- 1Clinic Unit of Regenerative Medicine and Organ Transplants and Diabetes Research Institute, Istituto di Ricovero e Cura a Carattere Scientifico (IRCCS) Ospedale San Raffaele, Milan, Italy
- 2Diabetes Research Institute, Università Vita-Salute San Raffaele, Milan, Italy
- 3Pancreatic Surgery, Pancreas Translational and Clinical Research Center, Istituto di Ricovero e Cura a Carattere Scientifico (IRCCS) Ospedale San Raffaele, Milan, Italy
This opinion paper evaluates the potential of porcine islets as a promising alternative in beta cell replacement therapy for Type 1 Diabetes (T1D), juxtaposed with the current limitations of human donor islets. It analyzes the compatibility of pig islets with human glucose metabolism, their prospects as a limitless and high-quality source of beta cells, and the unique immunogenic challenges they present in xenotransplantation. Additionally, the paper discusses the regulatory and ethical considerations pertinent to the use of porcine islets. By synthesizing current research and expert perspectives, the paper highlights both the opportunities and significant barriers that need addressing to advance pig islets as a viable therapeutic option. The findings advocate for a balanced and forward-looking approach to the integration of pig islets in T1D treatment, underscoring the need for continued research and dialogue in this evolving field.
Introduction
The path to curing Type 1 Diabetes (T1D) through beta cell replacement is filled with both promise and complexity [1, 2]. At its core, the strategy is simple and compelling: by restoring the insulin-producing function of pancreatic beta cells, one can tackle T1D at its root [3]. The use of islets from organ donors in these therapies has convincingly shown that such an approach can significantly improve or even temporarily reverse diabetes [4–8]. Yet, this achievement also highlights the critical hurdles that need to be cleared to reach a universal cure. The main challenges in beta cell replacement therapies involve finding a limitless supply of beta cells, reducing or avoiding the need for immunosuppressive drugs, and ensuring the transplanted cells survive and function over the long term [9]. The reliance on donor islets is severely limited by donor scarcity, variable islet quality, and the complexities tied to working with primary culture cells, which together pose a significant challenge to the broad applicability of beta cell replacement methods. The pursuit of alternative sources of cells holds potential; however, these alternatives should deliver distinct advantages over current options in terms of availability, quality consistency, and ease of handling to truly transform the landscape of beta cell replacement therapies. Given these foundational challenges, it becomes crucial to consider if pig islets could potentially offer advantages over traditional organ donor sources or alternative strategies, such as those involving the differentiation of pluripotent stem cells [10]. Addressing this issue effectively requires answering some key questions.
How Compatible is the Glucose Metabolism Regulation of Pig Islets With Human Physiology for Transplantation Purposes?
The critical prerequisite for evaluating pig islets for transplantation is that the regulation of glucose metabolism by the pig islet must be compatible with human physiology [11]. While pig and human insulin are remarkably similar, differing only in the 30th amino acid of the β-chain, and despite the historical use of porcine insulin in treating human diabetes, there are differences in how porcine islets respond to glucose and other stimuli compared to human islets (summarize in Table 1) [12–22, 24–26]. Although the precise long-term consequences of these differences in pig islet function remain uncertain, they seem to align with the requirements for a short to medium-term approach.
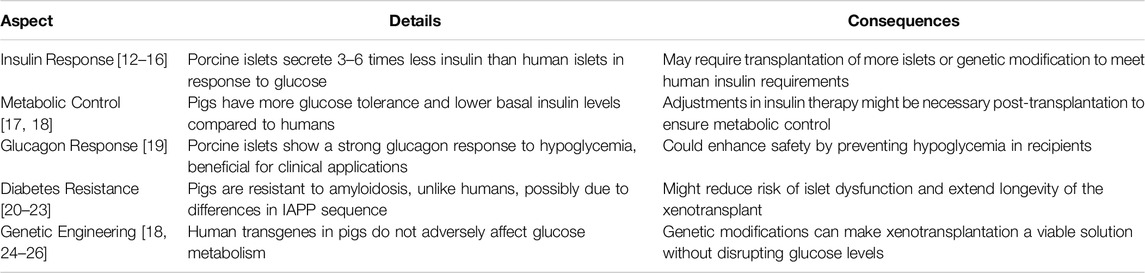
Table 1. Comparative analysis of porcine and human islet function and potential in xenotransplantation.
Is the Supply of Insulin-Producing Cells From Pig Islets a Limitless and Consistently High-Quality Source?
Bypassing the obstacles related to genetic adjustments and the circumvention of immune system rejection, the notion of pig islets serving as an endless and consistently superior source of insulin-producing cells encounters multiple critical areas in need of continuous improvement [27]. It is imperative to ensure the uniform quality and longevity of islet cells across various preparations, which mandates the formulation of uniform protocols for the extraction, refinement, and preservation of pig islets to maintain their operational effectiveness and durability post-transplant [28–30]. Additionally, the ability to upscale the production of pig islets poses an essential challenge [31, 32]. This encompasses the initiation of responsible and ethical practices in pig rearing, the advancement of proficient methods for islet extraction, and the creation of effective logistical solutions for their distribution [33]. These factors need to be refined to address the worldwide demand for insulin-producing cells while safeguarding animal wellbeing and ecological integrity. The ongoing debate (summarize in Table 2), indicating that after extensive research there still has not been a consensus on the most suitable pig age and strain for providing sufficiently viable isolated islet cells for clinical xenotransplantation [47–50], underscores the current limitations in achieving a limitless and consistent supply [51].
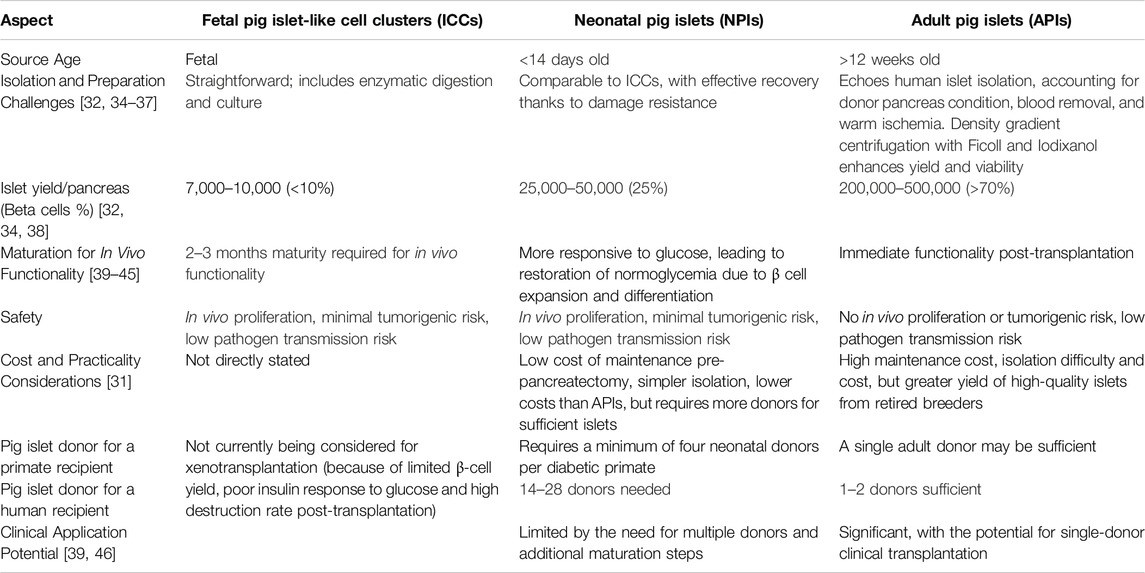
Table 2. Advantages and disadvantages of fetal, neonatal and adult pig islets for clinical xenotransplantation.
Do Pig Islet Cells Possess Inherent Advantages in Terms of Immunogenicity for Xenotransplantation Purposes?
In principle, xenotransplantation of pig islets could present a less specific target for the recipient’s autoimmune response compared to human islets, due to the differences in cellular antigens between species. Thus, while the pig islet cells could still be recognized as foreign by the recipient’s immune system, they might not be specifically targeted by the autoreactive T cells that are involved in the autoimmune attack on native pancreatic beta cells. However, this potential advantage is complicated by the broad immune response against xenogeneic tissue, which includes not only adaptive immune responses [52–56] but also innate responses [57] and issues like the instant blood-mediated inflammatory reaction (IBMIR) [58–63], hyperacute rejection, and acute cellular rejection. These xenogeneic reactions can be strong and present significant barriers to the long-term survival and function of the transplanted islets. Furthermore, cross-reactivity between swine leukocyte antigen (SLA) and human anti-HLA-specific antibodies is another factor that complicates xenotransplantation [64]. It is well-known that human anti-HLA antibodies, especially in sensitized individuals, can sometimes bind to SLA molecules due to structural similarities [65, 66]. This cross-reactivity can lead to both innate and adaptive immune responses, potentially causing early rejection of the pig islets. While the basic concept of cross-reactivity is established and supported by various experimental studies [67–70], the extent to which cross-reactivity impacts clinical outcomes is still debated [71, 72]. The predictability of which specific human antibodies will cross-react with SLA, and how this cross-reactivity might vary among different individuals or pig donors, remains an area of ongoing research [73, 74]. There is also debate about the best strategies to mitigate these risks, including whether genetic modifications to reduce SLA expression or to incorporate human-like antigens in pigs are sufficient to prevent cross-reactivity [75]. Thus, while pig islet cells might have certain inherent advantages in terms of reduced targeting by autoreactive T cells, the risk of cross-reactivity with pre-existing anti-HLA antibodies adds a significant layer of complexity to the immunogenicity of xenotransplanted pig islets.
Are Methods to Alleviate Xenogeneic Rejection More Feasible or Practical Compared to Those Used for Other Transplant Sources?
Alleviating xenogeneic rejection involves several unique and sophisticated approaches due to the significant biological differences between species. These methods can be categorized into three main strategies: gene editing of the donor (e.g., pigs), pharmacological immunosuppression, and physical barriers such as encapsulation. Focusing specifically on gene modification efforts, pig islet xenotransplantation indeed represents the most extensive application of genetic engineering in the realm of islet transplantation (Table 3) [91]. The most extensively modified animals have undergone 69 genomic alterations, which include the removal of glycan antigens, the enhancement of human transgene expression, and the deactivation of porcine endogenous retroviruses [92]. This extensive level of genetic editing is primarily possible because of the wider array of modifications that are both ethically and technically feasible in pigs, in contrast to what is possible with human islets or stem cells. Conversely, evidence suggests that traditional immunosuppressive treatments, such as those based on tacrolimus, are less effective at managing the adaptive immune response against pig xenografts [93]. Instead, targeting the CD40/CD154 T-cell co-stimulation pathway has shown greater efficacy [94]. Notably, islets from adult wild-type (non-genetically modified) pigs have successfully functioned in diabetic non-human primates (NHPs) treated with anti-CD154 monoclonal antibody-based immunosuppression for up to 965 days [95]. Nonetheless, genetically editing pigs could potentially achieve comparable or superior outcomes with less aggressive immunosuppression (Table 4). The concern, however, lies in the intensity of immunosuppressive protocols required, particularly for conditions requiring long-term management like diabetes. Islet encapsulation may offer greater immediate benefits for pig islet transplantation compared to human islets [110]. This is due to pig islets’ higher immunogenicity and the ample supply they offer, which is critical for overcoming xenotransplantation’s unique challenges [111]. While both pig and human islet transplants can benefit from encapsulation technologies, the necessity and feasibility of these strategies might be more pronounced for pig islets to ensure successful transplantation outcomes. Although significant progress has been made [112–114], most strategies are yet to meet the criteria of obtaining sustainable and consistent diabetes management for >6 months in preclinical trials before they can be introduced in human clinical trials.
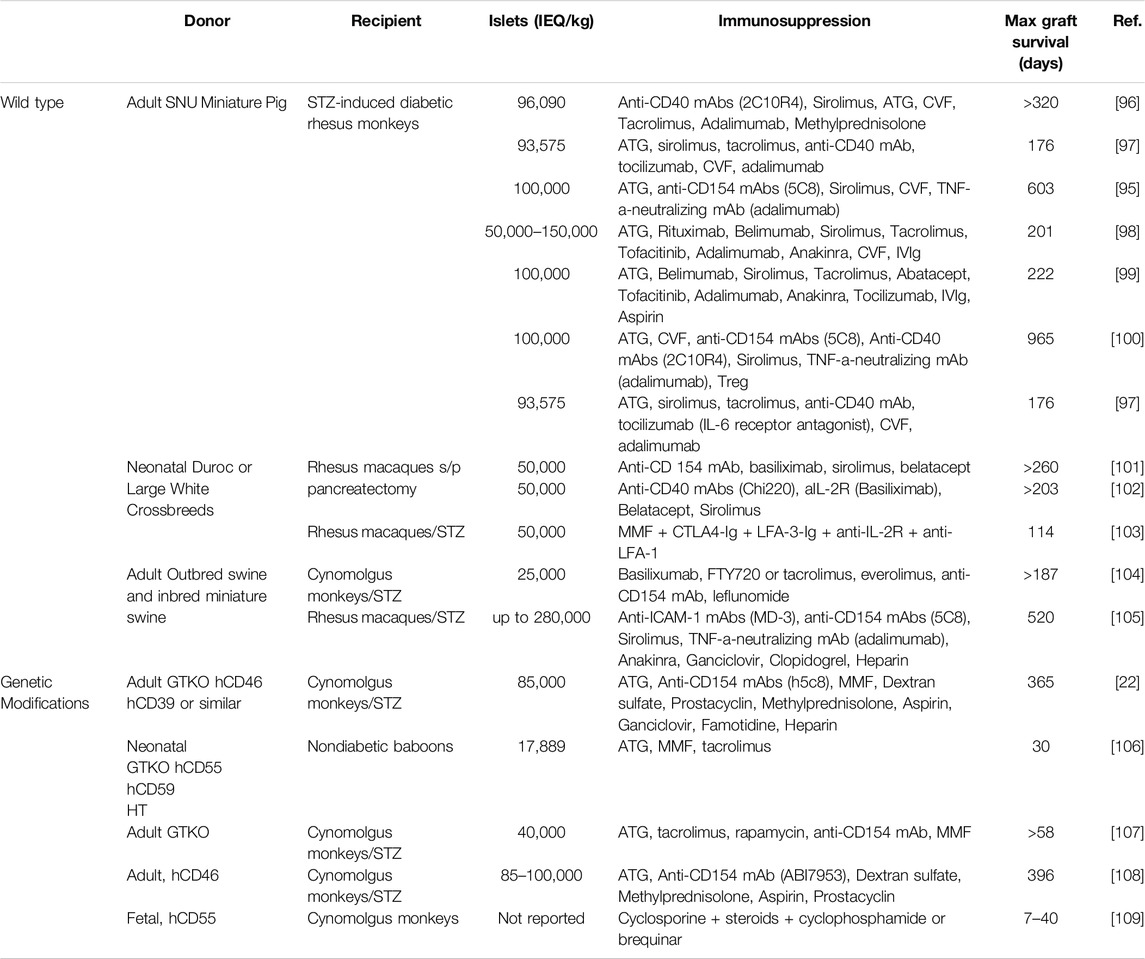
Table 4. Immunosuppressive protocols associated with prolonged periods of insulin-independence and islet xenograft survival.
Is There Evidence That Pig Islets Are More Effective in Regulating Human Blood Sugar Levels Compared to Other Sources in Clinical Trials?
Beyond the pioneering studies by Groth et al. [115] and Wang et al. [116], which examined free islet xenotransplantation, there’s a notable scarcity of clinical trials in this area (Table 5). Instead, much of the focus has been on encapsulated islets transplanted without the need for immunosuppression [117]. These endeavors have yet to achieve unequivocal success. In several instances, improvements in blood sugar management could be attributed to rigorous medical oversight, including dietary changes, strict glucose monitoring, and specialized healthcare, rather than the transplanted pig islets themselves. However, research led by Matsumoto et al. stands out, having successfully reduced HbA1c levels significantly over 600 days in recipients of encapsulated pig islets, without resorting to immunosuppressive medications [118, 119]. Despite minimal side effects reported, the quest for more dependable and enhanced results continues. When these findings are weighed against the outcomes from human islet and stem cell-derived islet transplants, it’s clear that transplants using human islets are currently the most effective for clinical management of diabetes, primarily due to their significant impact on glycemic control [6]. While pig islet xenotransplantation holds potential and has shown varied degrees of success, it still needs further development for it to be consistently reliable and widely applicable. On the other hand, islets derived from stem cells hold immense promise due to their potentially unlimited availability and fewer compatibility challenges. However, while their effectiveness has been shown in limited trials [120], confirmation of their clinical efficacy is still awaiting results from larger-scale studies.
Is There Evidence to Suggest that Porcine Islets Are Safer and More Regulatorily Straightforward Compared to Alternative Sources?
The safety and regulatory ease of using porcine islets compared to other sources such as human islets or stem cell-derived islets are areas of active research and debate. Adult porcine pancreatic islets could represent a safer and more effective alternative for therapeutic use than stem cells, due to their complete and immediate hormonal compatibility with human physiology and lower oncogenic risk, as they skip the need for differentiation and do not proliferate. While neonatal and fetal pig islets are less ideal due to their immature and proliferative characteristics, they likely still pose a lower oncogenic risk than stem cells. On the other hand, porcine pancreatic islets raise concerns regarding the risk of infectious disease transmission. Porcine islets carry the risk of transmitting porcine endogenous retroviruses (PERVs) to humans. However, extensive research and clinical trials have shown no evidence of PERV transmission so far, which is encouraging for their safety profile [121–125]. Nonetheless, the long-term risks of zoonotic disease transmission remain a concern that requires ongoing surveillance [126]. One of the key challenges limiting the widespread adoption of human islet allo-transplantation in countries like the USA is the stringent regulatory framework [127]. While porcine islets are subject to established xenotransplantation guidelines, which include rigorous safety and ethical evaluations, it is not entirely clear if they are better positioned to meet these requirements compared to human islets. Genetic modifications to reduce xenoreactivity and address safety concerns are promising but their effectiveness in fully satisfying regulatory criteria remains to be confirmed. Additionally, while regulatory frameworks for xenotransplantation aim to facilitate approval, the comparative ease with which porcine islets might navigate these requirements versus human islets is still uncertain. Ongoing research and regulatory developments will be essential to determining whether porcine islets can more effectively meet these stringent standards. Lastly, the employment of porcine islets in certain contexts could be perceived as more ethically permissible than the use of islets derived from stem cells, contingent upon the origins of these stem cells. Specifically, the ethical controversies surrounding the utilization of embryonic stem cells are not relevant to porcine islets. Nonetheless, employing animal organs and tissues introduces distinct ethical challenges, such as those pertaining to the welfare of animals involved in breeding and procurement processes, as well as cultural and religious considerations. Regulatory agencies have established frameworks for xenotransplantation [128], which includes the transplantation of porcine islets. These frameworks address both safety and ethical issues, but they also mean that porcine islets must undergo rigorous preclinical and clinical testing to prove their safety and efficacy. In comparison, human islets and stem cell-derived islets, while also subject to strict regulatory scrutiny, are not confronted with the same level of concern regarding zoonotic disease transmission.
Conclusion
The quest for a cure for T1D through beta cell replacement therapy encompasses a dynamic interplay of potential and complexities. The efficacy of islet transplantation from human donors underscores the foundational promise of this approach by directly addressing T1D’s root cause. However, the realization of a universal cure is hindered by several critical challenges: the quest for an inexhaustible and high-quality source of beta cells, the minimization or elimination of reliance on immunosuppressive drugs, and the assurance of the long-term viability and functionality of the transplanted cells. Alternative sources such as porcine islets and stem cell-derived islets offer intriguing possibilities, each with its unique set of advantages and challenges. Porcine islets, while providing a potentially unlimited supply, raise concerns regarding physiological compatibility, immunogenicity, and regulatory complexities. On the other hand, stem cell-derived islets, benefiting from advancements in cellular reprogramming and differentiation, appear to have a slight edge in current discussions, primarily due to their potential for unlimited supply and reduced ethical concerns compared to embryonic stem cells. However, the recent successes in whole organ xenotransplantation inject a renewed vigor into the exploration of porcine islets [129–135]. These advancements may pave the way for addressing some of the longstanding issues in a relatively short timeframe, particularly those related to immunogenicity and physiological compatibility [136]. This progress, alongside the ongoing refinement of techniques for stem cell-derived islets, underscores a dynamic research landscape. Thus, the future of beta cell replacement therapy for T1D remains an open field of scientific inquiry and innovation. It is propelled by the collective goal of developing a comprehensive, effective cure, navigating through the interwoven challenges of supply, compatibility, safety, and regulatory acceptance. The path forward is marked by the potential of recent breakthroughs and the promise of overcoming current limitations through concerted research efforts.
Author Contributions
LP conceptualized the review, led the project, and reviewed the manuscript. AC analyzed literature and contributed to manuscript drafting and revisions. VT collected literature and assisted in drafting the manuscript. SP provided critical revisions focused on clinical insights. RC provided critical revisions focused on clinical insights and critically reviewed the manuscript. All authors approved the final manuscript.
Funding
The author(s) declare that no financial support was received for the research, authorship, and/or publication of this article.
Conflict of Interest
The authors declare that the research was conducted in the absence of any commercial or financial relationships that could be construed as a potential conflict of interest.
References
1. Shapiro, AMJ, and Verhoeff, K. A Spectacular Year for Islet and Stem Cell Transplantation. Nat Rev Endocrinol (2023) 19:68–9. doi:10.1038/s41574-022-00790-4
2. Vantyghem, MC, de Koning, EJP, Pattou, F, and Rickels, MR. Advances in Beta-Cell Replacement Therapy for the Treatment of Type 1 Diabetes. Lancet (2019) 394:1274–85. doi:10.1016/S0140-6736(19)31334-0
3. Piemonti, L. Felix Dies Natalis, Insulin. Ceterum Autem Censeo “Beta Is Better”. Acta Diabetol (2021) 58:1287–306. doi:10.1007/s00592-021-01737-3
4. Marfil-Garza, BA, Hefler, J, Verhoeff, K, Lam, A, Dajani, K, Anderson, B, et al. Pancreas and Islet Transplantation: Comparative Outcome Analysis of a Single-Centre Cohort Over 20-Years. Ann Surg (2023) 277:672–80. doi:10.1097/SLA.0000000000005783
5. Rickels, MR, Eggerman, TL, Bayman, L, Qidwai, JC, Alejandro, R, Bridges, ND, et al. Long-Term Outcomes With Islet-Alone and Islet-After-Kidney Transplantation for Type 1 Diabetes in the Clinical Islet Transplantation Consortium: The CIT-08 Study. Diabetes Care (2022) 45:2967–75. doi:10.2337/dc21-2688
6. Marfil-Garza, BA, Imes, S, Verhoeff, K, Hefler, J, Lam, A, Dajani, K, et al. Pancreatic Islet Transplantation in Type 1 Diabetes: 20-Year Experience From a Single-Centre Cohort in Canada. Lancet Diabetes Endocrinol (2022) 10:519–32. doi:10.1016/S2213-8587(22)00114-0
7. Vantyghem, M-C, Chetboun, M, Gmyr, V, Jannin, A, Espiard, S, Le Mapihan, K, et al. Ten-Year Outcome of Islet Alone or Islet After Kidney Transplantation in Type 1 Diabetes: A Prospective Parallel-Arm Cohort Study. Diabetes Care (2019) 42:2042–9. doi:10.2337/dc19-0401
8. Lablanche, S, Borot, S, Wojtusciszyn, A, Skaare, K, Penfornis, A, Malvezzi, P, et al. Ten-Year Outcomes of Islet Transplantation in Patients With Type 1 Diabetes: Data From the Swiss-French GRAGIL Network. Am J Transpl (2021) 21:3725–33. doi:10.1111/ajt.16637
9. Caldara, R, Tomajer, V, Monti, P, Sordi, V, Citro, A, Chimienti, R, et al. Allo Beta Cell Transplantation: Specific Features, Unanswered Questions, and Immunological Challenge. Front Immunol (2023) 14:1323439. doi:10.3389/fimmu.2023.1323439
10. Ekser, B, and Cooper, DK. Overcoming the Barriers to Xenotransplantation: Prospects for the Future. Expert Rev Clin Immunol (2010) 6:219–30. doi:10.1586/eci.09.81
11. Cooper, DKC, Mou, L, and Bottino, R. A Brief Review of the Current Status of Pig Islet Xenotransplantation. Front Immunol (2024) 15:1366530. doi:10.3389/fimmu.2024.1366530
12. Cooper, DK, Matsumoto, S, Abalovich, A, Itoh, T, Mourad, NI, Gianello, PR, et al. Progress in Clinical Encapsulated Islet Xenotransplantation. Transplantation (2016) 100:2301–8. doi:10.1097/TP.0000000000001371
13. Krickhahn, M, Meyer, T, Bühler, C, Thiede, A, and Ulrichs, K. Highly Efficient Isolation of Porcine Islets of Langerhans for Xenotransplantation: Numbers, Purity, Yield and In Vitro Function. Ann Transplant (2001) 6:48–54.
14. Mueller, KR, Balamurugan, A, Cline, GW, Pongratz, RL, Hooper, RL, Weegman, BP, et al. Differences in Glucose-stimulated Insulin Secretion In Vitro of Islets From Human, Nonhuman Primate, and Porcine Origin. Xenotransplantation (2013) 20:75–81. doi:10.1111/xen.12022
15. Mourad, NI, Nenquin, M, and Henquin, J-C. cAMP-Mediated and Metabolic Amplification of Insulin Secretion Are Distinct Pathways Sharing Independence of β-Cell Microfilaments. Endocrinology (2012) 153:4644–54. doi:10.1210/en.2012-1450
16. Mourad, NI, Nenquin, M, and Henquin, J-C. Amplification of Insulin Secretion by Acetylcholine or Phorbol Ester Is Independent of β-Cell Microfilaments and Distinct From Metabolic Amplification. Mol Cell Endocrinol (2013) 367:11–20. doi:10.1016/j.mce.2012.12.002
17. Manell, E, Hedenqvist, P, Svensson, A, and Jensen-Waern, M. Establishment of a Refined Oral Glucose Tolerance Test in Pigs, and Assessment of Insulin, Glucagon and Glucagon-Like Peptide-1 Responses. PloS one (2016) 11:e0148896. doi:10.1371/journal.pone.0148896
18. Casu, A, Bottino, R, Balamurugan, A, Hara, H, Van Der Windt, D, Campanile, N, et al. Metabolic Aspects of Pig-To-Monkey (Macaca fascicularis) Islet Transplantation: Implications for Translation Into Clinical Practice. Diabetologia (2008) 51:120–9. doi:10.1007/s00125-007-0844-4
19. Mourad, NI, Xhema, D, and Gianello, P. In Vitro Assessment of Pancreatic Hormone Secretion From Isolated Porcine Islets. Front Endocrinol (2022) 13:935060. doi:10.3389/fendo.2022.935060
20. Gerstein, HC, and Waltman, L. Why Don’t Pigs Get Diabetes? Explanations for Variations in Diabetes Susceptibility in Human Populations Living in a Diabetogenic Environment. Cmaj (2006) 174:25–6. doi:10.1503/cmaj.050649
21. Potter, K, Abedini, A, Marek, P, Klimek, A, Butterworth, S, Driscoll, M, et al. Islet Amyloid Deposition Limits the Viability of Human Islet Grafts But Not Porcine Islet Grafts. Proc Natl Acad Sci (2010) 107:4305–10. doi:10.1073/pnas.0909024107
22. Bottino, R, Wijkstrom, M, van Der Windt, D, Hara, H, Ezzelarab, M, Murase, N, et al. Pig-to-Monkey Islet Xenotransplantation Using Multi-Transgenic Pigs. Am J Transplant (2014) 14:2275–87. doi:10.1111/ajt.12868
23. Potter, KJ, Abedini, A, Marek, P, Klimek, AM, Butterworth, S, Driscoll, M, et al. Islet Amyloid Deposition Limits the Viability of Human Islet Grafts But Not Porcine Islet Grafts. Proc Natl Acad Sci U S A (2010) 107:4305–10. doi:10.1073/pnas.0909024107
24. Casu, A, Echeverri, GJ, Bottino, R, Van Der Windt, DJ, He, J, Ekser, B, et al. Insulin Secretion and Glucose Metabolism in Alpha 1, 3-Galactosyltransferase Knock-Out Pigs Compared to Wild-Type Pigs. Xenotransplantation (2010) 17:131–9. doi:10.1111/j.1399-3089.2010.00572.x
25. Wijkstrom, M, Bottino, R, Iwase, H, Hara, H, Ekser, B, Van Der Windt, D, et al. Glucose Metabolism in Pigs Expressing Human Genes Under an Insulin Promoter. Xenotransplantation (2015) 22:70–9. doi:10.1111/xen.12145
26. Salama, A, Mosser, M, Lévêque, X, Perota, A, Judor, J-P, Danna, C, et al. Neu5Gc and α1-3 GAL Xenoantigen Knockout Does Not Affect Glycemia Homeostasis and Insulin Secretion in Pigs. Diabetes (2017) 66:987–93. doi:10.2337/db16-1060
27. Smood, B, Bottino, R, and Cooper, DK. Xenotransplantation of the Endocrine Pancreas. Transplant Bioeng Regen Endocr pancreas (2020) 423–46. doi:10.1016/b978-0-12-814831-0.00031-2
28. Kwak, K, Park, J, Shim, J, Ko, N, Kim, HJ, Lee, Y, et al. Comparison of Islet Isolation Result and Clinical Applicability According to GMP-Grade Collagenase Enzyme Blend in Adult Porcine Islet Isolation and Culture. Xenotransplantation (2021) 28:e12703. doi:10.1111/xen.12703
29. Marchetti, P, Finke, EH, Gerasimidi-Vazeou, A, Falqui, L, Scharp, DW, and Lacy, PE. Automated Large-Scale Isolation, In Vitro Function and Xenotransplantation of Porcine Islets of Langerhans. Transplantation (1991) 52:209–13. doi:10.1097/00007890-199108000-00005
30. Rijkelijkhuizen, JK, van der Burg, MP, Töns, A, Terpstra, OT, and Bouwman, E. Pretransplant Culture Selects for High-Quality Porcine Islets. Pancreas (2006) 32:403–7. doi:10.1097/01.mpa.0000220866.87658.b2
31. Vanderschelden, R, Sathialingam, M, Alexander, M, and Lakey, JR. Cost and Scalability Analysis of Porcine Islet Isolation for Islet Transplantation: Comparison of Juvenile, Neonatal and Adult Pigs. Cel Transplant (2019) 28:967–72. doi:10.1177/0963689719847460
32. Socci, C, Bertuzzi, F, De Nittis, P, Piemonti, L, Taglietti, M, Berra, C, et al. Isolation and Function of Adult Pig Islets. Xenotransplantation (1995) 2:218–21. doi:10.1111/j.1399-3089.1995.tb00098.x
33. Dufrane, D, and Gianello, P. Pig Islets for Clinical Islet Xenotransplantation. Curr Opin Nephrol Hypertens (2009) 18:495–500. doi:10.1097/MNH.0b013e328331a8e3
34. Korbutt, GS, Elliott, JF, Ao, Z, Smith, DK, Warnock, GL, and Rajotte, RV. Large Scale Isolation, Growth, and Function of Porcine Neonatal Islet Cells. J Clin Invest (1996) 97:2119–29. doi:10.1172/JCI118649
35. Yonekawa, Y, Matsumoto, S, Okitsu, T, Arata, T, Iwanaga, Y, Noguchi, H, et al. Effective Islet Isolation Method With Extremely High Islet Yields From Adult Pigs. Cel Transplant (2005) 14:757–62. doi:10.3727/000000005783982512
36. Rajotte, RV. Isolation and Assessment of Islet Quality. Xenotransplantation (2008) 15:93–5. doi:10.1111/j.1399-3089.2008.00459.x
37. Jin, SM, Shin, JS, Kim, KS, Gong, CH, Park, SK, Kim, JS, et al. Islet Isolation From Adult Designated Pathogen-Free Pigs: Use of the Newer Bovine Nervous Tissue–Free Enzymes and a Revised Donor Selection Strategy Would Improve the Islet Graft Function. Xenotransplantation (2011) 18:369–79. doi:10.1111/j.1399-3089.2011.00677.x
38. Ricordi, C, Socci, C, Davalli, A, Staudacher, C, Baro, P, Vertova, A, et al. Isolation of the Elusive Pig Islet. Surgery (1990) 107:688–94.
39. Hardikar, AA, Wang, XY, Williams, LJ, Kwok, J, Wong, R, Yao, M, et al. Functional Maturation of Fetal Porcine β-Cells by Glucagon-Like Peptide 1 and Cholecystokinin. Endocrinology (2002) 143:3505–14. doi:10.1210/en.2001-211344
40. Otonkoski, T, Ustinov, J, Rasilainen, S, Kallio, E, Korsgren, O, and Häyry, P. Differentiation and Maturation of Porcine Fetal Islet Cells In Vitro and After Transplantation. Transplantation (1999) 68:1674–83. doi:10.1097/00007890-199912150-00010
41. Korsgren, O, Jansson, L, Eizirik, D, and Andersson, A. Functional and Morphological Differentiation of Fetal Porcine Islet-Like Cell Clusters After Transplantation Into Nude Mice. Diabetologia (1991) 34:379–86. doi:10.1007/BF00403174
42. Bogdani, M, Suenens, K, Bock, T, Pipeleers-Marichal, M, In’t Veld, P, and Pipeleers, D. Growth and Functional Maturation of β-Cells in Implants of Endocrine Cells Purified From Prenatal Porcine Pancreas. Diabetes (2005) 54:3387–94. doi:10.2337/diabetes.54.12.3387
43. Trivedi, N, Hollister-Lock, J, Lopez-Avalos, M, O’Neil, J, Keegan, M, Bonner-Weir, S, et al. Increase in β-Cell Mass in Transplanted Porcine Neonatal Pancreatic Cell Clusters Is Due to Proliferation of β-Cells and Differentiation of Duct Cells. Endocrinology (2001) 142:2115–22. doi:10.1210/endo.142.5.8162
44. Yoon, K-H, Quickel, RR, Tatarkiewicz, K, Ulrich, TR, Hollister-Lock, J, Trivedi, N, et al. Differentiation and Expansion of Beta Cell Mass in Porcine Neonatal Pancreatic Cell Clusters Transplanted Into Nude Mice. Cel Transplant (1999) 8:673–89. doi:10.1177/096368979900800613
45. Weir, G, Quickel, R, Yoon, K, Tatarkiewicz, K, Ulrich, T, Hollister-Lock, J, et al. Porcine Neonatal Pancreatic Cell Clusters (NPCCs): A Potential Source of Tissue for Islet Transplantation. Ann Transplant (1997) 2:63–8.
46. Söderlund, J, Wennberg, L, Castaños-Velez, E, Biberfeld, P, Zhu, S, Tibell, A, et al. Fetal Porcine Islet-Like Cell Clusters Transplanted To Cynomolgus Monkeys: An Immunohistochemical Study: 1. Transplantation (1999) 67:784–91. doi:10.1097/00007890-199903270-00002
47. Nagaraju, S, Bottino, R, Wijkstrom, M, Trucco, M, and Cooper, DK. Islet Xenotransplantation: What Is the Optimal Age of the Islet-Source Pig? Xenotransplantation (2015) 22:7–19. doi:10.1111/xen.12130
48. Bottino, R, Balamurugan, A, Smetanka, C, Bertera, S, He, J, Rood, PP, et al. Isolation Outcome and Functional Characteristics of Young and Adult Pig Pancreatic Islets for Transplantation Studies. Xenotransplantation (2007) 14:74–82. doi:10.1111/j.1399-3089.2006.00374.x
49. Prabhakaran, S, and Hering, BJ. What Strain of Pig Should Be Used? Xenotransplantation (2008) 15:83–6. doi:10.1111/j.1399-3089.2008.00456.x
50. Kim, JH, Kim, HI, Lee, KW, Yu, JE, Kim, SH, Park, HS, et al. Influence of Strain and Age Differences on the Yields of Porcine Islet Isolation: Extremely High Islet Yields From SPF CMS Miniature Pigs. Xenotransplantation (2007) 14:60–6. doi:10.1111/j.1399-3089.2006.00364.x
51. Dhanasekaran, M, George, JJ, Loganathan, G, Narayanan, S, Hughes, MG, Williams, SK, et al. Pig Islet Xenotransplantation. Curr Opin Organ Transplant (2017) 22:452–62. doi:10.1097/MOT.0000000000000455
52. Scalea, J, Hanecamp, I, Robson, SC, and Yamada, K. T-Cell-Mediated Immunological Barriers to Xenotransplantation. Xenotransplantation (2012) 19:23–30. doi:10.1111/j.1399-3089.2011.00687.x
53. Gill, R, Wolf, L, Daniel, D, and Coulombe, M. CD4+ T Cells Are Both Necessary and Sufficient for Islet Xenograft Rejection. In: Transplantation Proceedings, 1994 (1994).
54. Olack, BJ, Jaramillo, A, Benshoff, ND, Kaleem, Z, Swanson, CJ, Lowell, JA, et al. Rejection of Porcine Islet Xenografts Mediated by CD4+ T Cells Activated Through the Indirect Antigen Recognition Pathway. Xenotransplantation (2002) 9:393–401. doi:10.1034/j.1399-3089.2002.01070.x
55. Koulmanda, M, Laufer, TM, Auchincloss, JH, and Neal Smith, R. Prolonged Survival of Fetal Pig Islet Xenografts in Mice Lacking the Capacity for an Indirect Response. Xenotransplantation (2004) 11:525–30. doi:10.1111/j.1399-3089.2004.00174.x
56. Lindeborg, E, Kumagai-Braesch, M, and Möller, E. Phenotypic and Functional Characterization of Human T Cell Clones Indirectly Activated against Adult Pig Islet Cells. Xenotransplantation (2006) 13:41–52. doi:10.1111/j.1399-3089.2005.00257.x
57. Van Der Windt, DJ, Marigliano, M, He, J, Votyakova, TV, Echeverri, GJ, Ekser, B, et al. Early Islet Damage After Direct Exposure of Pig Islets to Blood: Has Humoral Immunity Been Underestimated? Cel Transplant (2012) 21:1791–802. doi:10.3727/096368912X653011
58. Nilsson, B. The Instant Blood-mediated Inflammatory Reaction in Xenogeneic Islet Transplantation. Xenotransplantation (2008) 15:96–8. doi:10.1111/j.1399-3089.2008.00460.x
59. Goto, M, Tjernberg, J, Dufrane, D, Elgue, G, Brandhorst, D, Ekdahl, KN, et al. Dissecting the Instant Blood-Mediated Inflammatory Reaction in Islet Xenotransplantation. Xenotransplantation (2008) 15:225–34. doi:10.1111/j.1399-3089.2008.00482.x
60. Bennet, W, Sundberg, B, Lundgren, T, Tibell, A, Groth, C-G, Richards, A, et al. Damage to Porcine Islets of Langerhans After Exposure to Human Blood In Vitro, or After Intraportal Transplantation To Cynomologus Monkeys: Protective Effects of sCR1 and Heparin: 1. Transplantation (2000) 69:711–9. doi:10.1097/00007890-200003150-00007
61. Liuwantara, D, Chew, YV, Favaloro, EJ, Hawkes, JM, Burns, HL, O'Connell, PJ, et al. Characterizing the Mechanistic Pathways of the Instant Blood-Mediated Inflammatory Reaction in Xenogeneic Neonatal Islet Cell Transplantation. Transplant direct (2016) 2:e77. doi:10.1097/TXD.0000000000000590
62. Nagaraju, S, Bertera, S, Tanaka, T, Hara, H, Rayat, GR, Wijkstrom, M, et al. In Vitro Exposure of Pig Neonatal Isletlike Cell Clusters to Human Blood. Xenotransplantation (2015) 22:317–24. doi:10.1111/xen.12178
63. Ji, M, Yi, S, Smith-Hurst, H, Phillips, P, Wu, J, Hawthorne, W, et al. The Importance of Tissue Factor Expression by Porcine NICC in Triggering IBMIR in the Xenograft Setting. Transplantation (2011) 91:841–6. doi:10.1097/TP.0b013e3182106091
64. Ladowski, JM, Hara, H, and Cooper, DKC. The Role of SLAs in Xenotransplantation. Transplantation (2021) 105:300–7. doi:10.1097/TP.0000000000003303
65. Naziruddin, B, Durriya, S, Phelan, D, Duffy, BF, Olack, B, Smith, D, et al. HLA Antibodies Present in the Sera of Sensitized Patients Awaiting Renal Transplant Are Also Reactive to Swine Leukocyte Antigens1, 2. Transplantation (1998) 66:1074–80. doi:10.1097/00007890-199810270-00018
66. Taylor, CJ, Tang, KG, Smith, SI, White, DJ, and Davies, HF. HLA-Specific Antibodies in Highly Sensitized Patients Can Cause a Positive Crossmatch Against Pig Lymphocytes. Transplantation (1998) 65:1634–41. doi:10.1097/00007890-199806270-00016
67. Barreau, N, Godfrin, Y, Bouhours, J-F, Bignon, J-D, Karam, G, Leteissier, E, et al. Interaction of Anti-HLA Antibodies With Pig Xenoantigens. Transplantation (2000) 69:148–56. doi:10.1097/00007890-200001150-00025
68. Varela, ID, Mozo, PS, Cortés, AC, Blanco, CA, and Can, FV. Cross-Reactivity Between Swine Leukocyte Antigen and Human Anti–HLA-Specific Antibodies in Sensitized Patients Awaiting Renal Transplantation. J Am Soc Nephrol (2003) 14:2677–83. doi:10.1097/01.asn.0000088723.07259.cf
69. Martens, GR, Reyes, LM, Butler, JR, Ladowski, JM, Estrada, JL, Sidner, RA, et al. Humoral Reactivity of Renal Transplant-Waitlisted Patients to Cells From GGTA1/CMAH/B4GalNT2, and SLA Class I Knockout Pigs. Transplantation (2017) 101:e86–e92. doi:10.1097/TP.0000000000001646
70. Ladowski, JM, Reyes, LM, Martens, GR, Butler, JR, Wang, Z-Y, Eckhoff, DE, et al. Swine Leukocyte Antigen Class II Is a Xenoantigen. Transplantation (2018) 102:249–54. doi:10.1097/TP.0000000000001924
71. Bartholomew, A, Latinne, D, Sachs, D, Arn, JS, Gianello, P, de Bruyere, M, et al. Utility of Xenografts: Lack of Correlation Between PRA and Natural Antibodies to Swine. Xenotransplantation (1997) 4:34–9. doi:10.1111/j.1399-3089.1997.tb00162.x
72. Hara, H, Ezzelarab, M, Rood, PP, Lin, YJ, Busch, J, Ibrahim, Z, et al. Allosensitized Humans Are at No Greater Risk of Humoral Rejection of GT-KO Pig Organs Than Other Humans. Xenotransplantation (2006) 13:357–65. doi:10.1111/j.1399-3089.2006.00319.x
73. Martens, GR, Ladowski, JM, Estrada, J, Wang, Z-Y, Reyes, LM, Easlick, J, et al. HLA Class I–Sensitized Renal Transplant Patients Have Antibody Binding to SLA Class I Epitopes. Transplantation (2019) 103:1620–9. doi:10.1097/TP.0000000000002739
74. Zhang, Z, Hara, H, Long, C, Iwase, H, Qi, H, Macedo, C, et al. Immune Responses of HLA Highly Sensitized and Nonsensitized Patients to Genetically Engineered Pig Cells. Transplantation (2018) 102:e195–e204. doi:10.1097/TP.0000000000002060
75. Wong, BS, Yamada, K, Okumi, M, Weiner, J, O'Malley, PE, Tseng, Y-L, et al. Allosensitization Does Not Increase the Risk of Xenoreactivity to α1, 3-Galactosyltransferase Gene-Knockout Miniature Swine in Patients on Transplantation Waiting Lists. Transplantation (2006) 82:314–9. doi:10.1097/01.tp.0000228907.12073.0b
76. Phelps, CJ, Koike, C, Vaught, TD, Boone, J, Wells, KD, Chen, S-H, et al. Production of α1, 3-Galactosyltransferase-Deficient Pigs. Science (2003) 299:411–4. doi:10.1126/science.1078942
77. Shim, J, Ko, N, Kim, H-J, Lee, Y, Lee, J-W, Jin, D-I, et al. Human Immune Reactivity of GGTA1/CMAH/A3GALT2 Triple Knockout Yucatan Miniature Pigs. Transgenic Res (2021) 30:619–34. doi:10.1007/s11248-021-00271-w
78. Cozzi, E, and White, DJ. The Generation of Transgenic Pigs as Potential Organ Donors for Humans. Nat Med (1995) 1:964–6. doi:10.1038/nm0995-964
79. Chen, RH, Naficy, S, Logan, JS, Diamond, LE, and Adams, DH. Hearts From Transgenic Pigs Constructed With CD59/DAF Genomic Clones Demonstrate Improved Survival in Primates. Xenotransplantation (1999) 6:194–200. doi:10.1034/j.1399-3089.1999.00017.x
80. Diamond, LE, Quinn, CM, Martin, MJ, Lawson, J, Platt, JL, and Logan, JS. A Human CD46 Transgenic Pig Model System for the Study of Discordant Xenotransplantation. Transplantation (2001) 71:132–42. doi:10.1097/00007890-200101150-00021
81. Ahrens, HE, Petersen, B, Ramackers, W, Petkov, S, Herrmann, D, Hauschild-Quintern, J, et al. Kidneys From α1, 3-Galactosyltransferase Knockout/Human Heme Oxygenase-1/Human A20 Transgenic Pigs Are Protected From Rejection During Ex Vivo Perfusion With Human Blood. Transplant direct (2015) 1:1–8. doi:10.1097/txd.0000000000000533
82. Mohiuddin, MM, Singh, AK, Corcoran, PC, Thomas, IIIML, Clark, T, Lewis, BG, et al. Chimeric 2C10R4 Anti-CD40 Antibody Therapy Is Critical for Long-Term Survival of GTKO. hCD46. hTBM Pig-To-Primate Cardiac Xenograft. Nat Commun (2016) 7:11138. doi:10.1038/ncomms11138
83. Costa, C, Zhao, L, Burton, WV, Bondioli, KR, Williams, BL, Hoagland, TA, et al. Expression of the Human Alpha1,2-Fucosyltransferase in Transgenic Pigs Modifies the Cell Surface Carbohydrate Phenotype and Confers Resistance to Human Serum-Mediated Cytolysis. Faseb j (1999) 13:1762–73. doi:10.1096/fasebj.13.13.1762
84. Hara, H, Witt, W, Crossley, T, Long, C, Isse, K, Fan, L, et al. Human Dominant-Negative Class II Transactivator Transgenic Pigs–Effect on the Human Anti-Pig T-cell Immune Response and Immune Status. Immunology (2013) 140:39–46. doi:10.1111/imm.12107
85. Fu, R, Fang, M, Xu, K, Ren, J, Zou, J, Su, L, et al. Generation of GGTA1-/-Β2m-/-CIITA-/- Pigs Using CRISPR/Cas9 Technology to Alleviate Xenogeneic Immune Reactions. Transplantation (2020) 104:1566–73. doi:10.1097/TP.0000000000003205
86. Wheeler, DG, Joseph, ME, Mahamud, SD, Aurand, WL, Mohler, PJ, Pompili, VJ, et al. Transgenic Swine: Expression of Human CD39 Protects Against Myocardial Injury. J Mol Cell Cardiol (2012) 52:958–61. doi:10.1016/j.yjmcc.2012.01.002
87. Buermann, A, Petkov, S, Petersen, B, Hein, R, Lucas-Hahn, A, Baars, W, et al. Pigs Expressing the Human Inhibitory Ligand PD-L1 (CD 274) Provide a New Source of Xenogeneic Cells and Tissues With Low Immunogenic Properties. Xenotransplantation (2018) 25:e12387. doi:10.1111/xen.12387
88. Weiss, EH, Lilienfeld, BG, Müller, S, Müller, E, Herbach, N, Keler, B, et al. HLA-E/Human Beta2-Microglobulin Transgenic Pigs: Protection Against Xenogeneic Human Anti-Pig Natural Killer Cell Cytotoxicity. Transplantation (2009) 87:35–43. doi:10.1097/TP.0b013e318191c784
89. Rao, JS, Hosny, N, Kumbha, R, Levy, H, Matson, AW, Walters, E, et al. HLA-G1+ Expression in GGTA1KO Pigs Suppresses Human and Monkey Anti-Pig T, B and NK Cell Responses. Front Immunol (2021) 12:730545. doi:10.3389/fimmu.2021.730545
90. Bähr, A, Käser, T, Kemter, E, Gerner, W, Kurome, M, Baars, W, et al. Ubiquitous LEA29Y Expression Blocks T Cell Co-Stimulation But Permits Sexual Reproduction in Genetically Modified Pigs. PLoS One (2016) 11:e0155676. doi:10.1371/journal.pone.0155676
91. Ali, A, Kemter, E, and Wolf, E. Advances in Organ and Tissue Xenotransplantation. Annu Rev Anim Biosci (2024) 12:369–90. doi:10.1146/annurev-animal-021122-102606
92. Anand, RP, Layer, JV, Heja, D, Hirose, T, Lassiter, G, Firl, DJ, et al. Design and Testing of a Humanized Porcine Donor for Xenotransplantation. Nature (2023) 622:393–401. doi:10.1038/s41586-023-06594-4
93. Mou, L, Shi, G, Cooper, DKC, Lu, Y, Chen, J, Zhu, S, et al. Current Topics of Relevance to the Xenotransplantation of Free Pig Islets. Front Immunol (2022) 13:854883. doi:10.3389/fimmu.2022.854883
94. Buhler, L, Awwad, M, Basker, M, Gojo, S, Watts, A, Treter, S, et al. Experimental Transplantation-High-Dose Porcine Hematopoietic Cell Transplantation Combined With CD40 Ligand Blockade in Baboons Prevents an Induced Anti-Pig Humoral Response. Transplantation-Baltimore (2000) 69:2296–303. doi:10.1097/00007890-200006150-00013
95. Shin, J, Kim, J, Kim, J, Min, B, Kim, Y, Kim, H, et al. Long-Term Control of Diabetes in Immunosuppressed Nonhuman Primates (NHP) by the Transplantation of Adult Porcine Islets. Am J Transplant (2015) 15:2837–50. doi:10.1111/ajt.13345
96. Shin, JS, Kim, JM, Min, BH, Yoon, IH, Kim, HJ, Kim, JS, et al. Pre-Clinical Results in Pig-to-Non-Human Primate Islet Xenotransplantation Using Anti-CD 40 Antibody (2C10R4)-Based Immunosuppression. Xenotransplantation (2018) 25:e12356. doi:10.1111/xen.12356
97. Min, BH, Shin, JS, Kim, JM, Kang, SJ, Kim, HJ, Yoon, IH, et al. Delayed Revascularization of Islets After Transplantation by IL-6 Blockade in Pig to Non-Human Primate Islet Xenotransplantation Model. Xenotransplantation (2018) 25:e12374. doi:10.1111/xen.12374
98. Kim, JM, Hong, SH, Shin, JS, Min, BH, Kim, HJ, Chung, H, et al. Long-Term Control of Diabetes in a Nonhuman Primate by Two Separate Transplantations of Porcine Adult Islets Under Immunosuppression. Am J Transplant (2021) 21:3561–72. doi:10.1111/ajt.16704
99. Kim, JM, Hong, SH, Chung, H, Shin, JS, Min, BH, Kim, HJ, et al. Long-Term Porcine Islet Graft Survival in Diabetic Non-Human Primates Treated With Clinically Available Immunosuppressants. Xenotransplantation (2021) 28:e12659. doi:10.1111/xen.12659
100. Shin, JS, Min, BH, Kim, JM, Kim, JS, Yoon, IH, Kim, HJ, et al. Failure of Transplantation Tolerance Induction by Autologous Regulatory T Cells in the Pig-to-Non-Human Primate Islet Xenotransplantation Model. Xenotransplantation (2016) 23:300–9. doi:10.1111/xen.12246
101. Cardona, K, Korbutt, GS, Milas, Z, Lyon, J, Cano, J, Jiang, W, et al. Long-Term Survival of Neonatal Porcine Islets in Nonhuman Primates by Targeting Costimulation Pathways. Nat Med (2006) 12:304–6. doi:10.1038/nm1375
102. Thompson, P, Cardona, K, Russell, M, Badell, I, Shaffer, V, Korbutt, G, et al. CD40-Specific Costimulation Blockade Enhances Neonatal Porcine Islet Survival in Nonhuman Primates. Am J Transplant (2011) 11:947–57. doi:10.1111/j.1600-6143.2011.03509.x
103. Thompson, P, Badell, IR, Lowe, M, Turner, A, Cano, J, Avila, J, et al. Alternative Immunomodulatory Strategies for Xenotransplantation: CD40/154 Pathway-Sparing Regimens Promote Xenograft Survival. Am J Transplant (2012) 12:1765–75. doi:10.1111/j.1600-6143.2012.04031.x
104. Hering, BJ, Wijkstrom, M, Graham, ML, Hårdstedt, M, Aasheim, TC, Jie, T, et al. Prolonged Diabetes Reversal After Intraportal Xenotransplantation of Wild-Type Porcine Islets in Immunosuppressed Nonhuman Primates. Nat Med (2006) 12:301–3. doi:10.1038/nm1369
105. Lee, JI, Kim, J, Choi, YJ, Park, HJ, Park, HJ, Wi, HJ, et al. The Effect of Epitope-Based Ligation of ICAM-1 on Survival and Retransplantation of Pig Islets in Nonhuman Primates. Xenotransplantation (2018) 25:e12362. doi:10.1111/xen.12362
106. Hawthorne, W, Salvaris, E, Phillips, P, Hawkes, J, Liuwantara, D, Burns, H, et al. Control of IBMIR in Neonatal Porcine Islet Xenotransplantation in Baboons. Am J Transplant (2014) 14:1300–9. doi:10.1111/ajt.12722
107. Rood, PP, Bottino, R, Balamurugan, AN, Smetanka, C, Ayares, D, Groth, C-G, et al. Reduction of Early Graft Loss After Intraportal Porcine Islet Transplantation in Monkeys. Transplantation (2007) 83:202–10. doi:10.1097/01.tp.0000250680.36942.c6
108. Van Der Windt, D, Bottino, R, Casu, A, Campanile, N, Smetanka, C, He, J, et al. Long-Term Controlled Normoglycemia in Diabetic Non-human Primates After Transplantation With hCD46 Transgenic Porcine Islets. Am J Transplant (2009) 9:2716–26. doi:10.1111/j.1600-6143.2009.02850.x
109. Mandel, TE, Koulmanda, M, Cozzi, E, Waterworth, P, Tolan, M, Langford, G, et al. Transplantation of Normal and DAF-Transgenic Fetal Pig Pancreas Into Cynomolgus Monkeys. Transpl Proc (1997) 29:940. doi:10.1016/s0041-1345(96)00261-8
110. Zhang, Q, Gonelle-Gispert, C, Li, Y, Geng, Z, Gerber-Lemaire, S, Wang, Y, et al. Islet Encapsulation: New Developments for the Treatment of Type 1 Diabetes. Front Immunol (2022) 13:869984. doi:10.3389/fimmu.2022.869984
111. Duvivier-Kali, VF, Omer, A, Lopez-Avalos, MD, O'Neil, JJ, and Weir, GC. Survival of Microencapsulated Adult Pig Islets in Mice in Spite of an Antibody Response. Am J Transplant (2004) 4:1991–2000. doi:10.1111/j.1600-6143.2004.00628.x
112. Elliott, R, Escobar, L, Tan, P, Garkavenko, O, Calafiore, R, Basta, P, et al. Intraperitoneal Alginate-Encapsulated Neonatal Porcine Islets in a Placebo-Controlled Study With 16 Diabetic Cynomolgus Primates. In: Transplantation Proceedings. Elsevier (2005). p. 3505–8.
113. Elliott, R, Escobar, L, Calafiore, R, Basta, G, Garkavenko, O, Vasconcellos, A, et al. Transplantation of Micro-and Macroencapsulated Piglet Islets Into Mice and Monkeys. In: Transplantation Proceedings. Elsevier (2005). p. 466–9.
114. Sun, Y, Ma, X, Zhou, D, Vacek, I, and Sun, AM. Normalization of Diabetes in Spontaneously Diabetic Cynomologus Monkeys by Xenografts of Microencapsulated Porcine Islets Without Immunosuppression. J Clin Invest (1996) 98:1417–22. doi:10.1172/JCI118929
115. Groth, C, Tibell, A, Tollemar, J, Bolinder, J, Östman, J, Möller, E, et al. Transplantation of Porcine Fetal Pancreas to Diabetic Patients. The Lancet (1994) 344:1402–4. doi:10.1016/s0140-6736(94)90570-3
116. Wang, W, Mo, Z, Ye, B, Hu, P, Liu, S, and Yi, S. A Clinical Trial of Xenotransplantation of Neonatal Pig Islets for Diabetic Patients. Zhong nan da Xue Xue Bao Yi Xue ban= J Cent South Univ Med Sci (2011) 36:1134–40. doi:10.3969/j.issn.1672-7347.2011.12.002
117. Orlando, G, Piemonti, L, Ricordi, C, Stratta, RJ, and Gruessner, RW Transplantation, Bioengineering, and Regeneration of the Endocrine Pancreas, 2. Academic Press (2019).
118. Matsumoto, S, Tan, P, Baker, J, Durbin, K, Tomiya, M, Azuma, K, et al. Clinical Porcine Islet Xenotransplantation Under Comprehensive Regulation. In: Transplantation Proceedings. Elsevier (2014). p. 1992–5.
119. Matsumoto, S, Abalovich, A, Wechsler, C, Wynyard, S, and Elliott, RB. Clinical Benefit of Islet Xenotransplantation for the Treatment of Type 1 Diabetes. EBioMedicine (2016) 12:255–62. doi:10.1016/j.ebiom.2016.08.034
120. Reichman, TW, Ricordi, C, Naji, A, Markmann, JF, Perkins, BA, Wijkstrom, M, et al. 836-P: Glucose-Dependent Insulin Production and Insulin-Independence in Type 1 Diabetes From Stem Cell–Derived, Fully Differentiated Islet Cells—Updated Data From the VX-880 Clinical Trial. Diabetes (2023) 72. doi:10.2337/db23-836-p
121. Morozov, VA, Wynyard, S, Matsumoto, S, Abalovich, A, Denner, J, and Elliott, R. No PERV Transmission During a Clinical Trial of Pig Islet Cell Transplantation. Virus Res (2017) 227:34–40. doi:10.1016/j.virusres.2016.08.012
122. Valdes-Gonzalez, R, Dorantes, LM, Bracho-Blanchet, E, Rodríguez-Ventura, A, and Djg, W. No Evidence of Porcine Endogenous Retrovirus in Patients With Type 1 Diabetes After Long-Term Porcine Islet Xenotransplantation. J Med Virol (2010) 82:331–4. doi:10.1002/jmv.21655
123. Garkavenko, O, Croxson, M, Irgang, M, Karlas, A, Denner, J, and Elliott, R. Monitoring for Presence of Potentially Xenotic Viruses in Recipients of Pig Islet Xenotransplantation. J Clin Microbiol (2004) 42:5353–6. doi:10.1128/JCM.42.11.5353-5356.2004
124. Elliott, R, Escobar, L, Garkavenko, O, Croxson, M, Schroeder, B, McGregor, M, et al. No Evidence of Infection With Porcine Endogenous Retrovirus in Recipients of Encapsulated Porcine Islet Xenografts. Cel Transplant (2000) 9:895–901. doi:10.1177/096368970000900616
125. Garkavenko, O, Dieckhoff, B, Wynyard, S, Denner, J, Elliott, RB, Tan, PL, et al. Absence of Transmission of Potentially Xenotic Viruses in a Prospective Pig to Primate Islet Xenotransplantation Study. J Med Virol (2008) 80:2046–52. doi:10.1002/jmv.21272
126. Denner, J. Xenotransplantation of Pig Islet Cells: Potential Adverse Impact of Virus Infections on Their Functionality and Insulin Production. Xenotransplantation (2023) 30:e12789. doi:10.1111/xen.12789
127. Piemonti, L, Andres, A, Casey, J, de Koning, E, Engelse, M, Hilbrands, R, et al. US Food and Drug Administration (FDA) Panel Endorses Islet Cell Treatment for Type 1 Diabetes: A Pyrrhic Victory? Transpl Int (2021) 34:1182–6. doi:10.1111/tri.13930
128. Hawthorne, WJ. Ethical and Legislative Advances in Xenotransplantation for Clinical Translation: Focusing on Cardiac, Kidney and Islet Cell Xenotransplantation. Front Immunol (2024) 15:1355609. doi:10.3389/fimmu.2024.1355609
129. Reardon, S. First Pig Kidneys Transplanted Into People: What Scientists Think. Nature (2022) 605:597–8. doi:10.1038/d41586-022-01418-3
130. Montgomery, RA, Stern, JM, Lonze, BE, Tatapudi, VS, Mangiola, M, Wu, M, et al. Results of Two Cases of Pig-To-Human Kidney Xenotransplantation. N Engl J Med (2022) 386:1889–98. doi:10.1056/NEJMoa2120238
131. Porrett, PM, Orandi, BJ, Kumar, V, Houp, J, Anderson, D, Cozette Killian, A, et al. First Clinical-Grade Porcine Kidney Xenotransplant Using a Human Decedent Model. Am J Transplant (2022) 22:1037–53. doi:10.1111/ajt.16930
132. Reardon, S. First Pig-To-Human Heart Transplant: What Can Scientists Learn? Nature (2022) 601:305–6. doi:10.1038/d41586-022-00111-9
133. Moazami, N, Stern, JM, Khalil, K, Kim, JI, Narula, N, Mangiola, M, et al. Pig-to-Human Heart Xenotransplantation in Two Recently Deceased Human Recipients. Nat Med (2023) 29:1989–97. doi:10.1038/s41591-023-02471-9
134. Mallapaty, S. First Pig Liver Transplanted Into a Person Lasts for 10 Days. Nature (2024) 627:710–1. doi:10.1038/d41586-024-00853-8
135. Mallapaty, S, and Kozlov, M. First Pig Kidney Transplant in a Person: What It Means for the Future. Nature (2024) 628:13–4. doi:10.1038/d41586-024-00879-y
136. Cooper, DKC, and Pierson, RN. Milestones on the Path to Clinical Pig Organ Xenotransplantation. Am J Transpl (2023) 23:326–35. doi:10.1016/j.ajt.2022.12.023
137. Elliott, RB, Escobar, L, Tan, PL, Muzina, M, Zwain, S, and Buchanan, C. Live Encapsulated Porcine Islets From a Type 1 Diabetic Patient 9.5 Yr After Xenotransplantation. Xenotransplantation (2007) 14:157–61. doi:10.1111/j.1399-3089.2007.00384.x
138. Valdes-Gonzalez, R, Rodriguez-Ventura, A, White, D, Bracho-Blanchet, E, Castillo, A, Ramírez-González, B, et al. Long-Term Follow-Up of Patients With Type 1 Diabetes Transplanted With Neonatal Pig Islets. Clin Exp Immunol (2010) 162:537–42. doi:10.1111/j.1365-2249.2010.04273.x
Keywords: porcine islets, xenotransplantation, type 1 diabetes, immunogenicity, regulatory framework
Citation: Piemonti L, Citro A, Tomajer V, Partelli S and Caldara R (2024) Pig Xenotransplantation in Beta Cell Replacement: Addressing Challenges and Harnessing Potential for Type 1 Diabetes Therapy. Transpl Int 37:13122. doi: 10.3389/ti.2024.13122
Received: 11 April 2024; Accepted: 14 October 2024;
Published: 24 October 2024.
Copyright © 2024 Piemonti, Citro, Tomajer, Partelli and Caldara. This is an open-access article distributed under the terms of the Creative Commons Attribution License (CC BY). The use, distribution or reproduction in other forums is permitted, provided the original author(s) and the copyright owner(s) are credited and that the original publication in this journal is cited, in accordance with accepted academic practice. No use, distribution or reproduction is permitted which does not comply with these terms.
*Correspondence: Lorenzo Piemonti, cGllbW9udGkubG9yZW56b0Boc3IuaXQ=