- 1Department of Soil Science and Agricultural Chemistry, Engineering Polytechnic School, University of Santiago de Compostela, Lugo, Spain
- 2Department of Analytical Chemistry, Nutrition and Bromatology, Faculty of Veterinary Medicine, University of Santiago de Compostela, Lugo, Spain
- 3Soil Science and Agricultural Chemistry, Faculty of Sciences, University of Vigo, Vigo, Spain
The huge worldwide use of antibiotics triggers the accumulation of these substances in sludge from wastewater treatment plants (WWTP) and the possible contamination of soils amended with it, as well as of crops growing in these soils. In this work we analyzed the presence of the antibiotics amoxicillin (AMO), cefuroxime (CEF), ciprofloxacin (CIP), clarithromycin (CLA), levofloxacin (LEV), lincomycin (LIN), norfloxacin (NOR), sulfadiazine (SUL), and trimethoprim (TRI), in sludge from different WWTPs in Galicia (NW Spain), as well as in sludge technically treated by waste-managers, in soils where treated sludge was applied, and in crops (corn and vineyard) growing in the amended areas. The antibiotics were quantified by means of high resolution HPLC-mass-chromatography. The results indicate that almost all the sludge samples contained antibiotics, being ciprofloxacin and levofloxacin the most abundant reaching maximum values of 623 and 893 ng/g, respectively. The sludge treatment significantly reduced the number and the concentrations of antibiotics. In 12% of the soil samples where sludge was applied, some antibiotics were detected, but always in small concentrations. Regarding the crops, no antibiotic was detected in the roots, stalk, leaves and grain of corn, neither in grapes sampled in vineyards. It can be concluded that the treatments currently applied in the WWTPs under study are not totally effective in removing antibiotics from the sludge, although the findings of this research suggest that the additional specific treatment of the sludge derived from these WWTPs is effective in reducing the risk of environmental pollution due to a variety of antibiotics, and specifically in the case of soils amended with these organic materials and crops growing on it.
Introduction
Antibiotics have been widely used in human medicine since the discovering of penicillin in 1928, mainly to treat bacterial infections. Their consumption reaches globally up to 15.7 daily doses (DDDs) per 1,000 people (Sriram et al., 2021) with a slightly higher average for Europe, with 16.4 DDD per 1,000 inhabitants (ECDC, 2021). These pharmaceuticals are also provided as food supplement to animals for growth promotion, although this it is banned in Europe (Spielmeyer, 2018), and for animal health control which can lead to the soil persistence of these compounds after repeated application of manure and slurry (Gros et al., 2019). Globally, between the years 2000–2015, 42.3 billion DDD were consumed by humans and the antibiotics consumption rate increased 39%, being higher in high-income countries (Klein et al., 2018), with amoxicillin and amoxicillin/clavulanic acid being the most frequently consumed antibiotics in most countries (Aldeyab et al., 2020). Within the European Union, the antibiotics more consumed are the ones from the group of β-Lactam antibacterial molecules and penicillins (42.3%) and the general consumption pattern has not significantly change in the period 1997–2017 (Bruyndonckx et al., 2021). The country raking of antibiotic consumption in Europe is led by Greece, with Cyprus and Spain in second and third place, respectively (Bruyndonckx et al., 2021).
After its administration, these compounds are partially metabolized and then excreted, reaching the sewage systems and wastewater treatment plants (WWTP). The sludge generated in the WWTP has a great capacity for adsorbing antibiotics, favoring their accumulation, which has been previously described. Specifically, in a study carried out in China, 21 different antibiotics were detected with concentrations up to 5,800 ng g−1 in two WWTPs (Zhou et al., 2013), and in Sweden 9 different antibiotics were detected in 11 WWTPs (Östman et al., 2017). Some of the most relevant antibiotics present in WWTPs are: ciprofloxacin, appearing in 90% of the sampled WWTP effluents in a European survey (Loos et al., 2013); lincomycin, which is among the three main individual pollutants usually found in concentrations over 10 μg L−1 in the sewage influents in Korea (Behera et al., 2011); and clarithromycin, detected in 73% of sludge samples analyzed in Sweden (Östman et al., 2017) and in effluents from WWTPs in Switzerland (Mcardell et al., 2003). Other antibiotics like levofloxacin (Okuda et al., 2009), norfloxacin (Gao et al., 2012) and trimethoprim (Östman et al., 2017) have been detected as well in the sludge of different WWTPs. Amoxicillin has been detected both in wastewater (Henninger et al., 2001) and in WWTP sludge (Kimosop et al., 2016). Namely, the conventional treatments implemented in WWTPs are not efficient in removing antibiotics from both water and sludge, and additional treatments are required (Sabri et al., 2020). These compounds are considered among the most critical pharmaceuticals and personal care products (PPCPs) regarding environmental risk (Verlicchi and Zambello 2015).
According to the Spanish National Sludge Register, 1.2 million tons of sludge (dry matter) are produced annually in Spain and are considered residues, therefore specific residues regulations are applied related to their disposal and agricultural use (MAMRM, 2009). The sludge should be treated before field application, that in Spain represented around 80% of the sludge use (MAMRM, 2009). These treatments can be applied in the WWTP or after transfer to authorized residue managers. The current normative regulates the maximum concentration of heavy metals allowed in the sludge, meanwhile presence of antibiotics is not controlled. Despite the regulations regarding sludge management, antibiotics might end up in agricultural soils amended with the sludge, and therefore available to the crops growing in these soils (Verlicchi and Zambello 2015). The plants would tend to accumulate antibiotics in the roots, after uptake from the soil solution (Azanu et al., 2016). The presence of these pharmaceuticals has been identified as a new threat due to the application of sewage sludge in agriculture (Ivanová et al., 2018), generating risks for the consumers (Buta et al., 2021). Several studies have detected antibiotic uptake in crops like carrot and barley (Eggen et al., 2011), corn, green onion and cabbage (Kumar et al., 2005), lettuce and radish (Bassil et al., 2013) and grass, corn and wheat (Conde-Cid et al., 2018). It has been shown that different antibiotics may also impact on the soil microbial biomass (Santás-Miguel et al., 2020), and that their degradation in the soil environment is affected by variables such as soil pH, humic acids and salts (Rodríguez-López et al., 2022), which could substantially change after sludge spreading.
The aim of the current research is to screen the presence of antibiotics in sludge from different WWTPs in Galicia (NW Spain), as well as in sludge treated by residue managers, in soils where the treated sludge mixtures were applied, and in crops (corn and vine plants) growing on these soils. The study will focus on assessing the eventual success as regards the following aspects: 1) the eventual decrease in the amount of antibiotics present in sewage sludge subjected to technical treatment applied to these residues, and 2) after the spreading of the treated sludge, the concentrations of antibiotics present in soils and crops would remain low. In addition, the research could shed further light on processes taking place and affecting the fate of these emerging pollutants in the environment, which is a matter of real concern as regards environmental and human health.
Materials and Methods
Experimental Design
Sludge samples were collected from 30 different WWTPs in Galicia (NW Spain) (Figure 1) during two sampling campaigns, in spring and autumn of 2019. In the spring campaign, 21 urban WWTP, plus 1 industrial WWTP, were sampled. The urban WWTPs were placed in cities with 65,000–248,000 habitants (A Coruña, Ferrol, Pontevedra, Santiago de Compostela), as well as in villages with above 17,000 habitants (A Estrada, Boiro, Ribeira, Teo, Tui, Sanxenxo, Vilagarcía), and in smaller villages (A Guarda, Ares, Betanzos, Cedeira, Curtis, Miño, Ordes, Ribadeo, Ribadumia). In the second sampling campaign (autumn), 28 WWTP sludge samples were taken (the same as for the first sampling, except Cedeira, Ferrol and Teo 2), plus additional WWTPs from Cambados, Cangas, Moaña, Sada, Lugo, and 3 more industrial WWTPs. The industrial ones belong to the agri-food industry, poultry and canned fish production. Regarding configurations adopted in the treatment lines, all urban WWTPs use both primary (gritting, sieving, sedimentation) and secondary treatments (organic matter removal), while tertiary treatments are applied differently in each WWTP (only UV disinfection is applied is most of them, Supplementary Table S1).
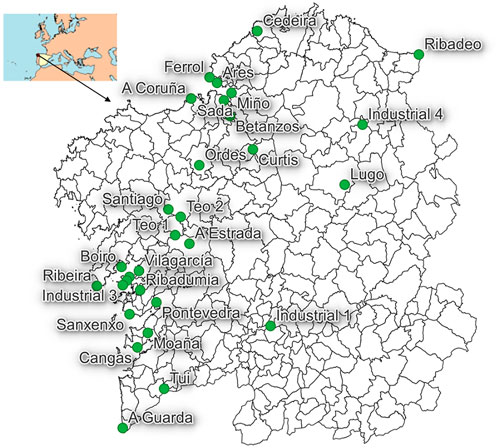
FIGURE 1. Location of the wastewater treatment plants included in the study, all of them in Galicia (NW Spain).
Within Spain, the specific Galician legislation requires the sludge to be treated before being suitable to be used as an amendment in agricultural soils. The sludge generated from different WWTPs is processed by residue managers, generating compost or not composted mixtures. These processed sludge-derived materials were sampled in spring and autumn, from four different residue managers (RM) who produced different mixtures of WWTP sludges with several animal and vegetal by-products (Table 1). These treated mixtures were applied as fertilizers to several agricultural fields, where corn and vineyard were cultivated. Also soils and crops were sampled, as indicated below.
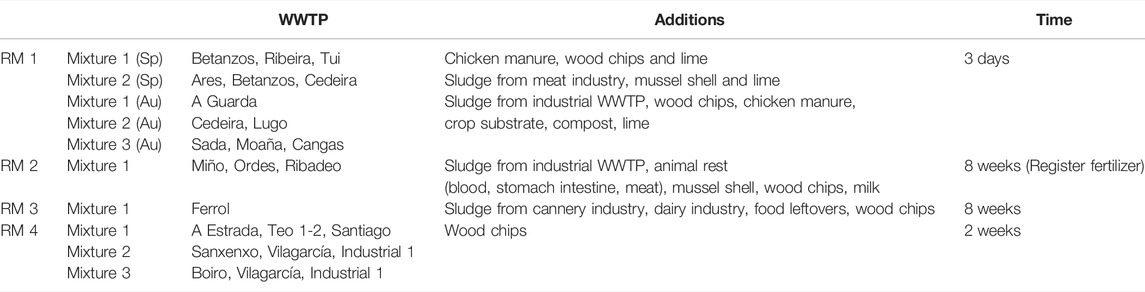
TABLE 1. Treatments applied by the residue managers (RM) to the wastewater treatment plants (WWTP) sludge, by adding and mixing different by-products during certain time. Sp: spring sampling; Au: autumn sampling.
Sludge, Compost, Soil, and Plant Sampling
The WWTP sludge and treated mixtures were sampled at the WWTPs and residue managers facilities, freeze-dried and stored at −4°C until analysis. Soils from amended fields, which had received that kind of fertilization during at least the last 3 years, specifically with the treated mixtures from residue managers (RM) RM1, RM2 and RM4 were sampled at harvest time. A total of 59 amended soils were sampled, 27 under corn (10 amended by RM1, 5 by RM2, and 12 by RM4) and 32 under vineyard (all amended by RM4). The fields were located in different parts of Galicia with (according to the Köppen-Geigen classification) Csb climate (warm temperature with dry and warm summers) and characterized by high precipitations. In each field 10 subsamples were collected in a zigzag transect in the soil surface (depth 0–20 cm) and mixed as a composite sample per field. Soil was dry (at 40°C) until stable weight, sieved (by 2 mm mesh) and stored until analysis. At those same fields vegetal material was sampled at harvest time. In each corn field, three corn plants were randomly selected, and leaves, stem, grains, and roots were taken, making a composite sample per site (Figure 2). In the vineyard fields, three plants were also randomly selected, and several bunches of grapes were sampled from each plant making a composite sample per site. In the laboratory, the roots were washed with distilled water, and in stems and leaves the parts closer to the cob were selected for analysis, discarding the central nerve and the sides in the leaves.
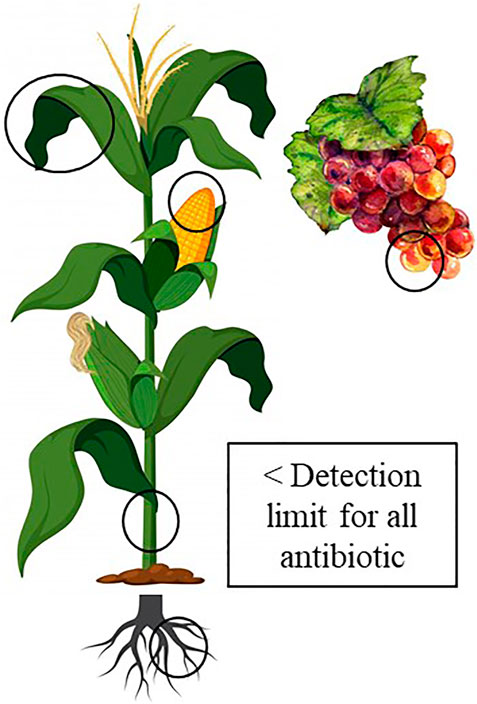
FIGURE 2. Scheme of plant material sampling. It is shown that all the antibiotics studied in plant samples were below their detection limits using high performance HPLC-mass-chromatography.
Physicochemical Analyses of Soil and Sludge Samples
Standard procedures were used to carry out the analyses (Guitián Ojea and Carballas, 1976; Tan, 1996). Soil texture was determined using the international method of Robison Pipette. For both soil and sludge samples, pH values were obtained in water and KCl (1:2.5), using a pH-meter CRISON, model 2001. Total C and N were determined by elemental analysis using a LECO equipment, model TRUSPEC CHNS. Extractable P was measured in soil samples using the Olsen method (Olsen and Sommers, 1982). Soil exchange cations (Ca, Mg, Na, K, Al) were extracted using NH4Cl 1M (Peech et al., 1947) in 1:10 solution. Ca, Mg and Al were measured by atomic absorption spectrophotometry; Na and K were quantified using emission spectrophotometry. The sum of all this exchange cations was considered the effective cation exchange capacity (eCEC) (Kamprath., 1970). Non-crystalline Al and Fe (Blackmore, 1978) and organic Al and Fe (Bascomb, 1968) were measured by atomic absorption after specific extractions. In sludge samples, total element analysis was performed according to the EPA 3051 method, using ICP-MS for measuring Na, Mg, Al, K, P, Ca, Cr, Mn, Fe, Ni, Cu, Zn, As, Cd, Hg and Pb concentrations.
Chemicals, Reagents, and Stock Solutions for the Quantification of the Antibiotics
Amoxicillin, cefuroxime, ciprofloxacin, clarithromycin, levofloxacin, lincomycin, norfloxacin, sulfadiazine, and trimethoprim (purity >98%) and formic acid (purity >99% for analysis) were bought from Sigma-Aldrich (St. Louis, MO, USA). Acetonitrile and methanol HPLC grade were obtained from Merk (Darmstadt, Germany) and Milli-Q water was obtained from a Millipore system (Bedford, MA, USA) installed in the laboratory.
For each antibiotic, an amount of 20 mg was accurately weight (±0.1 mg) and transferred to a 25 ml volumetric flask containing approximately 10 ml of solvent, the volume was finally made up to the graduation mark and the concentration was calculated taking in consideration the purity of the compound. Specifically, ciprofloxacin was dissolved in methanol:water:formic acid (50:49.95:0.05), amoxicillin in water acidified with formic acid, and the rest of antibiotics in methanol. Each day of the analysis a new standard solution of amoxicillin was prepared as this pharmaceutical is very unstable and it degrades easily. The other standard solutions were stored in the dark at −18°C. These stock solutions were mixed and diluted several times with a mixture of methanol and water (50:50) to obtain a working standard solution mixture of antibiotics at 1 and 10 μg ml−1.
Antibiotics Extraction Protocol
The extraction solvent was not the same for all matrices, however, independently of the matrix type, 2 g of sample was weight in a 15 ml Falcon tube. From roots, leaves and soils, the antibiotics were extracted with a mixture of acetonitrile:water:formic acid (50:49.95:0.05), whereas from grapes and stalk were extracted with acetonitrile acidified with 0.2% of formic acid, and from sewage sludge with acetonitrile. After the addition of the extraction solvent, the falcon tubes were shaken on a rotary shaker for 30 min and centrifuged at 4,500 rpm for 15 min. A portion of 1 ml of the supernatant was filtered through an Acrodisk Syringe Filter (Waters, USA) and transferred to a HPLC amber vial for analysis. For each batch of samples and type of matrix (soil, sludge, stalk, roots, leaves and grapes) a matrix-matched calibration curved with analyte-free sample was prepared. The concentration of each antibiotic in the calibration curved varied with the matrix but in average ranged from 10 to 1,000 ng g−1. These calibration curves were used to identify and correctly quantify each of the antibiotics.
Analyses of the Antibiotics by HPLC-MS/MS
The samples were analyzed in an LC-MS/MS system from Bruker (Bremen, Germany) consisting of a UPLC® Elute with degasser, pump, oven, column and autosampler connected to an Evoq Elite triple quadrupole detector. Analytes were separated on an HPLC column Kinetex biphenyl (2.6 µm, 2.1 × 50 mm) from Phenomenex (Torrance, CA, USA). The temperature of the column was set up at 43°C, and the separation of the analytes was achieved on a gradient mode mixture of phase A (0.1% acidified water with formic acid) and phase B (acetonitrile 0.1% acidified with formic acid). The flow was maintained at 375 μL min−1, the column temperature at 43°C, and the samples at 8°C. The mass spectrometer was calibrated as follows: the capillary voltage at 4,800 V, the cone pressure at 20 psi, the cone temperature at 300°C, the source pressure at 30 psi, the source temperature at 500°C, and the misting gas pressure at 60 psi. The injection volume was 15 µL. The MRM transitions monitored for each compound as well as the collision energy employed are compiled in Table 2.
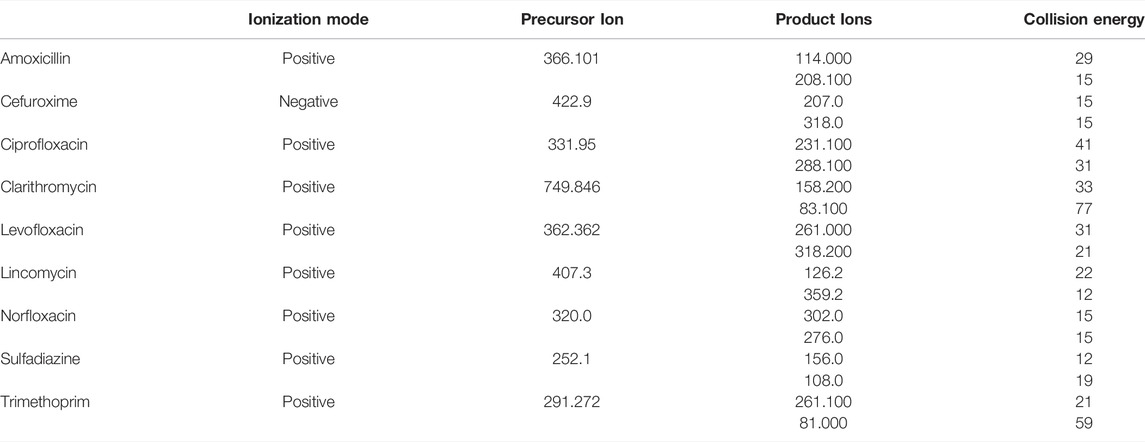
TABLE 2. Ionization mode, precursor and product ion and collision energy employed for pharmaceuticals quantification.
Statistical Treatment
Mean ± SD values of the general soil properties were used to compare the soils amended with different sludges and under different crops. These data were analyzed by performing a standard analysis of variance (ANOVA 1), and in the cases of significant F statistics, the Tukey’s minimum significant different test was used to separate the means, using the statistical package SPSS 27.0 (IBM, USA). For the sludge and mixtures, the study of Pearson correlations (p < 0.01) among concentrations of antibiotics and total elements and physicochemical properties was performed using the R software package (R studio, version 4.1.0).
Results and Discussion
Presence of Antibiotics in Sludge From WWTPs
Altogether in both sampling campaigns (spring and autumn of 2019) seven different antibiotics were detected in the WWTP sludge samples analyzed, with concentrations generally bigger in the first than in the second sampling date. In the first sampling 15 out of 22 of the sludge samples (68%) had at least one antibiotic detected, while 7 of the sludge samples had concentrations below the detection level for all the antibiotics analyzed (Table 3).
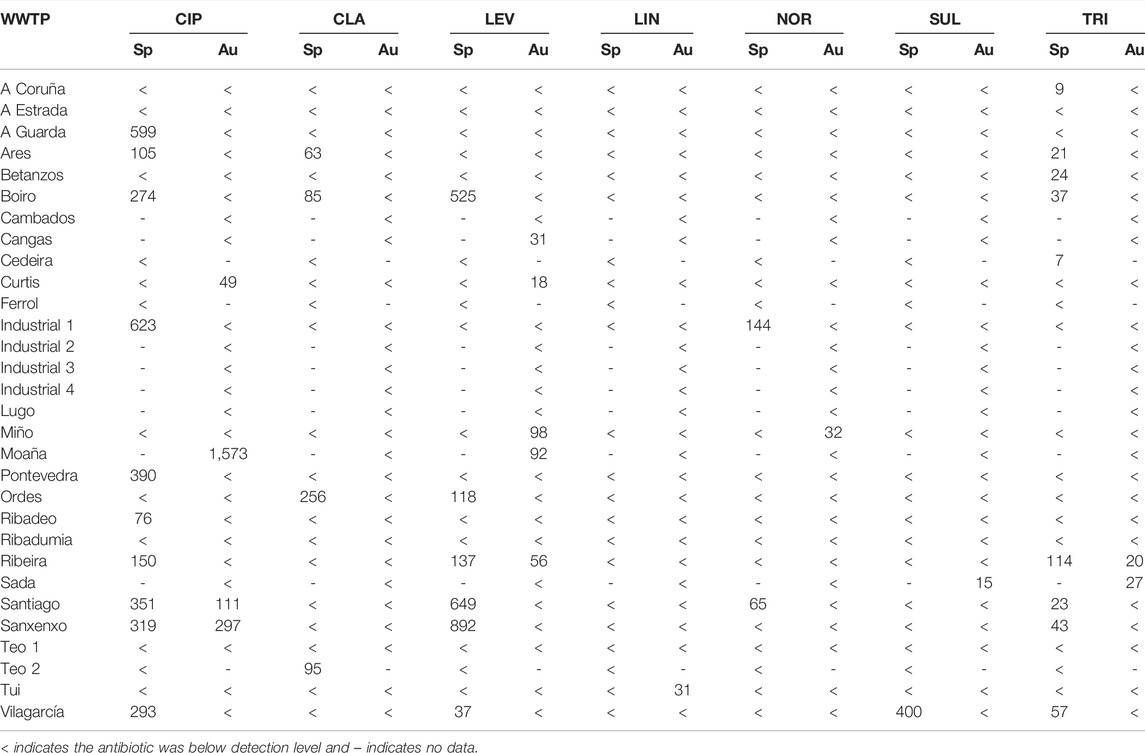
TABLE 3. Concentration (ng g−1) of antibiotic in the sludge from the different WWTP at the spring (Sp) and autumn (Au) sampling time. Antibiotic detected: ciprofloxacin (CIP), clarithromycin (CLA), levofloxacin (LEV), lincomycin (LIN), norfloxacin (NOR), sulfadiazine (SUL), trimethoprim (TRI).
In the second sampling, which was performed in autumn, just 32% of the samples (9 out of 28) presented some antibiotic at detectable concentrations, even though one the samples showed the highest antibiotic concentration detected in the whole study, which reached 1,573 ng g−1 for ciprofloxacin (Table 3). Previous studies have shown that the presence of antibiotics in WWTP sludge has a seasonal pattern. In this regard, Golovko et al. (2014) found in water from WWTPs higher concentrations of different antibiotics in winter than in the rest of the year. In the northern hemisphere, antibiotics consumption peaks between January and March (Van Boeckel et al., 2014), which can lead to a total daily pharmaceutical load in the sludge as high as 1 kg day−1 in that season (Aydın et al., 2022). In the current study, the higher presence of antibiotics in spring than in autumn might be due to this seasonal consumption pattern.
The number of antibiotics detected in the present study was generally lower than values reported by other authors. Aydın et al. (2022) found ten different antibiotics in WWTP sludge in Turkey, with maximum concentrations detected in the same range that in the current study. In different works carried out in China, the maximum concentration of antibiotic detected in sewage sludge was higher than in our study. Zhou et al. (2013) detected 21 different antibiotics with maximum values up to 5,800 ng g−1, 4-fold higher than our maximum; while Huang et al. (2020) described the presence of 24 different antibiotics in WWTP sludge with maximum concentration of the antibiotics reaching up to 11,000 ng g−1, 7-fold higher than our maximum values. Ivanová et al. (2018) reported the presence of 15 different antibiotics in five WWTP in Slovakia, but the maximum concentration was relatively low, reaching 336 ng g−1 for azithromycin, antibiotic which was not detected in our study. On the other hand, Östman et al. (2017) detected only four different antibiotics in sludge samples in Sweden, but with the maximum concentration being 7-fold higher than for our samples. In the same geographic area of our study, the NW of Spain, Conde-Cid et al. (2018) found 8 different antibiotics in 42% of manure samples analyzed, but with maximum concentration values being more than 60-fold higher than those corresponding to our results for sludge.
The WWTP´s with greater number of antibiotics detected in our first sampling were Boiro, Santiago de Compostela and Vilagarcía (Table 3). Ciprofloxacin was the more frequently detected antibiotic in this sampling, present in 45% of the sampled sludge, with a maximum value of 623 ng g−1 detected in the industrial WWTP (Table 3). This same antibiotic showed the maximum concentration detected in our study, found in the second sampling, with a concentration as elevated as 1,573 ng g−1 in Moaña´s WWTP (Table 3). Several authors have described this antibiotic (ciprofloxacin) as one of the dominant emerging contaminants in all environmental compartments, detecting maximum values of 1,040 ng g−1 in sludge (Huang et al., 2020), or founding it in most of the WWTP effluents, with maximum concentration from 264 ng L−1 (Loos et al., 2013) to 2,733 96 ng L−1 (Nas et al., 2021). In the current study, the second most frequent antibiotic detected in the spring sampling was trimethoprim, which was present in 41% of the samples, with maximum values up to 114 ng g−1 in Ribeira´s WWTP sludge (Table 3). In a previous investigation this antibiotic was detected in 93% of the WWTP effluents analyzed in Europe (Loos et al., 2013). In the present work, the high frequency of trimethoprim detected could be related to the lack of a specific tertiary treatment (a sand filtration system) which has been described to reduce 60% of the amount of this antibiotic in activated sludge (Gobel et al., 2005). As shown in Supplementary Table S1, this filtration system was not present in any of the WWTPs of our study.
Among the less frequently detected antibiotics in the spring sampling, levofloxacin appeared just in 6 of the WWTPs (27%) but showed the highest antibiotic concentration detected at this sampling campaign, 892 ng g−1 in Sanxenxo´s WWTP. Other authors have included this antibiotic among the emerging compounds with highest concentration in sludge in WWTPs (Okuda et al., 2009), with values ranging from 204 ng g−1–8,680 ng g−1. Also, the difficulty to remove it by means of the treatments usually applied in WWTPs has been previously described (Martínez-Orgániz et al., 2021). The other antibiotics detected, clarithromycin, norfloxacin and sulfadiazine, were present in 4, 2 and 1 of the samples, respectively, with the maximum value found for the later (reaching 400 ng g−1) in Vilagarcía´s WWTP. Contrarily to our results, other authors detected clarithromycin in 67% of the sludge samples (Aydın et al., 2022), and norfloxacin in 100% of the samples (Gao et al., 2012), with maximum values of 1,496 and 3,200 ng g−1 respectively. Loos et al. (2013), in a study of the WWTP effluents in Europe detected sulfadiazine only in 3% of the samples.
In the second sampling campaign of the current study (autumn) the most frequent antibiotic was levofloxacin, which was present in 5 samples (one of them present in the first sampling as well) with maximum values of 98 ng g−1 in Miño´s WWTP. Ciprofloxacin was present in 4 samples, showing the highest concentration found in this research, and was present as well in the first sampling in 2 of these WWTPs (Table 3). Norfloxacin and trimethoprim appeared in 2 samples, with maximum values of 42 and 27 ng g−1, respectively, while sulfadiazine and lincomycin were present in just one sample (at 15 and 31 ng g−1 respectively). This last one antibiotic only appeared in this sampling, but was found in 37% of the WWTP effluents in an European survey (Loos et a., 2013).
Several previous studies have indicated that the primary and secondary treatments usually applied in WWTPs are not enough for removing many emerging pollutants, such as most antibiotics from both water effluents and sludge (Jelic et al., 2011; Verlicchi et al., 2012; Verlicchi and Zambello 2015; Nguyen et al., 2021), even though some tertiary treatments, like ozone disinfection, can provoke the degradation of macrolide antibiotics such as clarithromycin (Lange et al., 2006). In our study, only one of the WWTPs that presented this antibiotic in the sludge had a disinfection treatment, but was with UV and not ozone, which was not used in any of the WWTPs covered. In our samples the less frequent antibiotics were lincomycin and sulfadiazine. The latest belongs to the sulfonamides group, which have been reported to be easily biodegradable (García-Galán et al., 2011) and transformed during anaerobic sludge digestion (Gobel et al., 2005), a secondary treatment present in most WWTPs.
Presence of Antibiotics in the Sludge Treated by Residue Managers
The sludge treated by the different residue managers (RM) showed a reduced number of antibiotics, compared with the WWTP sludge not subjected to treatment. Specifically, the mixtures from RM1 presented norfloxacin (551 ng g−1) in one of the mixtures from the spring sampling, and lincomycin in the three mixtures from the autumn sampling (maximum value 40 ng g−1) (Table 4). The mixture from RM3 had, similarly to RM1, a high concentration of norfloxacin (587 ng g−1), while no antibiotic was detected in the mixture from RM2. The three mixtures from RM4 sampled in spring showed two antibiotics, ciprofloxacin and levofloxacin, with maximum values of 394 ng g−1 and 393 ng g−1, respectively, while in the autumn sampling only the mixture 3 presented levofloxacin (at 169 ng g−1, Table 4).
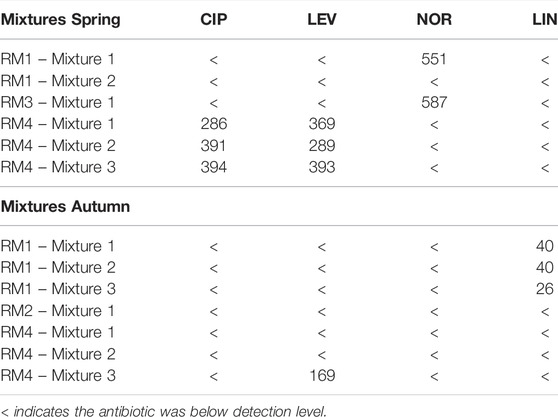
TABLE 4. Concentration (ng g−1) of antibiotic in the sludge mixtures from the different residue managers (RM) at the spring and autumn sampling time. Antibiotic detected: ciprofloxacin (CIP), levofloxacin (LEV), norfloxacin (NOR), lincomycin (LIN).
As indicated by Nguyen et al. (2021) and Jelic et al. (2011), most of the treatments currently applied in WWTPs are not effective for removing antibiotics, some of which are accumulated in sludge, triggering the need of further sludge treatment to be carried out by residue managers. In the current work, this management consisted in the addition of other organic wastes (animal or vegetal) obtaining a final mixture, in some cases subjected to composting. The comparison between the concentrations of antibiotics detected in the sludge used by each RM and the resulting mixture (Figure 3), indicates the effectiveness of the residue management in the antibiotic removal. Specifically, RM1 successfully removed ciprofloxacin, clarithromycin, levofloxacin, sulfadiazine and trimethoprim from the sludge, even though two other antibiotics that were not detected in the sludge (lincomycin and norfloxacin) appeared later in the treated mixtures (Figure 3), the latter in high concentrations, which could be originated in the materials added by this RM. In this regard, it should be noted that the mixture 1, that showed high norfloxacin concentrations, had chicken manure included and poultry production industry uses norfloxacin for the prevention and therapy of infectious diseases in some countries (Al-Mustafa and Al-Ghamdi, 2000). However, in the European Union norfloxacin is used in human medicine, but it is not allowed as a veterinary medicine (Pereira et al., 2018), remaining unclear the origin of the norfloxacin in this mixture in the present work. Also to note that the same RM generates three other mixtures that presented small concentrations of lincomycin, without being this antibiotic present in the urban sludge used for the mixing. Sludge from an additional industrial WWTP was added to these mixtures, suggesting a possible industrial origin of the lincomycin, since this antibiotic has been detected in all the WWTPs analyzed in a study in an industrial city in Korea (Behera et al., 2011). However, in the current study we found it only in one of the urban samples and in none on the industrial ones analyzed, thus its origin also being unclear.
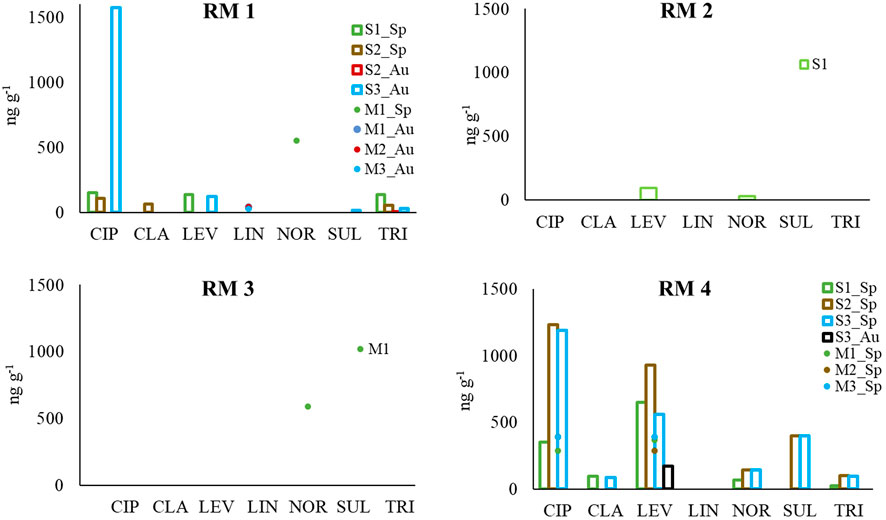
FIGURE 3. Concentration (ng g−1) of antibiotics present in the sludge (S) from wastewater treatment plants (sum of all antibiotics present in the sludge used for each mixture) and in the mixtures (M) generated by different residue managers (RM). Antibiotics detected: ciprofloxacin (CIP), clarithromycin (CLA), levofloxacin (LEV), lincomycin (LIN), norfloxacin (NOR), sulfadiazine (SUL), trimethoprim (TRI).
RM2 successfully removed levofloxacin and norfloxacin from the sludge. The sludge used by RM3 had no antibiotic detected, but the residue management introduced norfloxacin in the treated mixture. This residue manager, like RM1, added different sludge from industrial WWTPs that may introduce antibiotics that were no present in the urban sludge. On the other hand, RM4 managed to remove most of the antibiotics detected in the sludge, and decreases the amount of ciprofloxacin and levofloxacin, which showed high concentrations in the WWTP sludge (Figure 3). This RM carried out a composting process with wood chips. Previous studies reported the removal of levofloxacin from aqueous solutions with a biochar made of wood chips (Yi et al., 2016) and the persistence of ciprofloxacin in composted sludges (Khadra et al., 2019). The compost process is a well-known technology effective in the reduction of antibiotics from sludge, with ranges of removal 17%–100% (Verlicchi and Zambello 2015; Ezzariai et al., 2018). In general, in the current study the management executed by the different residue managers was successful in the removal or reduction of antibiotics concentrations from the WWTPs sludge.
Physicochemical Characteristics of Sludge and Mixtures
The physicochemical properties of the various sludges and mixtures differed widely, with ranges 5-12 for pH, 2.25%–57.47% for C, and 0.75%–60.85% for N (Supplementary Table S2). Similarly, the total elements contents showed great variation among the different sludge and mixture samples, with values (in mg kg−1) between 107-9078 (for Na), 549-6395 (Mg), 143-26106 (Al), 0.6-25.1 (P), 343-8161 (K), 3-230 (Ca), 0-797 (Cr), 25-3595 (Mn), 133-48800 (Fe), 0.9-426 (Ni), 7-1229 (Cu), 0-1777 (Zn), 0.5-120 (As), 0-29 (Cd), 0-447 (Hg), and 0.4-110 (Pb). The WWTPs that presented the highest concentrations of total elements were Lugo (Na and P), Pontevedra (Cr, Fe, and Ni), Curtis (Cu and As), and Ferrol (Hg and Pb) (Supplementary Table S2). Regarding the sludge mixtures, the highest values for most of the elements were detected in mixtures from RM1 (Na, P, K, Ca, Cr, Mn, Ni, Cu, Zn, Cd, Hg and Pb), meanwhile a mixture from RM2 had higher concentration of Fe, and mixtures from RM4 showed the highest scores for Mg, Al, Cr and As (Supplementary Table S2).
Comparing with other studies, the values of pH were in the same range (Onchoke et al., 2022), while another study carried out in the USA with a lower number of WWTPs (Onchoke et al., 2018) showed narrower ranges for C (30–32%) and N (8–10%), and higher values of P (935–1705 mg kg−1), and Ca (1,521–3,869 mg kg−1), but the maximum values of Mg (709 mg kg−1), K (679 mg kg−1), Na (2,303 mg kg−1), Al (12,857 mg kg−1), Cr (20 mg kg−1), Mn (1,103 mg kg−1), Fe (22,688 mg kg−1), Ni (19.8 mg kg−1), Cu (550 mg kg−1), Zn (772 mg kg−1), As (13 mg kg−1), Cd (0.5 mg kg−1), Hg (0.6 mg kg−1), and Pb (34 mg kg−1) were significantly smaller than the maximum concentrations detected in our samples. Similarly, in a study in Poland Latosinska et al. (2021) reported that the maximum concentrations of Cu (196 mg kg−1), Cr (2,760 mg kg−1), Cd (12 mg kg−1), and Ni (44 mg kg−1) in sludge were smaller than in our samples, but Pb (427 mg kg−1) and Zn (5,351 mg kg−1) showed higher concentrations.
According to the Spanish regulation regarding the maximum concentration of heavy metals allowed in sludge to be suitable for field application (Royal Decree 1,310/1990), practically all the sludge samples from the spring sampling had values above the threshold allowed for Hg (16–25 mg kg−1). The values detected in our sludge samples, with maximum of 447 mg kg−1, far outweigh the results from other authors, which were in the range 0.6–6.1 mg kg−1 of total Hg (Mao et al., 2016; Onchoke et al., 2018). The management of the sludge did not manage to remove Hg in three of the spring mixtures (mixtures 1 and 2 from RM1, and RM3 mixture), as they presented Hg concentrations in the range 171–337 mg kg−1. The mixture from RM3 was no applied to the field, but the mixtures from RM4 were spread, which supposes a clear pollution risk.
The fact that the sludge from the industrial WWTPs had Hg values conforming to the regulations, contrary to the urban ones, and that the samples with the highest concentrations belong to the WWTPs of the bigger cities, suggests a domestic origin of these elevated concentration of Hg, such as amalgams, food, laundry detergent, or other, like dentist business of pipe sediments (Sörme et al., 2003). Previous studies correlated the high concentration of Hg detected in cattle in Galicia with the coal-fired power plants present in the area, that caused high Hg dispersion and deposition (López-Alonso et al., 2003), however nowadays these stations have been closed. The concentrations of the other heavy metals across the different WWTPs were among the Spanish regulation limits, with punctual exceptions.
The analysis of the correlations among antibiotics concentrations and chemical properties in sludge and sludge mixtures showed that lincomycin was correlated with pH (R = 0.52, p < 0.001), C/N ratio (R = 0.4, p < 0.05) and P content (R = -0.34, p < 0.05); while trimethoprim was correlated with N (R = -0.32, p = 0.01); and Na (R = 0.34, p < 0.05) (Figure 4). To note that parameters related to organic matter appear in the correlations, evidencing its marked influence on the retention of antibiotics the soil environment. Physicochemical properties are determinant in the adsorption of antibiotics in soils or bio-adsorbents (Cela-Dablanca et al., 2021, 2022), and similar adsorption mechanisms might take place in sludge. The concentrations of some of the antibiotics were positively correlated with each other: trimethoprim with levofloxacin (R = 0.37, p < 0.01) and with sulfadiazine (R = 0.37, p < 0.05), and ciprofloxacin with levofloxacin (R = 0.33, p < 0.01). In this regard, it should be noted that trimethoprim is used against different infections, from urinary to respiratory ones, and the antibiotic levofloxacin has emerged as an alternative for respiratory infections (Sarzynski et al., 2022). On the other hand, trimethoprim is sometimes administrated simultaneously with sulfadiazine to combat bacterial infections in animals, and ciprofloxacin and levofloxacin (both quinolone antibiotics) are used in a wide range of bacterial infections.
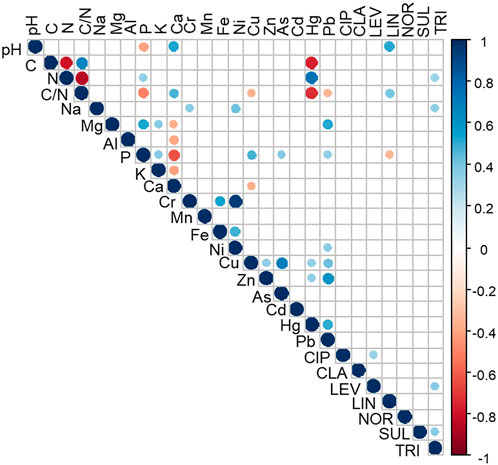
FIGURE 4. Correlations among the sludge and sludge mixtures physicochemical properties, total element concentration and the sludge and sludge mixtures antibiotics concentrations. Blue and red dots show positive or negative (respectively) significant correlation Pearson coefficients (p < 0.01). Ciprofloxacin (CIP), clarithromycin (CLA), levofloxacin (LEV), lincomycin (LIN), norfloxacin (NOR), sulfadiazine (SUL), trimethoprim (TRI).
Impact on Agricultural Soils and Crops due to the Spreading of Treated Sludge
The physicochemical properties of the soils amended with the mixtures treated by the different residue managers are shown in Table 5. Regarding grain sizes and soil texture, the sand fraction clearly predominates, with a percentage above 45% in all cases. Soils amended with mixtures from RM1 (corn) and RM4 (vineyard) show high pH values (7.90 and 7.01, respectively), meanwhile the corn soils amended with mixtures from RM2 and RM4 had an acidic pH (5.32 and 5.53, respectively). The dominant exchange cation was Ca in all cases, and it is especially clear in the RM1-amended soils. These soils also presented the highest values of the other exchange cations, as well as the lowest values of exchangeable Al, coinciding with the highest pH. Also noteworthy are the high values of organic matter in the soils with the mixtures from RM1 (13.04%), while in the other soils the values were lower (between 6 and 6.7%) (Table 5). Non-crystalline Fe compounds (Feox and Fepir) were more abundant in soils amended with RM2 and RM4 (corn). Non-crystalline Al compounds, however, showed higher levels in soils amended with RM1 mixtures.
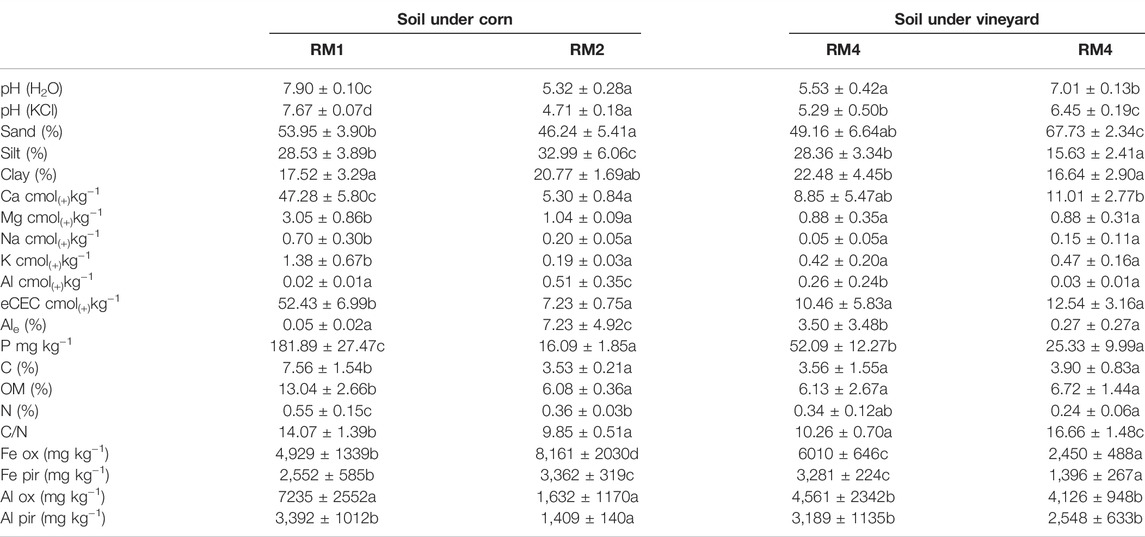
TABLE 5. Physicochemical properties (average ± DE) of soil amended with mixtures from different residue managers (RM) and under corn and vineyard crops. OM (organic matter); eCEC (effective cation exchange capacity); Ale (extractable Al); Fe ox (non-crystalline Fe); Fe pir (organic Fe); Al ox (non-crystalline Al); Al pir (organic Al). Different letters mean significant differences p < 0.05.
Out of the 59 soils sampled, only seven soils showed detectable levels of antibiotics: two soils amended by RM2, and the other five by RM4 (Table 6). The two soils amended by RM2 had corn as crop, and one of them showed a concentration of 57 ng g−1 of amoxicillin, while the other one had 41 ng g−1 of clarithromycin. Regarding the soils amended by RM4, the three soil samples under corn contained the antibiotic cefuroxime, with maximum values of 276 ng g−1, while the other two soils which were placed under vineyard showed small amounts of amoxicillin (Table 6). The mixture from RM2 did not show clarithromycin and the other two antibiotics were not detected in any of the sludge samples, nor for those coming directly from WWTPs, neither after the processing in the residue manager facilities (Tables 3, 4). The presence of antibiotics in these soil samples might be due to their dispersion through contaminated WWTPs effluents (Zuccato et al., 2010) or to the persistence of antibiotics in the soil after several years of sludge application, even though long-term application of sludge in arable lands has been shown to not being a probable cause of antibiotics accumulation in soils (Rutgersson et al., 2020). On the other hand, the continues application of slurry and manure, especially swine slurry, has been demonstrated that contributes building up persistent antibiotic residues in the uppermost layers of the soil (Gros et al., 2019). No antibiotic was detected in any of the leaves, stem, grain, roots or grape samples (Figure 2). The post-WWTP management of the sludge is crucial to avoid soil contamination with antibiotics. Our results, with just 12% of the sampled soils presenting one antibiotic per sample, indicate the efficiency of the different management methods. Conde-Cid et al. (2018) studied the presence of veterinary antibiotics in soils amended with manure, detecting these compounds in 17% of the soil samples, with maximum values of 600 ng g−1, doubling the maximum detected in the soils amended with the managed sludge in the current research. Composting have been proved to reduce antibiotics in both manure and sludge samples (Dolliver et al., 2008; Ezzariai et al., 2018). In the present study, the presence of antibiotics in the mixtures that were lacking in the initial sludge highlights the need of carefully selecting the materials to be combined with the sludge for the composting process. Indeed, the co-composting of contaminated sludges with agricultural wastes has been suggested as a good strategy for the sanitation of compost (Alvarenga et al., 2015). These latter authors mentioned, however, that the risk of heavy metals accumulation driven by the composting process should be considered.
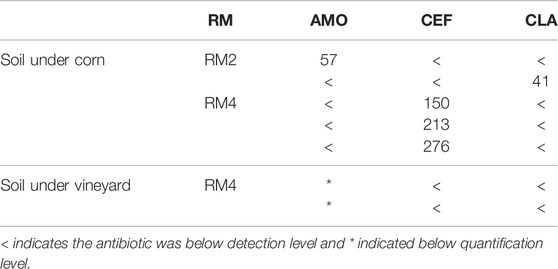
TABLE 6. Concentration (ng g−1) of antibiotic in soils under corn and vineyard crops and amended with compost/mixtures from the different residue managers (RM). Antibiotic detected: amoxicillin (AMO), cefuroxime (CEF), clarithromycin (CLA).
In previous investigations, different crops growing in manure-applied soils have shown antibiotic uptake (Bassil et al., 2013), but in some cases the values were below the quantification limit (Kang et al., 2013). In Galicia, previous studies showed that 44% of the crops amended with manure had detectable concentrations of veterinary antibiotics (Conde-Cid et al., 2018), these crops including grass, corn and wheat samples, with maximum values detected in corn, reaching up to 600 ng g−1 for some veterinary antibiotics. The absolute absence of any antibiotic detected in plant material in the current research highlights the importance of the residue management before application to agricultural fields. In this sense, the spreading of manure, contrarily to what happens with sludge, is not regulated, and no management its required before field application in Spain, with less than 10% of the total animal manure being processed at the European level (Foged et al., 2011). It is possible that the situation in other regions and countries differ from this, although it means that we need to do further efforts regarding emerging pollutants monitoring. The impact of antibiotic contamination includes the presence of ARGs (antibiotic resistance genes) that has been reported in crops growing in soils where un-treated WWTP sludge was applied (Rahube et al., 2016), which is a real issue of concern as regards environmental and human health.
Conclusion
The treatments applied in the wastewater treatment plants facilities investigated in the current study were not effective in removing the variety of antibiotics used in human medicine that were determined, which were specifically accumulated in sludge. In fact, most of the sludge studied contained some antibiotics, especially in the spring sampling, which is related to the greater consumption of these drugs in certain months. The antibiotics most frequently detected and in the greatest quantity in these sludge materials were ciprofloxacin and levofloxacin. The lack of specific regulation regarding these emergent pollutants in sludge and soils makes this an environmental problem needing further efforts to be correctly addressed. Our findings suggest that additional treatments of the sludge derived from these WWTP, carried out by waste managers, are effective in reducing the risk of environmental pollution due to antibiotics, specifically in the case of soils amended with these organic materials, preventing their entry in the food chain through the crops that grow in those agricultural soils.
Data Availability Statement
The original contributions presented in the study are included in the article/Supplementary Material, further inquiries can be directed to the corresponding author.
Author Contributions
All authors listed have made a substantial, direct, and intellectual contribution to the work and approved it for publication.
Funding
This work was supported by the Spanish Ministry of Economy and Competitiveness (grant numbers RTI2018-099574-BC21 and RTI2018-099574-B-C22), with European Regional Development Funds (FEDER in Spain). RL-L holds a predoctoral FPU contract (FPU19/03758 Ministry of Education and Professional Formation).
Conflict of Interest
The authors declare that the research was conducted in the absence of any commercial or financial relationships that could be construed as a potential conflict of interest.
Supplementary Material
The Supplementary Material for this article can be found online at: https://www.frontierspartnerships.org/articles/10.3389/sjss.2022.10741/full#supplementary-material
References
Al-Mustafa, Z. H., and Al-Ghamdi, M. S. (2000). Use of Norfloxacin in Poultry Production in the Eastern Province of Saudi Arabia and its Possible Impact on Public Health. Int. J. Environ. Health Res. 10, 291–299. doi:10.1080/0960312002001483
Aldeyab, M., López-Lozano, J. M., and Gould, I. M. (2020). “Global Antibiotics Use and Resistance,” in Global Pharmaceutical Policy. Editor Z. Babar (Singapore: Palgrave Macmillan), 331.
Alvarenga, P., Mourinha, C., Farto, M., Santos, T., Palma, P., Sengo, J., et al. (2015). Sewage Sludge, Compost and Other Representative Organic Wastes as Agricultural Soil Amendments: Benefits versus Limiting Factors. Waste Manag. 40, 44–52. doi:10.1016/j.wasman.2015.01.027
Aydın, S., Ulvi, A., Bedük, F., and Aydın, M. E. (2022). Pharmaceutical Residues in Digested Sewage Sludge: Occurrence, Seasonal Variation and Risk Assessment for Soil. Sci. Total Environ. 817, 152864. doi:10.1016/j.scitotenv.2021.152864
Azanu, D., Mortey, C., Darko, G., Juhl Weisser, J., Styrishave, B., and Abaidoo, R. C. (2016). Uptake of Antibiotics from Irrigation Water by Plants. Chemosphere 157, 107–114. doi:10.1016/j.chemosphere.2016.05.035
Bascomb, C. (1968). Distribution of Pyrophosphate-Extractable Iron and Organic Carbon in Soils of Various Groups. J. Soil Sci. 19, 251–268. doi:10.1111/j.1365-2389.1968.tb01538.x
Bassil, R. J., Bashour, , Sleiman, F. T., and Abou-Jawdeh, Y. A. (2013). Antibiotic Uptake by Plants from Manure-Amended Soils. J. Environ. Sci. Health Part B 48, 570–574. doi:10.1080/03601234.2013.774898
Behera, S. K., Kim, H. W., Oh, J. E., and Park, H. S. (2011). Occurrence and Removal of Antibiotics, Hormones and Several Other Pharmaceuticals in Wastewater Treatment Plants of the Largest Industrial City of Korea. Sci. Total Environ. 409, 4351–4360. doi:10.1016/j.scitotenv.2011.07.015
Blackmore, L. C. (1978). “Exchange Complex Dominated by Amorphous Material (ECDAM),” in The Andisol Proposal. Editor G. D. Smith (Lower Hutt, New Zealand: New Zealand Soil Bureau), 21.
Bruyndonckx, R., Adriaenssens, N., Versporten, A., Hens, N., Monnet, D. L., Molenberghs, G., et al. (2021). Consumption of Antibiotics in the Community, European Union/European Economic Area. J. Antim Chemo, 76. Supplement_2 ii7-ii13. doi:10.1093/jac/dkab172
Buta, M., Hubeny, J., Zieliński, W., Harnisz, M., and Korzeniewska, E. (2021). Sewage Sludge in Agriculture – the Effects of Selected Chemical Pollutants and Emerging Genetic Resistance Determinants on the Quality of Soil and Crops – a Review. Ecotoxicol. Environ. Saf. 214, 112070. doi:10.1016/j.ecoenv.2021.112070
Cela-Dablanca, R., Barreiro, A., López, L. R., Santás-Miguel, V., Arias-Estévez, M., Núñez-Delgado, A., et al. (2022). Relevance of Sorption in Bio-Reduction of Amoxicillin Taking Place in Forest and Crop Soils. Environ. Res. 208, 112753. doi:10.1016/j.envres.2022.112753
Cela-Dablanca, R., Nebot, C., López, L. R., Fernández-Calviño, D., Arias-Estévez, M., Núñez-Delgado, A., et al. (2021). Efficacy of Different Waste and By-Products from Forest and Food Industries in the Removal/retention of the Antibiotic Cefuroxime. Processes 9, 1151. doi:10.3390/pr9071151
Conde-Cid, M., Álvarez-Esmorís, C., Paradelo-Núñez, R., Nóvoa-Muñoz, J. C., Arias-Estévez, M., Álvarez-Rodríguez, E., et al. (2018). Ocurrence of Tetracyclines and Sulfonamides in Manures, Agricultural Soils and Crops from Different Areas in Galicia (NW Spain). J. Clean. Prod. 197, 491–500. doi:10.1016/j.jclepro.2018.06.217.2
Dolliver, H., Gupta, S., and Noll, S. (2008). Antibiotic Degradation during Manure Composting. J. Environ. Qual. 37, 1245–1253. doi:10.2134/jeq2007.0399
ECDC (2021). European Centre for Disease Prevention and Control. Antimicrobial Consumption in the EU/EEA (ESAC-Net). Stockholm: Annual Epidemiological Report 2020.
Eggen, T., Asp, T. N., Grave, K., and Hormazabal, V. (2011). Uptake and Translocation of Metformin, Ciprofloxacin and Narasin in Forage- and Crop Plants. Chemosphere 85, 26–33. doi:10.1016/j.chemosphere.2011.06.041
Ezzariai, A., Hafidi, M., Khadra, A., Aemig, Q., El Fels, L., Barret, M., et al. (2018). Human and Veterinary Antibiotics during Composting of Sludge or Manure: Global Perspectives on Persistence, Degradation, and Resistance Genes. J. Hazard. Mat. 359, 465–481. doi:10.1016/j.jhazmat.2018.07.092
Foged, H. L., Flotats, X., Blasi, A. B., Palatsi, J., Magri, A., and Schelde, K. M. (2011). “Inventory of Manure Processing Activities in Europe,”. Technical Report No. I in Concerning ‘Manure Processing Activities in Europe' to the European Commission (Directorate General Environment), 138
Gao, L., Shi, Y., Li, W., Niu, H., Liu, J., and Cai, Y. (2012). Occurrence of Antibiotics in Eight Sewage Treatment Plants in Beijing, China. Chemosphere 86, 665–671. doi:10.1016/j.chemosphere.2011.11.019
García-Galán, M. J., Diaz-Cruz, M. S., and Barceló, D. (2011). Occurrence of Sulfonamide Residues along the Ebro River Basin Removal in Wastewater Treatment Plants and Environmental Impact Assessment. Environ. Int. 37, 462–473. doi:10.1016/j.envint.2010.11.011
Gobel, A., Thomsen, A., McArdell, C. S., Joss, A., and Giger, W. (2005). Occurrence and Sorption Behavior of Sulfonamides, Macrolides, and Trimethoprim in Activated Sludge Treatment. Environ. Sci. Technol. 39, 3981–3989. doi:10.1021/es048550a
Golovko, O., Kumar, V., Fedorova, G., Randak, T., and Grabic, R. (2014). Seasonal Changes in Antibiotics, Antidepressants/psychiatric Drugs, Antihistamines and Lipid Regulators in a Wastewater Treatment Plant. Chemosphere 111, 418–426. doi:10.1016/j.chemosphere.2014.03.132
Gros, M., Mas-Pla, J., Boy-Roura, M., Geli, I., Domingo, F., and Petrović, M. (2019). Veterinary Pharmaceuticals and Antibiotics in Manure and Slurry and Their Fate in Amended Agricultural Soils: Findings from an Experimental Field Site (Baix Empordà, NE Catalonia). Sci. Total Environ. 654, 1337–1349. doi:10.1016/j.scitotenv.2018.11.061
Henninger, E., Herrel, M., Strehl, E., and Kummer, K. (2001). “Emission of Pharmaceuticals, Contrast Media, Disinfectants, and AOX from Hospitals,” in Pharmaceuticals in the Environment Sources, Fate, Effects, and Risks (Berlin: Springer), 29–41.
Huang, F., An, Z., Moran, M. J., and Liu, F. (2020). Recognition of Typical Antibiotic Residues in Environmental Media Related to Groundwater in China (2009-2019). J. Hazard. Mat. 399, 122813. doi:10.1016/j.jhazmat.2020.122813
Ivanová, L., Mackuľak, T., Grabic, R., Golovko, O., Koba, O., Staňová, A. V., et al. (2018). Pharmaceuticals and Illicit Drugs - A New Threat to the Application of Sewage Sludge in Agriculture. Sci. Total Environ. 634, 606–615. doi:10.1016/j.scitotenv.2018.04.001
Jelic, A., Gros, M., Ginebreda, A., Cespedes-Sánchez, R., Ventura, F., Petrovic, M., et al. (2011). Occurrence, Partition and Removal of Pharmaceuticals in Sewage Water and Sludge during Wastewater Treatment. Water Res. 45, 1165–1176. doi:10.1016/j.watres.2010.11.010
Kamprath, E. (1970). Exchangeable Aluminum as a Criterion for Liming Leached Mineral Soils. Soil Sci. Soc. Am. J. 34, 252–254. doi:10.2136/sssaj1970.03615995003400020022x
Kang, D. H., Gupta, S., Rosen, C., Fritz, V., Singh, A., Chander, Y., et al. (2013). Antibiotic Uptake by Vegetable Crops from Manure-Applied Soils. J. Agric. Food Chem. 61, 9992–10001. doi:10.1021/jf404045m
Khadra, A., Ezzariai, A., Merlina, G., Capdeville, M. J., Budzinski, H., Hamdi, H., et al. (2019). Fate of Antibiotics Present in a Primary Sludge of WWTP during Their Co-composting with Palm Wastes. Waste Manag. 84, 13–19. doi:10.1016/j.wasman.2018.11.009
Kimosop, S. J., Getenga, Z. M., Orata, F., Okello, V. A., and Cheruiyot, J. K. (2016). Residue Levels and Discharge Loads of Antibiotics in Wastewater Treatment Plants (WWTPs), Hospital Lagoons, and Rivers within Lake Victoria Basin, Kenya. Environ. Monit. Assess. 188, 532. doi:10.1007/s10661-016-5534-6
Klein, E. Y., Van Boeckel, T., Martinez, E. M., Pant, S., Gandra, S., Levin, S. A., et al. (2018). Global Increase and Geographic Convergence in Antibiotic Consumption between 2000 and 2015. Proc. Natl. Acad. Sci. U. S. A. 115, E3463-E3470–E3470. doi:10.1073/pnas.1717295115
Kumar, K., Gupta, S. C., Baidoo, S. K., Chander, Y., and Rosen, C. J. (2005). Antibiotic Uptake by Plants from Soil Fertilized with Animal Manure. J. Environ. Qual. 34, 2082–2085. doi:10.2134/jeq2005.0026
Lange, F., Cornelissen, S., Kubac, D., Sein, M. M., von Sonntag, J., Hannich, C. B., et al. (2006). Degradation of Macrolide Antibiotics by Ozone: A Mechanistic Case Study with Clarithromycin. Chemosphere 65, 17–23. doi:10.1016/j.chemosphere.2006.03.014
Latosinska, J., Kowalik, R., and Gawdzik, J. (2021). Risk Assessment of Soil Contamination with Heavy Metals from Municipal Sewage Sludge. Appl. Sci. (Basel). 11, 548. doi:10.3390/app11020548
Loos, R., Carvalho, R., António, D. C., Comero, S., Locoro, G., Tavazzi, S., et al. (2013). EU-Wide Monitoring Survey on Emerging Polar Organic Contaminants in Wastewater Treatment Plant Effluents. Water Res. 47, 6475–6487. doi:10.1016/j.watres.2013.08.024
López Alonso, M., Benedito, J. L., Miranda, M., Fernández, J. A., Castillo, C., Hernández, J., et al. (2003). Large-scale Spatial Variation in Mercury Concentrations in Cattle in NW Spain. Environ. Pollut. 125, 173–181. doi:10.1016/S0269-7491(03)00073-3
MAMRM, (2009). Characterization of Wastewater Treatment Plants Sludge Genetared in Spain (In Spanish).” in Ministerio Medio Ambiente Y Medio Rural Y Marino.
Mao, Y., Cheng, L., Ma, B., and Cai, Y. (2016). The Fate of Mercury in Municipal Wastewater Treatment Plants in China: Significance and Implications for Environmental Cycling. J. Hazard. Mat. 306, 1–7. doi:10.1016/j.jhazmat.2015.11.058
Martínez-Orgániz, A., Becerril Bravo, J. E., Llompart, M., Dagnac, T., Lamas, J. P., Vázquez, L., et al. (2021). Emerging Pollutants and Antibiotics Removed by Conventional Activated Sludge Followed by Ultraviolet Radiation in a Municipal Wastewater Treatment Plant in Mexico. Wat Q. Res. J. 56, 167–179. doi:10.2166/wqrj.2021.013
Mcardell, C. S., Molnar, E., Suter, M. J. F., and Giger, W. (2003). Occurrence and Fate of Macrolide Antibiotics in Wastewater Treatment Plants and in the Glatt Valley Watershed, Switzerland. Environ. Sci. Technol. 37, 5479–5486. doi:10.1021/es034368i
Nas, B., Dolu, T., and Koyuncu, S. (2021). Behavior and Removal of Ciprofloxacin and Sulfamethoxazole Antibiotics in Three Different Types of Full-Scale Wastewater Treatment Plants: A Comparative Study. Water Air Soil Pollut. 232, 127. doi:10.1007/s11270-021-05067-6
Nguyen, A. Q., Vu, H. P., Nguyen, L. N., Wang, Q., Djordjevic, S. P., Donner, E., et al. (2021). Monitoring Antibiotic Resistance Genes in Wastewater Treatment: Current Strategies and Future Challenges. Sci. Total Environ. 783, 146964. doi:10.1016/j.scitotenv.2021.146964
Okuda, T., Yamashita, N., Tanaka, H., Matsukawa, H., and Tanabe, K. (2009). Development of Extraction Method of Pharmaceuticals and Their Occurrences Found in Japanese Wastewater Treatment Plants. Environ. Int. 35, 815–820. doi:10.1016/j.envint.2009.01.006
Olsen, S. R., and Sommers, L. E. (1982). “Phosphorus,” in Methods of Soil Analysis. Part 2. Chemical and Microbiological Properties. Editor A. L. Page (Madison, WI: Agronomy Monographs), 9.
Onchoke, K. K., Franclemont, C. M., and Weatherford, P. W. (2018). Structural Characterization and Evaluation of Municipal Wastewater Sludge (Biosolids) from Two Rural Wastewater Treatment Plants in East Texas, USA. Spectrochimica Acta Part A Mol. Biomol. Spectrosc. 204, 514–524. doi:10.1016/j.saa.2018.06.096
Onchoke, K. K., Oluwadamilola, O. F., Friedfeld, R. B., and Weatherford, P. W. (2022). Evaluation and Analysis of Perlite and Municipal Wastewater Sludge (Biosolids) from Three Wastewater Treatment Plants in East Texas, USA. Environ. Monit. Assess. 194, 121. doi:10.1007/s10661-022-09794-z
Östman, M., Lindberg, R. H., Fick, J., Björn, E., and Tysklind, M. (2017). Screening of Biocides, Metals and Antibiotics in Swedish Sewage Sludge and Wastewater. Water Res. 115, 318–328. doi:10.1016/j.watres.2017.03.011
Peech, L., Alexander, L. T., and Dean, L. A. (1947). Methods of Soil Analysis for Soil-Fertility Investigations. Washington D.C. USA: USDA Cir. N° 757.
Pereira, A. M. P. T., Silva, L. J. G., Rodrigues, J., Lino, C., and Pena, A. (2018). Risk Assessment of Fluoroquinolones from Poultry Muscle Consumption: Comparing Healthy Adult and Pre-school Populations. Food Chem. Toxicol. 118, 340–347. doi:10.1016/j.fct.2018.05.035
Rahube, T. O., Marti, R., Scott, A., Tien, Y. C., Murray, R., Sabourin, L., et al. (2016). Persistence of Antibiotic Resistance and Plasmid-Associated Genes in Soil Following Application of Sewage Sludge and Abundance on Vegetables at Harvest. Can. J. Microbiol. 62, 600–607. doi:10.1139/cjm-2016-0034
Rodríguez-López, L., Santás-Miguel, V., Núñez-Delgado, A., Álvarez-Rodríguez, E., Pérez-Rodríguez, P., and Arias-Estévez, M. (2022). Influence of pH, Humic Acids, and Salts on the Dissipation of Amoxicillin and Azithromycin under Simulated Sunlight. Span. J. Soil Sci. 12, 10438. doi:10.3389/sjss.2022.10438
Rutgersson, C., Ebmeyer, S., Lassen, S. B., Karkmana, A., Fick, J., Kristiansson, E., et al. (2020). Long-term Application of Swedish Sewage Sludge on Farmland Does Not Cause Clear Changes in the Soil Bacterial Resistome. Environ. Int. 137, 105339. doi:10.1016/j.envint.2019.105339
Sabri, N. A., van Holst, S., Schmitt, H., van der Zaan, B. M., Gerritsen, H. W., Rijnaarts, H. H. M., et al. (2020). Fate of Antibiotics and Antibiotic Resistance Genes during Conventional and Additional Treatment Technologies in Wastewater Treatment Plants. Sci. Total Environ. 741, 140199. doi:10.1016/j.scitotenv.2020.140199
Santás-Miguel, V., Díaz-Raviña, M., Martín, A., García-Campos, E., Barreiro, A., Núñez-Delgado, A., et al. (2020). Medium-term Influence of Tetracyclines on Total and Specific Microbial Biomass in Cultivated Soils of Galicia (NW Spain). Span. J. Soil Sci. 10, 217–232. doi:10.3232/SJSS.2020.V10.N3.05
Sarzynski, S. H., Warner, S., Sun, J., Matsouaka, R., Dekker, J. P., Babiker, A., et al. (2022). Trimethoprim-sulfamethoxazole versus Levofloxacin for Stenotrophomonas Maltophilia Infections: A Retrospective Comparative Effectiveness Study of Electronic Health Records from 154 US Hospitals. Open Forum Infect. Dis. 9, ofab644. doi:10.1093/ofid/ofab644
Sörme, L., Lindqvist, A., and Söderberg, H. (2003). Capacity to Influence Sources of Heavy Metals to Wastewater Treatment Sludge. Environ. Manage. 31, 421–428. doi:10.1007/s00267-002-2810-8
Spielmeyer, A. (2018). Occurrence and Fate of Antibiotics in Manure during Manure Treatments: A Short Review. Sustain. Chem. Pharm. 9, 76–86. doi:10.1016/j.scp.2018.06.004
Sriram, A., Kalanxhi, E., Kapoor, G., Craig, J., Balasubramanian, R., Brar, S., et al. (2021). State of the World'santibiotics 2021: A Global Analysis of Antimicrobial Resistance and its Drivers. Washington DC: Center for Disease Dynamics, Economics & Policy.
Van Boeckel, T. P., Gandra, S., Ashok, A., Caudron, Q., Grenfell, B. T., Levin, S. A., et al. (2014). Global Antibiotic Consumption 2000 to 2010: an Analysis of National Pharmaceutical Sales Data. Lancet Infect. Dis. 14, 742–750. doi:10.1016/S1473-3099(14)70780-7
Verlicchi, P., Al Aukidy, M., and Zambello, E. (2012). Occurrence of Pharmaceutical Compounds in Urban Wastewater: Removal, Mass Load and Environmental Risk after a Secondary Treatment-A Review. Sci. Total Environ. 429, 123–155. doi:10.1016/j.scitotenv.2012.04.028
Verlicchi, P., and Zambello, E. (2015). Pharmaceuticals and Personal Care Products in Untreated and Treated Sewage Sludge: Occurrence and Environmental Risk in the Case of Application on Soil - A Critical Review. Sci. Total Environ. 538, 750–767. doi:10.1016/j.scitotenv.2015.08.108
Yi, S., Gao, B., Sun, Y., Wu, J., Shi, X., Wu, B., et al. (2016). Removal of Levofloxacin from Aqueous Solution Using Rice-Husk and Wood-Chip Biochars. Chemosphere 150, 694–701. doi:10.1016/j.chemosphere.2015.12.112
Zhou, L. J., Ying, G. G., Liu, S., Zhao, J. L., Yang, B., Chen, Z. F., et al. (2013). Occurrence and Fate of Eleven Classes of Antibiotics in Two Typical Wastewater Treatment Plants in South China. Sci. Total Environ. 452-453, 365–376. doi:10.1016/j.scitotenv.2013.03.010
Keywords: antibiotics, composting, crop soils, sludge treatment, wastewater treatment plants
Citation: Barreiro A, Cela-Dablanca R, Nebot C, Rodríguez-López L, Santás-Miguel V, Arias-Estévez M, Fernández-Sanjurjo M, Núñez-Delgado A and Álvarez-Rodríguez E (2022) Occurrence of Nine Antibiotics in Different Kinds of Sewage Sludge, Soils, Corn and Grapes After Sludge Spreading. Span. J. Soil Sci. 12:10741. doi: 10.3389/sjss.2022.10741
Received: 04 July 2022; Accepted: 15 September 2022;
Published: 26 September 2022.
Edited by:
Irene Ortiz-Bernad, University of Granada, SpainCopyright © 2022 Barreiro, Cela-Dablanca, Nebot, Rodríguez-López, Santás-Miguel, Arias-Estévez, Fernández-Sanjurjo, Núñez-Delgado and Álvarez-Rodríguez. This is an open-access article distributed under the terms of the Creative Commons Attribution License (CC BY). The use, distribution or reproduction in other forums is permitted, provided the original author(s) and the copyright owner(s) are credited and that the original publication in this journal is cited, in accordance with accepted academic practice. No use, distribution or reproduction is permitted which does not comply with these terms.
*Correspondence: Ana Barreiro, YW5hLmJhcnJlaXJvLmJ1amFuQHVzYy5lcw==