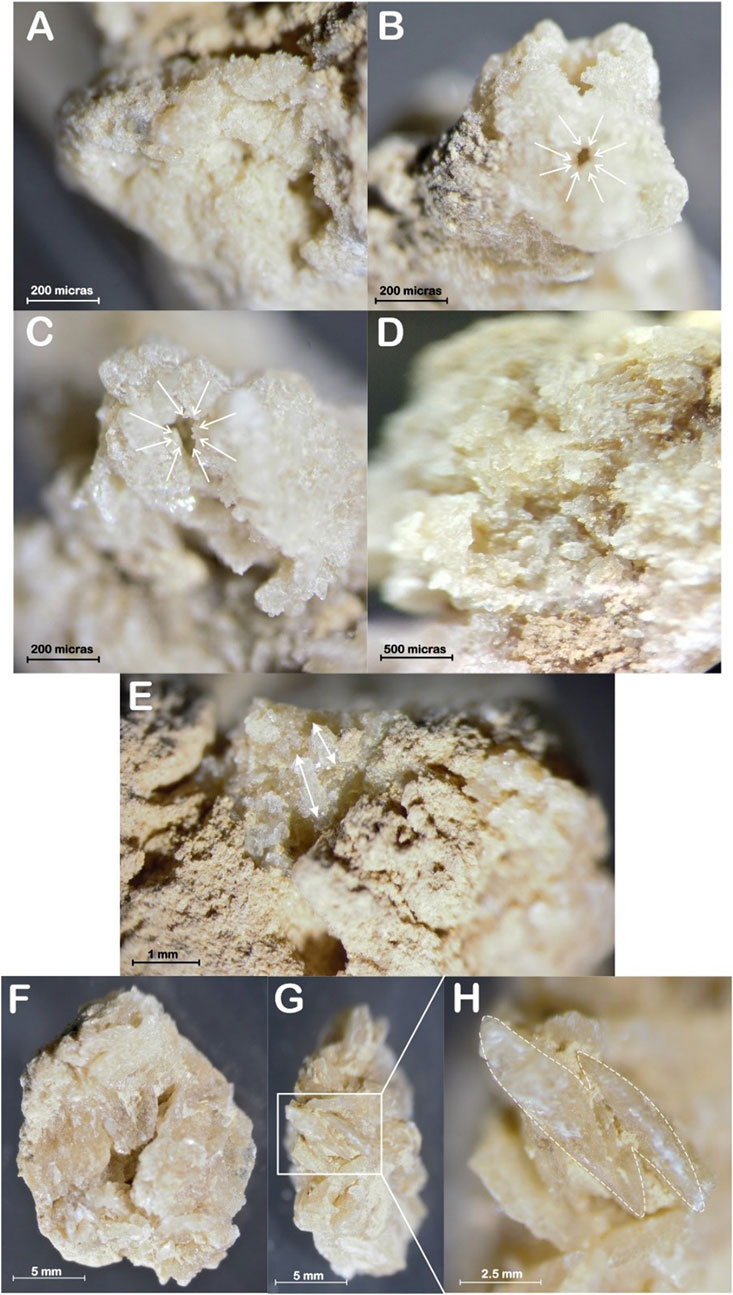
FIGURE 3. Some examples of the size and morphology of secondary gypsum accumulations observed with a binocular, present in the profile studied. Images (A–D) are accumulations found in the 2Cy1 horizon. They show aggregates of small gypsum crystals (30–50 µm) with no preferred orientation. Images (B,C) correspond to gypsum crystal fillings in channels, with radial and central orientation (arrows indicate orientation). Image (E) corresponds to the 3Bwy horizon: crystalline morphologies with good development of the long axis, generating perfectly formed lenticular crystals. Images (F–H) correspond to the 5 Bym horizon. Images (F,G) are desert roses with large, lenticular (up to 1 mm) gypsum crystals. Photograph H is a detail of well-developed twinned lenticular crystals.
Observing the accumulations with binocular reinforces the information described in the field. The horizons with vermiform gypsum and gypsum coatings (2Cy1, 3Bwy, 4Bky2) present crystals with too small sizes (<200 µm) to observe them in detail. The coarse fragments of horizons with larger accumulations, such as gypsum rhizocretions, crusts or hard nodules (3Cy2, 5Bym) can be better observed with binocular because they reach larger sizes, which allow a detailed description of the morphologies, growths and orientations.
Determination of Gypsum Content
Qualitative Tests
The results obtained with the qualitative test of BaCl2 give a first indication of the horizons that may contain gypsum. This test has a lower limit of 0.26% (Porta et al., 1986), detecting very small amounts of gypsum. However, it is not a definitive test, since it gives positive results in soils with a very low amount of gypsum, probably due to the presence of other soluble salts or sulphates.
This case is exemplified in the BC horizon, in which no gypsum has been described in the field and the EC1:5 value is very low. The most likely explanation is that there may be a very small amount of unobservable sulphate in the matrix, that makes the test positive.
Measuring the electrical conductivity (1:5 extract) helps to better specify the possibility of the detection of gypsum. The horizons with visible gypsum in the field are positive in the qualitative test and yield EC1:5 < values over 1 dS/m, and between 2.3 and 2.6 dS/m in the four central horizons. Likewise, horizons with conductivities higher than 1.3 dS/m, despite having a low amount of gypsum, show that this amount is already sufficient to raise their electrical conductivity above 0.35 dS/m, the limit to be considered slightly saline (Porta & López-Acevedo 2005).
Table 2 contains the results of the qualitative test for sulphates using BaCl2, and the value of EC in the 1:5 filtered extract that can serve as indicator of the presence of gypsum.
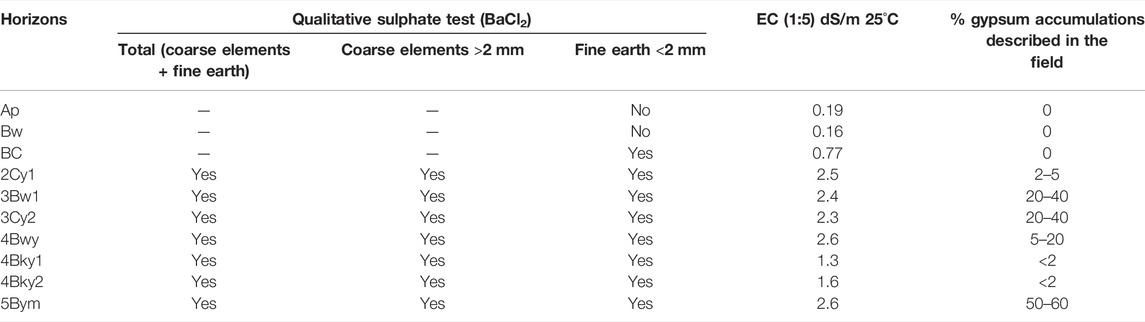
TABLE 2. Results of the qualitative tests for sulphate on the three fractions and of the EC analysis (fine Earth) on a 1:5 soil:water suspension of the studied horizons.
Gypsum Quantification
The results obtained with the different methods (Artieda 40–105°C; Artieda 70–105°C; turbidimetry and thermogravimetry) are shown in Tables 3, 4. Figure 4 is the thermogravimetric diagram obtained from the analyzed 5Bym horizon. The green lines (weight loss (%)) and blue lines (peaks at which weight loss occurs, as a derivative of weight versus temperature (%/°C)) are related to the variation in sample weight at temperature rise. The pink line (heat flow (W/g)) and brown line (sample temperature difference (°C/mg)) are related to the temperature variation during the analysis. Finally, the peaks of gypsum and carbonate loss have been marked with vertical red lines.
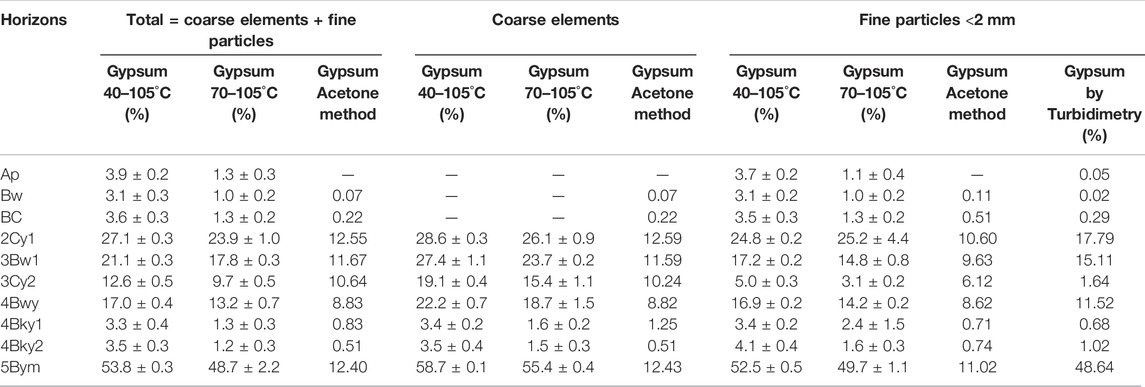
TABLE 3. Gypsum contents of the 3 fractions (total, coarse elements, fine Earth) following Artieda et al. (2006) and acetone method, and of the fine Earth by turbidimetry (Porta et al., 1986). Values are the mean of 3 replications ±standard error.
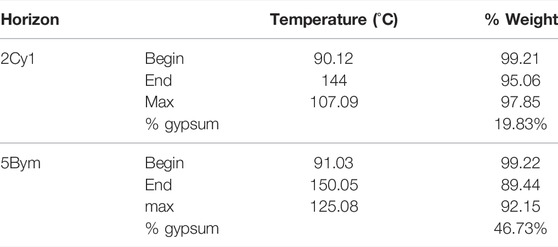
TABLE 4. Temperature ranges (°C) and weight (%) of the 2Cy1 and 5Bym horizons, obtained from their thermogravimetric analyses. The temperature ranges correspond to the peaks from which the weight difference (complete dehydration) has been used for the calculation of the gypsum content.
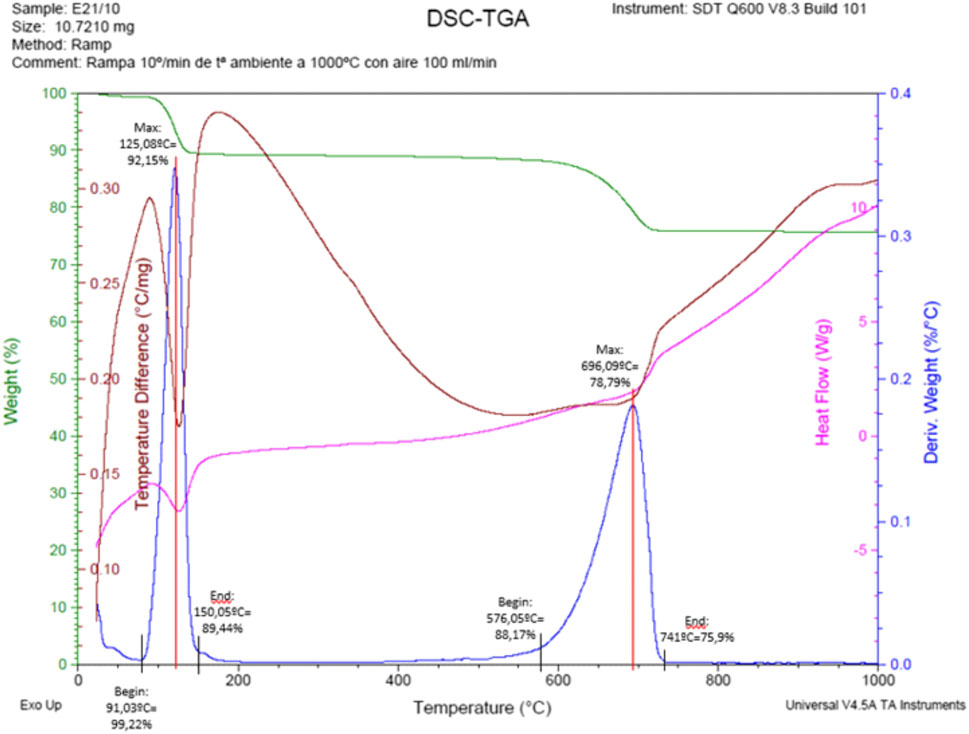
FIGURE 4. Thermogravimetric (TGA) diagram of the 5Bym horizon (depth: 110–130 cm). The temperature peaks of gypsum and carbonate loss are indicated as red vertical lines.
In general, most of the results obtained with Artieda 40–105°C give the highest values, while the results obtained with turbidimetry and thermogravimetry give lower values and reach higher precision for low amounts of gypsum. The acetone method, however, clearly underestimates gypsum, especially in the gypsum-richest samples, without exceeding 15% gypsum with this method, in any of the analysed fractions.
In addition, the standard deviation obtained with the Artieda method in both temperature ranges is relatively low, except for the 2Cy1 horizon, which presents a higher value in the result obtained in the total sample, probably due to a low representativeness of the sample, as will be discussed later.
The total gypsum calculated from the results obtained in each size fraction (fine and coarse fraction) have been compared with the total gypsum obtained from the samples without sieving (total sample), for the two temperature ranges (Table 5). This comparison allows us to see the goodness of the analytical method, since the results obtained are similar.
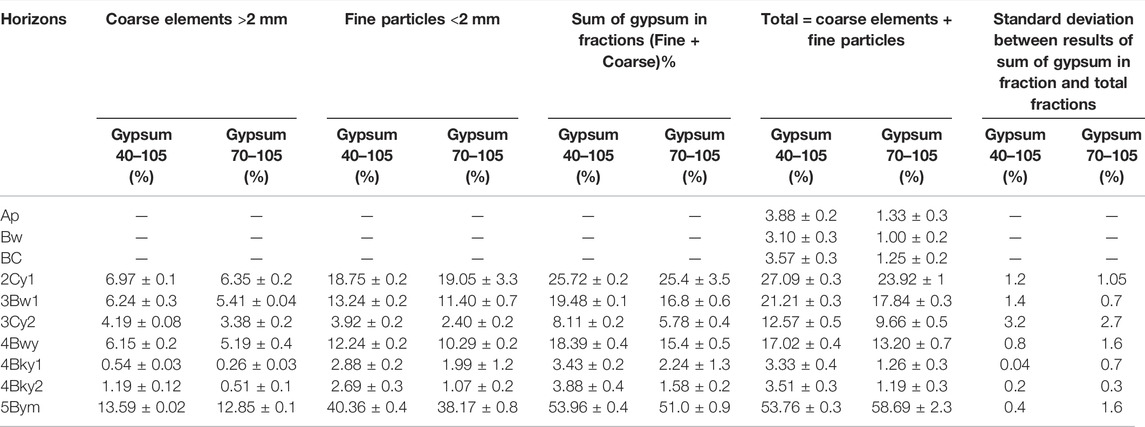
TABLE 5. Average of results obtained with the balance± standard deviation of the three measured fractions with Artieda method (40–105°C; 70–105°C). Standard deviation between results obtained from sum of gypsum in fraction and the results obtained in the total sample.
Discussion
Use of the Qualitative Tests for Gypsum
The results obtained with the qualitative test show that there is no gypsum in the first 2 horizons, that is, the amount of gypsum is less than 0.26% (Porta, 1986). However, the third horizon, BC, gives a positive result in the BaCl2 test, indicating that, despite having quantified a very small amount of gypsum with other applied methods, this amount is greater than 0.26%. In addition, this positive result may also be related to the existence of other sulphates that react with BaCl2.
The EC1:5 values are in agreement with the gypsum contents. In the 3rd horizon the little gypsum present does not saturate the solution although it increases the EC1:5 by over 0.35 dS/m from the overlying gypsum-free horizons. The same occurs with the 4Bky1 and 4Bky2 horizons, with gypsum contents below 5%, but with CE values above 1 dS/m, which indicate the presence of gypsum, although they do not reach the expected values for a gypsum-saturated solution. This may be related to the high carbonate content in the whole profile (reaching 59% CaCO3 in the 4Bky1 horizon), which decreases the solubility of gypsum due to the effect of the common ion (Artieda 1993). The EC1:5 values of the other horizons with gypsum contents higher than 5% are the expected ones, between 2 and 2.5 dS/m in absence of other salts more soluble than gypsum (Lebron et al., 2009).
Comparison of the Results With the Different Methods
The results obtained with the method proposed by Artieda 1993 and Artieda et al. (2006) are generally higher than those obtained with turbidimetry and TGA, as well as the results obtained with the acetone method give significantly lower values, when the sample has a high amount of gypsum (Figure 5). The presence of interstratified clays (montmorillonite-illite and illite) observed with X-ray diffraction, can retain some water in the air-dried sample and this can be released by heating the sample, giving overestimated results. However, as indicated by Herrero et al. (2020), when applying the temperature range between 40 and 105°C, this loss of water from the clays does not interfere with the results.
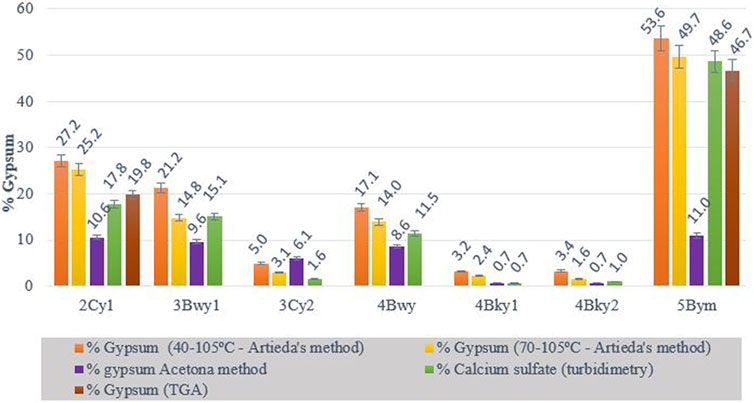
FIGURE 5. Comparative histograms of the results for gypsum quantification according to different methods on the fine Earth fractions.
The values obtained with the method of Artieda et al. are higher for the interval 40°C -105°C than for the interval 70°C - 105°C. Again, this may be due to the presence of residual moisture in the air-dried samples (from 2% to 6%; Porta, 1986) and to the presence of interstratified expandable clays (2%–7.2% error; Artieda, 1996). This author indicates that only half of this total moisture is lost on heating to 40°C and therefore the values may be overestimated. When heating to 70°C, the residual moisture is completely lost (Artieda et al., 2006).
These results confirm the better performance of the method when using the temperature range between 70 and 105°C, in accordance to several references (Nelson et al., 1978; Lebron et al., 2009) that state at this temperature, almost all the hygroscopic water of the gypsum is lost (Herrero et al., 2020).
The acetone method appears to be relatively accurate for low amounts of gypsum (lower than 10%, in agreement with the rest of the methods), however it presents problems in soils with high amounts of gypsum because it clearly underestimates the results. This method has several limitations in these cases, related to the loss of gypsum precipitate in the re-precipitation process, the incomplete final dissolution of the gypsum before the EC measurement, and finally, an error associated with the representativeness of the sampling, when it presents very large gypsum crystals, which is common to some of the other methods. In addition, the presence of high concentrations of other sulphates (Na and K) that also react with acetone, will cause an erroneous quantification of the gypsum.
Finally, the results obtained with TGAs are similar to those obtained by turbidimetry.
Total Gypsum Content
The results of the assessment of the total content of gyspum (Table 5; Figure 5) show a good match between the amount of gypsum quantified in the different fractions (fine Earth and coarse elements) and the amount of gypsum obtained when analyzing the gypsum in the full sample. Indeed, the differences between the gypsum contents of the unsieved sample and the one obtained after the sum of the gypsum in the fine Earth and in the coarse fragments range from 0.4% to 3%, except in the 3Cy2 horizon where the difference is 4%–5% (Table 5). The estimate of gypsum in this horizon is larger when considering the total sample than when analyzing the fractions separately due to the high amount of gypsum present as coarse fragments (almost half of the total volume). These results stress the importance of taking larger samples in the horizons with high proportion of gypsum in the coarse fragments, as well as of the need to carefully homogenize the samples to ensure the accuracy of the analyses. In relation to the recommended amount of sample, Artieda et al. (2006) point out that approximately 20 g of sample are needed to carry out the analyses. However, Lebron et al. (2009) mention that only 1 g of sample is needed when there is more than 30% gypsum, but 5–6 g will be necessary if the amount of gypsum is low (≈1%) (Herrero et al., 2020). In view of our results, we would recommend to follow the proposals of Artieda et al. (2006).
Implications for the Classification of Diagnostic Horizons and Materials
The current international classification systems, “Soil Taxonomy” (Soil Survey Staff, SSS-NRCS, 2014) and “World Reference Base for Soil Resources” (WRB 2014–2015), are not as precise with gypsum as when dealing with other soil components. This lack of precision is shown in Table 6, where a classification exercise has been carried out on the horizons of the studied profile based on field descriptions and gypsum quantification by Artieda method (Artieda 1993) and acetone method, reference method used in classification.
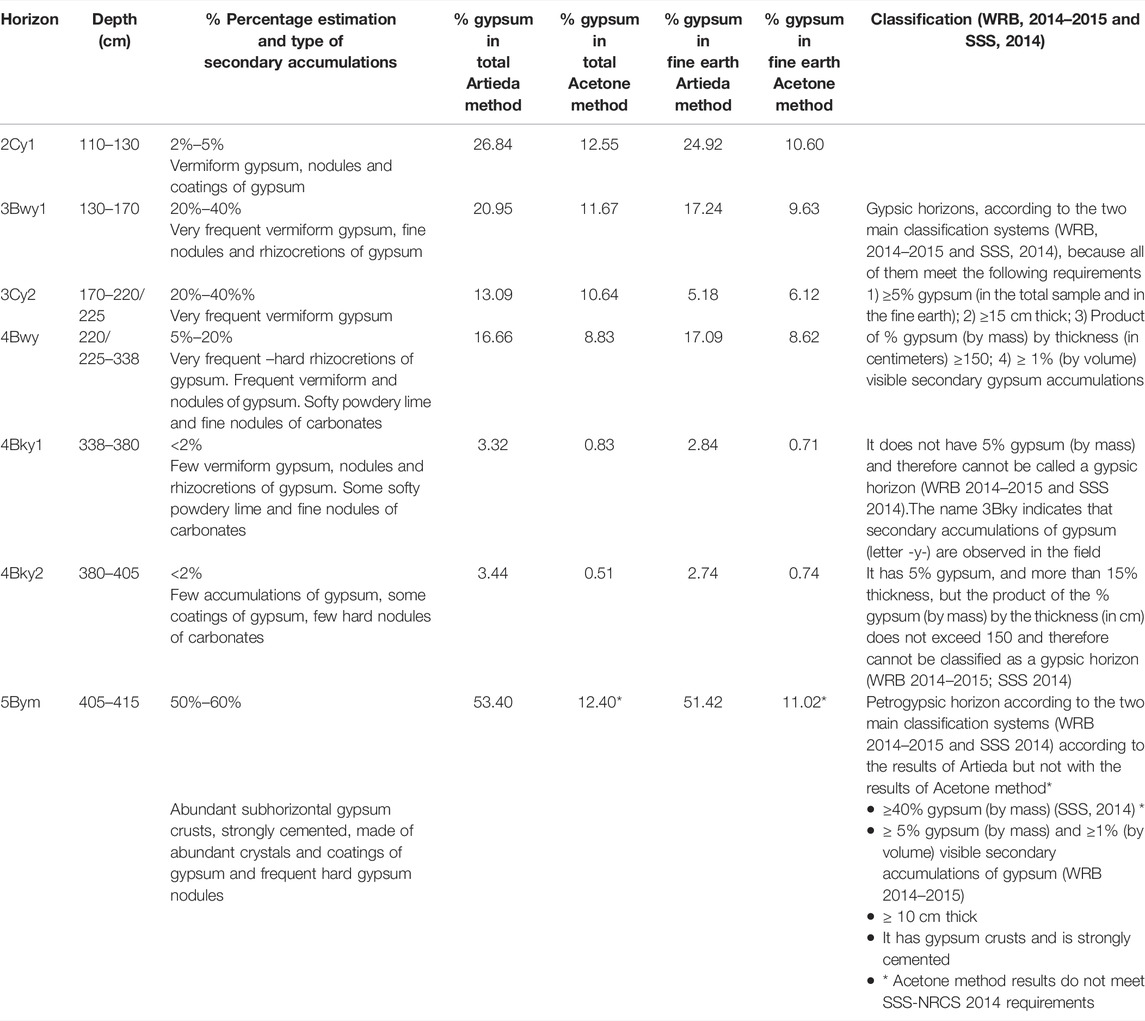
TABLE 6. Classification (WRB 2014–2015 and SSS, 2014) of diagnostic horizons. Profile Mas de Caspolí (Mequinensa).
All the gypsum-containing horizons of this profile meet the requirements to be classified as Gypsic or as Petrogypsic horizons in both systems, except the 4Bky1 and 4Bky2 horizons. In this case, field observations and previous qualitative tests demonstrate the presence of gypsum, but they cannot be classified as gypsic horizons since they do not reach the minimum percentage of gypsum necessary (5% by mass), neither in the total sample nor in the fine Earth. Horizon 3Cy2 is clearly a Gypsic horizon, since it has more than 5% in both fractions, but it is at the limit when only the value with Artieda method is considered, in fine Earth. The results obtained with the acetone method also seem to support the classification, since values >5% have been obtained in all fractions of the gypsic horizons. On the other hand, the 5Bym horizon is classified as petrogypsic in the two main classification systems, according to the results obtained with Artieda. However, based on the results obtained with the acetone method, it cannot be classified as petrogypsic, in the “Soil Taxonomy” Classification system (SSS- NRCS 2014), because it does not reach the minimum gypsum required (40%)
In summary, there are different issues that should be better defined in the classification systems, to avoid misunderstandings and errors:
• Despite observing gypsum accumulations in the field, it may happen that the horizon cannot be classified as a Gypsic horizon, because it does not meet all the requirements proposed by the main classification systems (WRB 2014–2015 and SSS- NRCS 2014).
• Although the minimum limit reported in is 2% (Artieda et al., 2006), it has been observed that the accuracy clearly decreases when the gypsum content is less than 5%. This lack of precision affects the classification objective, with a minimum of 5% (WRB 2014–2015 and SSS- NRCS 2014).
• The values obtained with the acetone method are subject to several limitations, especially in the gypsum-richest materials, where this method clearly underestimates gypsum contents. This can lead to errors when soils with gypsum are classified, since the values may not meet the requirements for, e.g., petrogypsic horizons.
• The analyzed fine Earth samples may not be representative of the total gypsum when there are coarse crystals, which in addition may have a similar colour of the matrix and therefore are difficult to spot and to give visual estimations of their volume in the field.
Proposal for gypsum Determination in Soils
After applying the different methods (qualitative preliminary test, Artieda method (Artieda 1993 and modification of the Artieda method (Artieda et al., 2006) following the recommendations of Herrero et al. (2020), besides the acetone, turbidimetric and thermogravimetric methods, a procedure is proposed to minimize the errors when analyzing gypsum soils (Figure 6).
It is essential to carry out a good description of the field in order to have a first estimate of the gypsum content, which will determine the method to be used. A sufficient amount of sample has to be taken in the field (minimum 500 g), and hand-sieved (care not to destroy large accumulations of gypsum) after air-drying at room temperature. Coarse fragments have to be kept if volume determination in the field has not been precise enough. The fraction to be analyzed must be crushed and homogenized, and when turbidimetry is used the sample must be pulverized to a size less than approx. 50 µm. If the acetone method is applied and there are very large crystals, it is also recommended that the sample be pulverized to reduce the measurement error associated with the behavior of this mineral (Nelson 1982).
It is advisable, in those samples that have more than 0.35 dS/m at 25°C, and especially in samples with conductivities higher than 2.5 dS/m, to carry out a previous wash with ethanol to eliminate the sulphate salts that are more soluble than gypsum. This process must be very fast (a few seconds) to avoid significant loss of gypsum. The subsequent drying of the samples is always carried out at temperatures below 40°C.
The first step is to perform a qualitative test with BaCl2 10% which detects gypsum concentrations higher than 0.26% (Porta, 1986), especially when the horizon is expected to have less than 2% gypsum (Artieda et al., 2006).
If this qualitative test gives a positive result, the electrical conductivity (1:5 soil:water) of the sample should be determined. When the conductivity is higher than 2.5 dS/m, we are dealing with a saline soil that has more soluble salts than gypsum (Lebron et al., 2009). This presence of salts invalidates the turbidimetry method, since sulphate ions can come from salts other than gypsum, causing measurement errors.
In spite of the fact that the reported precision of the Artieda method is 2% (Artieda et al., 2006), our results with different methods (Figure 6) indicate that in samples with low gypsum content (less than 5%) the precision is lower. Therefore, when the estimated quantity is <2%, it is not recommended to use the Artieda method (70–105°C), and if the quantity is <5%, it is advisable to use a more precise method, or to carry out a previous washing of other sulphated salts with ethanol, to increase the precision of the results.
However, when an appreciable amount of gypsum has been reported in the field, it is recommended that this method be used due to its simplicity and low cost.
Conclusion
The analysis of the gypsum content in 9 horizons of a loess profile in the Ebro Valley reveals that in order to achieve a good and complete characterization, the detailed description of the gypsum at different scales (field, macro, micro) is as important for its complete characterization as the method of choice for quantification in the laboratory. The protocol includes a previous good and detailed description of the field (including an estimation of the volume of gypsum), a collection of representative samples (500 g) and an adequate pre-processing of the sample (manual sieving that allows to preserve large accumulations of gypsum).
After that, two qualitative tests (BaCl2 and CE to a suspension 1:5 soil:water) should be performed to determine the presence or absence of gypsum. Depending on the results, the most appropriate method (of the four proposed methods) can be chosen. If gypsum is a major component (>2%), it is recommended to use the Artieda method, based on the loss of water from the gypsum at different temperatures intervals (40–105°C; 70–105°) due to its simplicity and low cost. If the amount of gypsum expected is less than 8%, only the temperature range between 70 and 105°C should be applied. When gypsum is a minor component in the field description (<2%) and the qualitative test is positive, another more precise method is advised. In this case, a thermogravimetric analysis is proposed when the samples are saline (EC1:5 > 2.5 dS/m at 25°C), or a turbidimetric analysis, which of the four methods applied is the most precise, but also the most complex. Furthermore, if this method is to be applied to saline samples with conductivities greater than 2.5 dS/m, it is necessary to remove sulphated salts by other means before the analyses.
For its part, the acetone method, the reference method used in the main classification systems (SSS- NRCS 2014 and WRB 2014–15), although it seems to give correct values for low amounts of gypsum (i.e. less than 15%), it is clearly inaccurate when gypsum is the main component of the horizon, since it underestimates gypsum contents. In addition, the results can be erroneous if the sample contains other sulphates that react with acetone.
We demonstrate that gypsum can be fully quantified using relatively simple and cheap procedures, and that this quantification is sufficiently precise for many purposes. However, when methods capable of detecting very low amounts of gypsum (less than 2%) are required, more complex and expensive methods such as acetone, turbidimetry or thermogravimetry will have to be chosen.
Data Availability Statement
The original contributions presented in the study are included in the article/Supplementary Material, further inquiries can be directed to the corresponding authors.
Author contributions
DA: Carrying out laboratory analyses, obtaining results and writing the manuscript. MA: Collaboration with laboratory analyzes and help in the revision. SP: Collaboration with laboratory analyzes and help in the revision. RRO: Field description and help in the final writing. JRO: Field description and help in the final writing. RMP: Tutoring and guide of the paper.
Funding
This publication is part of the I+D+i RTI 2018-094927-B-I00 project, funded by the Spanish MCIN/AEI/10.13039/501100011033/and FEDER “A way to make Europe”.
Conflict of Interest
The authors declare that the research was conducted in the absence of any commercial or financial relationships that could be construed as a potential conflict of interest.
Supplementary Material
The Supplementary Material for this article can be found online at: https://www.frontierspartnerships.org/articles/10.3389/sjss.2022.10669/full#supplementary-material
Supplementary Table S1 | Types of gypsum accumulations in soils (partly based on Poch et al., 2018).
Supplementary Table S2 | Selected field and chemical characteristics of the horizons of the Mas de Caspolí (Mequinensa) profile (CBDSA, 1983).
Supplementary Table S3 | Percentage and average of fractions of fine earth (<2 mm) and coarse fraction (>2 mm) of the studied horizons.
References
Al-Muktar, A. O. (1987). Mapping and Microscopic investigation of the Gypsiferous Soils in the Dour and Jezir-J area of Iraq. PhD thesis. RUG.
Artieda, O. (1993). Factores geológicos que inciden en el desarrollo de los suelos en un medio semiá rido. El caso de Quinto, Zaragoza. (In Spanish.Spain: Univ. of Zaragoza. M.S. Thesis.
Artieda, O. (1996). Génesis y distribución de suelos en un medio semiárido. Madrid: Quinto, Zaragoza. Ministerio de Agricultura, Pesca y Alimentación.
Artieda, O., Herrero, J., and Drohan, P. J. (2006). Refinement of the Differential Water Loss Method for Gypsum Determination in Soils. Soil Sci. Soc. Am. J. 70 (6), 1932–1935. doi:10.2136/sssaj2006.0043N
Burns, D. T., Danzer, K., and Townshend, A. (2002). Use of the Terms “recovery” and “Apparent Recovery” in Analytical Procedures. Pure Appl. Chem. 74, 2201–2205.
Casby-Horton, S., Herrero, J., and Rolong, N. A. (2015). Gypsum Soils—Their Morphology, Classification, Function, and Landscapes. Adv. Agron. 130, 231–290. doi:10.1016/bs.agron.2014.10.002
CBDSA- Comisión del Banco de Datos de Suelos y Aguas (1983). Sinedares: sistema de información edafológica y agronómica de España: manual para la descripción codificada de suelos en el campo. Ministerio de Agricultura, Pesca y Alimentación.
Dekkiche, B. (1974). Contribution à l'étude des sols du Hodna et géochimique des eaux de la nappe: Thèse Doctorale. Belgium: U. Ghent.
Eswaran, H., Ilaiwi, M., and Osman, A. (1981). Mineralogy and Micromorphology of Aridisols. Proc. 3rd Int. Soil Classif. Workshop, ACSAD, Damascus, 153–170.
FAO (1988). FAO/UNESCO Soil Map of the World, Revised Legend. World Resources Report 60, FAO Rome. Reprinted as Technical Paper 20. Wageningen: ISRIC, 138.
Halitim, A. (1985). Contribution a l’étude des sols des zones arides (hautes plaines steppiques de l’Algerie): morphologie, distribution et role des sels dans la genese et le comportement des sols. Chapitre 111: Les accumulations gypseuses. Sciences du Vivant [q-bio]. Français: Thèse Doctorale. Ecole Nationale Supérieure Agronomique. tel-02858263.
Herrero, J., Artieda, O., and Hudnall, W. H. (2009). Gypsum, a Tricky Material. Soil Sci. Soc. Am. J. 73 (6), 1757–1763. doi:10.2136/sssaj2008.0224
Herrero, J., Artieda, O., and Weindorf, D. C. (2020). Soil Gypsum Determination. Soil Sci. Soc. Am. J. 84 (5), 1477–1484. doi:10.1002/saj2.20156
Herrero, J., and Porta, J. (1991). Aridisols of Spain. Proc. VI Int. Soil Correlation Meeting. Lincoln: USDA-SCS, 61–66.
Herrero, J., Porta, J., and Fedoroff, N. (1987). “Gypsiferous Soils in the North-East of Spain,” in Soil Micromorphology", AFES-Plaisir. Editor N. Fédoroff, 187–192.
Herrero, J., Porta, J., and Fedoroff, N. (1992). Hypergypsic Soil Micromorphology and Landscape Relationships. Soil Sci. Soc. Am. J. 56. doi:10.2136/sssaj1992.03615995005600040031x
Herrero, J. (2004). Revisiting the Definitions of Gypsic and Petrogypsic Horizons in Soil Taxonomy and World Reference Base for Soil Resources. Geoderma 120 (1–2), 1–5. doi:10.1016/j.geoderma.2003.07.004
Hirst, C. T., and Greaves, J. E. (1922). Factors Influencing the Determination of Sulphates in Soil. Soil Sci. 13 (4), 231–250. doi:10.1097/00010694-192204000-00001
Horton, A. D., and Thomason, P. F. (1951). Polarographic Determinationof Sulphate. Anal. Chem. 23 (12), 1859–1860. doi:10.1021/ac60060a038
Ilaiwi, M. (1983). Contribution to the Knowledge of the Soils of Syria. Ph. D. Thcsis. Belgium: Universiteit Gent, 259. + Annexes.
Kulkc, H. (1974). Zur Geologie und Mineralogie der Knlk- und Gipskrusten Algeriens. Geologische Rundschau 63, 970–998.
Lagerwerff, J. V., Akin, G. W., and Moses, S. W. (1965). Detection and Determination of Gypsum in Soils. Soil Sci. Soc. Am. J. 29, 535–540. doi:10.2136/sssaj1965.03615995002900050019x
Lebron, I., Herrero, J., and Robinson, D. A. (2009). Determination of Gypsum Content in Dryland Soils Exploiting the Gypsum–Bassanite Phase Change. Soil Sci. Soc. Am. J. 73 (2), 403–411. doi:10.2136/sssaj2008.0001
Loeppert, R. H., and Suarez, D. L. (1996). “Carbonate and Gypsum,” in Methods of Soil Analysis. Part 3 Chemical Methods. SSSA Book Series No. 5. Editor D. L. Sparks (Madison, WI: SSSA), 437–474.
Nelson, R. E. (1982). “Carbonate and Gypsum,” in Methods of Soil Analysis. Part 2. Chemical and Microbiological Properties. Editors A. L. Page, R. H. Miller, and D. R. Keeney. 2nd ed. (Madison, WI: Agron. Monogr. 9. ASA and SSSA), 181–197.
Nelson, R. E., Klameth, L. C., and Nettleton, W. D. (1978). Determining Soil Gypsum Content and Expressing Properties of Gypsiferous Soils. Soil Sci. Soc. Am. J. 42, 659–661. doi:10.2136/sssaj1978.03615995004200040025x
Omran, E. S. E. (2016). A Simple Model for Rapid Gypsum Determination in Arid Soils. Model. Earth Syst. Environ. 2 (4), 1–12. doi:10.1007/s40808-016-0242-3
Poch, R. M., Rodríguez-Ochoa, R., Artieda, O., Balasch, J. C., and Boixadera, J. (2021). Silt-Sized Sediments and Gypsum on Surface Formations in the Ebro Basin: a Disambiguation of the Term "gypsiferous Silts. Geol. Acta 19, 2021. doi:10.1344/GeologicaActa2021.19.8
Poch, R. M., Artieda, O., and Lebedeva, M. (2018). “Gypsic Features,” in Interpretation of Micromorphological Features of Soils and Regoliths. Editors G. Stoops, V. Marcelino, and F. Mees (Elsevier), 259–287.
Poch, R. M. (1992). Characteristics of the Sand Fraction of Two Hypergypsic Horizons of 'El Pla d'Urgell' NE (Spain). Egypt. J. Soil Sci. 31 (4), 523–535.
Porta, J., and Herrero, J. (1988). Micromorfología de suelos con yeso. An.Edafol. Agrobiol. T. XLVII (1-2), 179–197.
Porta, J. (1986). Edafogénesis de suelos yesíferos en medio semiárido. Dep. Lleida: Meteorología i Ciencia del Sol - ETSEA Lleida, 136.
Porta, J., and López-Acevedo, M. (2005). Agenda de campo de suelos: información de suelos para la agricultura y el medio ambiente. Madrid: Mundi-Prensa Libros.
Porta, J., López-Acevedo, M., and Rodríguez-Ochoa, R. (1986). “Técnicas y experimentos en edafología In Spanish,” in Colegio Oficial Ingenieros Agrónomos Lleida: Barcelona, Spain.
Porta, J. (1998). Methodologies for the Analysis and Characterization of Gypsum in Soils: a Review. Geoderma 87 (1-2), 31–46. doi:10.1016/s0016-7061(98)00067-6
Soil Survey Staff (1990). “Keys to Soil Taxonomy,” in SMSS Tech.Monogr. 19. 4th ed. (Blacksburg: Virg. Polytech. and State Univ.).
Stoops, G., and Ilaiwi, M. (1981). “Gypsum in Arid Soils: Morphology and Genesis,” in Proceedings of the Third International Soil Classification Workshop. The Arab Center for the Studies of Arid Zones and Dry Lands (ACSAD): Damascus. Editors F. H. Beinroth, and A. Osman, 175–178.
U.S. Salinity Laboratory Staff (1954). Diagnosis and Improvement of Saline and Alkali Soils. Agric. Handb. 60, 175.
Van Reeuwijk, L. P. (Editor) (1987). Procedures for Soil Analysis (Wageningen, the Netherlands: Int. Soil Ref. Inf. Ctr.).
Watson, A. (1985). Structure, Chemistry and Origins of Gypsum Crusts in Southern Tunisia and the Central Namib Desert. Sedimentology 32, 855–875. doi:10.1111/j.1365-3091.1985.tb00737.x
Keywords: soil classification, gypsum, secondary accumulations, loss of water crystallization, turbidimetry, analytical protocol
Citation: Álvarez D, Antúnez M, Porras S, Rodríguez-Ochoa R, Olarieta JR and Poch RM (2022) Quantification of Gypsum in Soils: Methodological Proposal. Span. J. Soil Sci. 12:10669. doi: 10.3389/sjss.2022.10669
Received: 24 May 2022; Accepted: 05 October 2022;
Published: 18 October 2022.
Edited by:
Engracia Madejon, Institute of Natural Resources and Agrobiology of Seville (CSIC), SpainCopyright © 2022 Álvarez, Antúnez, Porras, Rodríguez-Ochoa, Olarieta and Poch. This is an open-access article distributed under the terms of the Creative Commons Attribution License (CC BY). The use, distribution or reproduction in other forums is permitted, provided the original author(s) and the copyright owner(s) are credited and that the original publication in this journal is cited, in accordance with accepted academic practice. No use, distribution or reproduction is permitted which does not comply with these terms.
*Correspondence: Daniela Álvarez, ZGFuaWVsYS5hbHZhcmV6QHVkbC5jYXQ=; Montserrat Antúnez, bW9udHNlcnJhdC5hbnR1bmV6QHVkbC5jYXQ=; Silvia Porras, c2lsdmlhLnBvcnJhc0B1ZGwuY2F0; Rafael Rodríguez-Ochoa, cmFmYWVsLnJvZHJpZ3VlekB1ZGwuY2F0; José Ramón Olarieta, am9zZXJhbW9uLm9sYXJpZXRhQHVkbC5jYXQ=; Rosa M. Poch, Um9zYS5Qb2NoQHVkbC5jYXQ=