- 1Division of Plastic and Reconstructive Surgery, School of Medicine, Yale University, New Haven, CT, United States
- 2Institute of Musculoskeletal Medicine, University Hospital Münster, Münster, Germany
- 3Division of General Internal Medicine, Nephrology and Rheumatology, Department of Medicine D, University Hospital Münster, Münster, Germany
- 4Department of Plastic, Aesthetic, Hand and Reconstructive Surgery, Hannover Medical School, Hanover, Germany
- 5Division of Plastic Surgery, Department of Trauma, Hand and Reconstructive Surgery, Institute of Musculoskeletal Medicine, University Hospital Münster, Münster, Germany
- 6Department of Plastic, Reconstructive and Aesthetic Surgery, Hand Surgery, Fachklinik Hornheide, Münster, Germany
Pre-clinical studies are an obligatory tool to develop and translate novel therapeutic strategies into clinical practice. Acute and chronic rejection mediated by the recipient’s immune system remains an important limiting factor for the (long-term) survival of vascularized composite allografts (VCA). Furthermore, high intensity immunosuppressive (IS) protocols are needed to mitigate the immediate and long-term effects of rejection. These IS regiments can have significant side-effects such as predisposing transplant recipients to infections, organ dysfunction and malignancies. To overcome these problems, tolerance induction has been proposed as one strategy to reduce the intensity of IS protocols and to thereby mitigate long-term effects of allograft rejection. In this review article, we provide an overview about animal models and strategies that have been used to induce tolerance. The induction of donor-specific tolerance was achieved in preclinical animal models and clinical translation may help improve short and long-term outcomes in VCAs in the future.
Introduction
To date, about 150 vascularized composite allotransplants (VCAs) including over 40 face and 120 extremity transplantations, have been performed worldwide. Approximately 40 VCA programs across five continents have been established to date, and more VCA centers are anticipated to be established in the future (1,2).
In the field of reconstructive surgery, the geometric uniqueness and often resulting functional deficit of a composite tissue defect are major challenges. Vascularized composite allotransplantation (e.g., facial VCA) has revolutionized restoration of form and function of the most complex defects. For example, facial transplantation can now be offered to selected patients at experienced centers with reproducible results (3-5). However, acute and chronic rejection as well as the resulting need for life-long multidrug immunosuppression (IS) limit the more widespread use of this revolutionary biotechnology.
Side-effects related to these IS protocols (e.g., increased susceptibility to infection, malignancy, and organ dysfunction) continue to adversely affect the risk-benefit ratio transplants, particularly in case of VCA which is not a lifesaving but rather a life-giving procedure.
In this context, tolerance induction, the long-term immunosuppression-free graft acceptance without clinical or histological evidence of rejection, has become the topic of many preclinical and clinical research endeavors. The strategy of tolerance induction is often viewed as the holy grail of achieving improved transplant outcomes for example by preventing or slowing the development of chronic rejection, which is still an important long-term cause of graft loss in solid organ transplantation and presumably also in VCA (6,7).
Given the inherent complexity and diversity of VCA procedures, different animal models have been established and evaluated, while additional models are being developed to help answer specific VCA related immunological questions. We present a brief review of the most commonly used preclinical approaches of tolerance induction in the field of VCA.
Tolerance Induction in VCA
Tolerance induction strategies can be broadly categorized into cell- and pharmaceutical based strategies. The goal is to achieve graft acceptance and minimize the need for less specific and highly toxic systemic immunosuppression such as tacrolimus and MMF. The interest in cell-based strategies has been increasing over the past 10 years. Cell-based strategies include the use of stem (e.g.,: bone marrow transplant-derived, mesenchymal stem cells) and/or immune cells (e.g.,: dendritic cells, T reg cells) plus usually a co-administration of a drug therapy regimen (8). Figure 1 illustrates standard tolerance induction strategies that have been used in preclinical models of VCA. The goal of stem cell-based therapies is the establishment of mixed chimerism which was described in detail in 1988 by Sykes and Sachs as a phenomenon of host and donor bone marrow-derived cell coexistance (9). For example, mixed chimerism can be induced by transfer of donor hematopoietic stem cells (HSCs) from bone marrow or cytokine-mobilized peripheral stem cells. Typically, the recipient is conditioned in order to control alloreactivity and generate space for donor HSCs engraftment. The co-existence of donor and recipients HSC is thought to promote donor-specific tolerance while maintaining immunocompetence (10,11).
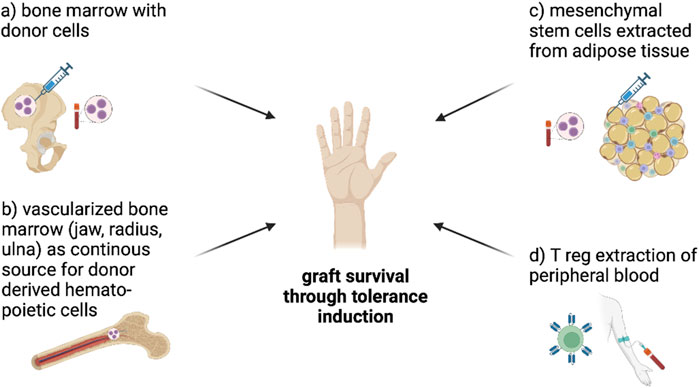
FIGURE 1. Figure shows cell-based tolerance induction approaches in VCA. Bone marrow transplantation with donor-derived stem cells can induce mixed chimerism and thereby induce tolerance (A), vascularized bone marrow as continuous source for donor derived hematopoietic cells might be able to help minimize immunosuppressants (B) and mesenchymal stem cells extracted from adipose tissue (C) are already tested in VCA to induce tolerance through mixed chimerism and direct effects on the graft. The extraction of Treg cells (D), modification into CAR-Tcells using CRISPR/C9 and infusion into humans is tested in clinical trials with SOT so far and seems to be a promising approach to induce tolerance in VCA. CAR-Tcells can recognize MHC-I on donor cells and block their interaction with the recipient´s immune system to prevent rejection (Figure created with BioRender.com).
Mixed chimerism alone was shown to not consistently achieve tolerance induction and thus Leonard et al. described different approaches achieving mixed chimerism combined with adjuvant cellular therapies leading to tolerance induction in pigs (3,10). The longest tolerance induction through establishment of mixed chimerism was demonstrated in a pig model by Leonard et al. (12,13) in 2015 (14) after performing VCA in stable mixed chimerism or concurrent with induction of mixed chimerism following hematopoietic stem cell transplantation. Both groups showed no signs of rejection up to 504 days after transplantation.
To achieve a continuous supply of donor derived bone marrow elements, Barth et al. further analyzed the role of vascularized bone in a heterotopic partial face transplantat model in NHPs (15). VCA containing vascularized bone not only led to prolonged, but rejection free graft survival (430 days) compared to VCA without vascularized bone (7 days). Schneeberger et al. developed the “Pittsburg Protocol” as an approach in humans following upper-extremity transplantation. Bone marrow cell-based treatment allowed a maintenance low-dose immunosuppression protocol compared to conventional protocols (16). However, long-term results have not been published and further studies are needed to evaluate the safety and reliability of this approach.
Conditioning of the recipient entails toxic side effects, thus approaches of bone marrow infusion-mediated immunomodulation without the necessity of conditioning are studied at the time (17). In this context other immune cells have been studied. Mesenchymal stem cells (MSCs) are multipotent stem cells that can differentiate into mesenchymal cell lineages. MSCs are derived from the bone marrow but can also be extracted from for example adipose tissue (= adipose derived MSCs) and have shown promising immunomodulatory effects by regulation of T-cell proliferation and inhibition of dendritic cell differentiation as they interact with the innate and adaptive immune system (18). Not only can MSCs induce mixed chimerism in the recipient, but they can also modulate cytokine expression in VCAs and hereby may be able to prolong graft survival(19). Promising results have been shown in small and large animal models, and clinical trials using mesenchymal stem cells have been established in solid organ transplants with promising results (8).
Ongoing clinical trials in humans evaluate the potential of dendritic cells as major regulator of the human immune system. An injection of immature dendritic cells was shown to have an immunosuppressant effect and to achieve inhibition of memory T cells (20). Another promising approach is the use regulatory T-cells. Tregs help maintain and regulate self-tolerance, antimicrobial resistance, tumor immunity and transplant rejection. Tregs exert their immunosuppressive effects via cell-to-cell interaction with target immune cells, via removal of IL-2 (potent factor in T cell survival and growth), via anti-inflammatory molecules (TGF-beta) or through costimulatory pathways such as binding to cytotoxic T-lymphocyte-associated protein 4. Nevertheless, irregularity in Tregs can lead to autoimmune disorders, reduced disease tolerance or higher risk for cancer(21). Recent studies have investigated the potential therapeutic value of genetically modified patient-derived Tregs, such as antigen-specific Chimeric Antigen Receptor (CAR)-Tregs, targeting for example MHC-class I expressed by donor cells. Tregs can be isolated from patient´s blood samples, cultured and expanded to produce for example CAR-Treg cells. Those conditioned T cells can be infused into the patient. Clinical trials did show the safety, efficacy, and proof of concept of Treg therapy in kidney and liver transplantation (22). The engineering of Tcells (e.g.: CAR-Tregs) might be a promising supplementary treatment of rejection in the future. CAR-T cells are typically designed to recognize donor MHC molecules, thus localizing to donor tissues and exerting their regulatory effects in a precise and targeted manner (21).
Pharmaceutical therapy regimens are the current gold standard in VCA and are mostly modifications of regimens that have already been established in SOT (23). Usually, an induction therapy (e.g.,: Anti-Thymoglobulin, Alemtuzumab) is administered first and a maintenance immunosuppressive regimen (Tacrolimus, Mycophenolate Mofetil (MMF), Prednisone) is given (8). Anti-Thymocyte globulin (ATG) is used as induction agent in most cases and leads to decreased T-cell mediated rejection (10). The maintaining therapy of the calcineurin inhibitor (CNI) Tacrolimus, Corticosteroids (Prednisone) and MMF (inhibits proliferation of lymphocytes) often involves side effects such as myopathy, diabetes mellitus, impaired kidney function, abdominal pain or diarrhea. Aside from the standard immunosuppressive regiments, other pharmaceutical approaches have been tested, for example tolerance inducing medications.
Cell mediated graft rejection in VCA is fueled by the CD28 and CD80/86 co-stimulatory pathway of T-cells (Figure 2). Preventing or interfering with co-stimulation of T cells is an approach for improved allograft survival and a more specific pharmaceutical approach that may cause less severe side effects compared to the conventional approaches (11). CD28 surface T-cell receptors communicate with antigen-presenting cells through CD80 and CD86 ligands and evokes both T-cell proliferation and pro-inflammatory pathways. Drugs like Belatacept or Abatacept are already established anti-rejection medications used in humans to interfere with co-stimulatory pathways. Both inhibit the costimulatory pathway through CTLA4-Ig or CD 125 (Fusion protein of cytotoxic T-lymphocyte-associated antigen 4 Immunoglobulin and IgG1 Fc) by inhibiting the linking of CD28 (T-cell surface) and CD80/86 (APC surface) (15,17). Belatacept can serve as replacement for CNI after kidney transplantation as side effects appear to be less than with CNI. Belatacept is already in clinical application following hand and/or face transplantation along with low dose of CNI/MMF/prednisone. Subsidiary, topical administration of immunosuppression might also reduce the systematically dosage of immunosuppressants. Tacrolimus and steroids are available as creams or mouthwashes as “transplant-targeted therapy” and show promising results in first approaches (8).
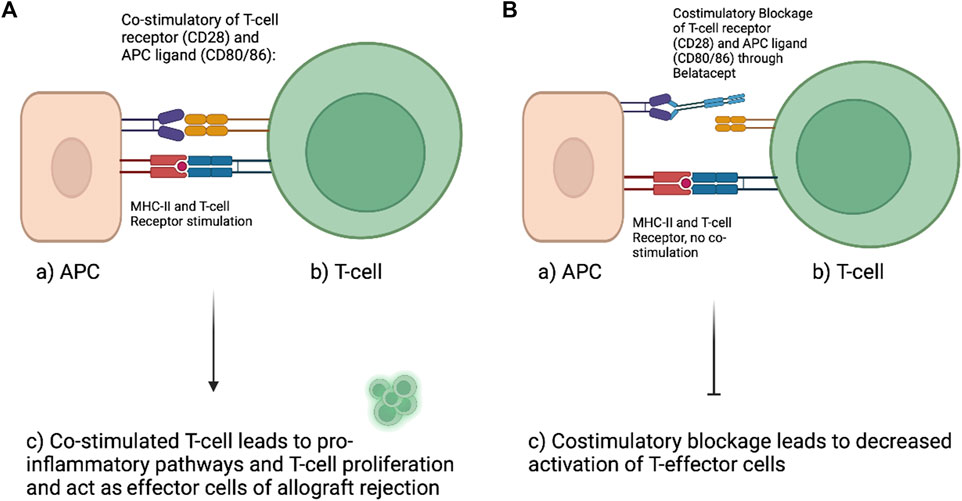
FIGURE 2. Left (A) demonstrates physiologic naïve T-cell activation. Antigen presenting cells (APC) interact with the CD28 surface receptor of naïve T-cells with their ligands CD80/86 while the antigen is presented to the T-cell. This interaction will promote T-cell proliferation and activation of cellular inflammatory pathways. (B) With the use of Belatacept (second-generation CTLA4-Ig) the costimulatory interaction between APC and T-cell can be altered. Belatacept blocks the interaction of CD28 with CD80/86 by binding to CD80/86. This interaction limits the activation of T-cells and was shown to reduce alloreactivity and thereby prolong allograft survival (Figure created with BioRender.com).
Large Versus Small Animal Model
Rodent models are of great value especially in VCA research and are most popular for preclinical studies as they allow precise genetic breeding, are easy to reproduce and handle, produce low costs in housing and breeding and enable therefore larger study groups. The majority of the described animal models in this article used rat models and most commonly the hindlimb model was used. Mice allow more precise genetic manipulation which makes analysis of immunological mechanisms easier when compared to other small animal models. However, disadvantages of rat and mice models are related to their smaller size in terms of anatomic structures and the lack of similarity to the human immune system. Furthermore, the results of rodent models have often failed to be successfully transferred to large animal models or human trials (8). In addition, their short lifespan can make it difficult to truly assess long-term graft survival. Most importantly, the development of a naive immune system in largely germ-free laboratory environments and the lack of class II antigen expression on rodent vascular endothelium suggests a fundamentally different immune system than that of humans (11, 24-27).
Large animals are suited as preclinical models as they allow evaluation of a hypothesis in a complex system that has strong similarities to humans in terms of anatomical structures, size, and immune responses, making data potentially more reliable for translation to humans (28). Swine models also allow genetic manipulation to control for example the degree of MHC mismatch in transplantation and share great similarity with humans in structure, cellular and antigenic attributes in skin (8). NHP models are also of great value as they are even more similar in human anatomy than pigs and showed similar transplant rejection to humans. Hand transplantation is feasible and did show good motor function in NHPs (29). Disadvantages are cost and for example with primates’ ethical concerns, slower reproductive cycles. Especially the skin of humans and pigs show a great similarity which is even more important with skin being a crucial part of VCA and complicating factor on the way to long-term graft survival and tolerance induction. Having a great similarity with pig skin makes pig animal model most valuable for research. Finally, the high costs of livestock breeding and research in large animals, the ethical status in NHP models on the one hand and the simplicity of reproducibility, handling of rodents and low costs on the other hand may explain why most research groups are using rodents.
Mouse Models
Costimulatory Blockade (CoB) takes advantage of the mechanism that naïve helper and cytotoxic Tcells must be activated through different pathways to become effector T-cells that can ultimately promote graft rejection. Antibodies, antigens, and immunosuppressive drugs (e.g.: Belatacept) can inhibit T-cell costimulatory pathways and, combined with adjuvant strategies such as total body irradiation (TBI) or stem cell transplantation, may induce mixed chimerism and thereby induce graft tolerance in VCA (10). Tolerance induction in all mice models described here (Table 1) was achieved via drug-based strategies utilizing costimulatory blockade in combination with adjuvant stem cell and/or TBI therapy regimen. Tolerance has been successfully induced up to 120–210 days in all mouse models described in this article. Five out of six models used an osteomyocutaneous hindlimb allograft transplant model while one used a full-thickness skin graft model without inclusion of bone or muscles. Lin et al. (2016) and Anggelia et al. (2021) showed successful induction of donor-specific tolerance (survival >120 days) by application of CoB (anti-CD154, CTLA4-Ig) with a short treatment of rapamycin (30,31). Both studies achieved graft survival up to 120 days. Lin et al. (2021) added high-dose bone marrow transplantation cells to the regimen of CoB (anti-CD154, CTLA4-Ig) and rapamycin and induced tolerance by mixed chimerism for >120 days in vascular bone marrow transplantation (32). Oh et al. (2020) combined the CoB (anti-CD154, CTLA4-Ig) with TBI and demonstrated tolerance induction and a depletion of alloreactive T cells for >210 days. Depletion of alloreactive T cells appears to be a promising mechanism for long-term graft feasibility and a key to long-term viability in this approach (33). Lin et al. (2013) used a different approach in vitro; the group used a donor antigen-specific CD4−CD8− double negative Treg-based therapy plus anti-lymphocyte serum (ALS) plus rapamycin plus Il-2/Fc fusion proteins and showed tolerance induction in VCA but interestingly not in full thickness grafts (34). Davis et al. (2014) applied adipose-derived stromal cells (ASC) with non-myeloablative low-dose busulfan plus anti-CD4/CD8 and induced tolerance for >180 days as well as mixed macrochimerism (35). The longest graft survival plus depletion of alloreactive Tcells for over 210 days could be shown via CoB plus TBI 1 day before surgery by Oh et al (2020). This approach is furthermore relinquishing immunosuppressants which could be a promising in terms of that patients are mostly young and healthy when receiving VCA and therefore suffer a lot from long-term toxic side effects.
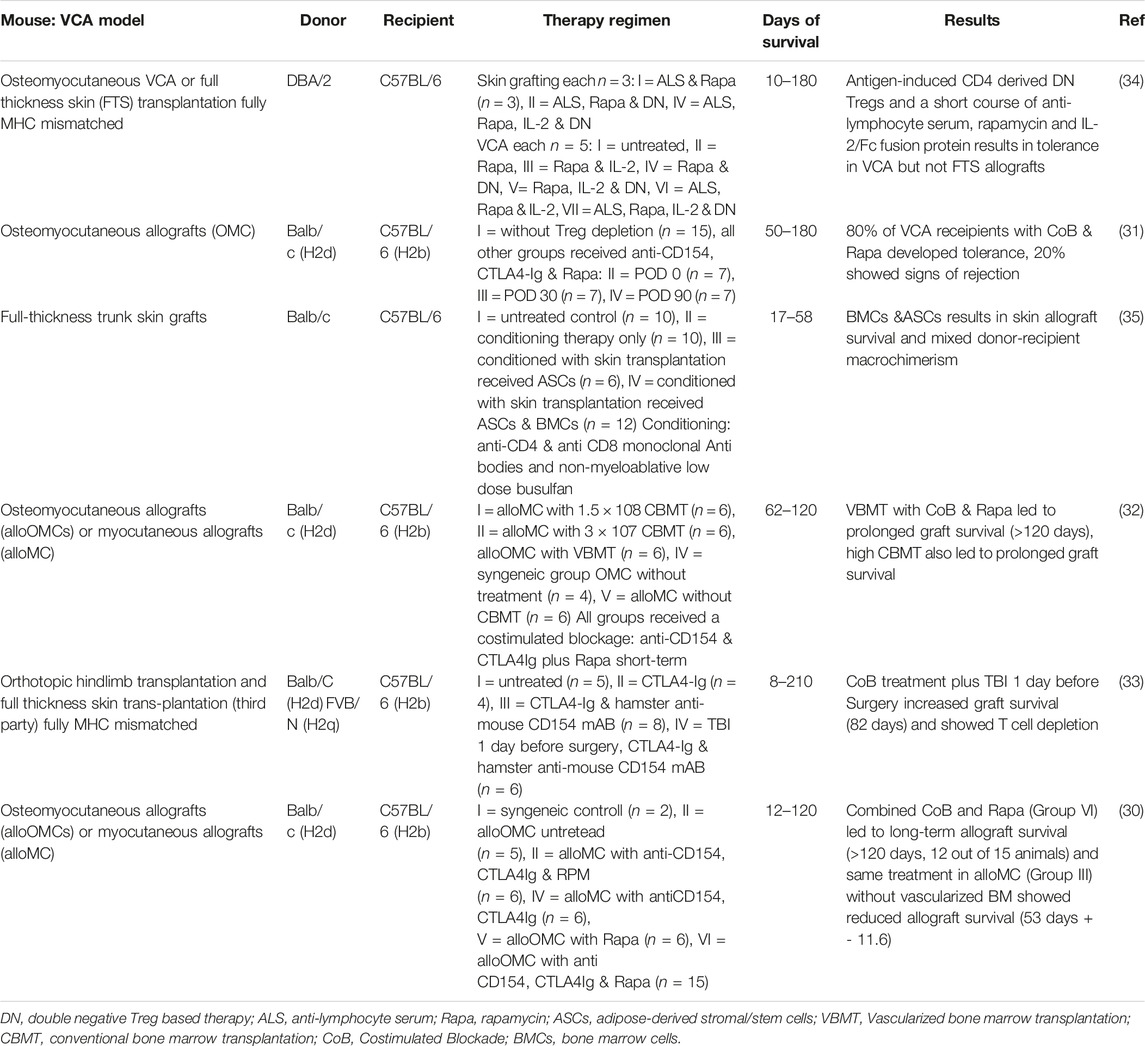
TABLE 1. VCA models with detailed therapy regimen in Mice, all models developed tolerance induction.
Rat Models
Tolerance induction was achieved in five out of eight described rat models (Table 2), and stem cells were used in most approaches to obtain longer-term graft survival. In total, four studies used a hindlimb, two a skin flap, one abdominal wall with/without hindlimb and one group an osseomusculuocutanous sternum with/without thymus model. Ramirez et al. (2013) showed a successfully induced tolerance and peripheral chimerism using a regimen of cyclosporine A (CsA), ALS, and adipocyte-derived stem cells (36). Jindal et al. (2015) used human IL-2 fusion protein and combined this regimen with ALS plus CsA (37). This regimen showed the longest graft survival with >150 days plus tolerance induction but no mixed chimerism were analyzed.
Plock et al. (2015) changed the regimen to only a short course of FK-506 plus either adipose- or bone marrow-derived mesenchymal stem cells and induced tolerance as well as long-term graft survival and transient mixed chimerism (>120 days) (38). Cheng et al. (2013) used adipocyte-derived stem cells, CsA and ALS. Tolerance induction and long-term graft survival could be achieved up to 150 days but no mixed chimerism were analyzed (39). A single donor-recipient chimeric cells (DRCC) therapy by ex vivo cell fusion (of bone marrow cells) was applied by Cwykiel et al. (2021) in combination with CsA and antibody therapy and resulted in graft survival of >79 days, tolerance induction plus peripheral chimerism (40). In 2016, Sieminow et al. showed establishment of mixed chimerism by preconditioning recipient bone marrow cells (BMC) followed by anti-
Swine Models
In swine (Table 3), tolerance induction was attempted by establishing mixed chimerism with and without co-stimulatory blockage. One group used a full-skin flap, the second group used an osteomyocutaneos flap. Leonard et al. (2014) successfully induced tolerance in their large animal model. The recipient was preconditioned by T-cell depletion plus TBI, followed by hematopoietic cell transplantation. VCA was either performed into stable mixed chimerism or after establishing of mixed chimerism 85–105 days later. After transplantation, a 45-day course of CsA was given. No signs of rejection were seen between 115–504 days posttransplant (14). Lellouch et al. (2022) showed tolerance induction by mixed chimerism across MHC class-I-mismatch up to 400 days posttransplant. Treatment was TBI and thymic irradiation 2 days before surgery. Bone marrow transplantation was performed on the day of surgery, followed by co-stimulatory blockade (CTLA4-Ig + FK405) for 30 days and treatment of anti-IL6R monoclonal antibody (mAb) plus methylprednisolone (44). Both approaches show promising results in terms of long-term graft survival, similarity to the human immune system and absence of long-term immunosuppression and serve as proof of concept in a preclinical large animal model.
NHP Model
As described above, to have a continuous source for donor stem cells, vascularized bone marrow transplantation might be an approach to induce tolerance through establishment of mixed chimerism (continuous source of donor-derived hematopoietic stem cells). This approach was tested by Barth et al. (Table 4) as he further analyzed the role of vascularized bone marrow transplantation (VBM) in a VCA model with a heterotopic partial face transplantation in NHPs. VCA containing vascularized bone not only led to prolonged, but also rejection free graft survival (430 days) compared to VCA without vascularized bone (7 days). However, withdrawal of immunosuppressants led to graft loss and tolerance induction was not achieved but VBM enabled a low dose maintenance immunosuppressants regimen and sporadic macrochimerism were detected (15). Although this approach was unable to achieve tolerance induction through mixed chimerism, these results serve as proof of concept. However, stable macro or micro chimerism has not been documented in human VCA. Further studies are necessary, for example on how to enhance the ability of vascularized bone marrow to induce chimerism, e.g., via Treg modulative strategies to increase acceptance of donor derived hematopoietic stem cells (21).

TABLE 4. VCA model with detailed therapy regimen in Non-Human Primate (NHP); vascularized bone marrow (VBM), bone marrow cells (BMC) infusion.
Conclusion
Both small and large animal models are relevant for preclinical VCA research. Small animal models are advantageous because of low costs in breeding and housing and especially mice are well suited for analysis of immunological mechanisms. Large animal models offer greater similarity to human immune system and human anatomy, but models tend to be more complex and cost intensive. Tolerance induction in preclinical models was achieved using both cellular based and pharmaceutical based strategies. Clinical translation of such strategies to human trials has yet to be done successfully, however, the induction of donor-specific tolerance may ultimately help improve the risk benefit ratio of VCA transplantation.
Author Contributions
LH and MK-N contributed to conducting and writing this review as shared first co-authors. VS and JF contributed in data analysis and literature research. MK-N, AD, SR, MK and TH contributed in research design and input on the manuscript. MK and AD supervised the manuscript process as shared last authors. All authors contributed to the article and approved the submitted version.
Conflict of Interest
LH is a recipient of a scholarship (Walter-Benjamin) from the German Research Foundation (DFG).
The remaining authors declare that the research was conducted in the absence of any commercial or financial relationships that could be construed as a potential conflict of interest.
References
1. Thuong, M, Petruzzo, P, Landin, L, Mahillo, B, Kay, S, Testelin, S, et al. Vascularized Composite Allotransplantation - a Council of Europe Position Paper. Transpl Int (2019) 32(3):233–40. doi:10.1111/tri.13370
2. Tchiloemba, B, Kauke, M, Haug, V, Abdulrazzak, O, Safi, AF, Kollar, B, et al. Long-term Outcomes after Facial Allotransplantation: Systematic Review of the Literature. Transplantation (2020) 105(8):1869–80. doi:10.1097/TP.0000000000003513
3. Kauke, M, Safi, AF, Zhegibe, A, Haug, V, Kollar, B, Nelms, L, et al. Mucosa and Rejection in Facial Vascularized Composite Allotransplantation: A Systematic Review. Transplantation (2020) 104(12):2616–24. doi:10.1097/TP.0000000000003171
4. Kauke, M, Panayi, AC, Safi, AF, Haug, V, Perry, B, Kollar, B, et al. Full Facial Retransplantation in a Female Patient—Technical, Immunologic, and Clinical Considerations. Am J Transplant (2021) 21(10):3472–80. doi:10.1111/ajt.16696
5. Kauke, M, Panayi, AC, Tchiloemba, B, Diehm, YF, Haug, V, Kollar, B, et al. Face Transplantation in a Black Patient — Racial Considerations and Early Outcomes. New Engl J Med (2021) 384(11):1075–6. doi:10.1056/NEJMc2033961
6. Leonard, DA, Cetrulo, CL, McGrouther, DA, and Sachs, DH. Induction of Tolerance of Vascularized Composite Allografts. Transplantation (2013) 95(3):403–9. doi:10.1097/TP.0b013e31826d886d
7. Ravindra, Kv., Xu, H, Bozulic, LD, Song, DD, and Ildstad, ST. The Need for Inducing Tolerance in Vascularized Composite Allotransplantation. Clin Dev Immunol (2012) 2012:438078–11. doi:10.1155/2012/438078
8. Kauke, M, Safi, AF, Panayi, AC, Palmer, WJ, Haug, V, Kollar, B, et al. A Systematic Review of Immunomodulatory Strategies Used in Skin-Containing Preclinical Vascularized Composite Allotransplant Models. J Plast Reconstr Aesthet Surg (2022) 75(2):586–604. doi:10.1016/j.bjps.2021.11.003
9. Sykes, M, and Sachs, DH. Mixed Allogeneic Chimerism as an Approach to Transplantation Tolerance. Immunol Today (1988) 9(1):23–7. doi:10.1016/0167-5699(88)91352-7
10. Giannis, D, Moris, D, and Cendales, LC. Costimulation Blockade in Vascularized Composite Allotransplantation. Front Immunol (2020) 11:544186. doi:10.3389/fimmu.2020.544186
11. Pilat, N, Sayegh, MH, and Wekerle, T. Costimulatory Pathways in Transplantation. Semin Immunol (2011) 23(4):293–303. doi:10.1016/j.smim.2011.04.002
12. Leonard, DA, McGrouther, DA, Kurtz, JM, and Cetrulo, CL. Tolerance Induction Strategies in Vascularized Composite Allotransplantation: Mixed Chimerism and Novel Developments. Clin Dev Immunol (2012) 2012:863264–8. doi:10.1155/2012/863264
13. Sachs, DH, Kawai, T, and Sykes, M. Induction of Tolerance through Mixed Chimerism. Cold Spring Harb Perspect Med (2014) 4(1):a015529. doi:10.1101/cshperspect.a015529
14. Leonard, DA, Kurtz, JM, Mallard, C, Albritton, A, DuRan-StRuuck, R, Farkash, EA, et al. Vascularized Composite Allograft Tolerance across MHC Barriers in a Large Animal Model. Am J Transplant (2014) 14(2):343–55. doi:10.1111/ajt.12560
15. Barth, RN, Rodriguez, ED, Mundinger, GS, Nam, AJ, Ha, JS, Hui-Chou, H, et al. Vascularized Bone Marrow-Based Immunosuppression Inhibits Rejection of Vascularized Composite Allografts in Nonhuman Primates. Am J Transplant (2011) 11(7):1407–16. doi:10.1111/j.1600-6143.2011.03551.x
16. Schneeberger, S, Gorantla, VS, Brandacher, G, Zeevi, A, Demetris, AJ, Lunz, JG, et al. Upper-Extremity Transplantation Using a Cell-Based Protocol to Minimize Immunosuppression. Ann Surg (2013) 257(2):345–51. doi:10.1097/SLA.0b013e31826d90bb
17. Johnstone, BH, Messner, F, Brandacher, G, and Woods, EJ. A Large-Scale Bank of Organ Donor Bone Marrow and Matched Mesenchymal Stem Cells for Promoting Immunomodulation and Transplant Tolerance. Front Immunol (2021) 12:622604. doi:10.3389/fimmu.2021.622604
18. Kuo, Y-R, Chen, C-C, Goto, S, Lin, P-Y, Wei, F-C, and Chen, C-L. Mesenchymal Stem Cells as Immunomodulators in a Vascularized Composite Allotransplantation. Clin Dev Immunol (2012) 2012:854846–8. doi:10.1155/2012/854846
19. Heyes, R, Iarocci, A, Tchoukalova, Y, and Lott, DG. Immunomodulatory Role of Mesenchymal Stem Cell Therapy in Vascularized Composite Allotransplantation. J Transpl (2016) 2016:6951693–10. doi:10.1155/2016/6951693
20. Vyas, K, Mohan, A, Morrison, S, Tran, D, and Mardini, S. Cell-Based Therapies in Vascularized Composite Allotransplantation. J Reconstr Microsurg (2018) 34(08):642–50. doi:10.1055/s-0038-1661336
21. Kauke-Navarro, M, Knoedler, S, Panayi, AC, Knoedler, L, Noel, OF, and Pomahac, B. Regulatory T Cells: Liquid and Living Precision Medicine for the Future of VCA. Transplantation (2023) 107(1):86–97. doi:10.1097/TP.0000000000004342
22. Qu, G, Chen, J, Li, Y, Yuan, Y, Liang, R, and Li, B. Current Status and Perspectives of Regulatory T Cell-Based Therapy. J Genet Genomics (2022) 49(7):599–611. doi:10.1016/j.jgg.2022.05.005
23. Schutte-Nutgen, K, Tholking, G, Suwelack, B, and Reuter, S. Tacrolimus - Pharmacokinetic Considerations for Clinicians. Curr Drug Metab (2018) 19(4):342–50. doi:10.2174/1389200219666180101104159
24. Page, EK, Dar, WA, and Knechtle, SJ. Tolerogenic Therapies in Transplantation. Front Immunol (2012) 3:198. doi:10.3389/fimmu.2012.00198
25. Mathes, DW, Noland, M, Graves, S, Schlenker, R, Miwongtum, T, and Storb, R. A Preclinical Canine Model for Composite Tissue Transplantation. J Reconstr Microsurg (2010) 26(3):201–7. doi:10.1055/s-0030-1247717
26. Pober, JS, Merola, J, Liu, R, and Manes, TD. Antigen Presentation by Vascular Cells. Front Immunol (2017) 8:1907. doi:10.3389/fimmu.2017.01907
27. Thelemann, C, Haller, S, Blyszczuk, P, Kania, G, Rosa, M, Eriksson, U, et al. Absence of Nonhematopoietic MHC Class II Expression Protects Mice from Experimental Autoimmune Myocarditis. Eur J Immunol (2016) 46(3):656–64. doi:10.1002/eji.201545945
28. Shengwu, Z, Qingfeng, L, Hao, J, Banich, J, Kaiding, F, Benson, C, et al. Developing a Canine Model of Composite Facial/Scalp Allograft Transplantation. Ann Plast Surg (2007) 59(2):185–94. doi:10.1097/SAP.0b013e31802c79a5
29. Brandacher, G, Grahammer, J, Sucher, R, and Lee, W-PA. Animal Models for Basic and Translational Research in Reconstructive Transplantation. Birth Defects Res C Embryo Today (2012) 96(1):39–50. doi:10.1002/bdrc.21002
30. Lin, CH, Wang, YL, Anggelia, MR, Chuang, WY, Cheng, HY, Mao, Q, et al. Combined Anti-CD154/CTLA4Ig Costimulation Blockade-Based Therapy Induces Donor-specific Tolerance to Vascularized Osteomyocutaneous Allografts. Am J Transplant (2016) 16(7):2030–41. doi:10.1111/ajt.13694
31. Anggelia, MR, Cheng, HY, Chuang, WY, Hsieh, YH, Wang, AYL, Lin, CH, et al. Unraveling the Crucial Roles of FoxP3+ Regulatory T Cells in Vascularized Composite Allograft Tolerance Induction and Maintenance. Transplantation (2021) 105(6):1238–49. doi:10.1097/TP.0000000000003509
32. Lin, C-H, Anggelia, MR, Cheng, HY, Wang, AYL, Chuang, WY, Lin, CH, et al. The Intragraft Vascularized Bone Marrow Component Plays a Critical Role in Tolerance Induction after Reconstructive Transplantation. Cell Mol Immunol (2021) 18(2):363–73. doi:10.1038/s41423-019-0325-y
33. Oh, BC, Furtmuller, GJ, Fryer, ML, Guo, Y, Messner, F, Krapf, J, et al. Vascularized Composite Allotransplantation Combined with Costimulation Blockade Induces Mixed Chimerism and Reveals Intrinsic Tolerogenic Potential. JCI Insight (2020) 5(7):e128560. doi:10.1172/jci.insight.128560
34. Lin, CH, Zhang, W, Ng, TW, Zhang, D, Jiang, J, Pulikkottil, B, et al. Vascularized Osteomyocutaneous Allografts Are Permissive to Tolerance by Induction-Based Immunomodulatory Therapy. Am J Transpl (2013) 13(8):2161–8. doi:10.1111/ajt.12275
35. Davis, TA, Anam, K, Lazdun, Y, Gimble, JM, and Elster, EA. Adipose-derived Stromal Cells Promote Allograft Tolerance Induction. Stem Cell Transl Med (2014) 3(12):1444–50. doi:10.5966/sctm.2014-0131
36. Ramirez, AE, Cheng, HY, Lao, WW, Wang, YL, Wen, CJ, Wallace, CG, et al. A Novel Rat Full-Thickness Hemi-Abdominal wall/hindlimb Osteomyocutaneous Combined Flap: Influence of Allograft Mass and Vascularized Bone Marrow Content on Vascularized Composite Allograft Survival. Transpl Int (2014) 27(9):977–86. doi:10.1111/tri.12364
37. Jindal, R, Unadkat, J, Zhang, W, Zhang, D, Ng, TW, Wang, Y, et al. Spontaneous Resolution of Acute Rejection and Tolerance Induction with IL-2 Fusion Protein in Vascularized Composite Allotransplantation. Am J Transplant (2015) 15(5):1231–40. doi:10.1111/ajt.13118
38. Plock, JA, Schnider, JT, Zhang, W, Schweizer, R, Tsuji, W, Kostereva, N, et al. Adipose- and Bone Marrow–Derived Mesenchymal Stem Cells Prolong Graft Survival in Vascularized Composite Allotransplantation. Transplantation (2015) 99(9):1765–73. doi:10.1097/TP.0000000000000731
39. Cheng, H-Y, Ghetu, N, Huang, WC, Wang, YL, Wallace, CG, Wen, CJ, et al. Syngeneic Adipose-Derived Stem Cells with Short-Term Immunosuppression Induce Vascularized Composite Allotransplantation Tolerance in Rats. Cytotherapy (2014) 16(3):369–80. doi:10.1016/j.jcyt.2013.06.020
40. Cwykiel, J, Jundzill, A, Klimczak, A, Madajka-Niemeyer, M, and Siemionow, M. Donor Recipient Chimeric Cells Induce Chimerism and Extend Survival of Vascularized Composite Allografts. Arch Immunol Ther Exp (Warsz) (2021) 69(1):13. doi:10.1007/s00005-021-00614-9
41. Siemionow, M, Rampazzo, A, Gharb, BB, Cwykiel, J, Klimczak, A, Madajka, M, et al. The Reversed Paradigm of Chimerism Induction: Donor Conditioning with Recipient-Derived Bone Marrow Cells as a Novel Approach for Tolerance Induction in Vascularized Composite Allotransplantation. Microsurgery (2016) 36(8):676–83. doi:10.1002/micr.30041
42. Ma, T, Luan, S, Tao, R, Lu, D, Guo, L, Liu, J, et al. Targeted Migration of Human Adipose-Derived Stem Cells to Secondary Lymphoid Organs Enhances Their Immunomodulatory Effect and Prolongs the Survival of Allografted Vascularized Composites. Stem Cells (2019) 37(12):1581–94. doi:10.1002/stem.3078
43. Zor, F, Bozkurt, M, Cwykiel, J, Karagoz, H, Kulahci, Y, Uygur, S, et al. The Effect of Thymus Transplantation on Donor-specific Chimerism in the Rat Model of Composite Osseomusculocutaneous Sternum, Ribs, Thymus, Pectoralis Muscles, and Skin Allotransplantation. Microsurgery (2020) 40(5):576–84. doi:10.1002/micr.30555
Keywords: Vascularized composite allotransplantation, tolerance induction, animal models, swine, rodent
Citation: Huelsboemer L, Kauke-Navarro M, Reuter S, Stoegner VA, Feldmann J, Hirsch T, Kueckelhaus M and Dermietzel A (2023) Tolerance Induction in Vascularized Composite Allotransplantation—A Brief Review of Preclinical Models. Transpl Int 36:10955. doi: 10.3389/ti.2023.10955
Received: 05 October 2022; Accepted: 24 January 2023;
Published: 09 February 2023.
Copyright © 2023 Huelsboemer, Kauke-Navarro, Reuter, Stoegner, Feldmann, Hirsch, Kueckelhaus and Dermietzel. This is an open-access article distributed under the terms of the Creative Commons Attribution License (CC BY). The use, distribution or reproduction in other forums is permitted, provided the original author(s) and the copyright owner(s) are credited and that the original publication in this journal is cited, in accordance with accepted academic practice. No use, distribution or reproduction is permitted which does not comply with these terms.
*Correspondence: Lioba Huelsboemer, lioba@huelsboemer.de
†These authors share first authorship
‡These authors share last authorship