- 1CRETUS, Departamento de Edafoloxía e Química Agrícola, Facultade de Bioloxía, Universidade de Santiago de Compostela, Santiago de Compostela, Spain
- 2REBUSC, Rede de Estación Biolóxicas da Universidade de Santiago de Compostela, Estación de Bioloxía Mariña da Graña, Ferrol, Spain
The increase in greenhouse gases (GHG) has been constant since the Industrial Revolution. For this reason, different international organizations have devoted special attention to GHG sinks such as terrestrial soils and ecosystems. However, the initially estimated balances did not include the carbon stock associated with ocean waters, coastal soils and sediments, known as blue carbon. Currently, blue carbon sinks are the subject of numerous studies due to the limited information available, especially on the Atlantic coast of the Iberian Peninsula. We studied the organic C stock present in soils and in Zostera marina biomass in the two main meadows in the Ría de Ferrol (O Baño and Castelo de San Felipe). The carbon stock associated with biomass was 0.37 Mg C ha−1, with 0.18 Mg C ha−1 corresponding to the epigeal portion and 0.19 Mg C ha−1 to the hypogeal portion. Soil carbon stock was much higher: 4.11 Mg C ha−1 at a depth of 5 cm and 82.14 Mg C ha−1 at a depth of 1 m. Together with carbon values in biomass, a stock of 82.5 Mg C ha−1 was obtained for the whole Ría de Ferrol. Z. marina accounted for 8.25% of total C in the Ría de Ferrol. These values were higher than those found in other regions. Isotope ratios (δ13C, δ15N) and C/N ratios indicated that the organic C stock in the O Baño soil may receive important organic matter inputs of terrestrial origin, while in San Felipe, it seems to have a marine origin.
Highlights
• Carbon stock in biomass was related to leaf length and shoot density of Z. marina.
• Carbon stock in soil was much higher than in biomass.
• Isotope and elemental ratios indicated the influence of allochthonous sources of soil carbon stock.
Introduction
Human-induced deforestation, whose beginnings date back from preindustrial times, along with fossil fuel burning and changes in land use, are leading to an exponential increase in carbon dioxide (CO2) and other greenhouse effect gases (Mcleod et al., 2011; IPCC, 2021). Thus, the preindustrial baseline atmospheric CO2 concentrations have multiplied by 1.5, going from 280 to 416.58 ppmv, at an annual increase rate of 2.31 ppmv (NOAA, 2021).
Ocean waters are the main carbon reservoir on the Earth’s surface, and coastal ecosystems with vascular plants play a key role as atmospheric CO2 sinks. Blue carbon is the proposed term to designate carbon stored by coastal marine ecosystems, particularly saltmarshes, mangroves, and seagrass meadows (Pendleton et al., 2012; Röhr et al., 2018). These ecosystems occupy only 0.2% of the ocean surface but store more than half the carbon present in terrestrial systems (green C) and 33% of the total carbon stored in oceans (Fourqurean et al., 2012). However, unlike green C, a highly relevant aspect for the study of carbon stocks is the long residence time of blue carbon. Green carbon can be retained for decades, while blue carbon can be stored for millennia due to the anaerobic conditions that are predominant in coastal sedimentary environments, along with other factors (e.g., refractance of organic compounds, toxicity associated with reduced Fe and S forms, etc.) that inhibit decomposition of organic matter (Fourqurean et al., 2012; Pendleton et al., 2012; Herr and Landis, 2016).
Seagrass meadows are formed by 60 phanerogam species included in the order Alismatales (Kuo and Den Hartog, 2001). They are among the most productive ecosystems worldwide, accounting for 15% of the ocean’s net primary production (Duarte and Chiscano, 1999). For this reason, they are considered an essential CO2 sink for climate change mitigation (Duarte et al., 2013). They currently occupy 30 million hectares (ha), but they are experiencing a sharp decline due to anthropogenic action and to the consequences of global change (Duarte et al., 2013). Estimates suggest that 29% of their area has already been lost and, if loss continues to occur at the current rates, 40% will have been lost 100 years from today (Pendleton et al., 2012; Claes, et al., 2022). Their disappearance entails the loss of an effective CO2 sink and the simultaneous release of CO2 and/or methane (CH4) into the atmosphere; the greenhouse effect of the latter gas is 23–25 times higher than that of CO2 (Short and Neckles, 1999; Pendleton et al., 2012; IPCC, 2021).
Despite the evidence supporting their importance in CO2 removal and retention, isotopy and elemental composition both of their biomass and soils are worth taking into account. The proportions of stable isotopes δ15N and δ13C, as well as C/N ratios, are a result of the origins of stored carbon (Prentice et al., 2020). The continental area can act as a carbon source; this has been observed in Zostera marina meadows in temperate areas (Prentice et al., 2020). Seagrass meadows are considered to be hotspots for allochthonous carbon sequestration, since isotopy has shown that only 50% of the carbon stock associated with these ecosystems is produced by phanerogam tissues. However, through the formation of meadows, phanerogams are able to remove particles from the water column and transfer them to the bottom (Kennedy et al., 2010).
Zostera marina is the most widely distributed species among marine phanerogams; in Galicia, it forms vast meadows in intertidal and shallow subtidal areas (Míguez, 2003; Cacabelos et al., 2015; García-Redondo et al., 2019). The Galician coastline features rias and inlets that provide optimal conditions for the growth of Zostera marina (García-Redondo et al., 2019).
The main objectives of this research were to quantify the total C stock (both associated with biomass and present in soils) in the two main Zostera marina meadows in the Ría de Ferrol; to study the variability in total C between the two main meadows in the Ría de Ferrol: O Baño and San Felipe; and to analyze whether said variability is related to plant biometry in meadows and/or to nutrient origin. To this end, the most relevant biometric parameters of Z. marina (i.e., epigeal and hypogeal biomass, leaf length and width), soil composition and properties, organic C content, and isotopic ratios (δ13C, δ15N) and organic C stock (Mg C ha−1) in Z. marina biomass and in soil were determined.
Material and Methods
Study Area
The study area covers the Ría de Ferrol (NW Iberian Peninsula, A Coruña). Its area is 21 km2, and its largest tributary is the Xubia River (Figure 1). Zostera marina occupies a total area of 11.42 ha in the Ría de Ferrol, divided among four meadows: O Baño (9.11 ha), Castelo de San Felipe (1.48 ha), O Segaño (0.66 ha), and San Felipe Núcleo (0.17 ha). The two main meadows have been sampled for this study: O Baño (43° 27′ 26.5″ N, 8° 16′ 0.6″ W) and Castelo de San Felipe (43° 27′ 51.1″ N, 8° 17′ 0.3″ W).
The O Baño meadow is located in the intertidal area within an inlet that is relatively sheltered from marine currents; the granulometry of its substrate is silt. The Z. marina meadow in Castelo de San Felipe is located in the subtidal area, at a depth of 2 m at low tide. Exposure to marine currents is greater in Castelo de San Felipe, and substrate is mostly sandy, with abundant gravel.
Sample Collection
Zostera marina Sampling
Sampling was performed in September 2021 following the instructions in the Blue Carbon Manual (Howard et al., 2014) in order to use standardized methods, thus allowing for result comparison among studies. Ten sampling points were defined in the O Baño meadow, distributed across the meadow following a zigzag pattern. Samples were collected using metal squares with dimensions 30 cm × 30 cm and a depth of 10 cm to collect the epigeal and hypogeal portions, i.e., leaves and rhizomes, respectively. Samples were frozen at 3°C. In the Castelo de San Felipe meadow, six squares with the above described measurements were collected.
Soil Sampling
The same number of soil samples were collected in both meadows. Eighteen soil samples were collected, 10 of which were taken from within the meadows, next to each of the plots from which plant samples were taken; these soil samples were collected using 8 cm wide, 15 cm deep PVC cores. The remaining 8 samples were collected using 5 cm deep PVC cores; 4 of them were collected within the meadow, following the zig-zag method, while the remaining 4 were collected in adjacent areas, at 4 sites around the perimeter of the meadow and outside the meadow, to serve as control samples. pH, electrical conductivity, and redox potential (Eh) were measured in situ using a Hanna multiparameter probe. Samples were then kept at −20°C until analysis in the laboratory.
Sample Analysis
Flood Water Analysis
A Hanna multiparameter probe was used to measure pH, electrical conductivity, redox potential (Eh), and salinity in situ in flood water in the two main meadows.
Plant Analysis
Prior to analysis, Zostera marina samples were washed in distilled water to remove all adhered materials. For each square, the number of bundles was counted to determine density. Subsequently, 15 bundles were randomly selected within each plot and divided into their hypogeal and epigeal portions. The hypogeal portion of each bundle was measured, the number of leaves was counted, and leaves were divided by maturity status from the outside towards the inside of the bundle, where new leaves sprout from the central portion, sheathed in one another. The following measurements were taken for each leaf within the bundle:
1. Category: 1: whole mature leaf, 2: large (>20 cm) immature leaf, 3: small (<20 cm) immature leaf, 4: broken leaf.
2. Leaf length,
3. Length of the petiole or base of the leaf (present only in mature leaves)
4. Leaf width (mid-leaf).
Moreover, biomass was measured as dry weight (at 105°C) both for the epigeal and the hypogeal portion, as well as total biomass. To determine organic C content and to perform isotope and elemental analyses, they were divided in 5-bundle groups (pooled sample), separating the epigeal and hypogeal sections, to reach sufficient weight for the required analyses. Each pool was ground using a Retsch MM400 electrical mill. From each ground hypogeal portion sample, 0.5 g were used to measure carbonate content using a BD brand, LOGL model digital calcimeter. A 6M HCl dissolution was used to dissolve carbonates. Total carbon content in plants was measured by combustion using a LECO CN828 autoanalyzer. Organic C content in the hypogeal portion was determined as the difference between total C content and carbonate content, while for the epigeal portion, total C was considered as organic C. Carbonates were absent from the epigeal portion, contrasting with the presence of carbonates found in the hypogeal portion.
The C stock associated with Zostera marina was calculated by multiplying the three carbon reservoirs (epigeal, hypogeal, and total) times the % of organic carbon and times the area of the plot (900 m2). In order to obtain comparable results, they were expressed in Mg (Mg = metric ton) C ha−1. Total biomass and total organic C were calculated by averaging the values from the hypogeal and epigeal portions.
Isotope ratios for δ13C (‰ vs. VPDB) and δ15N (‰ vs. Air) were analyzed at the University of A Coruña’s Research Support Services (Servicios de apoyo a la investigación, SAI) using a FlashEA1112 autoanalyzer (Thermo Finnigan) coupled with a Deltaplus isotope ratio mass spectrometer (ThermoFinnigan). Nitrogen percentages were also analyzed at the SAI.
Soil Analysis
Out of the 18 soil samples collected at each site, 10 were selected for the following depths: 0–2, 2–4, 4–6, 6–10, and 10–15 cm, and subsequently dried. From each section, 10 g were weighed and sieved to determine the proportion of gravel (>2 mm), coarse sand (2–0.20 mm), fine sand (0.20–0.05 mm), and clay and silt (<0.05 mm). Carbonate content, total C content, organic C content, and δ13C and δ15N isotope ratios, as well as elemental ratios, were determined using the same method as for plants. The remaining 8 samples from each site, 4 from control areas and 4 from meadows, were used to analyze soil bulk density [SBD (g cm−3) = Mass of dry soil (g)/volume (cm3)]. To this end, their height and width were measured, and they were weighed after drying at 105°C. In the 4 control samples, the percentages of carbonates, total C, and organic C present were also measured, along with isotopy and elemental ratios.
To obtain C stock in soil (SCS; Eq. 2), soil C density was first calculated (SCD; Eq. 1) (Howard et al., 2014).
The mean %CO value for the first three sections of the 15 cm deep cores and the apparent density value of 5 cm deep cores were used to calculate the SCS value for the upper 5 cm.
To calculate total SCS in the Ría de Ferrol, SCS values from the Z. marina meadows in the O Segaño and San Felipe Núcleo sites were also included. The values for these two meadows were estimated based on the data obtained for Castelo de San Felipe, which is the closest meadow and shows similar environmental parameters.
Statistical Analysis
A t-Student test was used for biometric parameters of Z. marina, except for those that did not follow a normal distribution, for which a Mann-Whitney test was used. In the case of soil, granulometric data were analyzed using a t-Student test, while C stock data were analyzed using a one-way Anova.
Results
Flood Water Parameters
Flood water from both sites was characterized by similar pH values between sites, as well as similar to seawater (pH ∼8.2; Table 1), and a high ion concentration resulting in a high electrical conductivity (54–55 mS cm−1) and salinity (Table 1), similar to seawater, which suggests a low influence of continental freshwater. Redox potential (Eh) showed mean values over 500 mV, which is typical of oxic environments.
Characterization of Soils of Z. marina Meadows
Soils from the O Baño meadow were characterized by mean pH values close to neutral (pH: 7.2 ± 0.1; Table 2), redox potential values typical of suboxic sedimentary environments (Eh 247 ± 70 mV), and electrical conductivity values notably lower than those of flood water (16 ± 4.3 mS cm−1). Granulometric composition was dominated by the sandy fraction, with an average proportion of 79.4%, which showed high contents of biogenic carbonates (21.0 ± 0.2%; mostly shells), and organic C content was 0.73 ± 0.24%.

TABLE 2. Geochemical characterization and composition of the soils on which Zostera marina grows in the ría de Ferrol.
Soils from the Zostera marina meadow of Castelo San Felipe showed differences with respect to those from the O Baño population. pH (7.9 ± 0.2) and redox potential (Eh: 439 ± 18 mV) were higher, defining an environment with oxic conditions, consistently with their granulometric composition with a greater proportion of sand and lower organic C contents. Another substantial difference was the lower concentration of salts in soils from Castelo San Felipe than in those from O Baño.
Variability in Biometric Parameters of Zostera marina
Significant differences were observed in terms of shoot density (O Baño 351.1 ± 131 shoots m−2; Castelo San Felipe 139 ± 65.1 shoots m−2), leaf width (cm) (O Baño 0.51 ± 0.06 cm; Castelo San Felipe 0.60 ± 0.15 cm), petiole length (cm) (O Baño 9.13 ± 2.12; Castelo San Felipe 14.3 ± 4.77 cm), and leaf category, with category 3 as the predominant one in O Baño and category 4 in Castelo San Felipe. Conversely, no significant differences were found either in the number of leaves (O Baño 5.05 ± 0.44 shoots; Castelo San Felipe 5.16 ± 0.50 shoots), in their length (cm) (O Baño 22.2 ± 6.29; Castelo San Felipe 26.9 ± 8.9 cm; Table 3), or in rhizome length (cm) (O Baño 8.27 ± 1.57; Castelo San Felipe 7.35 ± 0.24 cm). Similarly, no differences were found for epigeal biomass (O Baño 49.4 ± 27.0; Castelo San Felipe 48.1 ± 33.4 g m−2), hypogeal biomass (O Baño 60.2 ± 33.9; Castelo San Felipe 41.5 ± 16.2 g m−2; Table 3), or total biomass (O Baño 109.6 ± 59.1; Castelo San Felipe 89.5 ± 46.3 g m−2; Table 3).
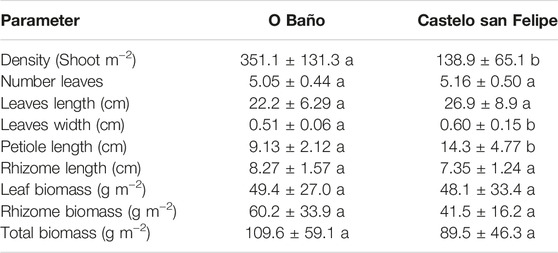
TABLE 3. Biometric characterization of Zostera marina in the two main meadows of the ría de Ferrol. Different letters indicate significant differences between meadows.
Carbon Stock in Plant Biomass and in Soils
The C stock associated with Z. marina biomass did not show significant differences either for epigeal (O Baño 0.18 ± 0.12; San Felipe 0.17 ± 0.13 Mg C ha−1), hypogeal (O Baño 0.21 ± 0.12; Castelo San Felipe 0.12 ± 0.05 Mg C ha−1), or total carbon (O Baño 0.39 ± 0.23; Castelo San Felipe 0.30 ± 0.17 Mg C ha−1) (Figure 2).
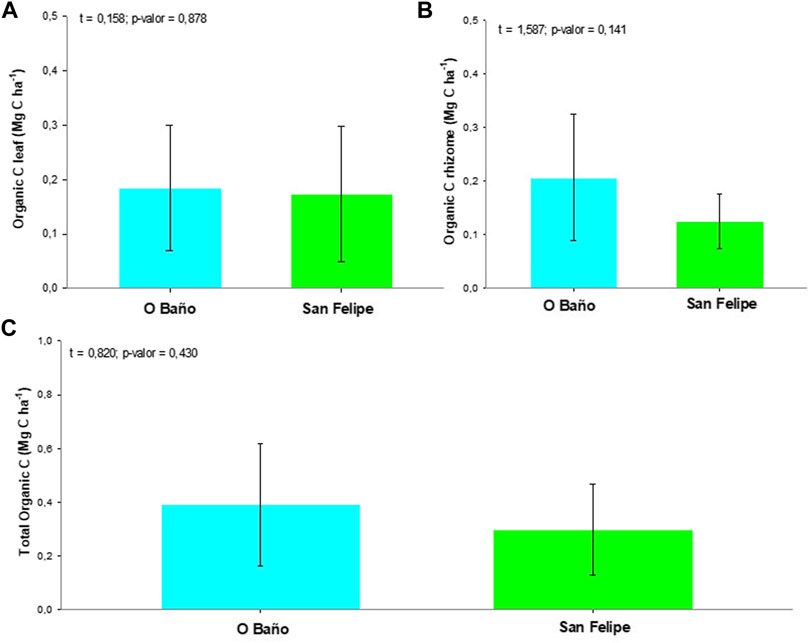
FIGURE 2. Mean organic C ± SD (Mg C ha−1) in each location. (A) Organic C in leaves, (B) in rhizomes, and (C) total.
Carbon stock in soil (Figure 3) was significantly higher in the O Baño Z. marina meadow (4.58 ± 1.46 Mg C ha−1) than in the Castelo San Felipe one (2.27 ± 1.69 Mg C ha−1; t = 3.76; p-value = 0.005). Significant differences were also observed between O Baño and the Castelo San Felipe control site (0.30 ± 0.1 Mg C ha−1; t = 5.26; p-value <0.001). However, no significant differences were observed between the O Baño control site (2.51 ± 0.40 Mg C ha−1) and the Castelo San Felipe meadow (t = 0.29; p-value = 0.78). No significant differences were observed between the control sites (t = 2.27; p-value = 0.06) or between control sites and their corresponding meadows (O Baño t = 2.55; p-value = 0.07; Castelo San Felipe t = 2.42; p-value = 0.07) (Figure 3).
Isotope Ratio (δ13C, δ15N) in Plants and in Soils
No significant differences in terms of δ13C content were observed in any of the sites, either for the epigeal (δ13C: O Baño −8.67 ± 0.51‰; Castelo San Felipe −8.87 ± 1.32‰) or the hypogeal portion of plants (δ13C: O Baño −10.00 ± 0.82‰; Castelo San Felipe −9.83 ± 0.95‰). Likewise, no significant differences in terms of δ15N content were observed for the epigeal (δ5N: O Baño 5.83 ± 0.80‰; San Felipe 6.43 ± 0.49‰) or the hypogeal portion of plants (δ5N: O Baño 5.57 ± 0.31‰; Castelo San Felipe 6.43 ± 0.50‰).
As for soils, δ13C did not show any significant differences between meadows and their matched control sites (δ13C: O Baño −22.25 ± 1.71‰ and O Baño control site −22.17 ± 0.47‰; Castelo San Felipe −5.40 ± 5.41‰ and Castelo San Felipe control site −6.60 ± 2.66‰). However, there were differences between both meadows (δ13C: O Baño −22.25 ± 1.71‰; Castelo San Felipe −5.40 ± 5.41‰). A similar situation was found for δ15N: there were no differences between soils from each meadow and their matched control site, but differences were observed between the two meadows (δ15N: O Baño 5.32 ± 0.075‰ and O Baño control site 5.10 ± 0.27‰; Castelo San Felipe 5.73 ± 0.39‰ and Castelo San Felipe control site 5.57 ± 0.15‰).
Discussion
C Stock in Zostera marina Biomass
Considering the area occupied by each one of the four meadows present in the Ría de Ferrol, blue carbon associated with Z. marina in the Ría de Ferrol was 4.25 Mg C, with the highest values found in the O Baño meadow, with 3.56 Mg C, followed by Castelo de San Felipe (0.44 Mg C), O Segaño (0.20 Mg C), and San Felipe Núcleo (0.05 Mg C). Carbon stocks in the O Segaño and San Felipe Núcleo meadows were estimated from the values obtained for the closest meadow: Castelo de San Felipe. The value obtained for biomass-associated carbon, 0.37 Mg C ha−1, was lower than the value observed in the Eastern Atlantic by Röhr et al., 2018 (1.29 Mg C ha−1; Table 4), who recorded C stock data for a wide range of Z. marina meadows across its entire distribution area. Mean density in the Ría de Ferrol was found to be much lower than the expected values for Western Atlantic Zostera marina meadows (774 ± 275 shoots m−2). These values were closer to those established for the Mediterranean Sea, Western Pacific, and the Korean coast (Kim et al., 2022; Table 4), the areas with the lowest density values. Epigeal biomass is much lower than any values described by said authors (Table 4). However, hypogeal biomass values were similar to previously detected, although they were at the lowest end of the range.
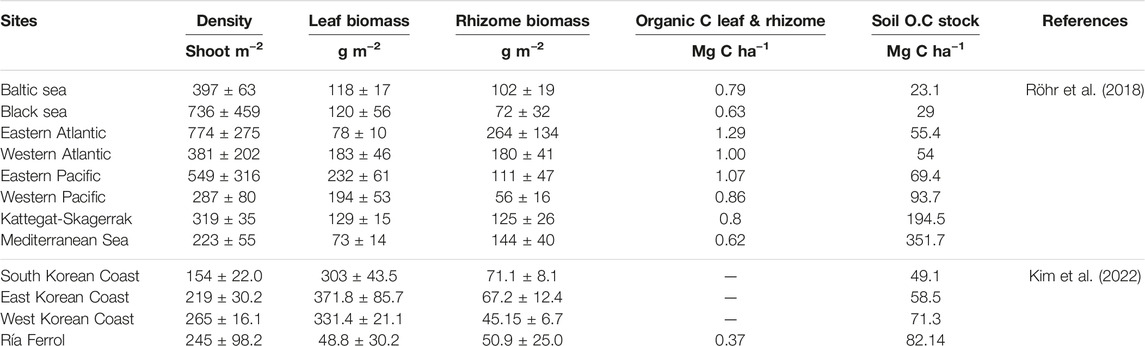
TABLE 4. Biometric parameters and organic C stock in soils from different geographic sites with Zostera marina in relation with meadows of the Ría de Ferrol.
As for leaf category, petiole length, and rhizome length, no detailed studies have been found. Our results allowed inferring that Castelo San Felipe showed more mature leaves (petiole length: 14.3 cm; category 4) than O Baño (petiole length: 9.13 cm; category 3). Moreover, our results showed a high biometric variability between both meadows, consistently with previous studies (Samper-Villarreal et al., 2016; García-Redondo et al., 2019). Said variability is common in the studied species due to its phenotypic plasticity, enhanced by spatial and seasonal variations (Samper-Villarreal et al., 2016; Bertelli et al., 2021), which makes data extrapolation difficult.
In our case, biometric variables have been observed to relate to plant biomass and to its C stock. Leaf length, which did not show any significant differences between the two meadows, appears to be a good predictor for epigeal biomass, as well as for epigeal and total carbon stock (Figure 4). Shoot density was highly correlated with hypogeal and total carbon stock (Figure 4). Therefore, correlations were obtained between biometric variables and carbon content in plants, as observed in other studies (Kennedy et al., 2010; Samper-Villarreal et al., 2016).
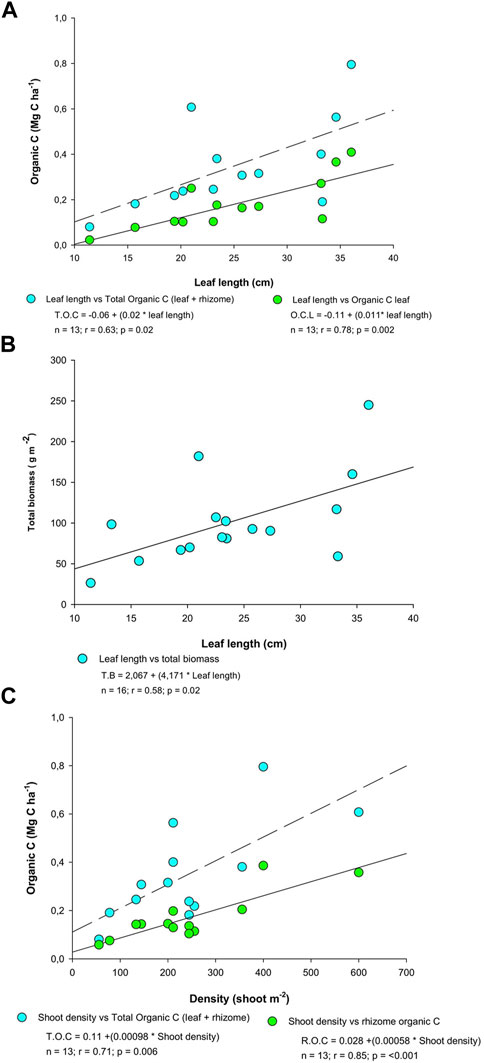
FIGURE 4. Individual relationships between (A) total and leaf carbon content (Mg C ha−1) and leaf length (cm); (B) total biomass (g m−2) and leaf length; and, (C) total and rhizome carbon content and shoot density (m−2).
Conversely, no correlation has been observed between biometric parameters and soil C stock. Kennedy et al. (2010) observed that carbon inputs from seagrass meadows into soils accounts for around 50% of total C. Nevertheless, other authors obtained lower estimates. Samper-Villarreal et al. (2016) observed that the meadow’s carbon contribution to the substrate depended on the environment’s physical characteristics (e.g., water turbidity and depth), ranging from 11.4% to 44.5%, while Röhr et al. (2016) observed that inputs from Z. marina explained 10.9% of variations in the substrate’s carbon stock.
Blue Carbon in Seagrass Meadow Soils
Carbon stock values in soils of Z. marina meadows were 41.7 Mg C in O Baño, 3.36 Mg C in Castelo San Felipe, 1.45 Mg C in Segaño, and 0.39 Mg C in San Felipe Núcleo, which adds up to a total 46.9 Mg C for the whole ria, or 4.11 Mg C ha−1 when expressed per unit of area, taking into account the upper 5 cm of the soil layer. Extrapolating to a soil depth of 100 cm and based on the assumption of homogeneous carbon, thus allowing for result standardization (Lavery et al., 2013; Howard et al., 2014; Röhr et al., 2018; Prentice, et al., 2020; Kim et al., 2022), carbon stock was estimated at 82.14 Mg C ha−1.
Soil carbon stock was higher than expected for Eastern Atlantic meadows, as well as for meadows in the Western Atlantic and in most areas of the world (Table 4). The sum of carbon contained in Z. marina biomass and the carbon stock in soil was 4.48 Mg C ha−1 for the upper 5 cm and 82.5 Mg C ha−1 for the upper 100 cm, which suggests that the plant portion accounted for 8.25% of total C, a value within the ranges described by other authors (Kennedy et al., 2010; Röhr et al., 2016; Samper-Villarreal et al., 2016).
Our results show great differences between carbon stock in soil and in meadows, suggesting that organic carbon in the substrate can have other origins besides the meadows themselves. The origin of organic carbon in soil can be inferred based on different proxies, such as isotope ratios (δ13C and δ15N) or the C/N ratio; based on these ratios, the marine or continental origin of organic matter in soils and sediments can be inferred (for additional details, see García Moya, 2014; Lamb et al., 2006; Kennedy et al., 2010; Röhr et al., 2018; and Samper-Villarreal et al., 2016; Figure 5). According to these ratios, organic carbon in the O Baño meadow could receive continental inputs of organic matter, while in Castelo San Felipe, organic matter seems to have an oceanic origin.
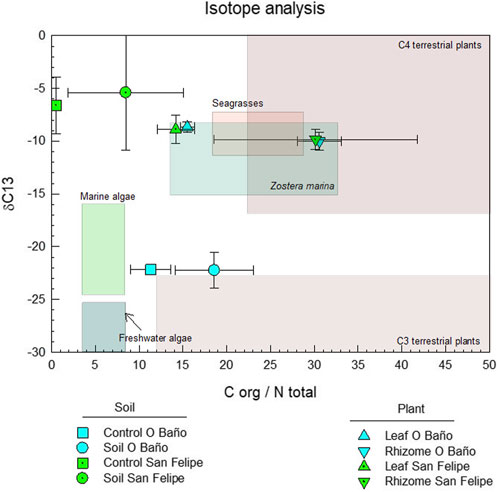
FIGURE 5. Typical δ13C and C/N ranges for organic matter inputs to coastal environments, together with data obtained from leaves, rhizomes, and soil in both meadows and in control sites. Data compiled from: Lamb et al. (2006), García Moya (2014), and Fourqurean, et al. (1997).
In the O Baño site, the low value obtained for δ13C (−22.25‰) and the high C/N ratio (18.58) were similar to those observed for C3 terrestrial plants, while in Castelo San Felipe, the higher values for δ13C (−5.40‰) and the lower C/N ratio (8.49) seem to suggest a marine source of organic matter (Kennedy et al., 2010) (Figure 5). Previous studies have observed that, due to the scarcity of nitrogen and to the fact that the light isotope does not tend to remain, the values of δ15N tend to be higher in sheltered meadows than in exposed ones (Fourqurean et al., 1997; Kennedy et al., 2010; Samper-Villarreal et al., 2016). In our case, the variability observed was minimal, and the correlation was not found, given that values in O Baño (5.3‰) were lower than in Castelo San Felipe (5.7‰).
Finally, it is worth noting that the difference in C/N ratios between O Baño and Castelo San Felipe may also be influenced by their location (Fourqurean et al., 1997). Since O Baño is located towards the innermost area, terrestrial matter tends to be deposited, and its low nitrogen contents (due to the presence of lignin and cellulose) result in high C/N ratios (Fourqurean et al., 1997). The low exposure levels lead to a bottom with a silt-clay granulometry, which promotes a higher degree of recalcitrance, in addition to favoring organic matter stabilization and retention, which could lead to a greater carbon stock. In Castelo San Felipe, the values obtained for the C/N ratio could be influenced by horizontal export processes due to exposure (Lamb et al., 2006; Röhr et al., 2016).
Conclusion
Blue carbon associated with Zostera marina biomass in the Ría de Ferrol (0.37 Mg C ha−1) was much lower than soil carbon stock (4.11 Mg C ha−1) and clearly lower than values observed in meadows of this species. However, soil carbon stock in the Ría de Ferrol for the upper 100 cm (82.1 Mg C ha−1) was higher than the data described by other authors for most meadows worldwide, likely as a consequence of inputs of organic matter of terrestrial origin received at some sites, as isotope ratios and C/N ratios seem to suggest. However, a more detailed characterization of the composition of organic matter (e.g., by NMR or pyrolysis) could help more accurately confirming its origin.
A high spatial variability was observed between both sites in terms of carbon stock in the substrate, which is related to the characteristics of the environment in which the meadows grow (e.g., degree of exposure to marine currents or content of silt).
Finally, the high correlation observed between certain biometric parameters of the plants, such as leaf length, and biomass carbon stock in meadows can constitute a good predictor to estimate the blue carbon globally associated with Z. marina meadows. Further studies in different geographical areas and during different seasons could provide additional data that are essential to develop models for a more accurate estimation of global carbon content in seagrass meadows.
Data Availability Statement
The raw data supporting the conclusion of this article will be made available by the authors, without undue reservation.
Author Contributions
Conceptualization; MdC, XO, and GD-A; methodology: MdC, XO, GD-A, MCR, RRT, AdSE, and MPS; software: MdC and XO; data curation: MdC, XO, GD-A, MCR, RRT, AdSE, and MPS; writing—original draft preparation: MdC, XO, GD-A, MCR, RRT, AdSE, and MPS; supervision: MdC, XO, and GD-A.
Funding
This research was funded by the Consellería de Educación, Universidade e Formación Profesional-Xunta de Galicia (Axudas á consolidación e estruturación de unidades de investigación competitivas do SUG do Plan Galego IDT, Ambiosol Group ref. ED431C 2022/40).
Conflict of Interest
The authors declare that the research was conducted in the absence of any commercial or financial relationships that could be construed as a potential conflict of interest.
Acknowledgments
Thanks are due to María José Santiso for her assistance with laboratory work and to Augusto Pérez Alberti for his contribution to the generation of Figure 1.
Abbreviations
SBD, soil bulk density; SCD, soil carbon density; SCS, soil carbon stock.
References
Bertelli, C. M., Bull, J. C., Cullen-Unsworth, L. C., and Unsworth, R. K. (2021). Unravelling the Spatial and Temporal Plasticity of Eelgrass Meadows. Front. Plant Sci. 12, 664523. doi:10.3389/fpls.2021.664523
Cacabelos, E., Quintas, P., Troncoso, J., Bárbara, I., García-Redondo, V., Cremades, J., et al. (2015). “Las praderas marinas de España: una visión general. Cuadro temático 1. La biodiversidad de las praderas españolas, Atlántico norte,” in Atlas de las praderas marinas de España (IEO/IEL/UICN, Murcia-Alicante-Málaga), 87–91.
Claes, J., Hopman, D., Jaeger, G., and Rogers, M. (2022). Blue Carbon: The Potential of Coastal and Oceanic Climate Action. Hong Kong, China: McKinsey & Company.
Duarte, C. M., and Chiscano, C. L. (1999). Seagrass Biomass and Production: a Reassessment. Aquat. Bot. 65, 159–174. doi:10.1016/S0304-3770(99)00038-8
Duarte, C. M., Sintes, T., and Marbà, N. (2013). Assessing the CO 2 Capture Potential of Seagrass Restoration Projects. J. Appl. Ecol. 50 (6), 1341–1349. doi:10.1111/1365-2664.12155
Fourqurean, J. W., Duarte, C. M., Kennedy, H., Marbà, N., Holmer, M., Mateo, M. A., et al. (2012). Seagrass Ecosystems as a Globally Significant Carbon Stock. Nat. Geosci. 5 (7), 505–509. doi:10.1038/ngeo1477
Fourqurean, J. W., Moore, T. O., Fry, B., and Hollibaugh, J. T. (1997). Spatial and Temporal Variation in C: N: P Ratios, δ15N, and δ13C of Eelgrass Zostera Marina as Indicators of Ecosystem Processes, Tomales Bay, California, USA. Mar. Ecol. Prog. Ser. 157, 147–157. doi:10.3354/meps157147
García Moya, A. (2014). Estudio de origen y variación temporal de la materia óraganica en los sedimentos de Refugio de Fauna" Laguna Guanaroca", Cienfuegos, Cuba. Doctoral dissertation (Las Villas: Universidad Central" Marta Abreu").
García-Redondo, V., Bárbara, I., and Díaz-Tapia, P. (2019). Zostera Marina Meadows in the Northwestern Spain: Distribution, Characteristics and Anthropogenic Pressures. Biodivers. conservation 28 (7), 1743–1757. doi:10.1007/s10531-019-01753-4
Herr, D., and Landis, E. (2016). “Coastal Blue Carbon Ecosystems,” in Opportunities for Nationally Determined Contributions. Policy Brief (Gland, Switzerland: IUCN).
Howard, J., Hoyt, S., Isensee, K., Pidgeon, E., and Telszewski, M. (2014). “Coastal Blue Carbon Methods for Assessing Carbon Stocks and Emissions Factors in Mangroves, Tidal Salt Marshes, and Seagrasses Meadows,” in Conservation International, Intergovernmental Oceanographic Commission of UNESCO, International Union for Conservation of Nature (Arlington, Virginia, USA: UNESCO).
IPCC (2021). The Physical Science Basis. Contribution of Working. Group I to the Fifth Assessment Report of the Intergovernmental Panel on Climate. Change. Cambridge, United Kingdom and New York, NY, USA: Cambridge University Press.
Kennedy, H., Beggins, J., Duarte, C. M., Fourqurean, J. W., Holmer, M., Marbà, N., et al. (2010). Seagrass Sediments as a Global Carbon Sink: Isotopic Constraints. Glob. Biogeochem. cycles 24 (4). doi:10.1029/2010gb003848
Kim, S. H., Suonan, Z., Qin, L. Z., Kim, H., Park, J. I., Kim, Y. K., et al. (2022). Variability in Blue Carbon Storage Related to Biogeochemical Factors in Seagrass Meadows off the Coast of the Korean Peninsula. Sci. Total Environ. 813, 152680. doi:10.1016/j.scitotenv.2021.152680
Kuo, J., and Den Hartog, C. (2001). “Seagrass Taxonomy and Identification Key,” in Global Seagrass Research Methods. Editors F. T. Short, and R. G. Coles (Amsterdam: Elsevier Science BV), 33, 31–58. doi:10.1016/B978-044450891-1/50003-7
Lamb, A. L., Wilson, G. P., and Leng, M. J. (2006). A Review of Coastal Palaeoclimate and Relative Sea-Level Reconstructions Using δ13C and C/N Ratios in Organic Material. Earth-Science Rev. 75 (1-4), 29–57. doi:10.1016/j.earscirev.2005.10.003
Lavery, P. S., Mateo, M. Á., Serrano, O., and Rozaimi, M. (2013). Variability in the Carbon Storage of Seagrass Habitats and its Implications for Global Estimates of Blue Carbon Ecosystem Service. PloS one 8 (9), e73748. doi:10.1371/journal.pone.0073748
Mcleod, E., Chmura, G. L., Bouillon, S., Salm, R., Björk, M., Duarte, C. M., et al. (2011). A Blueprint for Blue Carbon: toward an Improved Understanding of the Role of Vegetated Coastal Habitats in Sequestering CO2. Front. Ecol. Environ. 9 (10), 552–560. doi:10.1890/110004
Míguez, B. M. (2003). Descripción dinámica de la circulación en dos Rías Baixas: Vigo y Pontevedra. Doctoral dissertation, PhD Thesis (Vigo: University of Vigo).
NOAA (2021). EarthDay: Invest in Our Changing Planet Now to Secure a More Livable Future. Available from: https://gml.noaa.gov/ccgg/trends/weekly.html.
Pendleton, L., Donato, D. C., Murray, B. C., Crooks, S., Jenkins, W. A., Sifleet, S., et al. (2012). Estimating Global “Blue Carbon” Emissions from Conversion and Degradation of Vegetated Coastal Ecosystems. PLoS One 7 (9), e43542. doi:10.1371/journal.pone.0043542
Prentice, C., Poppe, K. L., Lutz, M., Murray, E., Stephens, T. A., Spooner, A., et al. (2020). A Synthesis of Blue Carbon Stocks, Sources, and Accumulation Rates in Eelgrass (Zostera Marina) Meadows in the Northeast Pacific. Glob. Biogeochem. Cycles 34 (2), e2019GB006345. doi:10.1029/2019gb006345
Röhr, M. E., Boström, C., Canal-Vergés, P., and Holmer, M. (2016). Blue Carbon Stocks in Baltic Sea Eelgrass (Zostera marina) Meadows. Biogeosciences 13 (22), 6139–6153. doi:10.5194/bg-13-6139-2016
Röhr, M. E., Holmer, M., Baum, J. K., Björk, M., Boyer, K., Chin, D., et al. (2018). Blue Carbon Storage Capacity of Temperate Eelgrass (Zostera Marina) Meadows. Glob. Biogeochem. Cycles 32 (10), 1457–1475. doi:10.1029/2018gb005941
Samper-Villarreal, J., Lovelock, C. E., Saunders, M. I., Roelfsema, C., and Mumby, P. J. (2016). Organic Carbon in Seagrass Sediments Is Influenced by Seagrass Canopy Complexity, Turbidity, Wave Height, and Water Depth. Limnol. Oceanogr. 61 (3), 938–952. doi:10.1002/lno.10262
Keywords: seagrass, biometric parameters, isotope ratios, carbon, nitrogen
Citation: de la Cerda-Marín MdC, Díaz-Agras G, Rodríguez Tato R, Candás Romero M, Pérez Señarís M, de Solaun Eimil A and Otero XL (2023) Blue Carbon Stock in Zostera marina Meadows in the Ría de Ferrol (NW Iberian Peninsula). Span. J. Soil Sci. 13:11352. doi: 10.3389/sjss.2023.11352
Received: 10 March 2023; Accepted: 17 April 2023;
Published: 10 May 2023.
Edited by:
Avelino Núñez-Delgado, University of Santiago de Compostela, SpainCopyright © 2023 de la Cerda-Marín, Díaz-Agras, Rodríguez Tato, Candás Romero, Pérez Señarís, de Solaun Eimil, Otero. This is an open-access article distributed under the terms of the Creative Commons Attribution License (CC BY). The use, distribution or reproduction in other forums is permitted, provided the original author(s) and the copyright owner(s) are credited and that the original publication in this journal is cited, in accordance with accepted academic practice. No use, distribution or reproduction is permitted which does not comply with these terms.
*Correspondence: Xosé Luis Otero, eGwub3Rlcm9AdXNjLmVz