- 1Division of Nephrology, University Hospital Zurich, Zurich, Switzerland
- 2Department of Immunology, University Hospital Zurich, Zurich, Switzerland
Kidney transplant recipients (KTRs) with ultralong-term survival represent a growing, yet insufficiently studied patient cohort. In this single-center retrospective study, we analyzed 248 ultralong-term survivors (≥20 years). KTRs were classified into those with superior graft function (defined as eGFR ≥45 ml/min + proteinuria ≤300 mg/day + eGFR-slope ≤ 2 ml/min/1.73 m2/year) and inferior graft function regarding the risk of CKD progression. 20 years post-transplant, median eGFR was 54 ml/min (11–114), proteinuria 200 mg/24 h (0–7,620), eGFR decline 0.45 ml/min/1.73 m2/year (11.7 6.5) and DSA had been detected in 19.7% of KTRs. We identified 96 KTRs (38.7%) with superior (group 1) and 152 KTRs (61.3%) with inferior graft function (group 2). Donation after cardiac death, female sex, glomerulonephritis as primary disease, and early TCMR were independently associated with inferior graft function. Graft survival was significantly better in group 1 compared to group 2 (LogRank, p < 0.001). Besides group affiliation (HR 20.515, p = 0.003), multivariable analysis identified DSA development (HR 3.081, p = 0.023) and donor age (HR 1.032, p = 0.024) as independent factors. Interestingly, there was no significant difference in patient survival (LogRank, p = 0.350). In ultralong-term survivors, excellent graft function refers to superior graft survival but does not extend ultimate patient survival. DSA-formation should be taken seriously even in the ultralong-term.
Introduction
Kidney transplantation has become standard procedure in care of patients with end stage kidney disease (ESKD) and by today, is the preferred treatment for most of them (1). Over the past decades, short- and long-term graft survival have improved remarkably (2,3,4,5). For Europe, the Collaborative Transplant Study (CTS) reports an estimated 20-year death-uncensored graft survival rate of 41% for first deceased donor kidney transplant recipients (KTRs) from 1990–2020 and 16.8 years death-uncensored graft half-life (6). According to Coemans et al., who performed a comprehensive analysis of CTS data, death-censored 20-year graft survival rate even exceeded 50% for the transplant decade 1996–2005 (2). However, the authors reported survival data beyond 20 years to be sparse (2). The latest registry report from Australia and New Zealand (ANZDATA) reveals 30% 20-year death-uncensored graft survival for first deceased donor KTRs (3). Other comprehensive registry reports limit their analysis to a maximum of 10-year death-uncensored graft survival (for deceased donors 49.5% in the US (4), 58.5% in Canada (5).
Hence there is a growing population of KTRs who have lived with a functioning graft for several decades (7,8,9,10,11,12,13,14,15). Considering this development, surprisingly little attention has been given to the study of ultralong-term survivors (ULS) (7,8,9,10,11,12,13,14,15). Knowledge about their clinical characteristics, graft function, and alloimmunization is extremely limited and outcome as well as causes of graft losses in ULS have rarely been reported (7,8,9,10,11,12,13,14,15).
To optimize ultralong-term aftercare and to overcome the still important challenge of further improving long-term outcome (16), it is crucial to learn more about this particular patient group (9). To address these needs, we studied a large cohort of KTRs who have lived with a functioning graft for ≥20 years and aimed to investigate the following questions:
(1) What graft function (estimated glomerular filtration rate (eGFR), proteinuria, eGFR decline) do KTRs display 20 years post-transplant?
(2) What factors influence graft function 20 years post-transplant?
(3) What is the incidence of donor specific antibody (DSA)-formation in ULS?
(4) What is the outcome regarding graft and patient survival beyond 20 years post-transplant?
(5) What factors influence ultimate graft and patient survival of ULS?
Methods
KTRs and Data Collection
This single-center retrospective study was approved by the local Ethics committee of Zurich, Switzerland (Basec Number: 2019–02082) without informed consent requirement and performed in adherence to the declaration of Helsinki.
We considered all adult (age ≥16 years at the date of transplantation) KTRs transplanted at University Hospital Zurich between 1 January 1981 and 31 December 1999. Among a total of 1,180 single-kidney transplantations performed at our institution during this era, we identified 304 KTRs with documented graft survival ≥20 years. 22 KTRs who had denied consent had to be excluded, further 34 KTRs due to insufficient data. This led to a total study cohort of 248 KTRs (Figure 1).
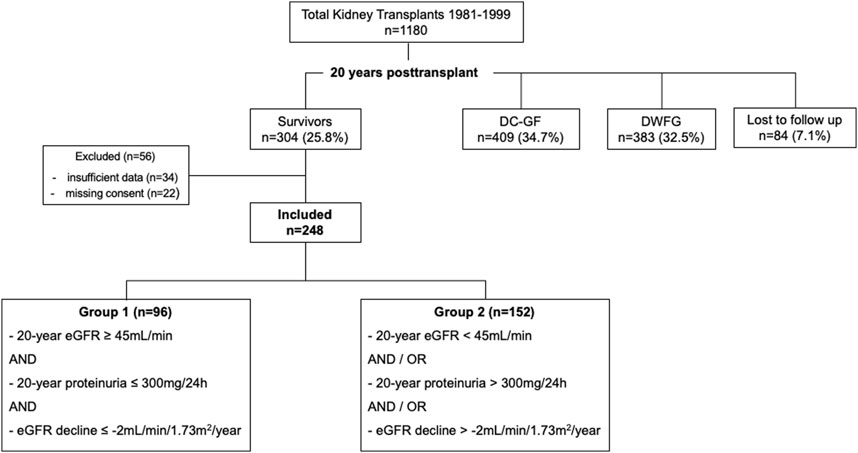
FIGURE 1. Flow chart all kidney transplants 1981–1999. DC-GF, death-censored graft failure. DWFG, death with functioning graft. Grouping criteria: 20-year eGFR: BSA-deindexed CKD-EPI at the 20-year post-transplant visit, 20-year proteinuria: Protein-to-creatinine ratio (mg/mmol) multiplied by 10 at the 20-year post-transplant visit. eGFR decline: eGFR CKD-EPI slope 15–20 years post-transplant.
At our center, follow-up care after the first post-transplant year is generally performed quarterly in our outpatient clinic or, in stable conditions, by local nephrologists, complemented by an annual visit at our center. For data collection, we reviewed medical records from the electronic database of the hospital registry. End of follow-up and data collection was 31 January 2021. To evaluate characteristics 20 years post-transplant, we identified the 20-year post-transplant visit for each KTR, defined as the closest and most complete visit to the date of transplantation plus 20 years. For all KTRs, median time from transplantation to the 20-year post-transplant visit was 240 months (range 228–248 months). If the 20-year post-transplant visit did not reveal full data, we checked medical records from 19–21 years post-transplant for completion. Cases with insufficient documentation between 19–21 years post-transplant were excluded, as stated above.
Graft Function
From serum-creatinine at the 1-year and 20-year post-transplant visit, we calculated baseline 1-year and 20-year eGFR, using the following formulas: Modification of Diet in Renal Disease (MDRD) (17), Chronic Kidney Disease Epidemiology Collaboration (CKD-EPI) (18), and Cockcroft-Gault (19). We did not include the race coefficient for MDRD and CKD-EPI (20). For MDRD and CKD-EPI, we additionally calculated body surface area (BSA)-deindexed eGFR, multiplying eGFR by KTR’s individual BSA (21), divided by 1.73 m2 (22,23). To indicate stability or decline of graft function, respectively, eGFR (CKD-EPI) slopes (24) were calculated for the last 5 years of the 20-year period, i.e., 15–20 years post-transplant. Baseline 20-year proteinuria was assessed by multiplying urine-to-creatinine ratio (PCR) (mg/mmol) from spot urine at the 20-year post-transplant visit by 10 (25). For 31 KTRs (12.5%), PCR was calculated from 24-hour collection urine, as before 2005, measurement of proteinuria was obtained by 24-hour collection urine at our center. PCR below detection limit was included in the analysis with a value of zero.
Maintenance Immunosuppression and DSA-Screening
All donors and recipients were typed for human leukocyte antigen (HLA)-A, -B and -DR. Since approximately 2009, annual HLA antibody-monitoring using Luminex based assay (One Lambda, Canoga Park, CA, United States) became standard procedure in KTR-care at our center. In case of worsening graft function or progression of proteinuria, screening may have been performed more often. If Luminex mix assay was positive and/or clinical suspicion was high, an additional Luminex single antigen bead assay was performed to test for DSA. We classified KTRs with ≥1 DSA-positive Luminex single antigen bead assay up to 21 years post-transplant as DSA-positive, irrespective of the level of mean fluorescence intensity (MFI). KTRs were classified as DSA-negative in case HLA antibody-monitoring (Luminex mix assay only or both, Luminex mix and single bead assay) did not show DSA up to 21 years post-transplant or if the very first screening was performed beyond 21 years post-transplant and negative for DSA. KTRs were excluded from this sub-analysis in case of missing HLA antibody-screening during the observation period (n = 36, 14.5%) or if the very first screening was performed beyond 21 years post-transplant and DSA-positive, thus the date of DSA-occurrence was indeterminable (n = 14, 5.6%).
Group Categorization
According to the KDIGO 2012 Clinical Practice Guideline for the risk of CKD progression, KTRs were stratified into two groups based on graft function 20 years post-transplant. (50) Criteria for superior graft function (Group 1) were: 1) 20-year eGFR ≥45 ml/min (BSA-deindexed CKD-EPI), 2) 20-year proteinuria ≤300 mg/24 h, and 3) eGFR (CKD-EPI) decline ≤-2 ml/min/1.73 m2/year 15–20 years post-transplant. Subjects in group 1 had to meet all 3 criteria. KTRs who did not pass ≥1 criteria were assigned to group 2. Two cases with missing data on proteinuria that fulfilled the other criteria for group 1 were classified as insufficient data and excluded, consequently. Two cases were categorized according to BSA-indexed CKD-EPI, because of unknown BSA (missing documentation of patient’s height).
Survival
We separately studied patient survival (treating graft loss as a censored event), death-uncensored and death-censored (treating death as a censored event) graft survival, calculated from the date of transplantation to KTR’s death or graft loss (return to permanent dialysis or re-transplantation), whatever came first. If there was no event, survival dates were censored at the date of last follow-up or end of data collection (31 January 2021).
Specific causes of graft loss and results of indication biopsy were evaluated for KTRs with death-censored graft loss in group 1.
Calculation of Predicted Indirectly ReCognizable HLA-Epitopes Scores
The HLA-derived mismatched peptide epitopes presented by KTRs HLA-molecules were calculated using the PIRCHE-II algorithm. Presentation of both HLA class I (HLA-A, B) and HLA class II derived peptides (HLA-DR, DQ) were calculated for each HLA locus. Detection of HLA antigens was performed by DNA-based HLA-typing technology using blood samples. Either sequence-specific oligonucleotide (SSO) or sequence-specific primer (SSP) technologies were used to generate low-resolution HLA typing results. The imputation of probable allele resolution results needed for the PIRCHE-II calculation was achieved by the use of the imputation algorithm included in the PIRCHE-II calculation. The PIRCHE-II algorithm is available online (https://www.PIRCHE-II.org).
Statistical Analysis
Statistical analysis was performed using SPSS (Version 26, IBM, Armonk, NY, United States). Continuous variables are expressed as median (range minimum-maximum) and compared using Mann Whitney-U Test. Categorical data are expressed as number (%) and compared using Chi (2) test, corrected for Yates in 2x2 tables. If expected cell count was ≤5, we used Fisher’s Exact test instead. Missing values were not imputed. Survival was analysed using the Kaplan-Meier method and compared with LogRank test. Univariable and multivariable Cox proportional hazards models with enter method were used to investigate factors associated with survival. Variables with a p-value ≤0.05 in the univariable analysis were included in the multivariable model. For categorical variables in the multivariable model, assumption of proportional hazards was assessed visually by Kaplan Meier curves (26). For all tests, statistical significance was assumed for a two-tailed p-value <0.05.
Results
Table 1 shows basic characteristics, Table 2 post-transplant complications and 1-year graft function, and Table 3 multivariable Cox regression analysis for group categorization of the 248 KTRs included in this study. Median KTR-age at the date of transplantation was 39.9 years, 92/248 (37.1%) KTRs were female.
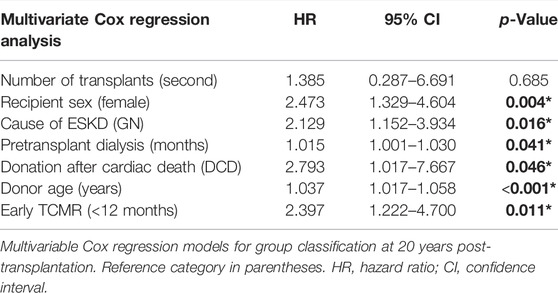
TABLE 3. Cox Regression analysis to assess group classification of KTRs 20 years post-transplantation.
Graft Function
Table 4 shows detailed information on graft function 20 years post-transplant. Median serum-creatinine was 124 μmol/L, median eGFR 54 ml/min (BSA-deindexed CKD-EPI), median proteinuria 200 mg/24 h, and median eGFR decline −0.45 ml/min/1.73 m2/year. CKD-related laboratory findings are shown in the Supplementary Table S1.
Immunosuppression and DSA-formation
Maintenance immunosuppression is shown in Table 5, results of HLA antibody-screenings in Table 6. Within the first two post-transplant decades, 39/198 (19.7%) KTRs had developed ≥1 DSA, predominantly (29/39, 74.4%) against HLA Class II. Total PIRCHE-II scores (median 42.61 (range: 10.00–111.63) vs. median 33.46 (range: 0.00–99.22)) and PIRCHE-II scores for HLA-class II (HLA-DR; median 11.27 (range: 00.00–58.58) vs. median 4.29 (range: 0.00–28.70)) were significantly higher among KTRs developing DSA compared to KTRs not developing DSA (p = 0.021, p = 0.020). No differences were observed for PIRCHE-II scores for HLA-class I (HLA-A, -B; median 29.69 (range: 2.07–85.74) vs. median 23.26 (range: 0.00–76.93) between KTRs developing and not developing DSA (p = 0.116). Group 1 and group 2 did neither significantly differ in amount of DSA-positive KTRs nor in number, category, or maximal MFI of detected DSA (all p > 0.05). No differences were observed for the total PIRCHE-II scores (A, B, DR) and the PIRCHE-II scores per locus between group 1 and group 2 (p > 0.05).
KTR-Categorization and Group Comparison
Subdivision of the cohort is shown in Figure 1. 96/248 (38.7%) KTRs fulfilled the criteria for superior graft function (group 1). The remaining 152/248 (61.3%) KTRs were classified to group 2. Figure 2 displays distribution of all KTRs according to baseline 20-year eGFR and proteinuria, group subdivision is marked by color.
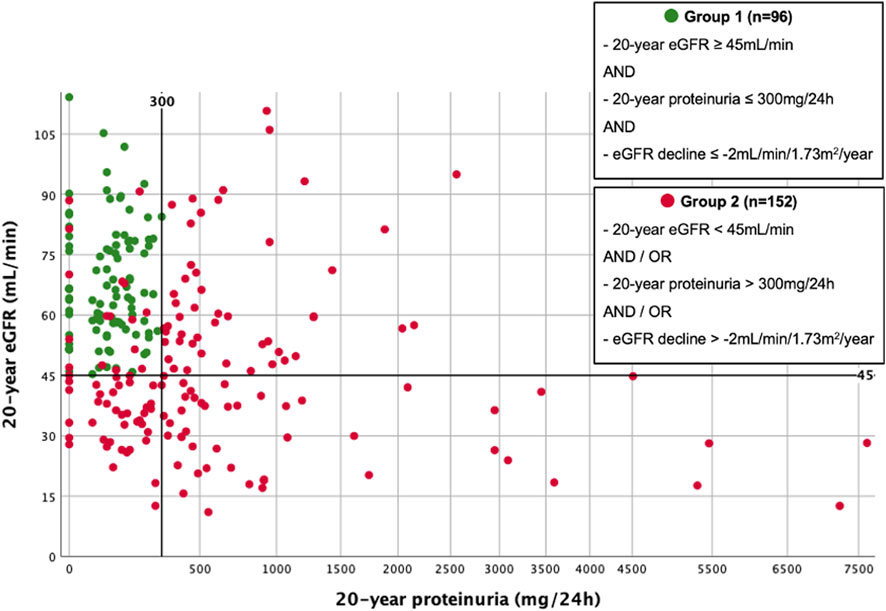
FIGURE 2. Scatterplot illustrating group subdivision. Scatterplot of all 20-year survivors, according to 20-year eGFR (BSA-deindexed CKD-EPI) and 20-year proteinuria. Group subdivision is marked by color.
Multivariable Cox regression analysis is shown in Table 3. The strongest impact on group affiliation was observed for donation after cardiac death (DCD; HR 2.793, 95% CI 1.017–7.667, p = 0.041), female sex (HR 2.473, 95% CI 1.329–4.604, p = 0.004), early TCMR (HR 2.397, 95% CI 1.222–4.700, p = 0.011), and glomerulonephritis as primary disease (HR 2.129, 95% CI 1.152–3.934, p = 0.016). While 17 of 152 KTRs (11.2%) of group 2 developed recurrence of primary disease, only 1 of 96 KTRs (1.0%) of group 1 did (p < 0.001). A minor impact was observed for donor age (HR 1.037, 95% CI 1.017–1.058, p < 0.001) and length of pretransplant dialysis (HR 1.015, 95% CI 1.001–1.030, p = 0.041).
Survival
Survival analyses are shown in Figures 3A–C, 4A–C. 93/248 (37.5%) graft losses were recorded during follow-up: 53/248 (21.4%) KTRs died with a functioning graft (death with functioning graft, DWFG), 40/248 (16.1%) KTRs lost their graft while still alive (death-censored graft failure, DC-GF). Median death-uncensored graft survival was 29.9 years (95% Confidence Interval (CI) 28.4–31.4 years). For death-censored graft survival and for patient survival Kaplan Meier curves did not reach 50%.
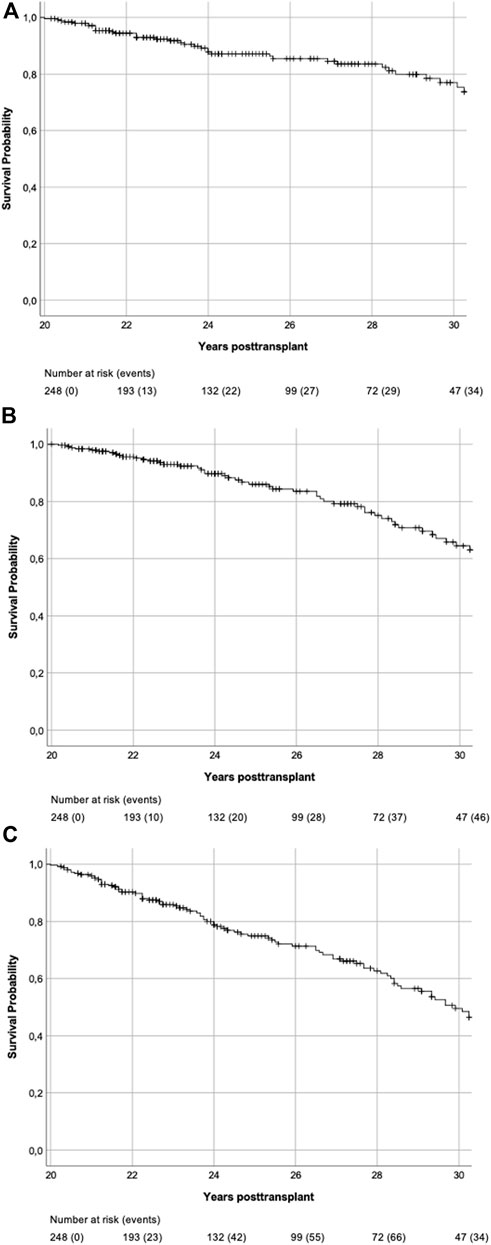
FIGURE 3. (A) Death-censored graft survival. Kaplan-Meier Plot of death-censored graft survival of all 20-year survivors. (B) Patient survival. Kaplan-Meier Plot of patient survival of all 20-year survivors. (C) Death-uncensored graft survival. Kaplan-Meier Plot of death-uncensored graft survival of all 20-year survivors. Median death-uncensored graft survival was 29.9 years (95% CI 28.4–31.4 years).
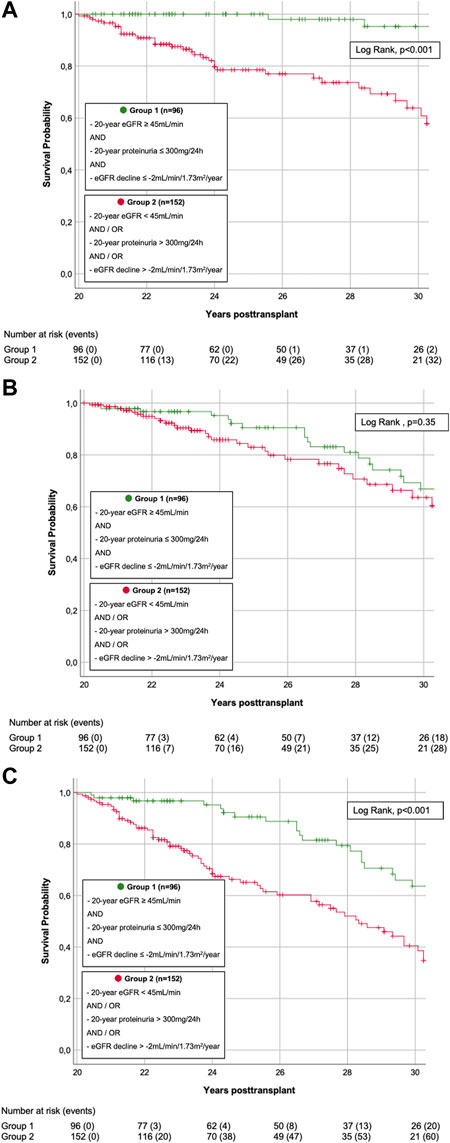
FIGURE 4. (A) Death-censored graft survival. Kaplan-Meier Plot of death-censored graft survival. Death-censored graft survival was significantly superior in KTRs s with superior graft function (group 1) compared to KTRs with inferior graft function (group 2) (LogRank, p < 0.001). (B) Patient survival. Kaplan-Meier Plot of patient survival. Patient survival did not significantly differ in KTRs with superior graft function (group 1) compared to those with inferior graft function (group 2) (LogRank, p = 0.350). (C) Death-uncensored graft survival. Kaplan-Meier Plot of death-uncensored graft survival. Death-uncensored graft survival was significantly superior in KTRs s with superior graft function (group 1) compared to KTRs with inferior graft function (group 2) (LogRank, p < 0.001).
In group 1, 26/96 (27.1%) grafts failed during follow-up: 23/26 (88.5%) due to DWFG, 3/26 (11.5%) due to DC-GF. These latter 3 KTRs were analyzed more closely: 1 KTR was DSA-negative 20 years post-transplant but developed de novo DSA (dnDSA) during the third post-transplant decade. Graft loss resulted from biopsy-proven chronic antibody mediated rejection (ABMR). The other two KTRs both had their first HLA antibody-screening performed during the 28th year post-transplant and were DSA-positive by then (which, due to indeterminable date of DSA-development, led to exclusion from DSA-sub-analysis, as stated above). Indication biopsy showed glomerulopathy and low level glomerulitis in one, and glomerulopathy and vasculopathy with signs of de novo IgA nephropathy in the other case. In group 2, 67/152 (44.1%) grafts failed during follow-up: 30/67 (44.8%) due to DWFG, 37/67 (55.2%) due to DC-GF. Death-censored and death-uncensored graft survival was significantly superior in group 1 (LogRank, both p < 0.001, Figures 4A,C). In contrast, there was no significant difference in patient survival (LogRank, p = 0.35, Figure 4B).
Univariable and multivariable Cox regression analysis are shown in Tables 7,8. For DC-GF (Table 7), we found a significant impact of group affiliation (HR 20.515, 95%CI 2.730–154.143, p = 0.003), overall DSA-development (HR 3.081, 95% CI 1.165–8.146, p = 0.023), donor age (HR 1.032, 95% CI 1.004–1.061, p = 0.024). For patient survival (Table 8), only KTR-age (HR 1.082, 95% CI 1.051–1.113, p < 0.001) and CsA-based immunosuppression (HR 0.297, 95% CI 0.149–0.593, p < 0.001) were significantly associated with outcome.
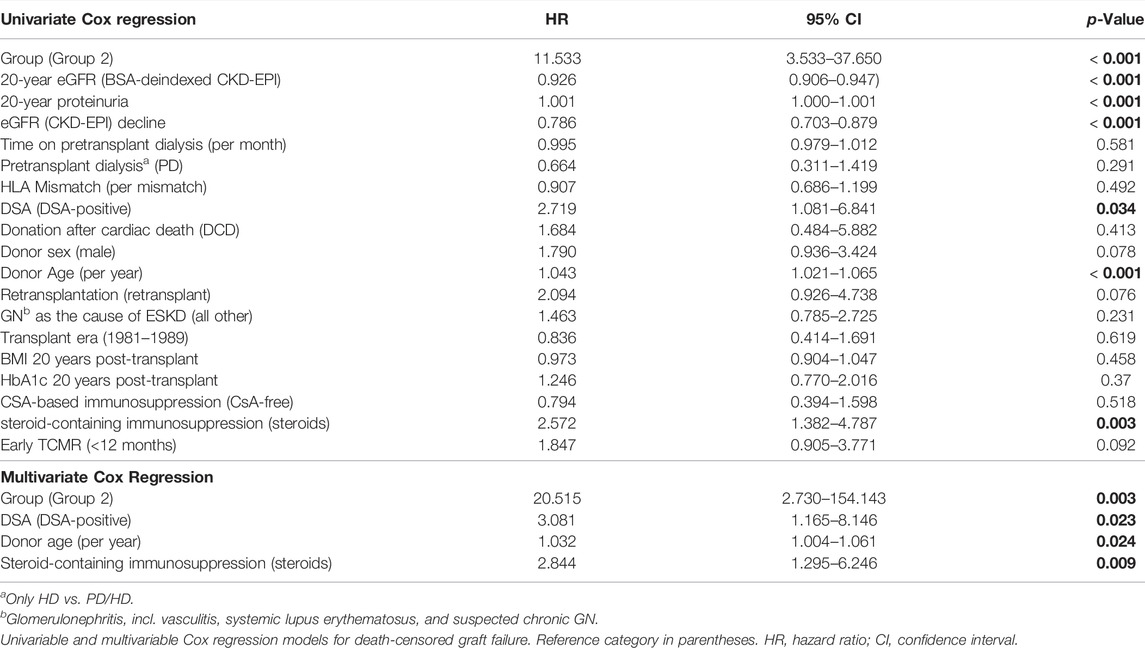
TABLE 7. Cox Regression analysis to assess the risk of kidney allograft loss in KTRs 20 years post-transplantation.
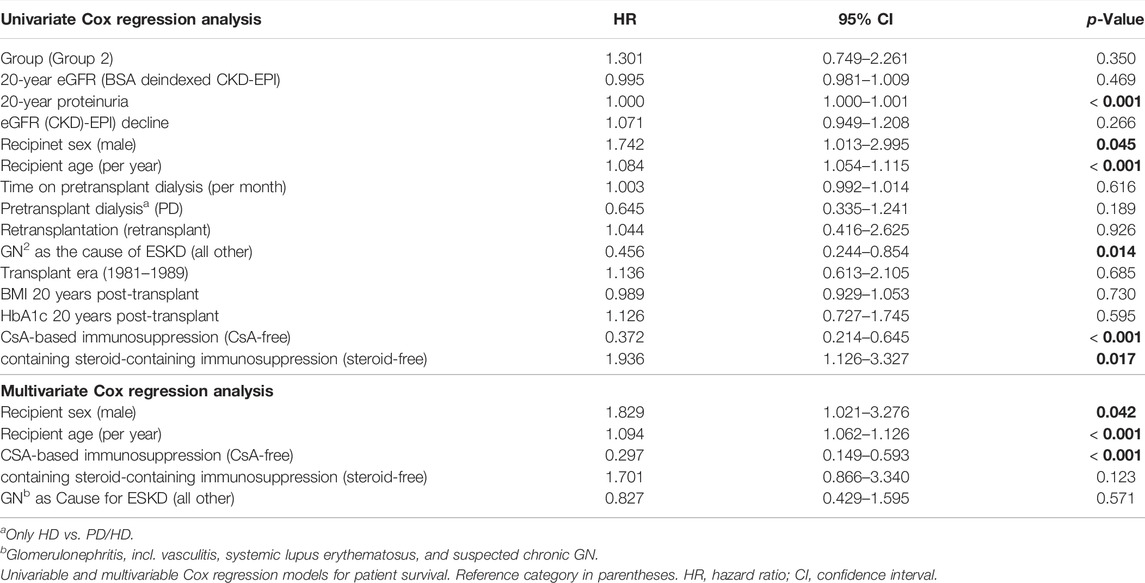
TABLE 8. Cox Regression analysis to assess the mortality risk of KTRs 20 years post-transplantation.
Discussion
ULS represent a growing, yet insufficiently studied patient population (8,9). To address this new challenge in transplant long-term aftercare (7,9), we herein analyzed 248 KTRs with a functioning graft ≥20 years. In line with earlier ULS-reports (7,8,9,11,12,14,15) graft function was remarkably good: 20 years post-transplant, the majority (64.5%) of the KTRs was in stage 1-3a of CKD classification (25). 38.7% fulfilled the criteria for superior graft function, i.e., had high and stable 20-year eGFR and low proteinuria (group 1).
Group comparison revealed a significant difference in DCD, early TCMR, recipient gender, and glomerulonephritis as primary disease. For the first, previous studies suggest comparable survival rates for kidneys from DCD. (27) For the second, although in general associated with reduced graft survival, the impact of successfully treated early TCMR on ultralong-term survival has not been well studied. (51) However, our data suggest that initial acute kidney injury and associated nephron loss either due to DCD or early TCMR may have an impact in the ultralong-term, and predispose these KTRs to decreased graft function and proteinuria through chronic hyperfiltration and chronic histologic lesions of interstitial fibrosis/tubular atrophy. Regarding the effect of KTR-gender on ultralong-term survival, results are conflicting (8,9,10,13). However, the predominance of men in group 1 surprises, given their higher risk of chronic graft failure (28). Our finding could result from potential underestimation of GFR in women by the applied equations and stresses the need for further, gender-specific studies (29). Although the time of onset and severity of recurrence of the underlying disease vary widely, our data suggest that glomerulonephritis recurrence strongly influences the risk of impaired renal function in the ultralong-term. However, in our analysis, no factor had an independent impact on further survival.
Data on long-term maintenance immunosuppression is extremely limited (30). In our center, standard immunosuppression during the respected period was composed of Ciclosporin, Azathioprine and Corticosteroids. 20 years post-transplant, 71% of the ULS were still under Ciclosporin-based maintenance therapy (no significant group difference). However, group 2 contained significantly more KTRs with Tacrolimus-based immunosuppression. Changes in immunosuppressive therapy over time could not be analyzed in this study, but we presume that this difference results from conversion from Ciclosporin to Tacrolimus in response to supposed immune-related injury and the development of DSA by intensifying maintenance immunosuppression (30). The deleterious effect of calcineurin inhibitors (CNI) on long-term graft outcome has become an increasing matter of debate (31,32). Regarding ULS, data is scarce and inconsistent: Bererhi et al. only found 3% of ULS with CNI-based maintenance immunosuppression and therefore hypothesized that avoiding CNI could favor ultralong-term graft survival (7). In contrast, Traynor et al. reported 40% and Kettler et al. even 68% of ULS with CNI-based therapy (8,12). Given the prolonged exposure to immunosuppression, determining optimal long-term immunosuppression is especially important for ULS (9). But this urgent question still remains unanswered (33).
To target therapeutic interventions and optimize ultralong-term aftercare, we need to improve our understanding of late graft loss (16,31), which includes patient’s death (DWFG) and loss of graft function while still alive (DC-GF) (16). In this study, we drew a detailed picture of graft and patient survival of 248 ULS. While overall graft survival was already remarkably good (median death-uncensored graft survival 29.9 years), for KTRs with superior graft function, it was outstanding. In fact, group 1 only involved 3 events of DC-GF. In contrast, graft survival in group 2 was clearly inferior. Corresponding with the fact that graft failure is preceded by graft dysfunction (16,34), 92.5% of all events of DC-GF in this study occurred in group 2.
In their comprehensive study of 177 ULS, McCaughan et al. observed that DC-GF after 20 years is uncommon (9). Our study shows that this is particularly true for ULS with preserved graft function, while in group 2, DC-GF accounted for the majority (55.2%) of graft losses. A multivariable Cox regression model for DC-GF confirmed a strong influence of group affiliation.
Donor age profoundly impacts graft quality (35) and is an important risk factor in graft outcome (2,35). In this study, comparable to earlier ULS-reports (7,8,9,10,12,13), donors were young (median 32 years), a clear difference to more recently transplanted KTRs (2). Very interestingly, donors were significantly younger in group 1, and donor age had significant impact on DC-GF beyond 20 years post-transplant. This phenomenon might be attributed to the loss of functional nephrons with aging and consecutive decreased functional reserve (36) and increased vulnerability to transplant-related injury (37,38). In previous studies, univariable analyses revealed significant association of donor age with ultralong-term survival, however, in multivariable models the effect showed only a trend and missed statistical significance (9,10).
Late DC-GF is profoundly driven by alloimmune mechanisms (31,39,51). DSA are associated with increased risk of late graft failure (40,41) and provide a well-established biomarker predicting ABMR and graft loss (40,42). However, little is known about the role of DSA in the context of ultralong-term survival (12,43). Analysis of DSA-screenings revealed several interesting findings: First, in our cohort of ULS only, cumulative incidence of DSA-formation during the first two post-transplant decades was 19.7% and thus within the range reported from general KTR-population (42,44). Secondly, we could not find any significant group difference in cumulative incidence of DSA-positive KTRs, duration to first DSA-detection, HLA-class, HLA-mismatches, PIRCHE-II socres, MFI, and number of detected DSA. These results surprise, as KTRs who develop dnDSA have been shown to have higher rates of eGFR decline (41). Thirdly, however, DSA-formation was identified as an independent risk factor for DC-GF. The association of steroid use with DC-GF must be interpreted here with the restart of steroids after the onset of DSA. In our cohort, DSA were detected surprisingly late (median 211 months post-transplant), a finding probably biased by transplant era and available techniques. However, it is known that DSA-formation can appear anytime, even several years post-transplant (42,43,44) and that time from dnDSA-onset to graft dysfunction ranges from months to years (44). So nevertheless, it is suggestive that ultralong-term survival of our cohort was favored by substantially late DSA-development and that their deleterious impact on graft survival manifested not until the third post-transplant decade. Given the close relationship between dnDSA, ABMR and ultimate graft loss, this result points towards a potential target of intervention in order to further improve long-term graft survival (45). However, further studies are needed to address this question (45).
Hence, DC-GF is predominantly seen in ULS with inferior graft function. However, despite the known link of declining graft function with increased mortality (46), there was no significant group difference in patient survival. The risk of DWFG increases with time since transplantation (47) and in ULS, it represents the leading cause of graft loss (8,9,14,15). Our results correspond with the findings from Gaston et al. who stated that mortality risk is largely independent of graft function (16). Beyond 20 years post-transplant, leading causes of death are cardiovascular disease and malignancy (8,9), both highly prevalent in ULS (7,8,9,14,15). For example, McCaughan et al. reported cancer in 37% and cardiovascular disease in 27% of 20-year survivors and therefore stated that, in management of ULS, focus should shift on prevention and optimal therapy of these comorbidities (9).
Our results allow us to specify this statement and lead to further clinical implications. Indeed, in case of good, stable graft function up to 20 years post-transplant, risk of ultimate DC-GF is very low, and focus should be on controlling the medical comorbidities (9). In contrast, in KTRs with inferior ultralong-term graft function, risk of DC-GF may not be neglected, and aftercare should equally concentrate on preventing ultimate loss of graft function. Given that DSA and ABMR, respectively, are potentially treatable conditions (45). Our findings argue for continuing DSA-monitoring even in the setting of ultralong-term survival. Additionally, KTRs with inferior ultralong-term graft function might be considered for biopsy, not only to evaluate immune injury, but also to detect evidence of CNI-toxicity and to adjust immunosuppression, accordingly (48,49).
Our study has several limitations. First, it is a retrospective and single-center analysis with the intrinsic limitations and potential biases. Secondly, our cohort differs in several aspects from more recently transplanted KTRs. Thirdly, DSA-subanalysis is limited by transplant era and available techniques: it affected time of first and frequency of subsequent screenings, 50 cases with missing or insufficient data had to be excluded, and KTRs were mostly not typed for HLA-DP and -DQ.
However, most of these limitations are inevitably associated with the retrospective design of research on ULS and the according necessity of lengthy follow-up (8). In addition, our study cohort is not dominated by living donor transplantations, which would suggest better organ quality and possibly better HLA matching, so our results translate well to the general transplant cohort. The reason for this is that only about 50 living donations were performed in the observation period from 1981 to 1999 at our center. Our study provides an important contribution in improving understanding of this unique, increasingly important patient population (7,8,9). Comprehensive follow-up enables us to give extensive overview of ultralong-term graft function, alloimmunization, and ultimate outcome beyond 20 years post-transplant and to identify corresponding risk factors and potential therapeutic targets required to improve ULS-aftercare.
Conclusion
Overall, KTRs with ultralong-term survival ≥20 years do extremely well. Particularly KTRs with stable and high eGFR and low proteinuria likely keep their graft function and ultimately die of medical comorbidities. The risk of graft failure is predominantly seen in KTRs with inferior graft function. This graft function-related risk profile could augment long-term monitoring and treatment.
Data Availability Statement
The data underlying this article will be shared on reasonable request to the corresponding author.
Ethics Statement
The studies involving human participants were reviewed and approved by Cantonal Ethics Committee Zurich. Written informed consent for participation was not required for this study in accordance with the national legislation and the institutional requirements.
Author Contributions
AR participated in data collection, data analysis, and writing of the paper. JN participated in data collection and writing of the paper. RW participated in writing of the paper. TM participated in research design and writing of the paper. TS participated in research design, data collection, data analysis and writing of the paper.
Conflict of Interest
The authors declare that the research was conducted in the absence of any commercial or financial relationships that could be construed as a potential conflict of interest.
Supplementary Material
The Supplementary Material for this article can be found online at: https://www.frontierspartnerships.org/articles/10.3389/ti.2022.10675/full#supplementary-material
Abbreviations
ABMR, Antibody-mediated rejection; BMI, Body mass index; BSA, Body surface area; CI, Confidence interval; CKD, Chronic kidney disease; CKD-EPI, Chronic kidney disease epidemiology collaboration; CNI, Calcineurin inhibitor; CTS, Collaborative Transplant Study; DCD, Donation after cardiac death; DGF, Delayed graft function; DSA, Donor specific antibody; dnDSA, De novo donor specific antibody; DC-GF, Death-censored graft failure; DWFG, Death with functioning graft; eGFR, Estimated glomerular filtration rate; ESKD, End stage kidney disease; HLA, Human leukocyte antigen; KTR, Kidney transplant recipient; MDRD, Modification of diet in renal disease; MFI, Mean fluorescence intensity; PCR, Protein-to-creatinine ratio; ULS, Ultralong-term survivors.
References
1. Chadban, SJ, Ahn, C, Axelrod, DA, Foster, BJ, Kasiske, BL, Kher, V, et al. Summary of the Kidney Disease: Improving Global Outcomes (KDIGO) Clinical Practice Guideline on the Evaluation and Management of Candidates for Kidney Transplantation. Transplantation (2020) 104(4):708–14. doi:10.1097/TP.0000000000003137
2. Coemans, M, Süsal, C, Döhler, B, Anglicheau, D, Giral, M, Bestard, O, et al. Analyses of the Short- and Long-Term Graft Survival after Kidney Transplantation in Europe between 1986 and 2015. Kidney Int (2018) 94(5):964–73. doi:10.1016/j.kint.2018.05.018
3.ANZDATA Registry. Chapter 7: Kidney Transplantation, Australia and New Zealand Dialysis and Transplant Registry. 43rd Report. Adelaide, Australia (2020). Available at: http://www.anzdata.org.au (Accessed December 14, 2021).
4.United States Renal Data System. USRDS Annual Data Report: Epidemiology of Kidney Disease in the United States. Bethesda, MD: National Institutes of Health, National Institute of Diabetes and Digestive and Kidney Diseases (2021).
5.Canadian Institute for Health Information. Treatment of End-Stage Organ Failure in Canada, Canadian Organ Replacement Register, 2011 to 2020: End-Stage Kidney Disease and Kidney Transplants – Data Tables. Ottawa, ON: CIHI (2021).
6.Collaborative Transplant Study. CTS Outcome Graphs (2022). Graph K-15103E-0222. Available at: https://www.ctstransplant.org/public/graphics/archive.shtml (Accessed December 14, 2021).
7. Bererhi, L, Pallet, N, Zuber, J, Anglicheau, D, Kreis, H, Legendre, C, et al. Clinical and Immunological Features of Very Long-Term Survivors with a Single Renal Transplant: Renal Transplant Patients after 30 Years. Transpl Int (2012) 25(5):545–54. doi:10.1111/j.1432-2277.2012.01451.x
8. Traynor, C, Jenkinson, A, Williams, Y, O’Kelley, P, Hickey, D, Denton, M, et al. Twenty-Year Survivors of Kidney Transplantation. Am J Transpl (2012) 12(12):3289–95. doi:10.1111/j.1600-6143.2012.04236.x
9. McCaughan, JA, and Courtney, AE. The Clinical Course of Kidney Transplant Recipients after 20 Years of Graft Function: Outcome after 20 Years of Transplant Function. Am J Transpl (2015) 15(3):734–40. doi:10.1111/ajt.13041
10. Manna, GL, Capelli, I, Gasperoni, L, Comai, G, Ravaioli, M, Marchetti, A, et al. Long Term Outcomes of Kidney Transplant: Characteristics of Recipients with 20 or More Years of Graft Survival. J Med Surg Pathol (2016) 01(02). doi:10.4172/2472-4971.1000109
11. Parajuli, S, Mandelbrot, DA, Aziz, F, Garg, N, Muth, B, Mohamed, M, et al. Characteristics and Outcomes of Kidney Transplant Recipients with a Functioning Graft for More Than 25 Years. Kidney Dis (2018) 4(4):255–61. doi:10.1159/000491575
12. Kettler, B, Scheffner, I, Bräsen, JH, Hallensleben, M, Richter, N, Heiringhoff, KH, et al. Kidney Graft Survival of >25 Years: a Single center Report Including Associated Graft Biopsy Results. Transpl Int (2019) 32(12):1277–85. doi:10.1111/tri.13469
13. El-Agroudy, AE, Bakr, MA, Hassan, NA, Ismail, AM, Ali-El-Dein, B, Shehab El-Dein, AB, et al. Characteristics of Long-Term Live-Donor Renal Allograft Survivors. Am J Nephrol (2003) 23(3):165–71. doi:10.1159/000070333
14. Peddi, V, Whiting, J, Weiskittel, P, Alexander, J, and First, M. Characteristics of Long-Term Renal Transplant Survivors. Am J Kidney Dis (1998) 32(1):101–6. doi:10.1053/ajkd.1998.v32.pm9669430
15. Rao, VK. Long Term Results and Complications of Renal Transplantation: Observations in the Third Decade. Indian J Nephrol (2001) 11:155–9.
16. Gaston, RS, Fieberg, A, Helgeson, ES, Eversull, J, Hunsicker, L, Kasiske, BL, et al. Late Graft Loss after Kidney Transplantation: Is “Death with Function” Really Death with a Functioning Allograft? Transplantation (2020) 104(7):1483–90. doi:10.1097/TP.0000000000002961
17. Levey, AS, Bosch, JP, Lewis, JB, Greene, T, Rogers, N, and Roth, D. A More Accurate Method to Estimate Glomerular Filtration Rate from Serum Creatinine: a New Prediction Equation. Modification of Diet in Renal Disease Study Group. Ann Intern Med (1999) 130(6):461–70. doi:10.7326/0003-4819-130-6-199903160-00002
18. Levey, AS, Stevens, LA, Schmid, CH, Zhang, YL, Castro, AF, Feldman, HI, et al. CKD-EPI (Chronic Kidney Disease Epidemiology Collaboration): a New Equation to Estimate Glomerular Filtration Rate. Ann Intern Med (2009) 150:604–12. doi:10.7326/0003-4819-150-9-200905050-00006
19. Cockcroft, DW, and Gault, MH. Prediction of Creatinine Clearance from Serum Creatinine. Nephron (1976) 16:31–41. doi:10.1159/000180580
20. Zelnick, LR, Leca, N, Young, B, and Bansal, N. Association of the Estimated Glomerular Filtration Rate with vs without a Coefficient for Race with Time to Eligibility for Kidney Transplant. JAMA Netw Open (2021) 4(1):e2034004. doi:10.1001/jamanetworkopen.2020.34004
21. DuBois, D, and DuBois, E. A Formula to Estimate the Approximate Surface Area if Height and Weight Be Known. Arch Intern Med (1916) 17:863–71. doi:10.1001/archinte.1916.00080130010002
22. Delanaye, P, and Krzesinski, JM. Indexing of Renal Function Parameters by Body Surface Area: Intelligence or Folly. Nephron Clin Pract (2011) 119(4):c289–92. doi:10.1159/000330276
23. Vlasschaert, C, Thibodeau, S, and Parmar, MS. De‐indexed Estimated Glomerular Filtration Rates: A Simple Step towards Improving Accuracy of Drug Dosing of Renally Excreted Medications in Moderate to Severe Obesity. Nephrology (2020) 25(1):29–31. doi:10.1111/nep.13621
24. Mitch, W, Walser, M, Buffington, G, and Lemann, J. A Simple Method of Estimating Progression of Chronic Renal Failure. Lancet (1976) 2:1326–8. doi:10.1016/s0140-6736(76)91974-7
25.Kidney Disease: Improving Global Outcomes (KDIGO) CKD Work Group. KDIGO 2012 Clinical Practice Guideline for the Evaluation and Management of Chronic Kidney Disease. Kidney inter., Suppl (2013) 3:1–150.
26. Zwiener, I, Blettner, M, and Hommel, G. Survival Analysis— Part 15 of a Series on Evaluation of Scientific Publications. Dtsch Arztebl Int (2011) 108(10):163–9. doi:10.3238/arztebl.2010.0163
27. Summers, DM, Watson, CJ, Pettigrew, GJ, Johnson, RJ, Collett, D, Neuberger, JM, et al. Kidney Donation after Circulatory Death (DCD): State of the Art. Kidney Int (2015) 88(2):241–9. doi:10.1038/ki.2015.88
28. Meier-Kriesche, HU, Ojo, AO, Leavey, SF, Hanson, JA, Leichtman, AB, Magee, JC, et al. Gender Differences in the Risk for Chronic Renal Allograft Failure. Transplantation (2001) 71(3):429–32. doi:10.1097/00007890-200102150-00016
29. Carrero, JJ, Hecking, M, Chesnaye, NC, and Jager, KJ. Sex and Gender Disparities in the Epidemiology and Outcomes of Chronic Kidney Disease. Nat Rev Nephrol (2018) 14(3):151–64. doi:10.1038/nrneph.2017.181
30. Heemann, U, Abramowicz, D, Spasovski, G, and Vanholder, R. Endorsement of the Kidney Disease Improving Global Outcomes (KDIGO) Guidelines on Kidney Transplantation: a European Renal Best Practice (ERBP) Position Statement. Nephrol Dial Transpl (2011) 26(7):2099–106. doi:10.1093/ndt/gfr169
31. El-Zoghby, ZM, Stegall, MD, Lager, DJ, Kremers, WK, Amer, H, Gloor, JM, et al. Identifying Specific Causes of Kidney Allograft Loss. Am J Transpl (2009) 9(3):527–35. doi:10.1111/j.1600-6143.2008.02519.x
32. Matas, AJ. Chronic Progressive Calcineurin Nephrotoxicity: an Overstated Concept. Am J Transpl (2011) 11(4):687–92. doi:10.1111/j.1600-6143.2011.03505.x
33. Wojciechowski, D, and Wiseman, A. Long-Term Immunosuppression Management: Opportunities and Uncertainties. Clin J Am Soc Nephrol (2021) 16(8):1264–71. doi:10.2215/CJN.15040920
34. Gourishankar, S, Leduc, R, Connett, J, Cecka, JM, Cosio, F, Fieberg, A, et al. Pathological and Clinical Characterization of the ‘Troubled Transplant’: Data from the DeKAF Study. Am J Transpl (2010) 10(2):324–30. doi:10.1111/j.1600-6143.2009.02954.x
35. Rao, PS, Schaubel, DE, Guidinger, MK, Andreoni, KA, Wolfe, RA, Merion, RM, et al. A Comprehensive Risk Quantification Score for Deceased Donor Kidneys: The Kidney Donor Risk Index. Transplantation (2009) 88(2):231–6. doi:10.1097/TP.0b013e3181ac620b
36. Denic, A, Glassock, RJ, and Rule, AD. Structural and Functional Changes with the Aging Kidney. Adv Chronic Kidney Dis (2016) 23(1):19–28. doi:10.1053/j.ackd.2015.08.004
37. Summers, DM, Johnson, RJ, Hudson, A, Collett, D, Watson, CJ, and Bradley, JA. Effect of Donor Age and Cold Storage Time on Outcome in Recipients of Kidneys Donated after Circulatory Death in the UK: a Cohort Study. Lancet (2013) 381(9868):727–34. doi:10.1016/S0140-6736(12)61685-7
38. Moreso, F, Seron, D, Gil-Vernet, S, Riera, L, Fulladosa, X, Ramos, R, et al. Donor Age and Delayed Graft Function as Predictors of Renal Allograft Survival in Rejection-free Patients. Nephrol Dial Transpl (1999) 14(4):930–5. doi:10.1093/ndt/14.4.930
39. Einecke, G, Sis, B, Reeve, J, Mengel, M, Campbell, PM, Hidalgo, LG, et al. Antibody-Mediated Microcirculation Injury Is the Major Cause of Late Kidney Transplant Failure. Am J Transpl (2009) 9(11):2520–31. doi:10.1111/j.1600-6143.2009.02799.x
40. Lachmann, N, Terasaki, PI, Budde, K, Liefeldt, L, Kahl, A, Reinke, P, et al. Anti-Human Leukocyte Antigen and Donor-specific Antibodies Detected by Luminex Post-transplant Serve as Biomarkers for Chronic Rejection of Renal Allografts. Transplantation (2009) 87(10):1505–13. doi:10.1097/TP.0b013e3181a44206
41. Wiebe, C, Gibson, IW, Blydt-Hansen, TD, Pochinco, D, Birk, PE, Ho, J, et al. Rates and Determinants of Progression to Graft Failure in Kidney Allograft Recipients with De Novo Donor-specific Antibody: Post- Dn DSA Clinical Histologic Progression. Am J Transpl (2015) 15(11):2921–30. doi:10.1111/ajt.13347
42. Zhang, R. Donor-Specific Antibodies in Kidney Transplant Recipients. Clin J Am Soc Nephrol (2018) 13(1):182–92. doi:10.2215/CJN.00700117
43. Calp-Inal, S, Ajaimy, M, Melamed, ML, Savchik, C, Masiakos, P, Colovai, A, et al. The Prevalence and Clinical Significance of C1q-Binding Donor-specific Anti-HLA Antibodies Early and Late after Kidney Transplantation. Kidney Int (2016) 89(1):209–16. doi:10.1038/ki.2015.275
44. Konvalinka, A, and Tinckam, K. Utility of HLA Antibody Testing in Kidney Transplantation. J Am Soc Nephrol (2015) 26(7):1489–502. doi:10.1681/ASN.2014080837
45. Schinstock, CA, Mannon, RB, Budde, K, Chong, AS, Haas, M, Knechtle, S, et al. Recommended Treatment for Antibody-Mediated Rejection after Kidney Transplantation: The 2019 Expert Consensus from the Transplantion Society Working Group. Transplantation (2020) 104(5):911–22. doi:10.1097/TP.0000000000003095
46. Lorenz, EC, El-Zoghby, ZM, Amer, H, Dean, PG, Hathcock, MA, Kremers, WK, et al. Kidney Allograft Function and Histology in Recipients Dying with a Functioning Graft: Kidney Function in DWF. Am J Transpl (2014) 14(7):1612–8. doi:10.1111/ajt.12732
47. Ojo, AO, Hanson, JA, Wolfe, RA, Leichtman, AB, Agodoa, LY, and Port, FK. Long-term Survival in Renal Transplant Recipients with Graft Function. Kidney Int (2000) 57(1):307–13. doi:10.1046/j.1523-1755.2000.00816.x
48. Kasiske, BL, Zeier, MG, Chapman, JR, Craig, JC, Ekberg, H, Garvey, CA, et al. KDIGO Clinical Practice Guideline for the Care of Kidney Transplant Recipients: a Summary. Kidney Int (2010) 77(4):299–311. doi:10.1038/ki.2009.377
49. Baker, RJ, Mark, PB, Patel, RK, Stevens, KK, and Palmer, N. Renal Association Clinical Practice Guideline in post-operative Care in the Kidney Transplant Recipient. BMC Nephrol (2017) 18(1):174. doi:10.1186/s12882-017-0553-2
50. Levin, A, and Stevens, PE. Summary of KDIGO 2012 CKD Guideline: behind the Scenes, Need for Guidance, and a Framework for Moving Forward. Kidney Int (2014) 85(1):49–61. doi:10.1038/ki.2013.444
Keywords: kidney transplantation, TCMR, de novo DSA, kidney allograft function, survival
Citation: Reimann AV, Nilsson J, Wuethrich RP, Mueller TF and Schachtner T (2022) Entering the Third Decade After Kidney Transplantation: Excellent Graft Function Refers to Superior Graft but Not Patient Survival. Transpl Int 35:10675. doi: 10.3389/ti.2022.10675
Received: 26 May 2022; Accepted: 18 October 2022;
Published: 31 October 2022.
Copyright © 2022 Reimann, Nilsson, Wuethrich, Mueller and Schachtner. This is an open-access article distributed under the terms of the Creative Commons Attribution License (CC BY). The use, distribution or reproduction in other forums is permitted, provided the original author(s) and the copyright owner(s) are credited and that the original publication in this journal is cited, in accordance with accepted academic practice. No use, distribution or reproduction is permitted which does not comply with these terms.
*Correspondence: Thomas Schachtner, dGhvbWFzLnNjaGFjaHRuZXJAdXN6LmNo, b3JjaWQub3JnLzAwMDAtMDAwMS01NTQ5LTQ3OTg=