- 1Soil Science and Environmental Technologies Group (GETECMA), Department of Agrochemistry and Environment, Miguel Hernández University, Elche, Spain
- 2Graduate Program in Integrative Ecology, Natural Resources Institute (INIRENA), Universidad Michoacana de San Nicolás de Hidalgo, Morelia, Mexico
- 3Earth Science Department, Natural Resources Institute (INIRENA), Universidad Michoacana de San Nicolás de Hidalgo, Morelia, Mexico
The forest floor is a critical component in maintaining the life cycles of forest ecosystems. It normally includes organic soil horizons, known as duff and litter, which are prone to be rapidly consumed after flaming and smoldering fires. This work aims to understand the legacy effects of surface and ground fires on the infiltration capacity of a volcanic forest soil. We studied five sites with fires recorded in the last 20 years. All of them are located in pine-oak forests of the volcanic mountain region in central Mexico with a temperate climate and Andic soil properties. Tension-infiltration tests were carried out to determine hydraulic conductivity and the number of active macropores. After each test, cores were taken to evaluate in a laboratory setting, where soil water repellency at different moisture concentrations and the integrative dynamic repellency index were determined. Field-saturated hydraulic conductivity was moderately high in all sites, with mean values of 13 and 42 mm·h−1 for burned and control plots, respectively. A non-linear relationship was found between recurrence and type of fires with the concentration of active pores and several dynamic water repellency parameters. This work confirmed the presence of latent combustion in these temperate neotropical forests. The changes in soil water repellency and hydraulic conductivity detected do not necessarily imply an exceeded soil infiltration capacity. However, many of the fires in this region are associated with increasing agricultural activities, so further studies are needed to determine if higher fire frequencies could exceed the resilience capacity of the soils triggering land degradation.
Introduction
Tropical mountain ecosystems are characterized by significant biological and lithological diversity, which plays a critical role in maintaining ecosystem services and supporting a variety of life forms (Bruijnzeel et al., 2011; Poorter et al., 2015). Among the various components of these ecosystems, the forest floor plays a critical role in maintaining the life cycles of forest ecosystems (Descroix et al., 2001; Keith et al., 2010; Neris et al., 2013). This typically includes organic soil horizons, known as duff (Oe and Oa horizons) and litter (Oi horizons), and in some cases of abundant moisture, even peat (Ha, He, and Hi horizons). Most meso- and microfauna and ectomycorrhizal fungi concentrate their life cycles in these first centimeters of forest soils (Prescott and Vesterdal, 2021; Peng et al., 2022).
Surface fires, which spread at a high speed, rapidly consume the understory without significantly changing the soil properties (Agee, 1993; Keeley, 2009). However, when the forest floor includes thick layers of peat, duff, or litter, slow smoldering combustion can take place after flame combustion, which can generate profound soil changes (Ogle and Schumacher, 1998; Rein, 2015; Santoso et al., 2022). These effects have been studied in tropical, boreal, and sub-boreal peatland ecosystems; however, there is a gap in this knowledge in neotropical mountain ecosystems (Santoso et al., 2019).
Different time intervals between fires could affect tree-seed sprouting (Busby et al., 2020) and, thus, the forest’s community composition and ecological services (Miller et al., 2011). However, the effects on soil properties are still an emerging field where non-linear relationships have been observed (Moghli et al., 2022).
Moreover, while it is well established that low soil water content in fire-affected water-repellent soils can exacerbate the risk of erosion (Doerr et al., 2000; Shakesby and Doerr, 2006; Bento-Gonçalves et al., 2012), the emergence and severity of water-repellency do not occur in a linear progression as it dries. Rather, it occurs as a complex phenomenon where slightly moist soils can be even more repellent than completely dry soils (Wallis and Horne, 1992; Majid et al., 2023). Parameters such as IRDI (Integrative Repellency Dynamic Index), gravimetric water content at maximum water repellency levels (w.max), or gravimetric water content where the water repellency starts to be negligible (w.min) are alternatives to capture this dynamism (Regalado and Ritter, 2006; Regalado and Ritter, 2008).
In this sense, this work arises as a response to understanding the legacy effects of surface and ground fires on the infiltration capacity of mountain forest soils and to evaluate the degree of resistance of the Andosols to up to two recurring fires. We will also validate the presence of smoldering fire in two plots with duff in their forest floor. The central hypothesis of this research is that there will be a negative effect of the fire recurrence on soil hydraulic properties with a positive correlation of the number of fires with soil water repellency, and a negative relationship with hydraulic conductivity.
Materials and Methods
Study Sites and Soil Description
We studied five sites, one of them an unburned control, and four affected by different fires between 2000 and 2018; showing blackening of the trunks up to a height of 1.5 m (Rodríguez Rodríguez, 2014; Olivares-Martínez, 2020). Their land tenure was collective (ejido) and private (Table 1 and Figure 1), so all landowners of all sites were contacted to obtain their informed consent before fieldwork.
Due to the serious insecurity caused by drug cartels in the region, ensuring a minimum security condition necessary to carry out the fieldwork was also an important criterion. This limited the final number of sites and samples studied, in order to ensure the safety and physical integrity of the staff who worked in the field.
All the sites are within the physiographic province called Neovolcanic Axis with a semi-humid temperate climate, with annual average temperatures of 12°C–18°C and annual rainfall between 520 and 1,000 mm concentrated almost entirely during the summertime (INEGI, 1985a, 1985b, 2013). It corresponds with the Köppen climate classification Cwb, and with the modified Köppen climate classification C(m)(w) and C(w2)(w) (García, 2004; Peel et al., 2007).
The vegetation is composed by pine-oak forest over volcanic soils with slopes between 15° and 35° (INEGI, 2017; Olivares-Martinez et al., 2023). The forest has dominance of Pinus pseudostrobus and co-dominance of Quercus rugosa, Quercus laurina, and Quercus crassipes (Olivares-Martínez, 2020). Tree densities are between 170 and 620 trees per hectare, canopies of more than 80%, and in these sites there are some forestry activities like resinating pine trees or collecting firewood without logging.
The burned sites were affected by small fires of less than 5 ha and the burned trees had flame scars up to 2 m. In two plots, in addition to the evidence of flames, there were charcoal residues between the deeper duff and down to 10 cm in the mineral soil, which suggested the presence of latent combustion (Olivares-Martínez, 2020). They were assigned codes according to their fire history status: capital letters to express if they were Surface (S), Ground (G) or Control (C) plots, and number for the number of fires in the 18 years period.
The burned soils have loamy textures with clay percentages between 9% and 20% and very high organic matter contents (Olivares-Martínez, 2020); the first 5 cm of the mineral soil have soil organic matter levels between 7% and 19.5%. The sites affected by ground fires had duff forest floors up to 12 cm in depth, with assumed soil organic carbon levels of more than 20%, by definition (IUSS Working Group WRB, 2022). On the other hand, the reference control plot has a silty loam texture, with 6% of clay, 7% of organic matter, thick organic layers of duff, and litter of 14 cm in depth (Rodríguez Rodríguez, 2014). All the soils have Andic properties and were classified as Silandic Andosols (IUSS Working Group WRB, 2022). At each site, the type of mulch was evaluated according to its thickness and mineralization/humification processes (Siebe et al., 1996), a soil pit was dug, and the genetic horizons were described (FAO, 2009).
Infiltration Tests
INDI tension infiltrometers were used to perform 15 to 20 infiltration tests per site to ensure adequate representativeness according to its log normal statistical distribution [Gómez-Tagle (Jr.) Ch. et al., 2011]. The tests were conducted on the forest floor, which comprised mainly mineral A horizons, and in some cases organic Oa horizons after removing the litter. The INDI is a low-cost self-made device to characterize the saturated & unsaturated field hydraulic conductivity and estimate the effective soil porosity (Eqs A1–A3) (Watson and Luxmoore, 1986; Gómez-Tagle Chávez et al., 2014). The INDI tension infiltrometer is inspired by designs from Špongrová et al. (2009) and Perroux and White (1988). Further details can be reviewed by the reader in Gómez-Tagle et al. (2011).
We applied −9.0, −3.0, and −0.5 cm tensions. We considered geometric corrections and the non-linear numerical approximation from Wooding’s and Gardner’s equations (Eqs A1, A2) to know the saturated and unsaturated hydraulic conductivity of the field (Logsdon and Jaynes, 1993). The number of active pores and the volumetric percentage were estimated from the unsaturated infiltration curves and the Jurin Law (Eqs A3, A4) (Watson and Luxmoore, 1986; Gómez-Tagle Chávez et al., 2014). We assumed the dynamic water viscosity as 1.002E-03 kg·m−1·s−1 (standard at 20°C), the water density as 998.2 kg·m3 (standard at 20°C), and the gravity acceleration as 9.81 m·s−2, and a macropore size of more than 0.5 mm (according to Luxmoore, 1981).
To ensure the complete contact of the base of the infiltrometer and the soil surface, we used a fine sandy marble contact bed (90% sand, 8% silt, and 2% clay; “Marmolina comercial Tipo I, Fina”) (Gómez-Tagle Chávez et al., 2014; Rodríguez Rodríguez, 2014). In the case of steep slopes (more than 35%), we made small terraces to level the infiltrometer, ensuring the measurement occurred within the target soil depth. This is a reliable method with no risk of losses by lateral flow when there are no abrupt textural changes (Miyazaki, 1988).
Soil Sampling and Lab Analysis
A core of about 1 cm in thickness and 5 cm in diameter was taken inside the area where each infiltration test was performed. The cores were carefully put in Petri dishes to measure water repellency at different moisture levels, according to the Water Drop Penetration Time test and the Molarity of an Ethanol Droplet (Woudt, 1959; Letey et al., 2000). In each case, the integrative dynamic repellency index (IRDI), maximum water repellency angle (a.max or αmax), water repellency angle after drying the samples at 105°C (a.105 or α105°C), the trapezoid integral area below the α(θg) curve (S), and gravimetric water content where the water repellency starts to be neglectable (w.min or θg.min), were calculated (Regalado and Ritter, 2005).
Equation Solving and Statistical Analysis
R v4.2.2 statistical software was used to solve the Wooding & Gardner non-linear equations and to perform statistical tests (R Core Team, 2022). One-way ANOVAs were done to assess the effects of type of fire and recurrence onto the hydrophysical soil variables comparing with control plot. Two-way ANOVAs were carried out, excluding the control plot to explore the effect of the interaction between the factors of type (surface, and ground fire), and recurrence (one, or two fires inside the period). Log-normal adjustments to compare maximum likelihood estimators were performed to the four hydraulic conductivity-derived variables, the water repellency angle after drying the samples at 105°C, and the maximum water repellency angle. A post hoc honestly-significant-difference test by means of Tukey (HSD) was done in the significant models.
Results
Morphology of the Burned Volcanic Soils
The four burned sites showed fire scars with charcoal residues in the bark of their trees and soil water repellency. The volumetric field water contents of the sites, when sampled, were from 50% to 102%. The main burned trees were pines, but in the G(1) plot, scars were also found on some oaks.
The S(1) and S(2) plots presented a Mull humus (duff). On the other hand, the G(1) and G(2) plots had a Mor humus (duff), and there was evidence of smoldering combustion in both. There were visible charcoal residues found to a depth of more than 10 cm below the surface. A system of galleries and macropores with the rest of the root charcoal down to more than 50 cm was observed on the G(2) plot (Figure 2).
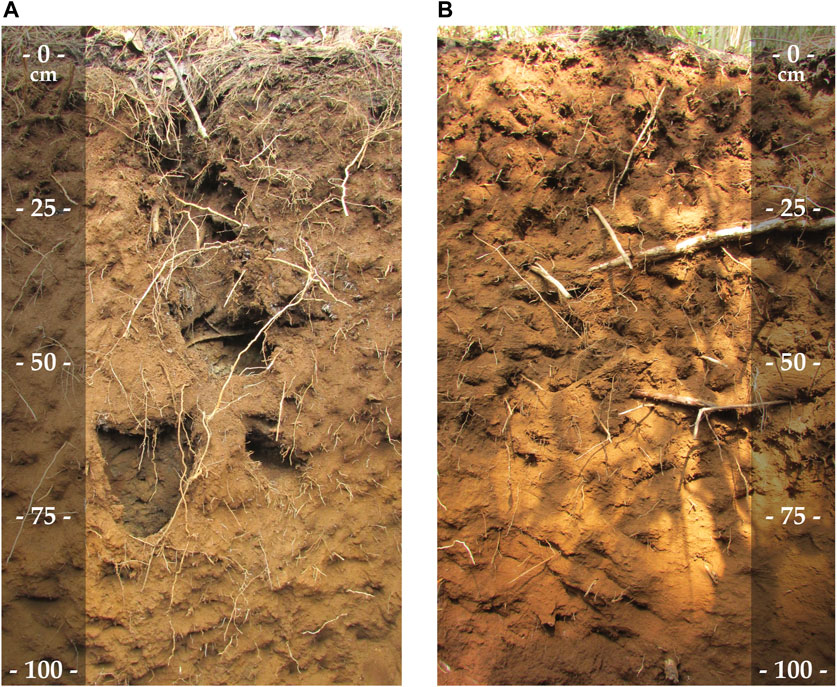
FIGURE 2. Morphology comparison between plots with strong (B) and insignificant (A) presence of smoldering combustion. Note the galleries with root charcoal until 80 cm.
Hydro-Physical Effects From Ground and Surface Fire
A total of 85 water infiltration tests were performed. Nine tests failed due to high water repellency levels on the field, so only 76 tests were completed. The field-saturated hydraulic conductivity was from moderately high to high in all sites, the control plot had 42 mm per hour, much higher compared to the burned plots, with a mean hydraulic conductivity of 12.88 mm per hour (Table 2).
The water flux via macropores decreased after fires, like the field-saturated hydraulic conductivity. Burned soils could have a macropore flux 20 times less than the reference soil. Nevertheless, the number of active pores (NM·m-²) had contrasting scenarios across the different types and fire recurrencies (Tables 2, 3).
A total of 63 water repellency tests were performed throughout different levels of soil moisture content. Almost all the samples showed water repellency to some extent; only tree samples in G(2) plot were completely hydrophilic. The mean a. max throughout the samples was 100.7° with no significant differences when type and recurrence of the fire were considered (p < 0.05, d. f. = 1, F = 5.53), significant differences could be found only considering a one-way ANOVA model (p > 0.001, d. f. = 4, F = 6.55). The rest of the water-repellency parameters showed statistically significant differences (Figure 3).
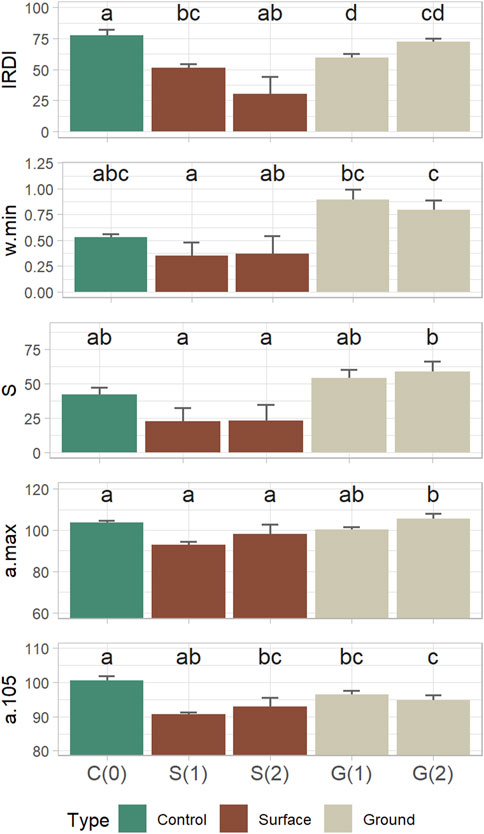
FIGURE 3. Water repellency across different fire histories. IRDI as a dimensionless index, w.min as volumetric water content, a.105 and a.max as the estimated contact angles. The bars represent standard errors, and the letters indicate the HSD statistical groups. Codes in the horizontal axis according to Table 1.
The control site showed a higher a.105 dry-soil water repellency than the burned plots: 100.6° against a range of 90.7°–96.5°, with only one significant difference between C(0) and S(1). The a.max, and the S parameters showed similar patterns. The w.min showed significant differences after the first fire, according to the type of fire but not when compared to the control plot (Figure 3). On the other hand, the IRDI showed significant differences in the control plot, specifically after a second surface fire. However, a second ground fire reestablished the IRDI to reference values.
The hydraulic soil properties and the active porosity showed a consistent and significant relationship to the type and the cumulative number of fires (Table 3). On the other hand, the soil water repellency dynamic parameters showed differences mainly according to the type of fire, and just the integrated index IRDI showed a significant relationship with fire type and recurrence (p > 0.01, d.f. = 1, and F = 11.06).
After two fires, the water repellency could have contrasting dynamics depending on the type of fire and the type of forest floor. For example, the duff-covered G(2) soil showed a similar total water repellency to the control plot with the IRDI index but with higher maximums at a. max and even a lower dry-soil water repellency at a.105 values; on the other hand, the S(2) showed a decrease in the IRDI, similar values to the control plot on w. min and a. max, but a lower a.105 (Figure 3).
Discussion
Fire Legacies on Soil Memory
The presence of residues of organic duff layers (Oa and Oe genetic horizons) were found only on ground-fire-affected plots. When the soil has high organic matter (nearly 20%), it is highly likely to develop smoldering combustion after a surface fire (Rein, 2015). It is possible that the increased number of galleries and macropores on the G(2) plot after a first fire may have enhanced the oxygen availability so that a second fire could burn deeper layers and leave deep charcoal residues such as those found in the field.
Of all the components of a fire regime, the rate of spread of fire (and therefore the time of residence of the heat) is the one that can have the greatest effect on the soil (Agee, 1993; Keeley, 2009). In this sense, the two sites with clear evidence of smoldering combustion presented higher hydrophobicity at deeper layers (Olivares-Martínez, 2020). These plots had scars on pines and even on oaks (Quercus spp.), whose bark is less prone to be burned unless there are lower fire spread rates. The longer the time of residence, the deeper and greater the fire severity in the soil (Neary et al., 1999; Varner et al., 2005; Rein, 2015).
Smoldering fires can increase soil temperatures by more than 60°C down to 20 cm on soil mineral horizons (Varner et al., 2005) and more than 50 cm on peat layers (Wösten et al., 2006). So, it is logical that a ground fire would show lower standard errors than a surface fire in its water-repellency-related properties since the former has more homogeneous fires. The G(2) plot could have presented deep charcoal residues and big galleries due to the smoldering of whole trees' root systems adding to the organic soil smoldering combustion.
Organic layers can start to smolder even with gravimetric water contents up to 120% (Neary et al., 1999). When sampled, the field water contents of the ground-fire-burned sites were from 79% to 102%, so they could have burned at that time even when they seemed wet. Soil humidity is a factor that regulates both ease of burning and soil water repellency and water infiltration rates (Jordán et al., 2013; Mataix-Solera et al., 2014; Marín-Castro et al., 2016). It suggests that the humus type of the forest floor drives the water thresholds on smoldering to fire dynamics and the charcoal imprinted legacies.
To understand better why the control plot and G(2) plot presented the higher IRDI values is necessary to understand how the index is structured. The index is constructed with the S and the w.min variables to describe the dynamic of water repellency according to soil humidity, so its changes can be due to the size of the S parameter, which tells us about the total area under the water repellency curve, and in an inversely proportional way, the index changes with the w. min parameter (Regalado and Ritter, 2005).
Moreover, other indirect effects on the IRDI could be explained by the size of the differences between a.max and a.105 (difference known as αerr) (Regalado and Ritter, 2005). Although a. max did not show great differences between sites except for the Sup(1) plot, a.105 was sensitive to the number and type of fire. It is possible that the presence of resins and other tree exudates could increase the dry water repellency at the control plot even more than the burned plots (Rodríguez Rodríguez, 2014).
The humus type, porosity, and water repellency were noticeable components of the soil memory after different fire histories in addition to other traits such as recalcitrant organic matter (Jordán et al., 2013; Mataix-Solera et al., 2014), aggregation (Lal, 1997; Mataix-Solera et al., 2011) and ashes, which in turn alter the electrical conductivity, nutrient availability, and texture of the soil (Bodí et al., 2012; Francos et al., 2018; Olivares-Martínez, 2020).
Dynamic Water Repellency Remarks
The IRDI index was proposed as an attempt to understand better the non-linear dynamic of water repellency, and it should be interpreted as a shape index more than a total repellency index (e.g., S parameter), which tells where there are major differences between the hydrophobicity of dry and moist soil in narrow moisture gradients (Regalado and Ritter, 2005). A high IRDI value does not necessarily imply a stronger water repellency, it tells us about the relationship between the total water repellency and how easy it disappears with humidity.
Although no significant differences were seen in S and w.min, when combined in the IRDI it was possible to find significant differences generated by both recurrence and type of fire (Figure 3). The soils have imprinted different shapes in the repellency curve according to their fire history, so we can understand the IRDI as a “repellency signature.” Moreover, although the plot two ground fires had increases in the persistence of soil water repellency, it reestablishes the form of this relationship, so the IRDI is very similar to the control plot. It would be interesting to further expand on this pattern by comparing with more sites that have a similar fire history.
It could be confusing when trying to consider the IRDI in hydrologic terms; the most prone sites to have problems with water infiltration and erosion will be those with the greatest water repellency area (S), a combination between high maximum water repellencies (a.max) and high minimum humidities (w.min) with low standard deviations. Water repellency is a patchy-distributed spatial variable (Woods et al., 2007), so greater standard errors such as those found at surface fires (bigger error bars at S and w.min on Figure 3) suggest a strong relationship with Hortonian water fluxes to a more hydrophilic matrix. Surface fires tend to have higher spread rates, so heat transmissions to soil are more scattered, and, consequently, the soil changes (Agee, 1993; Mataix-Solera et al., 2007).
Although without noticeable effects on the number of active macropores on these volcanic soils, the amount of water flowing through them was affected by the type and number of fires. These reductions may be linked to the persistence of water repellency without significant changes in organic matter content (Olivares-Martínez, 2020). The w.min, as a measure of the persistence of hydrophobicity in humid conditions, is important to show that even after the first rains, soil can still have water repellency. That could be important when modeling surface water runoff and soil erosion processes (Cerdà and Robichaud, 2009).
Resilience and Degradation
Although only the C(0) and the G(2) plots were above the global hydraulic conductivity average according to their textural class (Gupta et al., 2020), it could be common that Andosols have low hydraulic conductivities, especially when water repellency is present (Farahnak et al., 2019). Neris et al. (2013) have shown that Andosols beneath pine vegetation cover tend to have lower infiltration capacity due to the water repellency associated with the type of duff of its forest floor. This makes them more susceptible to erosion by runoff, despite the high resistance to degradation that these soils may have.
Average rainfall in the region can be up to 9 mm daily during the rainy season (CNA and SEMARNAT, 2020). If we assume that convective precipitation events could concentrate the day’s rainfall in 1 h, all the rainwater can be infiltrated with the cumulative effect of one or two surface fires. The assumed runoff exceeded the water infiltration capacity only in the G(1) plot, which has a mean hydraulic conductivity of 6.5 mm per hour, the rest of the burnt plots can buffer this mean rainfall (Table 2). So, this could indicate that even with a reduced infiltration and enhanced water repellency, one or even two fires in 18 years have no serious repercussions for surface runoff and water erosion. In any case, further studies are necessary to confirm this result.
Despite the infiltration increase after a second ground fire, it should be considered that a higher standard deviation (patchiness), coupled with a low percentage of macropore flow and a higher repellency, could imply preferential finger flows (Ruthrof et al., 2019). This type of water movement may lead to higher risks of nutrient and contaminant leaching at recursive soil points (Ritsema and Dekker, 1994; Debano, 2000) but higher water infiltration potential at the landscape level (Doerr et al., 2000). Therefore, there may be an increase in the ecological function of water supply but not in water purification.
In addition to preferential flow pathways, humidity’s non-linear effect on the soil hydraulic conductivity becomes negligible only when soil water content surpasses a certain threshold (Regalado and Ritter, 2005). Nevertheless, it is essential to note that a high soil water content is not always a safeguard against water repellency since certain soil types, including Andosols, can retain their hydrophobic properties even when moistened (Wallis and Horne, 1992; Regalado and Ritter, 2008; Jordán et al., 2013).
Landscape Concerns
As shown in the previous sections, sites with sporadic fires in these temperate neotropical forests of the avocado belt will not face major degradation problems, especially on surface fires, where scattered water repellency does not imply infiltration reductions at landscape levels (Doerr et al., 2000). Moreover, water repellency could enhance water storage in the soil by diminishing direct soil evaporation rates (Ruthrof et al., 2019). So, we suggest that inherited water repellency in the soil can be part of the dynamics of a healthy forest ecosystem when fire regimes do not exceed resilience thresholds on the soil.
Water repellency on soils is a common phenomenon that could be found even on reference sites (Weninger et al., 2019). The reference forest of this work could have water repellency even during the rainy seasons (Rodríguez Rodríguez, 2014). Activities like resining pine trees could increase the water repellency of the soils, decreasing their water infiltration potential; so recurrent low-severity fires could ameliorate this situation when they increase the patchiness of the soil water repellency distribution as long as they do not exceed land degradation thresholds.
However, sporadic fires tend to be an exception more than a rule, many of the fires in the forests of this region are associated with agricultural activities that are rapidly increasing their surface area (Barsimantov and Navia Antezana, 2012; Tejera Hernández et al., 2013; Olivares-Martinez et al., 2023). In this sense, it is pertinent to study whether areas with a higher fire frequency, enhanced by activities such as the international avocado trade, could exceed the soil’s resilience, and trigger degrading processes.
Conclusion
In this work, we ascertained the presence of latent combustion in two temperate neotropical forests. The infiltration capacity of the Andosols showed a high decrease after ground or surface low-severity fires with high resistance to change in periods of up to 20 years. The IRDI soil water repellency parameter was sensible for fire histories with up to two recurrent fires. Parameters such as the percentage of charcoal, pore space structure, and water repellency were useful indicators for understanding the fire history of these soils.
Parameters such as moisture at minimum hydrophobicity w. min and area S under the hydrophobicity curve were found to be edaphic memory traits related to fire history in the Andosols of subtropical regions. Although reduced, the infiltration capacity of S(1), S(2), and G(2) plots is not diminished to such an extent that surface runoff is generated in an average rainfall.
Data Availability Statement
The raw data supporting the conclusion of this article can be found at: https://github.com/OlivaresLD/fire-effects-on-soils.
Author Contributions
LO-M performed the statistical tests, developed figures and tables, sampled the soils, and wrote the first versions of the manuscript. AG-T performed resource and logistical management, soil sample processing, and laboratory analysis. JM-S reviewed and contributed to the writing process, and collaborated on the financing of the manuscript. All authors contributed to the article and approved the submitted version.
Funding
This research work received funding from CONACyT for the first author through his master’s grant (CVU 588502). Overall project funding was provided by the projects: CONACyT Project CONACyT/PDCPN2016/Proyecto3053 “Impactos y consecuencias del desarrollo de la franja aguacatera sobre aspectos hidrológicos, funcionales, genéticos y de biodiversidad en ecosistemas templados de México” (Impacts and consequences of avocado growing-belt development on hydrological, functional, genetic and biodiversity aspects in temperate ecosystems of Mexico), ICTI-Project PFCTI/ICTI/2019/A/315 “Aplicación del conocimiento ecológico para favorecer la sustentabilidad del cultivo de aguacate en el estado de Michoacán: Aspectos de suelo, hidrológicos e interacciones bióticas” (Application of ecological knowledge to promote the sustainability of avocado crops in the state of Michoacán: Soil, hydrological aspects and biological interactions).
Conflict of Interest
The authors declare that the research was conducted in the absence of any commercial or financial relationships that could be construed as a potential conflict of interest.
Acknowledgments
We thank the AG-T’s lab members: Mariela Casillas Corona, Alondra Alvarado Domínguez, Edgar Cruz-Cruz, Javier Rodríguez-Rodríguez, and Esbeydy Marquez Loeza for their help during the lab tests.
References
Barsimantov, J., and Navia Antezana, J. (2012). Forest Cover Change and Land Tenure Change in Mexico’s Avocado Region: Is Community Forestry Related to Reduced Deforestation for High Value Crops. Appl. Geogr. 32, 844–853. doi:10.1016/j.apgeog.2011.09.001
Bento-Gonçalves, A., Vieira, A., Úbeda, X., and Martin, D. (2012). Fire and Soils: Key Concepts and Recent Advances. Geoderma 191, 3–13. doi:10.1016/j.geoderma.2012.01.004
Bodí, M. B., Doerr, S. H., Cerdà, A., and Mataix-Solera, J. (2012). Hydrological Effects of a Layer of Vegetation Ash on Underlying Wettable and Water Repellent Soil. Geoderma 191, 14–23. doi:10.1016/j.geoderma.2012.01.006
Bruijnzeel, L. A., Kappelle, M., Mulligan, M., and Scatena, F. N. (2011). “Tropical Montane Cloud Forests: State of Knowledge and Sustainability Perspectives in a Changing World,” in Part VII - Cloud Forest Conservation, Restoration, and Management Issues. Editors L. A. Bruijnzeel, F. N. Scatena, and L. S. Hamilton (Cambridge University Press), 691–740. doi:10.1017/CBO9780511778384.074
Busby, S. U., Moffett, K. B., and Holz, A. (2020). High-Severity and Short-Interval Wildfires Limit Forest Recovery in the Central Cascade Range. Ecosphere 11, 22. doi:10.1002/ecs2.3247
Cerdà, A., and Robichaud, P. (2009). Fire Effects on Soils and Restoration Strategies. Taylor & Francis. Available at: http://www.crcnetbase.com/doi/book/10.1201/9781439843338.
CNA and SEMARNAT (2020). Estación Zirahuén. México. Available at: https://smn.conagua.gob.mx/tools/RESOURCES/Estadistica/16146.pdf (Accessed June 28, 2023).
Debano, L. F. (2000). Water Repellency in Soils: A Historical Overview. J. Hydrol. (Amst) 231–232, 4–32. doi:10.1016/S0022-1694(00)00180-3
Descroix, L., Viramontes, D., Vauclin, M., Gonzalez Barrios, J. L., and Esteves, M. (2001). Influence of Soil Surface Features and Vegetation on Runoff and Erosion in the Western Sierra Madre (Durango, Northwest Mexico). Catena 43, 115–135. doi:10.1016/S0341-8162(00)00124-7
Doerr, S. H., Shakesby, R. A., and Walsh, R. P. D. (2000). Soil Water Repellency: Its Causes, Characteristics and Hydro-Geomorphological Significance. Earth Sci. Rev. 51, 33–65. doi:10.1016/S0012-8252(00)00011-8
FAO (2009). Guía para la Descripción de Suelos. 4a ed. Roma: Organización de las Naciones Unidas para la Agricultura y la Alimentación. Available at: file:///C:/Users/Alina Belen Ortiz/Downloads/a0541s00 (1).pdf.
Farahnak, M., Mitsuyasu, K., Jeong, S., Otsuki, K., Chiwa, M., Sadeghi, S. M. M., et al. (2019). Soil Hydraulic Conductivity Differences Between Upslope and Downslope of Two Coniferous Trees on a Hillslope. J. For. Res. 24, 143–152. doi:10.1080/13416979.2019.1590967
Francos, M., Úbeda, X., Pereira, P., and Alcañiz, M. (2018). Long-Term Impact of Wildfire on Soils Exposed to Different Fire Severities. A Case Study in Cadiretes Massif (NE Iberian Peninsula). Sci. Total Environ. 615, 664–671. doi:10.1016/j.scitotenv.2017.09.311
García, E. (2004). Modificaciones al Sistema de Clasificación Climática de Köppen. Mexico: Instituto de Geografía Mexico city: Universidad Nacional Autónoma de México.
Gómez-Tagle Chávez, A., Geissert, D., and Enríquez-Fernández, E. (2014). Manual de Infiltrometría: Infiltrómetro de Tension INDI. Xalapa, Veracruz: Instituto de Ecología, AC.
Gómez-Tagle, A., Geissert, D., Pérez-Maqueo, O. M., Marin-Castro, B. E., and Rendon-Lopez, B. (2011). “Saturated Hydraulic Conductivity and Land Use Change, New Insights to the Payments for Ecosystem Services Programs: A Case Study From a Tropical Montane Cloud Forest Watershed in Eastern Central Mexico,” in Developments in Hydraulic Conductivity Research. Editor O. Dikinya (InTech), 270. doi:10.5772/15749
Gupta, S., Hengl, T., Lehmann, P., Bonetti, S., and Or, D. (2020). SoilKsatDB: Global Soil Saturated Hydraulic Conductivity Measurements for Geoscience Applications. Earth Syst. Sci. Data Discuss. 13, 1593–1612. doi:10.5194/essd-2020-149
INEGI (1985a). Conjunto de Datos Geográficos de la Carta Fisiográfica. Escala 1:1 000 000. Serie I (Continuo Nacional). Available at: https://inegi.org.mx/ (Accessed June 28, 2023).
INEGI (1985b). Conjunto de Datos Geográficos de las Cartas de Climas, Precipitación Total Anual y Temperatura Media Anual. Escala 1:1 000 000. Serie I (Continuo Nacional). Available at: https://inegi.org.mx/ (Accessed June 28, 2023).
INEGI (2013). Continuo de Elevaciones Mexicano (CEM). Available at: https://www.inegi.org.mx/app/geo2/elevacionesmex/ (Accessed June 28, 2023).
INEGI (2017). Conjunto de Datos Vectoriales de la Carta de Uso del Suelo y Vegetación Serie VI.Conjunto Nacional. Escala 1:250,000. Available at: https://inegi.org.mx/ (Accessed June 28, 2023).
IUSS Working Group WRB (2022). World Reference Base for Soil Resources. International Soil Classification System for Naming Soils and Creating Legends for Soil Maps. 4th ed. Vienna, Austria: International Union of Soil Sciences IUSS.
Jordán, A., Zavala, L. M., Mataix-Solera, J., and Doerr, S. H. (2013). Soil Water Repellency: Origin, Assessment and Geomorphological Consequences. Catena (Amst) 108, 1–5. doi:10.1016/j.catena.2013.05.005
Keeley, J. E. (2009). Fire Intensity, Fire Severity and Burn Severity: A Brief Review and Suggested Usage. Int. J. Wildland Fire 18, 116–126. doi:10.1071/WF07049
Keith, D. M., Johnson, E. A., and Valeo, C. (2010). A Hillslope Forest Floor (Duff) Water Budget and the Transition to Local Control. Hydrol. Process 24, 2738–2751. doi:10.1002/HYP.7697
Lal, R. (1997). Degradation and Resilience of Soils. Philosophical Trans. R. Soc. B Biol. Sci. 352, 997–1010. doi:10.1098/rstb.1997.0078
Letey, J., Carrillo, M. L. K., and Pang, X. P. (2000). Approaches to Characterize the Degree of Water Repellency. J. Hydrol. (Amst) 231–232, 61–65. doi:10.1016/S0022-1694(00)00183-9
Logsdon, S. D., and Jaynes, D. B. (1993). Methodology for Determining Hydraulic Conductivity With Tension Infiltrometers. Soil Sci. Soc. Am. J. 57, 1426–1431. doi:10.2136/sssaj1993.03615995005700060005x
Luxmoore, R. J. (1981). Micro-Meso-And Macroporosity of Soil. Soil Sci. Soc. Am. J. (United States) 45 (3), 671–672. doi:10.2136/SSSAJ1981.03615995004500030051X
Majid, N., Bahar, M. M., Harper, R., Megharaj, M., and Naidu, R. (2023). Influence of Biotic and Abiotic Factors on the Development of Non-Wetting Soils and Management Approaches: A Review. Soil Secur. 11, 100091. doi:10.1016/j.soisec.2023.100091
Marín-Castro, B. E., Geissert, D., Negrete-Yankelevich, S., and Gómez-Tagle Chávez, A. (2016). Spatial Distribution of Hydraulic Conductivity in Soils of Secondary Tropical Montane Cloud Forests and Shade Coffee Agroecosystems. Geoderma 283, 57–67. doi:10.1016/j.geoderma.2016.08.002
Mataix-Solera, J., Guerrero, C., Úbeda, X., Outeiro, L., Torres, P., Cerdà, A., et al. (2007). Incendios Forestales, Suelos Y Erosión Hídrica. Alcoi: Caja Mediterráneo.
Mataix-Solera, J., Cerdà, A., Arcenegui, V., Jordán, A., and Zavala, L. M. (2011). Fire Effects on Soil Aggregation: A Review. Earth Sci. Rev. 109, 44–60. doi:10.1016/j.earscirev.2011.08.002
Mataix-Solera, J., Arcenegui, V., Zavala, L. M., Pérez-Bejarano, A., Jordán, A., Morugán-Coronado, A., et al. (2014). Small Variations of Soil Properties Control Fire-Induced Water Repellency. Span. J. Soil Sci. 4, 51–60. doi:10.3232/SJSS.2014.V4.N1.03
Miller, A. D., Roxburgh, S. H., and Shea, K. (2011). How Frequency and Intensity Shape Diversity-Disturbance Relationships. Proc. Natl. Acad. Sci. U. S. A. 108, 5643–5648. doi:10.1073/pnas.1018594108
Miyazaki, T. (1988). Water Flow in Unsaturated Soil in Layered Slopes. J. Hydrol. (Amst) 102, 201–214. doi:10.1016/0022-1694(88)90098-4
Moghli, A., Santana, V. M., Baeza, M. J., Pastor, E., and Soliveres, S. (2022). Fire Recurrence and Time Since Last Fire Interact to Determine the Supply of Multiple Ecosystem Services by Mediterranean Forests. Ecosystems 25, 1358–1370. doi:10.1007/s10021-021-00720-x
Neary, D. G., Klopatek, C. C., DeBano, L. F., and Ffolliott, P. F. (1999). Fire Effects on Belowground Sustainability: A Review and Synthesis. For Ecol Manage 122, 51–71. doi:10.1016/S0378-1127(99)00032-8
Neris, J., Tejedor, M., Rodríguez, M., Fuentes, J., and Jiménez, C. (2013). Effect of Forest Floor Characteristics on Water Repellency, Infiltration, Runoff and Soil Loss in Andisols of Tenerife (Canary Islands, Spain). Catena (Amst) 108, 50–57. doi:10.1016/j.catena.2012.04.011
Ogle, R. A., and Schumacher, J. L. (1998). Fire Patterns on Upholstered Furniture: Smoldering Versus Flaming Combustion. Fire Technol. 34, 247–265. doi:10.1023/A:1015397907280
Olivares-Martinez, L. D., Gomez-Tagle, A., and Pérez-Salicrup, D. R. (2023). Regional Drivers Behind the Burning of Remanent Forests in Michoacán Avocado Belt, Central Mexico. Fire 6, 81. doi:10.3390/FIRE6030081
Olivares-Martínez, L. D. (2020). Regímenes de Incendios y sus Efectos en Suelos Forestales de la Franja Aguacatera. Available at: http://bibliotecavirtual.dgb.umich.mx:8083/xmlui/handle/DGB_UMICH/2809 (Accessed September 21, 2023).
Peel, M. C., Finlayson, B. L., and Mcmahon, T. A. (2007). Updated World Map of the Köppen-Geiger Climate Classification. Hydrol. Earth Syst. Sci. 11, 1633–1644. doi:10.5194/hess-11-1633-2007
Peng, Y., Holmstrup, M., Schmidt, I. K., De Schrijver, A., Schelfhout, S., Heděnec, P., et al. (2022). Litter Quality, Mycorrhizal Association, and Soil Properties Regulate Effects of Tree Species on the Soil Fauna Community. Geoderma 407, 115570. doi:10.1016/J.GEODERMA.2021.115570
Perroux, K. M., and White, I. (1988). Designs for Disc Permeameters. Soil Sci. Soc. Am. J. 52, 1205–1215. doi:10.2136/SSSAJ1988.03615995005200050001X
Poorter, L., van der Sande, M. T., Thompson, J., Arets, E. J. M. M., Alarcón, A., Álvarez-Sánchez, J., et al. (2015). Diversity Enhances Carbon Storage in Tropical Forests. Glob. Ecol. Biogeogr. 24, 1314–1328. doi:10.1111/geb.12364
Prescott, C. E., and Vesterdal, L. (2021). Decomposition and Transformations Along the Continuum From Litter to Soil Organic Matter in Forest Soils. For Ecol Manage 498, 119522. doi:10.1016/J.FORECO.2021.119522
R Core Team (2022). R: A Language and Environment for Statistical Computing. Available at: https://www.R-project.org/ (Accessed March 15, 2023).
Regalado, C. M., and Ritter, A. (2005). Characterizing Water Dependent Soil Repellency With Minimal Parameter Requirement. Soil Sci. Soc. Am. J. 69, 1955–1966. doi:10.2136/sssaj2005.0060
Regalado, C. M., and Ritter, A. (2006). Geostatistical Tools for Characterizing the Spatial Variability of Soil Water Repellency Parameters in a Laurel Forest Watershed. Soil Sci. Soc. Am. J. 70, 1071–1081. doi:10.2136/sssaj2005.0177
Regalado, C. M., and Ritter, A. (2008). Cokriging Spatial Interpolation of Soil Water Dependent Repellency Parameters Determined With Two Different Tests. Soil Sci. Soc. Am. J. 72, 1683–1693. doi:10.2136/sssaj2008.0008
Rein, G. (2015). Smoldering-Peat Megafires: The Largest Fires on Earth. Coal Peat Fires a Glob. Perspective 4, 1–11. doi:10.1016/B978-0-444-59510-2.00001-X
Ritsema, C. J., and Dekker, L. W. (1994). How Water Moves in a Water Repellent Sandy Soil: 2. Dynamics of Fingered Flow. Water Resour. Res. 30, 2519–2531. doi:10.1029/94WR00750
Rodríguez Rodríguez, J. (2014). Procesos y Factores del Flujo de Infiltración Edáfica en una Disturbosecuencia de Bosque de Pino-Encino del sur de la Cuenca de Cuitzeo, Michoacán. Avaliable At: http://bibliotecavirtual.dgb.umich.mx:8083/xmlui/handle/DGB_UMICH/1716 (Accessed March 03, 2014).
Ruthrof, K. X., Hopkins, A. J. M., Danks, M., O’Hara, G., Bell, R., Henry, D., et al. (2019). Rethinking Soil Water Repellency and Its Management. Plant Ecol. 220, 977–984. doi:10.1007/s11258-019-00967-4
Santoso, M. A., Christensen, E. G., Yang, J., and Rein, G. (2019). Review of the Transition From Smouldering to Flaming Combustion in Wildfires. Front. Mech. Eng. 5, 456580. doi:10.3389/fmech.2019.00049
Santoso, M. A., Christensen, E. G., Amin, H. M. F., Palamba, P., Hu, Y., Purnomo, D. M. J., et al. (2022). GAMBUT Field Experiment of Peatland Wildfires in Sumatra: From Ignition to Spread and Suppression. Int. J. Wildland Fire 31, 949–966. doi:10.1071/WF21135
Shakesby, R. A., and Doerr, S. H. (2006). Wildfire as a Hydrological and Geomorphological Agent. Earth Sci. Rev. 74, 269–307. doi:10.1016/j.earscirev.2005.10.006
Siebe, C., Reinhold, J., and Stahr, K. (1996). Manual Para la Descripción y Evaluación Ecológica de Suelos en el Campo. México: Sociedad Mexicana de la Ciencia del Suelo, A.C.
Špongrová, K., Kechavarzi, C., Dresser, M., Matula, S., and Godwin, R. J. (2009). Development of an Automated Tension Infiltrometer for Field Use. Vadose Zone J. 8, 810–817. doi:10.2136/vzj2008.0135
Tejera Hernández, B., Santos Ocampo, A., Santamaría, Q. H., Gómez Morales, T., and Olivares, V. C. (2013). El oro verde en Michoacán: ¿un Crecimiento sin Fronteras? Acercamiento a la Problemática y Retos del Sector Aguacatero Para el Estado y la Sociedad. Econ. Soc. 17, 15–40.
Varner, J. M., Gordon, D. R., Putz, F. E., and Kevin Hiers, J. (2005). Restoring Fire to Long-Unburned Pinus Palustris Ecosystems: Novel Fire Effects and Consequences for Long-Unburned Ecosystems. Restor. Ecol. 13, 536–544. doi:10.1111/j.1526-100X.2005.00067.x
Wallis, M. G., and Horne, D. J. (1992). “Soil Water Repellency,” in Advances in Soil Science. Editor B. A. Stewart (New York, NY: Springer), 91–146. doi:10.1007/978-1-4612-2930-8_2
Watson, K. W., and Luxmoore, R. J. (1986). Estimating Macroporosity in a Forest Watershed by Use of a Tension Infiltrometer. Soil Sci. Soc. Am. J. 50, 578–582. doi:10.2136/sssaj1986.03615995005000030007x
Weninger, T., Filipović, V., Mešić, M., Clothier, B., and Filipović, L. (2019). Estimating the Extent of Fire Induced Soil Water Repellency in Mediterranean Environment. Geoderma 338, 187–196. doi:10.1016/j.geoderma.2018.12.008
Woods, S. W., Birkas, A., and Ahl, R. (2007). Spatial Variability of Soil Hydrophobicity After Wildfires in Montana and Colorado. Geomorphology 86, 465–479. doi:10.1016/j.geomorph.2006.09.015
Wösten, J. H. M., Van Den Berg, J., Van Eijk, P., Gevers, G. J. M., Giesen, W. B. J. T., Hooijer, A., et al. (2006). Interrelationships Between Hydrology and Ecology in Fire Degraded Tropical Peat Swamp Forests. Int. J. Water Resour. Dev. 22, 157–174. doi:10.1080/07900620500405973
Woudt, B. D. (1959). Particle Coatings Affecting the Wettability of Soils. J. Geophys Res. 64, 263–267. doi:10.1029/JZ064I002P00263
Appendix
Equation 1: Gardner’s equation (1958).
Where K(ψ) is the unsaturated hydraulic conductivity at a specific water tension ψ, K(s) is the saturated hydraulic conductivity, and Gardner’s α is a shape parameter.
Equation 2: Logsdon & Jaynes’ non-linear approximation.
where q∞/πr2(ψ) is the volumetric flux in steady state by a specific water tension ψ, and r is the ratio of the infiltrometer circular base. The rest of the variables are the same as in Eq. A1.
Equation 3: Percentage of water flux by active macropores.
where PF is the flux percentage between the tension according to the macropore radius of 0.5 mm, corresponding to a water tension hM of −0.0297 m (according to Jurin’s Law), Ks is field saturated hydraulic conductivity (m ⋅ s−1) with its Gardner’s α.
Equation 4: Number of active pores per unit area.
where N is the number of effective pores per unit area A, μ is de dynamic water viscosity (kg ⋅ m−1 ⋅ s−1), ρ is the density of water (kg ⋅ m−3), g is the gravity acceleration (m ⋅ s−2), K(hM) is the field saturated hydraulic conductivity for the macropore water tension hM (m ⋅ s−1), r(hM) is the macropore radius (m), and r(I) is the infiltrometer base radius (m).
Keywords: soil water repellency, smoldering fire, resilience, mountain forest, field saturated hydraulic conductivity
Citation: Olivares-Martinez LD, Gomez-Tagle A and Mataix-Solera J (2023) Ground Fire Legacy Effects on Water-Dynamics of Volcanic Tropical Soils. Span. J. Soil Sci. 13:11757. doi: 10.3389/sjss.2023.11757
Received: 30 June 2023; Accepted: 05 October 2023;
Published: 23 October 2023.
Edited by:
Xavier Úbeda, University of Barcelona, SpainCopyright © 2023 Olivares-Martinez, Gomez-Tagle and Mataix-Solera. This is an open-access article distributed under the terms of the Creative Commons Attribution License (CC BY). The use, distribution or reproduction in other forums is permitted, provided the original author(s) and the copyright owner(s) are credited and that the original publication in this journal is cited, in accordance with accepted academic practice. No use, distribution or reproduction is permitted which does not comply with these terms.
*Correspondence: Luis D. Olivares-Martinez, bG9saXZhcmVzQHVtaC5lcw==