- 1Department of Plant Biology and Soil Science, Area of Soil Science and Agricultural Chemistry, Faculty of Sciences, University of Vigo, Ourense, Spain
- 2Agroecology and Food Institute (IAA), University of Vigo—Campus Auga, Ourense, Spain
- 3Department of Soil Science and Agricultural Chemistry, Engineering Polytechnic School, University of Santiago de Compostela, Lugo, Spain
This study aims to understand the adsorption/desorption process in six agricultural soils of two antibiotics, Ciprofloxacin (CIP) and Trimethoprim (TRI), widely used today and the influence of pH on this process. Antibiotics can reach the soil through the application of sludge and effluents from wastewater treatment plants and are directly influenced by changes in pH, once in the soil. Therefore, this study with batch experiments allows us to know the adsorption process in a pH range between 2 and 12, in six soils with different organic carbon content, between 1% and 7.7%. The results obtained show that the adsorption of CIP has its maximum at pH between 5 and 7; above and below this range, the adsorption decreases. The soils with the highest organic carbon content (between 4.4% and 7.7%) are those with the highest adsorption. The values for each forms in which the CIP molecule is found are: for KdCIP+, between 0.887 and 8.289 L kg−1; for KdCIP−, between 0.670 and 5.440 L kg−1, while for KdCIP0, the values do not differ from 0, except soils 1 and 3, whose values are 0.206 and 0.615 L kg−1, respectively. Regarding TRI, the maximum adsorption takes place at acidic pHs, below 6 for all soils. Above these values, desorption decreases. The Kd values for each of the species vary between 0.085 and 0.218 L kg−1 for KdTRI+, between 0.011 and 0.056 L kg−1 for KdTRI0, and between 0.092 and 0.189 L kg−1 for KdTRI−. For both antibiotics, the highest adsorption was achieved in the soil with the highest organic carbon content (7.7%). Comparing both antibiotics, we see that CIP presents the highest adsorption, and in the case of desorption, for CIP, it varies between 3.7% and 75.8%, with the maximum desorption at basic pHs. In the case of TRI, desorption is higher, varying between 9.4% and 99.1%, with the maximum around neutrality, except for two soils, whose maximums are at pH of 4.3 and 9.5. These results should be taken into account, as once they reach the soil, pH will be a determining factor in their behaviour and fate.
Introduction
One of the main problems in agricultural ecosystems is the presence of widely dispersed pollutants in the environment, such as heavy metals, pesticides or emerging contaminants, such as antibiotics (Khan et al., 2022). These chemicals, once they reach the environment, can be uptaken by crops, entering the food web, thus reaching humans, accelerating the development and dissipation of antibiotic-resistant bacteria and genes (Anuar et al., 2023). Therefore, maintaining the safety of agricultural soils must be a priority to prevent the spread of resistant genes and bacteria, ensuring food security and ecological balance (Lu et al., 2023). To this end, the processes that can influence the fate of these contaminants, such as biodegradation, photodegradation, hydrolysis or sorption (Kashyap et al., 2023), as well as factors that influence these processes, such as the edaphic properties of the soil, the properties of the contaminants themselves, as well as external factors such as environmental and climatic agents, among others, must be taken into account.
In the case of antibiotics, they can reach the soil as well as waterbodies after the application of water from wastewater treatment plants (WWTPs) as irrigation water and by the application of sewage sludge as manure and with a great antibiotic adsorption potential (Tran et al., 2018; Barreiro et al., 2022). This is because, in WWTPs, treatments do not completely remove those pollutants that reach them through human urine and faeces (Anuar et al., 2023), finding an average of 2,563 ng L−1 for Ciprofloxacin (CIP), one of the antibiotics of this study, and 17,387 ng L−1 for the other, Trimethoprim (TRI) (Sagaseta de Ilurdoz et al., 2022).
Therefore, it is necessary to know the behaviour of these compounds once they reach the soil or waterbodies, in order to determine their fate and prevent them from reaching humans. Exposure of humans to high concentrations of these compounds can reduce their immunity or even cause infections (Xiang et al., 2019). One of the main processes influencing their fate is adsorption to the soil surface. This process will depend on multiple factors of the antibiotics, such as their log KOW, pKa, ionisation state, binding properties and buffering conditions (Tran et al., 2018; Jodeh et al., 2022). The pH of the medium will play a fundamental role in the different process in soil like degradation (Rodríguez-López et al., 2022a) or mainly in adsorption and will clearly influence its fate since antibiotics tend to behave differently depending on the pH, as they are amphoteric molecules, which can behave as anions, zwitterions, or cations. The pH not only influences the solubility and behaviour of the compounds but also the characteristics of the sorbent and can change the interactions between the contaminant and the sorbent (Anuar et al., 2023).
Previous studies have indicated that adsorption/desorption of CIP and TRI onto soils have been influenced by soil properties such as mineral fractions and extreme values of carbon and nitrogen contents (Rodríguez-López et al., 2022b; Mejías et al., 2023). Although both were widely detected in agricultural soils (Chen et al., 2023), the knowledge related to the adsorption of both antibiotics is contradictory or scarce. Some studies have been conducted with CIP to understand the role of pH (Carmosini and Lee, 2009; Vasudevan et al., 2009; Pasket et al., 2022), but results are contradictory, while studies for TRI are scarce (Mejías et al., 2023). In this sense, it is important to understand their fate after they arrive in the soil and see the possible changes in adsorption as a function of variations in pH that take place in the soil due to multiple different processes, such as plant exudates waterlogging, among others. Therefore, the main objective of this work is to study the influence of pH on the adsorption of CIP and TRI under different soil organic carbon contents.
Material and Methods
Chemical Products
The antibiotics, ciprofloxacin (CIP), a fluoroquinolone, and trimethoprim (TRI), a diaminopyrimidine, have a purity of 98% and were provided by Sigma-Aldrich (Barcelona, Spain). Their characteristics can be found in Rodríguez-López et al. (2022b). They present two dissociation constants (pKa) each one, being the values for CIP 6.09 and 8.74 (Dimpe and Nomngongo, 2019); and in the case of TRI, 6.16 and 7.16 (Ghirardini et al., 2020). Once we have the values of dissociation constants, we can obtain the speciation diagram depending on pH. For each one of the antibiotics, as Figure 1 shows.
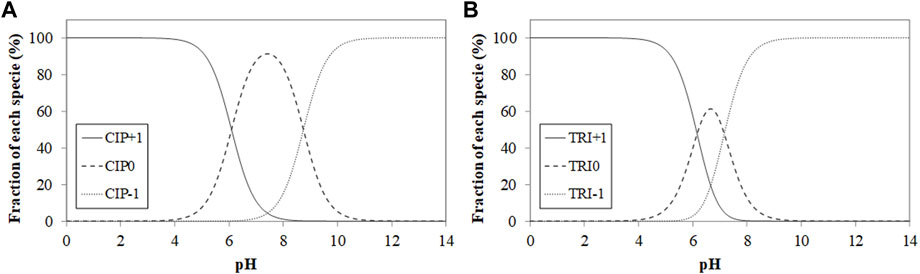
FIGURE 1. Ciprofloxacin (A) and trimethoprim (B) speciation diagrams as a function of pH (CIP+1 and TRI+1 = cationic species; CIP0 and TRI0 = not charged species; CIP−1 and TRI−1 = anionic species).
Soil Samples
For this study, six agricultural soils were collected at three different points (Valdeorras, Rías Baixas and A Coruña province) in Galicia (NW Spain). The samples were selected by the variety of soil organic carbon content (SOC), ranging between 1% and 7.7%. Three of the soils (1–3) are from vineyards, and the other three are from corn crops (4–6). The analysis of the soil samples was described in detail in a previous study (Rodríguez-López et al., 2022b).
Adsorption/Desorption Experiments in the Function of pH
The adsorption experiment is carried out with six soils at different pH values. To adjust the pH from 2 to 12, 0.5 M HCl and 0.5 M NaOH are used in different amounts, in the same way that Ferraro et al. (2023) did. The amount of antibiotic, CIP or TRI, added was 400µM, with a ratio soil:solution 0.5:40. The background electrolyte used was 0.005 M CaCl2. The suspensions were shaken at 50 rpm for 48 h in darkness, and then, they were centrifuged at 4,000 rpm for 15 min. Then, the samples were filtered, the supernatant was separated with a syringe and nylon filters, and the soil was used for the desorption study. The supernatant is measured for pH with a combined glass micro-electrode. The quantity of antibiotic was calculated as the difference between the amount initially added and the amount remaining in the solution after 48 h.
For desorption samples, the soil of samples of the adsorption process was weighed, and then, at these samples, 5 mL of 0.005 M CaCl2 with corresponding amounts of HCl or NaOH in the pH function were added. Then, once resuspended, the samples were shaken for 48 h, centrifuged for 15 min, filtered with a syringe and nylon filters, and analysed in the same way that samples of the adsorption process.
Quantification of the Antibiotics
The concentration of antibiotics in the supernatant for adsorption and desorption was measured with chromatography liquid equipment (Ultimate 3000 HPLC, Thermo Fisher Scientific, Madrid, Spain). A detailed description of the equipment was given by Rodríguez-López et al. (2021), and the measurement conditions for the two antibiotics by Rodríguez-López et al. (2022b). Briefly, the equipment is composed of a quaternary pump, an autosampler, a thermostatted columns compartment and an ultraviolet-visible detector. The characteristics of the analytical column (Luna C18) were 150 mm long, 4.6 mm internal diameter, 5 µm particle size (Phenomenex (Madrid, Spain)), and for the safety column, 4 mm long, 3 mm ID, 5 µm particle size. Both are packed with the same material. The quantification limits for both antibiotics, CIP and TRI, were 0.1 µM and 0.05 µM, respectively, while the detection limits were 0.03 µM and 0.015 µM. The injection volume and selected flow used were 50 µL and 1.5 mL min−1, respectively. The mobile phases used were acetonitrile (Phase A) and 0.01 M phosphoric acid (pH 2) (Phase B). The initial conditions were maintained for 0.5 min, 95% phase B and 5% phase A, then decreased from 5% to 32% for phase A and from 95% to 68% for phase B, in a period of 10.5 min. The initial conditions were restored in 2 min and were maintained for 3 min until the analysis time was finished. The time needed for total analysis was 15 min, and the retention times, were 6.5 min for CIP, and 5.6 min for TRI. The wavelength used was 212 nm.
Electro-Kinetic Determinations
In order to measure the soil’s electrokinetics, the electrophoretic apparatus was used (Zetasizer Nanoseries 3600 micro-electrophoresis Malvern Instruments, Malvern, United Kingdom). The results were shown as Z-potential values. Previously, the fine soil fraction was separated through a 0.05-mm-mesh sieve. To carry out this experiment, 50 mg of fine soil fraction suspended in 15 mL of 0.01 M NaNO3 was taken, adjusting the pH between 2 and 11 with HNO3 or NaOH. Following the method of Álvarez-Esmorís et al. (2022), the samples were shaken for 1 h and 700 µL of the sample was taken for measurement in the apparatus. The experiment was carried out in duplicate for each soil, and 30 replicate runs were performed for each sample of fine soil fraction.
Data Treatment
The model used to calculate the adsorption coefficients (Kd) corresponds to the different forms of the antibiotics (Kd+ is the adsorption coefficient for the positively charged form of the antibiotic; Kd0 is the coefficient for the zwitterion form; Kd- is the coefficient for the negatively charged form). Equation 1 (Eq. 1) (Álvarez-Esmorís et al., 2022) has been used to calculate them:
where, Kd is the total adsorption coefficient (L kg−1), which is calculated at different pHs, and α is the fraction of each antibiotic species as a function of pH. Specifically, α+, α0 and α− represent the proportion of the different chemical species of the antibiotic obtained from the pKa values (De-Levie, 1999; Enke, 2001). The Kd+ is the adsorption coefficient for the positively charged form of the antibiotic; Kd0 is the coefficient for the zwitterion form, and Kd- the coefficient for the negatively charged form. SPSS 21 software was used to obtain the fits of the experimental data to the model. All determinations were made in triplicate.
Results and Discussion
Soil Characteristics
The analysis results, Table 1, show that the pH range varies between 5.7 and 7.3 for pHH2O, but in the case of pHKCl, the range is lower, between 5.0 and 6.5. The values of the capacity cationic exchange vary between 5.43 and 24.35 cmol+ kg−1, and Cae is the majority cation. The soils have a carbon organic content of 1%–7.7% and a nitrogen content between 0.1% and 0.6%. The majority fraction of the soil is sand, and all soils are sandy loam.
Figure 2 shows the Z-potential for all the samples, and we can see that the potential is always negative for the pH range studied (2–12). The range of values is between −5.8 and −48.5 mV, and as the pH increases, the Z-potential of the soil decreases, which corresponds to that obtained by other authors such as Pérez-Novo et al. (2009), indicating that the soils had significant fractions with variable charge. The fact that the charge is always negative shows that the negative charges of the soil prevail over the positive ones.
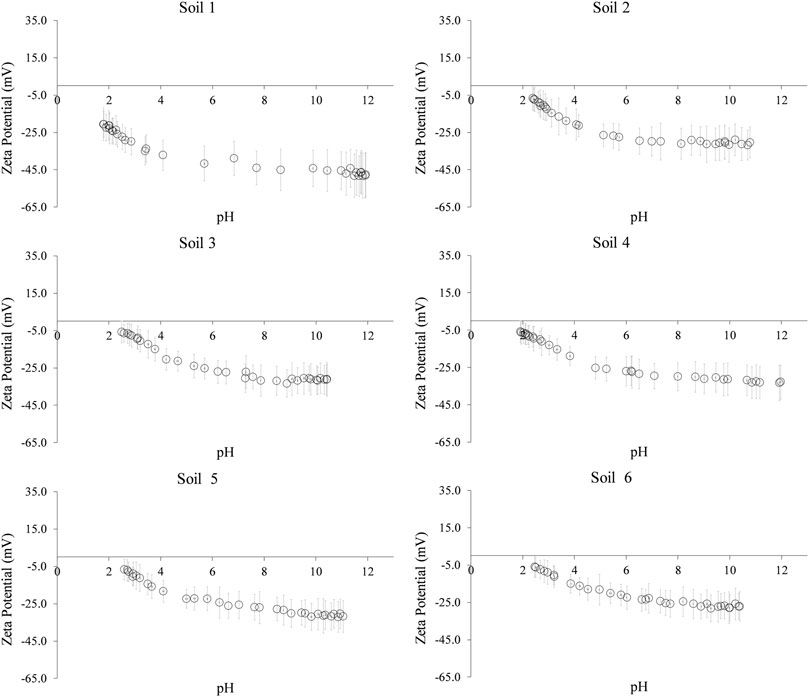
FIGURE 2. Graphs showing Zeta-potential values versus pH for the fine soil fraction extracted from the soil samples. Average values (n = 60). Error bars represent the standard deviation.
Adsorption
Figure 3 shows the results for the CIP in the six studied soils, and we can see that the behaviour is like those obtained by authors such as Jalil et al. (2015). It can be seen how the lowest adsorption corresponds to the most basic pHs, being around pH 10, where the curves of all soils decrease their adsorption values. On the contrary, the maximum adsorption, with values close to 30,000 μmol kg−1 for all soils except for soil 1, which presents values of 16,000 μmol kg−1, is found between pHs 5 and 7, except for soil 3, which is at pH 3.0. The maximum adsorption is found around pH 6.1, which is one of the pKa values of the antibiotic. Moreover, that the adsorption maximum is close to 7 may be due to the high hydrophobicity of the zwitterionic form of CIP, which implies higher adsorption at that pH (Li et al., 2014; Ncibi and Sillanpää, 2015). This compound shows higher solubility when it behaves as an ion, that is, as an anion or cation (Jalil et al., 2015), below or above pH 7.
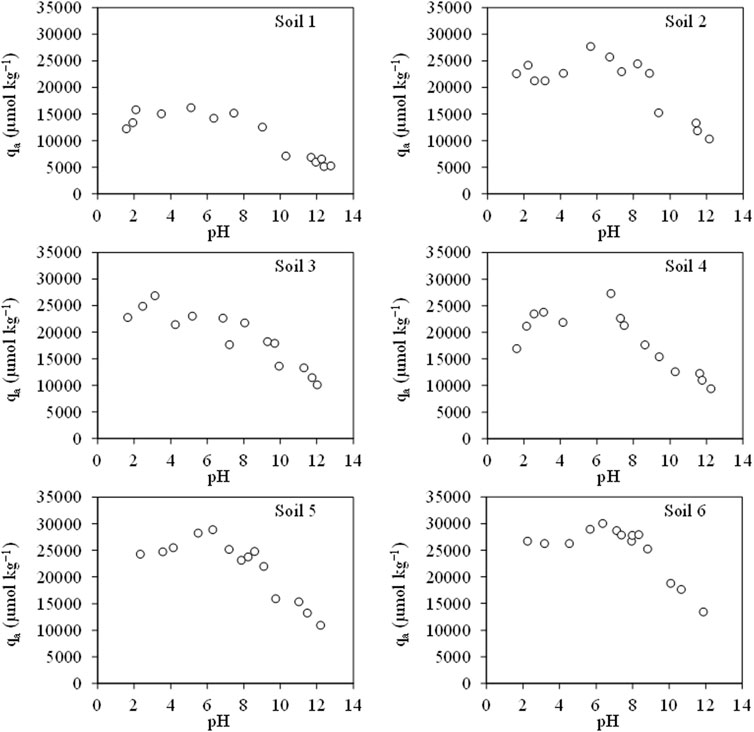
FIGURE 3. Ciprofloxacin adsorption in six soil samples in the function of pH. Average values (n = 3).
Above pH 7, we can see that adsorption shows a tendency in all soils to decrease, similar to that obtained by other authors such as Liu et al. (2022) or Xiang et al. (2019), who observed a decrease in adsorption at values above 7, as pH increases, and on the other hand, below 7, as pH increases, adsorption increases. The low adsorption at acidic pHs may be associated with the fact that the anionic form of the molecule (CIP−) may have repulsive interactions with the clay mineral negative surface (Jalil et al., 2015), and in this case, all soils are negatively charged (Figure 2).
Liu et al. (2022) show that the predominant adsorption mechanisms below pH 7.0 are hydrogen bonding or π-π interactions. However, other authors, such as Xiang et al. (2019), show that the main mechanisms for fluoroquinolones are hydrophobic attraction, electrostatic interaction or π-π dispersion force.
Among the soil properties, organic carbon has the greatest influence on CIP adsorption. Those soils with the highest carbon content are the soils with the highest adsorption values (between 27,500 and 31,000 μmol kg−1), being soils 2, 5 and 6. Authors such as Chen et al. (2023) have seen this relationship, but in this case for violet soils. Moreover, at pH 7.0, the point of maximum adsorption for most soils, the adsorption coefficient (Kd) was significatively correlated with the organic matter content (r = 0.864, p < 0.05).
Regarding the CIP molecule, it has a carboxylic acid group and an amino group in the piperazine moiety. The deprotonation of the carboxylic group causes the molecule to form complexes with positively charged sorbents, while the protonation of the amide group facilitates cation exchange on negatively charged surfaces (Li et al., 2014; Liu et al., 2022).
Since the antibiotic CIP has two pKa values, its speciation will depend on pH. Considering the pKa values, 6.09 and 8.74, we can obtain the Kd values for each of the species of the same by means of Eq. 1. Table 2 shows the values for each of the soils. We can see that the adjustments are significant judging by the R2 values, which vary between 0.504 and 0.874.
The results obtained from the equation show that all soils have higher values for the CIP+ species, except for soil 3, where the opposite occurs; the highest value is the Kd of the CIP− species. The values for the Kd of CIP+ are between 0.887 and 8.289 L kg−1, while for KdCIP− are from 0.670 to 5.440 L kg−1. In the case of CIP0, the values do not differ from 0, with the exception of soils 1 and 3, with values between 0.2 and 0.7 L kg−1, which may be due to the low solubility of the molecule in this zwitterionic form (Jalil et al., 2015). Soils 2, 5 and 6 show the highest values for the Kd of CIP+, between 3.529 and 8.289 L kg−1, which corresponds to soils with a higher organic carbon content, mainly soil 6, which is the soil with the highest values for three parameters, for KdCIP+, KdCIP− and SOC, being, 8.289, 5.440 L kg−1 and 7.7%, respectively.
The adsorption data as a function of pH for the TRI are shown in Figure 4. Here, we can see how the trend in all soils is the same, with the highest adsorption at acidic pHs and decreasing as the pH rises, which corresponds to the results obtained by other authors, such as Zhang et al. (2014), who attribute this behaviour to the pKa values of the antibiotic. In acidic systems, at low pHs, the antibiotic is found in its cationic form, while in neutral and basic systems, at pH 7 and above, it is found in its neutral and negative form. This means that the antibiotic has a higher affinity for the negative charges of the soil in its cationic form.
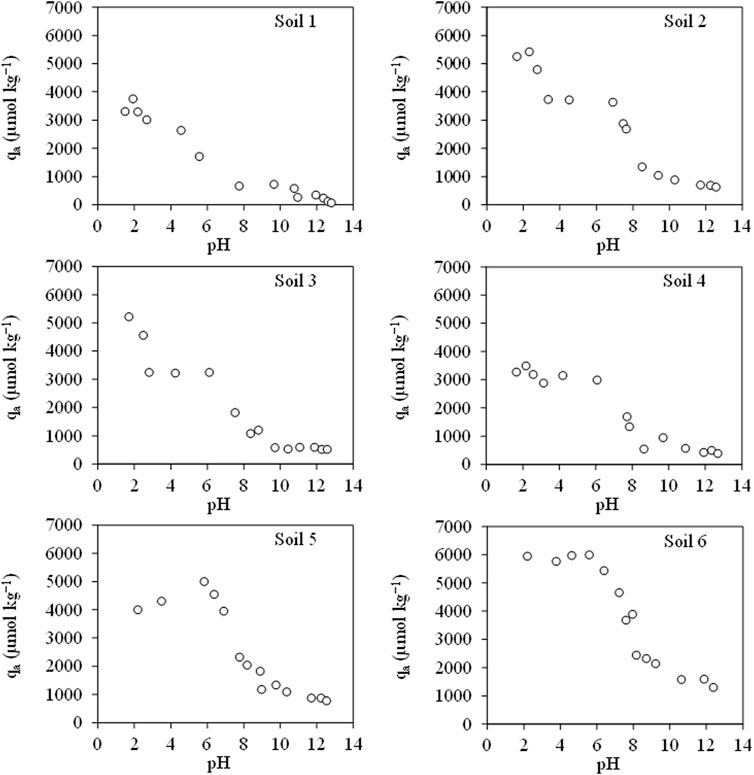
FIGURE 4. Trimethoprim adsorption in six soil samples in the function of pH. Average values (n = 3).
The maximum adsorption is found around pH 2 for soils 1, 2, 3 and 4 and around 6 for soils 5 and 6. Mpatani et al. (2021) showed that, according to the bioadsorbent, TRI would have the maximum adsorption around pH 5.04 in the case of montmorillonite, while in the case of charcoal synthesised from chicken feathers as an adsorbent, the maximum was at pH 7.5, close to the pKa2 value. Considering that the characteristics of by-products are different from those of soils, this variability in the maximum adsorption may be due to this factor; however, results obtained for soils, as indicated by Zhang et al. (2014), show that there is a correspondence to those obtained in this study. They found the maximum adsorption at pH 6.0, which may be due to the dominance of the neutral form of the antibiotic, which means that there is a strong attraction to the electronegative surface of the soil.
The maximum adsorption values are between 3,400 and 6,000 μmol kg−1, which are much lower than those obtained for CIP, indicating higher adsorption of CIP than TRI, as seen by other authors such as Rodríguez-López et al. (2022b). The adsorption of TRI is attributed by authors such as Kocárek et al. (2016) to its methoxy group, as it is able to behave as a proton acceptor within H-bonds.
In the case of TRI, factors such as organic matter content are not determinant to obtain clear conclusions on how it influences the adsorption of the antibiotic, as there is no correlation between the maximum adsorption values and this parameter. This corresponds to what has been obtained in other studies (Kocárek et al., 2016; Mejías et al., 2023).
Regarding TRI adsorption mechanisms, authors such as Mpatani et al. (2021) point out cation-π and π-π interactions. However, authors such as Li and Zhang, (2017) associate hydrophobic partitioning with the adsorption mechanism when the molecule is in its neutral state and the mechanism of electrostatic forces when the molecule is in its anionic state. For Kocárek et al. (2016), the main adsorption mechanisms are cation exchange, hydrogen bonding and Van der Waals forces.
Trimethoprim, as with CIP, presents two pKa (6.16 and 7.16); therefore, it will present three species as a function of pH. Table 3 shows the Kd values for each species obtained after the application of Eq. (1). We can see that the equation fits the experimental data better than for the other antibiotic, judging by R2, which is higher than in the case of CIP, being in the range between 0.883 and 0.968.
We can observe, as in the case of CIP, that the Kd values for the TRI+ species are higher than the values for the other species, followed by TRI− and the lowest TRI0 values, with the exception of soil 3, where the highest value is for TRI−, TRI+ and lastly TRI0. The highest values for the TRI+ species, about the other species, correspond to the highest adsorption values for TRI, since the maximum adsorption of this antibiotic takes place at acid pHs (Mejías et al., 2023). The values for TRI+ vary between 0.085 and 0.218 L kg−1. In the case of TRI0, the values range from 0.011 to 0.056 L kg−1, and finally, for TRI−, the values are between 0.092 and 0.189 L kg−1. As with CIP, it should be noted that the soil with the highest values is soil 6, with values of 0.218, 0.056 and 0.189 L kg−1 for KdTRI+, KdTRI0 and KdTRI−, respectively. Authors such as Kocárek et al. (2016) have seen how, for different soils, the majority of species is cationic (TRI+), as in the results obtained.
Desorption
Figure 5 shows the desorption percentages for the antibiotic CIP and, in the second axis, the amount of adsorbed CIP after a desorption cycle. The percentages vary between 3.7% and 45.9%, with the exception of soil 1, which shows higher desorption, with values between 18.6% and 75.8%, which corresponds to the soil with the lowest adsorption values after one desorption cycle, as indicated by Figure 5. The fact that soil 1 shows higher desorption may be related to its low organic carbon content; in fact, it is the soil with the lowest percentage compared to the others, 1%. The maximum values for desorption for each soil are between 24% and 45.9%, except for soil 1, which has a maximum of 76% and in adsorption values after a desorption cycle, the range is between 13,000 and 29,000 μmol kg−1. The maximum values of desorption are found for soils 1, 5 and 6 at basic pHs above 12, while for the other soils (2, 3 and 4), between 8 and 10, and in the case of the maximum of adsorbed amount corresponds to pH values between 3 and 6 for all soils, which corresponds to the adsorption data obtained. In general, at low pHs, desorption values are low at around 20% for all soils, and as pH increases, so does desorption; this trend can be seen to hold for all soils.
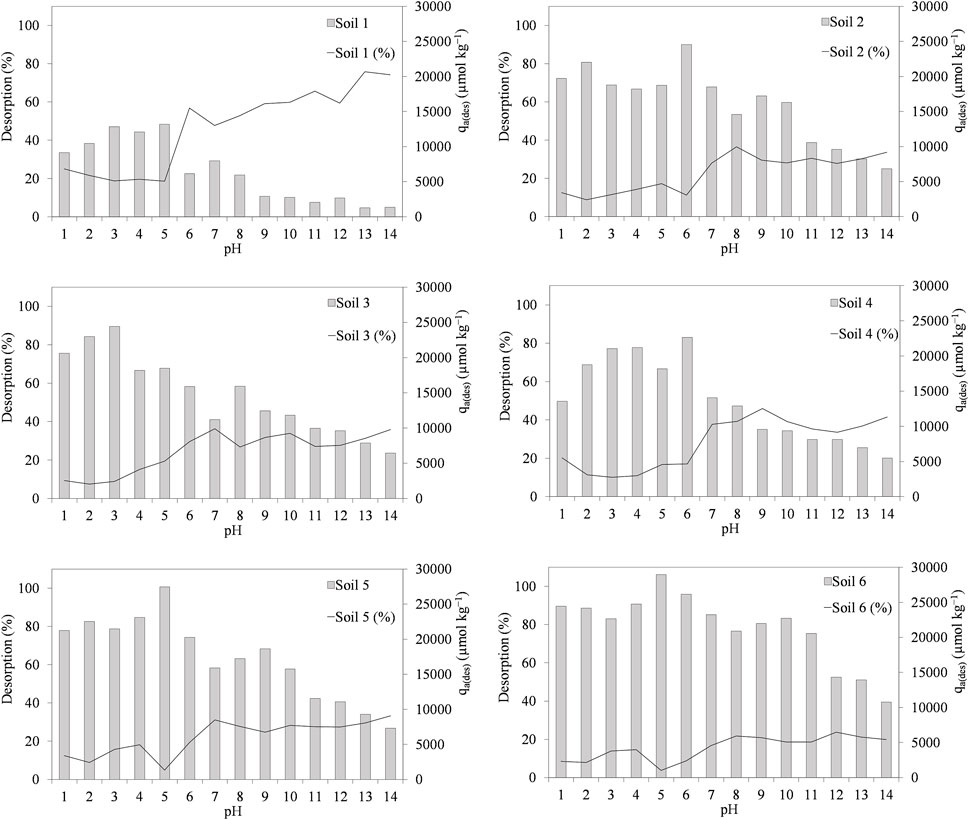
FIGURE 5. Ciprofloxacin desorption percentages and ciprofloxacin amount adsorbed after a desorption cycle (µmol kg−1) as a function of pH in the different soils. Average values (n = 3).
Many antibiotics, such as in this case fluoroquinolones or tetracyclines, have hydrophilic characteristics, hence some desorption, but at the same time, they tend to have high adsorption, as they have certain electrostatic interactions with soil components (Tran et al., 2018).
We can observe that, for the soils under study, the desorption of the antibiotic TRI is higher than CIP, as can be seen in Figure 6, where the desorption percentages are shown as a function of pH for this antibiotic, as well as, the amount of TRI adsorbed after a desorption cycle in μmol kg−1 in the second axis. Here, we can see how desorption presents a maximum of close to 100% in all soils. This maximum is found for all soils around pH 7.0, except for soil 1, which presents a maximum at pH 4.0 and for soil 6, which presents a maximum at pH 9.5. Regarding the amount adsorbed after the desorption process, it can be seen that the values are lower than those of CIP, with the maximum values varying between 900 and 2,700 μmol kg−1 and the maximum for all soils are found at very acidic pH, around values of 2. The trend for soils 2, 3 and 4 is that desorption at acidic and basic pHs decreases with respect to pH 7.0, where the maximum desorption is found, coinciding in the range of the two pKa values of TRI (6.16 and 7.16). The desorption percentages for these soils are between 35.9% and 99.1%.
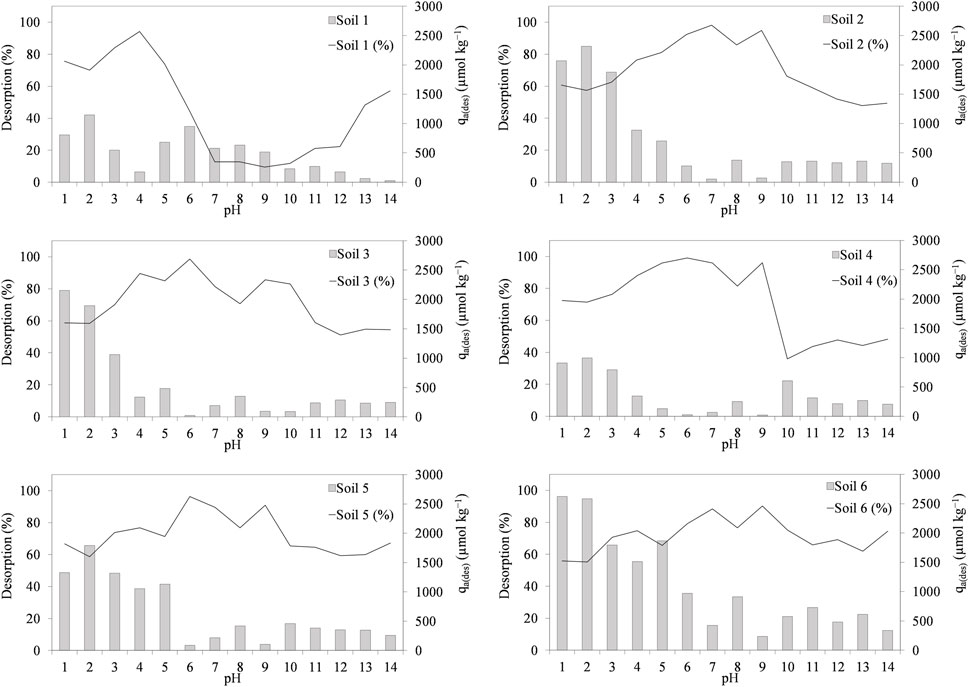
FIGURE 6. Trimethoprim desorption percentages and trimethoprim amount adsorbed after a desorption cycle (µmol kg−1) as a function of pH in the different soils. Average values (n = 3).
On the other hand, for soil 1, the behaviour is slightly different. We observe a maximum desorption at acidic pHs, and then a decrease as pH increases, and at very basic pHs above 12, desorption increases again. The desorption values in this soil vary over a wide range between 9.4% and 94.2%. In the case of soils 5 and 6, desorption is more linear, with all values between 55% and 97%, without a clear tendency to increase or decrease with pH change, as in the other soils. However, in the case of the amount adsorbed after the desorption process, we see for all soils that the amount decreases when pH increases. The desorption percentages are much higher than those found by other authors, such as Li and Zhang, (2017), for marine sediments, who have found that desorption percentages do not exceed 42%. Within the TRI molecule, the hydroxyl group probably has a positive effect on adsorption but not on desorption, as it forms new hydrogen bonds with the soil (Mejías et al., 2023).
With these data, we can say that CIP is an antibiotic that is less mobile than TRI, but at the same time, TRI is less mobile than other antibiotics, such as sulphonamides (Zhang et al., 2014). Moreover, as other authors such as Mejías et al. (2023) have seen, among the different factors that influence adsorption and desorption processes, such as the pH of soil for ionisable compounds, clay content, organic matter content, soil texture or cation exchange capacity, pH is one of the most important with regard to organic pollutants, as is the case of antibiotics, since they have different pKa that determine their speciation.
Conclusion and Future Perspectives
This study shows the different partitioning of two widely used antibiotics, CIP and TRI, as a function of pH. It can be observed that the adsorption of CIP shows higher values than TRI. As for CIP, the maximum adsorption is between 5 and 7, coinciding with both pKa of the molecule. As pH increases, adsorption decreases. The main mechanisms that can be associated with the adsorption of this compound are hydrogen bridges, π-π interactions, hydrophobic attraction, electrostatic interaction or π-π dispersion force. Regarding TRI adsorption, we can observe that the maximum adsorption values are found between pH 2 and 6, and at higher pH, the adsorption decreases for all soils. The main adsorption mechanisms are cation-π, π-π interactions, hydrophobic partitioning, electrostatic forces, cation exchange, hydrogen bonding and Van der Waals forces.
In terms of desorption, we can see that both show some desorption, presenting the maximum at different pHs, in the case of CIP, at basic pHs, but on the other hand, in the case of TRI, at pHs close to neutrality. It is observed that the mobility of TRI, with desorption percentages between 9.4% and 99.1%, is higher than CIP, which presents percentages between 3.7% and 75.8%.
For these reasons, it is necessary to take into account that certain agricultural and livestock activities, such as liming or the use of chemical compounds or simply strong changes in topography or climate that can significantly influence pH, can cause these antibiotics to be more mobile in the soil and move up the food web, and finally reaching humans. However, these changes will depend primarily on the edaphic characteristics of the soils themselves; it is therefore necessary to always be aware of all the variables that can affect them. Further studies should be focused on the combined effects of environmental factors (e.g., salinity, pH, and temperature) on antibiotic adsorption/desorption processes or find measures to enhance the process, such as the addition of different by-products that are used as bioadsorbents, such as wood waste, animal waste (shells or feathers) or biochar, for example.
Data Availability Statement
The original contributions presented in the study are included in the article, further inquiries can be directed to the corresponding author.
Author Contributions
Conceptualization, VS-M, AN-D, EÁ-R, AR-S, and MA-E; methodology, VS-M, AN-D, EÁ-R, AR-S and MA-E; software, LR-L, VS-M, and RC-D; investigation, LR-L and RC-D; data curation, LR-L, VS-M, AN-D, EÁ-R, AR-S, and MA-E; writing—original draft preparation, LR-L, VS-M, AR-S, and MA-E; writing—review and editing, AR-S; visualization, LR-L, VS-M, RC-D, AN-D, EÁ-R, AR-S, and MA-E; supervision, VS-M, AN-D, EÁ-R, AR-S, and MA-E; validation, VS-M, AN-D, EÁ-R, AR-S, and MA-E. All authors contributed to the article and approved the submitted version.
Funding
This work was funded by the Spanish Ministry of Science, Innovation and Universities with European Regional Development Funds (FEDER in Spain) (grant numbers RTI 2018-099574-B-C21 and RTI 2018-099574-B-C22). The authors acknowledge the financial support of the “Consellería de Cultura, Educación e Universidade (Xunta de Galicia)” through the contract ED431C2021/46-GCR granted to the research group BV1 of the University of Vigo. LR-L is holder of a pre-doctoral FPU contract (FPU19/03758; Ministry of Universities, Spanish Government), VS-M is holder of a postdoctoral fellowship (ED481B-2022-081; Xunta de Galicia, Spain), AR-S is supported by a JdCi research contract funded by MCIN/AEI/UVigo and European Union EU/PRTR (IJC 2020-044197-I/MCIN/AEI/10.13039/501100011033).
Conflict of Interest
The authors declare that the research was conducted in the absence of any commercial or financial relationships that could be construed as a potential conflict of interest.
References
Álvarez-Esmorís, C., Rodríguez-López, L., Núñez-Delgado, A., Álvarez-Rodríguez, E., Fernández-Calviño, D., and Arias-Estévez, M. (2022). Influence of pH on the Adsorption-Desorption of Doxycycline, Enrofloxacin, and Sulfamethoxypyridazine in Soils With Variable Surface Charge. Environ. Res. 214, 114071. doi:10.1016/j.envres.2022.114071
Anuar, N. F., Shah, DRSI, Ramli, F. F., Zaini, M. S. M., Mohammadi, N. A., Daud, A. R. M., et al. (2023). The Removal of Antibiotics in Water by Chemically Modified Carbonaceous Adsorbents From Biomass: A Systematic Review. J. Clean. Prod. 401, 136725. doi:10.1016/j.jclepro.2023.136725
Barreiro, A. P., Cela-Dablanca, R., Nebot, C., Rodríguez-López, L., Santás-Miguel, V., Arias-Estévez, M., et al. (2022). Occurrence of Nine Antibiotics in Different Kinds of Sewage Sludge, Soils, Corn and Grapes After Sludge Spreading. Span. J. Soil Sci. 12, 10741. doi:10.3389/sjss.2022.10741
Carmosini, N., and Lee, L. S. (2009). Ciprofloxacin Sorption by Dissolved Organic Carbon From Reference and Bio-Waste Materials. Chemosphere 77, 813–820. doi:10.1016/j.chemosphere.2009.08.003
Chen, G., Wu, J., Ma, J., Xu, M., Long, L., Chen, C., et al. (2023). Ciprofloxacin Sorption to Purple Soil: Potential Mechanisms and Factors Influencing Sorption. J. Environ. Chem. Eng. 11, 110626. doi:10.1016/j.jece.2023.110626
De-Levie, R. (1999). Aqueous Acid-Base Equilibria and Tritation. Oxford, New York, USA: Oxford University Press, 96.
Dimpe, K. M., and Nomngongo, P. N. (2019). Application of Activated Carbon-Decorated Polyacrylonitrile Nanofibers as an Adsorbent in Dispersive Solid-Phase Extraction of Fluoroquinolones From Wastewater. J. Pharm. Anal. 9, 117–126. doi:10.1016/j.jpha.2019.01.003
Ferraro, A., Marino, E., Trancone, G., Race, M., Mali, M., Pontoni, L., et al. (2023). Assessment of Environmental Parameters Effect on Potentially Toxic Elements Mobility in Foreshore Sediments to Support Marine-Coastal Contamination Prediction. Mar. Pollut. Bull. 194, 115338. doi:10.1016/j.marpolbul.2023.115338
Ghirardini, A., Grillini, V., and Verlicchi, P. (2020). A Review of the Occurrence of Selected Micropollutants and Microorganisms in Different Raw and Treated Manure – Environmental Risk Due to Antibiotics After Application to Soil. Sci. Total Environ. 707, 136118. doi:10.1016/j.scitotenv.2019.136118
Jalil, M. E. R., Baschini, M., and Sapag, K. (2015). Influence of pH and Antibiotic Solubility on the Removal of Ciprofloxacin From Aqueous Media Using Montmorillonite. Appl. Clay Sci. 114, 69–76. doi:10.1016/j.clay.2015.05.010
Jodeh, S., Jaber, A., Hanbali, G., Massad, Y., Safi, Z. S., Radi, S., et al. (2022). Experimental and Theoretical Study for Removal of Trimethoprim From Wastewater Using Organically Modified Silica With Pyrazole-3-Carbaldehyde Bridged to Copper Ions. BMC Chem. 16, 17. doi:10.1186/s13065-022-00814-0
Kashyap, A., Nashil, B., and Thatikonda, S. (2023). Experimental and Numerical Elucidation of the Fate and Transport of Antibiotics in Aquatic Environment: A Review. Environ. Monit. Assess. 195, 942. doi:10.1007/s10661-023-11482-5
Khan, S., Naushad, M., Govarthanan, M., Iqbal, J., and Alfadul, S. M. (2022). Emerging Contaminants of High Concern for the Environment: Current Trends and Future Research. Environ. Res. 207, 112609. doi:10.1016/j.envres.2021.112609
Kocárek, M., Kodesová, R., Vondrácková, L., Golovko, O., Fér, M., Klement, A., et al. (2016). Simultaneous Sorption of Four Ionizable Pharmaceuticals in Different Horizons of Three Soil Types. Environ. Pollut. 218, 563–573. doi:10.1016/j.envpol.2016.07.039
Li, H., Zhang, D., Han, X., and Xing, B. (2014). Adsorption of Antibiotic Ciprofloxacin on Carbon Nanotubes: PH Dependence and Thermodynamics. Chemosphere 95, 150–155. doi:10.1016/j.chemosphere.2013.08.053
Li, J., and Zhang, H. (2017). Factors Influencing Adsorption and Desorption of Trimethoprim on Marine Sediments: Mechanisms and Kinetics. Environ. Sci. Pollut. Res. 24, 21929–21937. doi:10.1007/s11356-017-9693-y
Liu, X., Luo, Y., Zhang, H., Wu, J., Zhu, R., and Wang, H. (2022). Spatial Heterogeneity of Particulate Organic Matter for the Sorption of Ciprofloxacin at the Microstructure Scale. Sci. Total Environ. 847, 157326. doi:10.1016/j.scitotenv.2022.157326
Lu, X.-M., Lu, L.-B., Lin, Y.-H., Chen, Z.-Y., and Chen, J.-H. (2023). Exploring the Interaction Between Agronomic Practices and Soil Characteristics on the Presence of Antibiotic Resistance Genes in Soil. Appl. Soil Ecol. 187, 104837. doi:10.1016/j.apsoil.2023.104837
Mejías, C., Santos, J. L., Martín, J., Aparicio, I., and Alonso, E. (2023). Thermodynamic and Kinetic Investigation of the Adsorption and Desorption of Trimethoprim and its Main Metabolites in Mediterranean Crop Soils. Molecules 28, 437. doi:10.3390/molecules28010437
Mpatani, F. M., Aryee, A. A., Kani, A. N., Han, R., Li, Z., Dovi, E., et al. (2021). A Review of Treatment Techniques Applied for Selective Removal of Emerging Pollutant-Trimethoprim From Aqueous Systems. J. Clean. Prod. 308, 127359. doi:10.1016/j.jclepro.2021.127359
Ncibi, M. C., and Sillanpää, M. (2015). Optimized Removal of Antibiotic Drugs From Aqueous Solutions Using Single, Double and Multi-Walled Carbon Nanotubes. J. Hazard Mater 298, 102–110. doi:10.1016/j.jhazmat.2015.05.025
Pasket, A., Zhang, H., Wang, Y., Krzmarzick, M. J., Gustafson, J. E., and Deng, S. (2022). Clay Content Played a Key Role Governing Sorption of Ciprofloxacin in Soil. Front. Soil Sci. 2, 814924. doi:10.3389/fsoil.2022.814924
Pérez-Novo, C., Bermúdez-Couso, A., López-Periago, E., Fernández-Calviño, D., and Arias-Estévez, M. (2009). The Effect of Phosphate on the Sorption of Copper by Acid Soils. Geoderma 150, 166–170. doi:10.1016/j.geoderma.2009.02.001
Rodríguez-López, L., Cela-Dablanca, R., Núñez-Delgado, A., Álvarez-Rodríguez, E., Fernández-Calviño, D., and Arias-Estévez, M. (2021). Photodegradation of Ciprofloxacin, Clarithromycin and Trimethoprim: Influence of pH and Humic Acids. Molecules 26, 3080. doi:10.3390/molecules.26113080
Rodríguez-López, L., Santás-Miguel, V., Núñez-Delgado, A., Álvarez-Rodríguez, E., Pérez-Rodríguez, P., and Arias-Estévez, M. (2022a). Influence of pH, Humic Acids, and Salts on the Dissipation of Amoxicillin and Azithromycin Under Simulated Sunlight. Span. J. Soil Sci. 12, 10438. doi:10.3389/sjss.2022.10438
Rodríguez-López, L., Santás-Miguel, V., Cela-Dablanca, R., Núñez-Delgado, A., Álvarez-Rodríguez, E., Pérez-Rodríguez, P., et al. (2022b). Ciprofloxacin and Trimethoprim Adsorption/Desorption in Agricultural Soils. Int. J. Environ. Res. Public Health 19, 8426. doi:10.3390/ijerph19148426
Sagaseta de Ilurdoz, M., Sadhwani, J. J., and Reboso, J. V. (2022). Antibiotic Removal Processes From Water and Wastewater for the Protection of the Aquatic Environment - a Review. J. Water Process Eng. 45, 102474. doi:10.1016/j.jwpe.2021.102474
Tran, N. H., Reinhard, M., and Gin, K. Y.-H. (2018). Occurrence and Fate of Emerging Contaminants in Municipal Wastewater Treatment Plants From Different Geographical Regions-A Review. Water Res. 133, 182–207. doi:10.1016/j.watres.2017.12.029
Vasudevan, D., Bruland, G. L., Torrance, B. S., Upchurch, V. G., and MacKay, A. A. (2009). pH-Dependent Ciprofloxacin Sorption to Soils: Interaction Mechanisms and Soil Factors Influencing Sorption. Geoderma 151, 68–76. doi:10.1016/j.geoderma.2009.03.007
Xiang, Y., Xu, Z., Wei, Y., Zhou, Y., Yang, X., Yang, Y., et al. (2019). Carbon-Based Materials as Adsorbent for Antibiotics Removal: Mechanisms and Influencing Factors. J. Environ. Manage 237, 128–138. doi:10.1016/j.jenvman.2019.02.068
Keywords: adsorption, emerging contaminants, sorption mechanisms, soil properties, speciation
Citation: Rodríguez-López L, Santás-Miguel V, Cela-Dablanca R, Núñez-Delgado A, Álvarez-Rodríguez E, Rodríguez-Seijo A and Arias-Estévez M (2024) Sorption of Antibiotics in Agricultural Soils as a Function of pH. Span. J. Soil Sci. 14:12402. doi: 10.3389/sjss.2024.12402
Received: 10 November 2023; Accepted: 29 February 2024;
Published: 12 March 2024.
Edited by:
Marco Race, University of Cassino, ItalyCopyright © 2024 Rodríguez-López, Santás-Miguel, Cela-Dablanca, Núñez-Delgado, Álvarez-Rodríguez, Rodríguez-Seijo and Arias-Estévez. This is an open-access article distributed under the terms of the Creative Commons Attribution License (CC BY). The use, distribution or reproduction in other forums is permitted, provided the original author(s) and the copyright owner(s) are credited and that the original publication in this journal is cited, in accordance with accepted academic practice. No use, distribution or reproduction is permitted which does not comply with these terms.
*Correspondence: Lucía Rodríguez-López, lucia.rodriguez.lopez@uvigo.gal