Delayed Graft Function After Kidney Transplantation: The Role of Residual Diuresis and Waste Products, as Oxalic Acid and Its Precursors
- 1Department of Internal Medicine, Nephrology and Transplantation, Erasmus MC Transplant Institute, University Medical Center, Rotterdam, Netherlands
- 2Department of Internal Medicine, Division of Dietetics, Erasmus MC, Rotterdam, Netherlands
- 3Department of Epidemiology and Biostatistics, Erasmus MC, Rotterdam, Netherlands
- 4Department of Anesthesiology Erasmus MC, Rotterdam, Netherlands
- 5Department of Clinical Chemistry Metabolic Diseases, University Medical Center, Groningen, Netherlands
Delayed graft function (DGF) after kidney transplantation heralds a worse prognosis. In patients with hyperoxaluria, the incidence of DGF is high. Oxalic acid is a waste product that accumulates when kidney function decreases. We hypothesize that residual diuresis and accumulated waste products influence the DGF incidence. Patients transplanted between 2018–2022 participated in the prospective cohort study. Pre-transplant concentrations of oxalic acid and its precursors were determined. Data on residual diuresis and other recipient, donor or transplant related variables were collected. 496 patients were included, 154 were not on dialysis. Oxalic acid, and glyoxylic acid, were above upper normal concentrations in 98.8%, and 100% of patients. Residual diuresis was ≤150 mL/min in 24% of patients. DGF occurred in 157 patients. Multivariable binary logistic regression analysis demonstrated a significant influence of dialysis type, recipient BMI, donor type, age, and serum creatinine on the DGF risk. Residual diuresis and glycolic acid concentration were inversely proportionally related to this risk, glyoxylic acid directly proportionally. Results in the dialysis population showed the same results, but glyoxylic acid lacked significance. In conclusion, low residual diuresis is associated with increased DGF incidence. Possibly accumulated waste products also play a role. Pre-emptive transplantation may decrease the incidence of DGF.
Introduction
Delayed graft function (DGF) after kidney transplantation has been associated with donor, recipient and transplant related factors [1–3]. However, DGF most probably is multifactorially determined while the contribution of those factors varies in different studies. DGF occurs in about 30% of recipients of a deceased donor organ [2, 3] and 3.6% of recipients of a living donor kidney [1]. DGF is associated with decreased short and long term graft survival, partly due to an increased rejection risk [4, 5]. This means that DGF should be prevented when possible.
Acute tubular necrosis (ATN) in DGF differs from native kidney ATN in several ways, but the most striking is the prevalence of polarizable crystals consistent with calcium oxalate in DGF [6]. Calcium oxalate deposition in the transplanted kidney heralds a bad prognosis [7–9]. Experience with transplantation in patients with primary or secondary hyperoxaluria demonstrated that this population had higher rates of DGF, partially accompanied by biopsy-proven calcium oxalate deposition, compared to the non-hyperoxaluria population [10].
Primary hyperoxaluria is a group of autosomal recessive genetic disorders of the glyoxylate metabolism (Figure 1) [14, 15]. Oxalic acid is the end product of many metabolic processes and cannot be metabolized in the human body. Apart from oxalic acid, the nephrotoxic glyoxylic acid concentration is high in all types of primary hyperoxaluria [15]. In type 1 primary hyperoxaluria, glycolic acid is also high, in type 2 glyceric acid concentration is high.
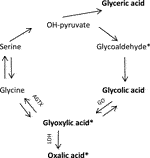
Figure 1. Endogenous oxalic acid synthesis pathway [11]. In bold are the substances whose concentrations were determined in this study. * known to be nephrotoxic, tubulotoxic [12, 13]. GO, glycolate oxidase; AGTX, Alanine glyoxylate aminotransferase; LDH, Lactic dehydrogenase.
High plasma oxalic acid concentrations may also be caused by several disorders associated with fat malabsorption (non-inherited, secondary or enteric hyperoxaluria) and that may lead to kidney injury and insufficiency as well [10, 16, 17].
Both primary and enteric hyperoxaluria may be associated with kidney stone formation and with oxalate crystal deposition, CKD and kidney failure [16, 18]. There is a high oxalate nephropathy (recurrence) rate after kidney transplantation in patients with primary and enteric hyperoxaluria [10, 15, 16, 19, 20]. Apart from crystal deposition, oxalic acid and its precursor glyoxylic acid have been shown to cause inflammation and tubulotoxicity [12, 13]. This means that kidney damage may be caused without or before tubular crystal depositions occur.
Finally, high plasma oxalic acid concentrations may be caused by kidney insufficiency and failure per se since the main excretion pathway is glomerular filtration and tubular secretion [21,22]. As urinary oxalic acid concentrations are unreliable in CKD stage 4 and 5, in analogy to primary hyperoxaluria, plasma oxalic acid concentrations are used instead [23]. Oxalic acid is easily removed by hemodialysis, but rebounds to pre dialysis concentrations within 48 h [24]. Clearance of oxalic acid is highest in the first hours of dialysis. Residual diuresis is superior to dialysis in removing various (non-urea) solutes and even clinically negligible residual kidney function has been shown to provide non-urea solute clearance [25–29]. With decreasing residual diuresis, accumulation of waste products increases even further. This means that almost all pre-transplant patients have high plasma oxalic acid concentrations.
We hypothesize that DGF is associated with high pre-transplantation concentrations of waste products, such as oxalic acid and its precursors. Under unfavorable conditions, these may lead to inflammation, tubular toxicity and in worse cases even depositions in the transplanted kidney.
Residual diuresis was included in our study in order to exclude the possibility that the effect of oxalic acid and precursors may represent the effect of a whole collection of waste products that have accumulated as a result of reduced residual diuresis. In that case residual diuresis may be a better representative for the whole collection of waste products.
Patients and Methods
All patients referred for kidney transplant work-up between September 2018 and January 2022 were asked to participate in this study. Follow-up was until January 1st, 2023. The study conforms with the principles outlined in the Declaration of Helsinki. It was approved by the medical ethics committee of Erasmus University Medical Center Rotterdam, and all patients gave their written informed consent before inclusion (MEC 2018-044). Participation comprised a 10 mL blood sample drawn on the operation ward immediately before transplantation. Oxalic acid and substrates in the metabolic pathway of oxalic acid (precursors, see Figure 1); glyoxylic acid, glycolic acid and glyceric acid concentrations were determined. Residual diuresis (remaining urine volume) was based on the patient’s last reported 24 h urine volume submitted. Besides, a questionnaire on dietary habits was filled in. Results of this food frequency questionnaire will be described separately.
Kidney function related variables were collected. Recipient variables studied were: age and gender, body mass index (BMI), pre-transplant CRP, pre-transplant vPRA (% panel reactive antibody), use of diuretics (yes versus no), pre-transplant oxalic acid, glyoxylic acid, glycolic acid, glyceric acid concentrations, cardiac disease, diabetes mellitus, vascular disease, cerebrovascular event, previous kidney transplantations (yes versus no), kidney function replacement therapy (none/hemodialysis/peritoneal dialysis), and time between start dialysis and current transplantation (months). Donor and transplantation related variables were: donor type (living versus donation after brain death (DBD) and donation after cardiac death (DCD)), donor age, gender, serum creatinine, BMI, hypertension, diabetes mellitus, HLA mismatches (A, B, DR; 1–6), and cold ischemia period. Delayed graft function (DGF) was defined as dialysis treatment in the first week after transplantation.
To quantify the plasma organic acids (oxalic acid, glyoxylic acid, glycolic acid and glyceric acid), blood was drawn and placed on ice followed by centrifugation at 4°C without delay. Heparinized plasma samples were de-proteinized by addition of 75 µL 37% hydrochloric acid to 0.5 mL plasma followed by centrifugation. The supernatant was stored at −70°C until analysis. For quantification, a gas chromatography mass spectrometry (GC-MS) method was used.
Data Analysis
Statistical analysis was performed using IBM SPSS Statistics 24. Baseline characteristics and outcomes were described as counts and percentages for categorical variables. For continuous variables, medians and interquartile ranges (IQR) were given for skewed continuous variables. Differences between continuous variables were studied using Mann-Whitney-U test. Differences between categorical variables were studied using Chi-square test.
Multivariable binary logistic regression analysis with backward elimination was used to study the influence of variables on the incidence of DGF, both in the total population (N = 496) and in the selection of patients on dialysis (n = 342). Kaplan Meier survival curves of the DGF and non-DGF populations were performed.
Spearman correlation analyses were performed to obtain correlation and 95%-confidence intervals between residual diuresis and plasma oxalic acid concentrations and residual diuresis and oxalic acid precursors. Correlation analyses were also performed for oxalic acid and its precursors and between precursors. Correlation values lower than 0.5 were considered weak. A p-value <0.05 was considered statistically significant.
Results
512 patients consented and underwent a kidney transplantation. In 16 patients concentrations of oxalic acid and/or its precursors were missing. Results of 496 patients were available for analysis. Table 1 shows patient characteristics, there were no missing values in these 496 patients. Residual diuresis was 150 mL/day or less in 121 patients (24%). Table 2 shows donor and transplantation characteristics. There were 230 (46%) living donor transplantations, 88 (18%) donation after brain death transplantations and 178 (36%) donatio after cardiac death transplantations.
Table 3 shows the concentrations of oxalic acid, glycolic acid, glyoxylic acid, and glyceric acid in the total patient population, the pre-dialysis population and in the population on dialysis. Only 1.2% of the patients had pre-transplant oxalic acid concentrations within the normal range. All glyoxylic acid concentrations were above the upper limit of normal. Glycolic acid and glyceric acid concentrations were within the reference range in 87% and 22% of cases respectively. Patients on dialysis had significantly higher oxalic acid, glycolic acid, glyoxylic acid and glyceric acid concentrations compared to pre-dialysis patients.
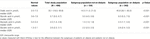
Table 3. Median values of oxalic acid, glycolic acid, glyoxylic acid and glyceric acid in the total study population and subgroup population on dialysis and not on dialysis.
Delayed graft function occurred in one-third (n = 157; 32%) of the population. There were 339 patients without DGF. In 84% of patients without DGF, serum creatinine at day 7 was at least halved compared to pre-transplant serum creatinine (Figure 2). Only 3 patients had an increase of serum creatinine on day 7 compared to day 0. One of them had a surgical complication with temporary increase in serum creatinine on day 7. Consequently, two patients without the diagnosis DGF that had an increase in serum creatinine on day 7, but adequate residual diuresis, ruling out the necessity for dialysis. Tables 1, 2 show that there are large differences between the populations with versus without DGF. The influence on the DGF risk of all variables shown in Tables 1, 2 was tested in binary logistic regression analysis. In univariable analysis, recipient variables with significant effect on DGF risk were: age, BMI, CRP, cardiac event, vascular disease, diabetes mellitus, residual diuresis, dialysis type, dialysis vintage, oxalic acid, glyoxylic acid and glyceric acid concentration, and number of previous kidney transplants. Donor variables with significant influence in univariable analysis were: donor type, age, gender, and cold ischemia time. In multivariable analysis, after backward elimination, categorical variables that remained in the model with a significant influence on the DGF risk were: donor type, and dialysis type. Besides, recipient BMI, donor age, donor serum creatinine, and glyoxylic acid concentration were significantly and directly proportionally related to the DGF risk, while residual diuresis and glycolic acid concentration were inversely proportionally related the DGF risk (Table 4). There was no interaction between any combination of residual diuresis, glyoxylic acid, and glycolic acid. There was no interaction between donor type and glyoxylic acid concentration, glycolic acid concentration, dialysis type, residual diuresis, and recipient BMI.
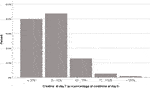
Figure 2. Ratio of serum creatinine on day 7 after transplantation and serum creatinine on day of transplantation in patients without delayed graft function (n = 339).
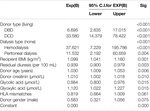
Table 4. Multivariable binary logistic regression analysis on delayed graft function, using backward elimination. Total population N = 496, events = 157.
The same analysis was performed in the population restricted to patients on dialysis (n = 342). Glyoxylic acid concentration failed significance, but all other variables with a significant influence in the total population also had a significant influence in the population on dialysis (Table 5).
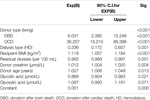
Table 5. Multivariable binary logistic regression analysis on delayed graft function, using backward elimination. Dialysis population n = 342, events = 155.
In a follow up period of almost 5 years, the survival curve shows significantly worse results in patients with DGF compared to those without DGF (p < 0.001; Figure 3).
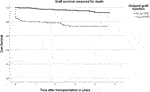
Figure 3. Kaplan Meier curves on graft survival censored for death in patients with and without delayed graft function (p < 0.001).
The relationship between residual diuresis and oxalic acid and its precursors was studied using Spearman’s correlation. It showed a significant, moderate correlation between oxalic acid and residual diuresis (N = 496; r = −0.529; p < 0.001). Correlation of residual diuresis with glycolic acid (r = −0.287; p < 0.001); with glyoxylic acid (r = −0.258; p < 0.001); and with glyceric acid (r = −0.260; p < 0.001) was weak but significant.
The relationship between oxalic acid and its precursors was studied using Spearman’s correlation. Correlation of oxalic acid with glyoxylic acid (r = 0.685; p < 0.001); and with glyceric acid (r = 0.570; p < 0.001) was significant. Correlation of oxalic acid with glycolic acid (r = 0.472; p < 0.001) was weak, but statistically significant.
Because glyoxylic acid significantly increased the DGF risk and glycolic acid decreased that risk in our multivariable regression analysis, their relationship was studied. Correlation of glyoxylic acid and glycolic acid was weak, but statistically significant (r = 0.370; p < 0.001). The scatterplot showed a dichotomy: In the extremes of the graph patients had either high glyoxylic acid or high glycolic acid concentrations, not both (Figure 4).
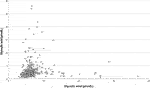
Figure 4. Scatterplot showing the relationship between pre-transplant glyoxylic acid concentration and glycolic acid concentration.
Discussion
Our study shows a significant effect of residual diuresis on the incidence of DGF after kidney transplantation: this holds true for the total population, but also, after exclusion of pre-dialysis patients, for the population on dialysis. Most probably, the association between low residual diuresis and DGF is the result of the accumulation of more or less toxic waste products that were not adequately removed via dialysis when residual diuresis decreased. Our study confirms that DGF is associated with decreased long-term graft survival [1, 5, 30].
There are two studies on the incidence of DGF after kidney transplantation, that also describe a significant influence of residual diuresis [30, 31]. Chaumont et al. studied the incidence of DGF in their center and concluded that perioperative saline loading and higher residual diuresis attributed to a lower risk [30]. Jahn aimed at risk factors for DGF and 1 year graft failure and concluded that residual diuresis influenced the DGF risk [31]. In patients on peritoneal dialysis [27, 32] and hemodialysis [33], patient survival has been shown to be negatively influenced by low residual diuresis. Besides, in dialysis patients, decreasing residual kidney function is associated with serious comorbidities [33–41]. This means that the pre-transplant patients with low or absent residual diuresis, are the less vital patients compared to those with significant residual diuresis volume. The cause of comorbidities is probably associated with accumulation, toxicity and/or deposition of toxic waste products, left behind as a result of failing diuresis [25, 28, 29, 42, 43]. Sudden excretion of these products by the newly transplanted kidney might cause inflammation, toxicity and possibly even depositions, that lead to kidney injury, and to impaired kidney function or even inhibition of the onset of donor kidney function.
Our study also shows that oxalic acid and its direct precursors glyoxylic acid, glycolic acid and glyceric acid are examples of waste products that accumulate when the kidney fails. In many pre-transplant patients, plasma oxalic acid concentrations are comparable to those of patients with primary hyperoxaluria. The glycolic acid concentrations are above the upper normal value in only 13% of cases (Table 3). Recently a small scale study showed normal glycolic acid concentrations in the dialysis population [44].
There was a significant and relevant correlation between oxalic acid concentration and glyoxylic and glyceric acid concentrations implicating that when high oxalic acid concentrations are found in pre-transplant patients, relatively high glyoxylic and glyceric acid concentrations may be expected. Highest concentrations of oxalic acid, glyoxylic acid, glycolic acid and glyceric acid were found in dialysis patients compared to pre-dialysis patients.
Both oxalic acid and its direct precursor glyoxylic acid are known for their tubulotoxicity, [12, 13, 45]. Although concentrations of both oxalic acid and glyoxylic acid significantly influenced DGF risk in univariable binary logistic regression analysis, in multivariable analysis only glyoxylic acid remained in the model and significantly influenced the DGF risk. This effect was independent of the effect of residual diuresis, emphasizing the individual toxic effect of glyoxylic acid. Glycolic acid on the other hand exerted a protective effect. Glycolic acid is not toxic. There is an inverse relationship between glyoxylic acid and glycolic acid as shown in Figure 4. The “protective” effect may be the result of shifting to non-toxic glycolic acid instead of toxic glyoxylic acid (Figure 1). On the other hand, in the restricted dialysis population glyoxylic acid failed significance, possibly as a result of lower numbers of patients included. When residual diuresis was removed from the model, the influence of glyoxylic acid became significant (p = 0.017), indeed suggesting that residual diuresis is a surrogate marker for at least glyoxylic acid, but probably also for many other toxic waste products.
A limitation of our study is that residual kidney function was not available in patients with residual diuresis, thus residual urine volume was used as a representative instead. Residual urine volume was based on the patient’s last 24-h urine collection submitted. Last collection may have been a few months before transplantation as 24-h urine collection must be submitted every 3 months for clinical care. Dialysis patients are aware of their 24 h urine production as it determines their fluid restriction.
Another limitation is the definition used for DGF, which is the most commonly used: dialysis in the first week after transplantation. However, fluid overload as the indication for dialysis may not be set in patients with preserved residual diuresis. When assessing transplant function on day 7 post-transplantation, there were only 2 patients without the diagnosis DGF that had an increase in serum creatinine, but adequate residual diuresis, ruling out the necessity for dialysis. All other patients without DGF had a decrease in serum creatinine. Without dialysis, even a small creatinine decrease of 10% is supposed to be the result of function of the transplanted kidney. This means that the definition for DGF turns out to be adequate in our population.
Preservation of residual diuresis, even after start dialysis, is useful and gains attention nowadays. Dietary and pharmacological interventions are defined to ensure optimal native kidney function preserving care [46]. Besides, on top of Kt/V there should be more attention for removal of other waste products, because high concentrations have a negative effect on graft function. This means that more intensive or optimal dialysis treatment, could have a beneficial effect on the prevention of DGF after transplantation. Besides, our study adds an argument to stimulate pre-emptive transplantation in patients who still have adequate diuresis and relatively low concentrations of waste products and thus are in relatively good condition.
Data Availability Statement
The raw data supporting the conclusions of this article will be made available by the authors, without undue reservation.
Ethics Statement
The studies involving humans were approved by the Medical ethics committee of Erasmus University Medical Center Rotterdam (MEC 2018-044). The studies were conducted in accordance with the local legislation and institutional requirements. The participants provided their written informed consent to participate in this study.
Author Contributions
GP: participated in research design, the performance of the research, data analysis, and the writing of the paper. WV: participated in research design, data collection and the writing of the paper. ML: participated in the performance of the research, data analysis, and the writing of the paper. SB: participated in statistical analysis and the writing of the paper. IM: participated in research design, data collection and the writing of the paper. DH: participated in research design, data collection and the writing of the paper. Participated in requesting patient consent. JV and AM-vE: participated in research design, data collection and the writing of the paper. MB, MvA, DS, JW, RZ, and MK: participated in requesting patient consent and the writing of the paper. MV: participated in data collection and determination of samples and the writing of the paper. IK and MR: participated in the writing of the paper. JR: participated in research design, the performance of the research, data analysis, the writing of the paper and requesting patient consent. All authors contributed to the article and approved the submitted version.
Funding
The author(s) declare that financial support was received for the research, authorship, and/or publication of this article. The authors declare that this study received funding from Foundation “Stichting de Merel”. The funder was not involved in the study design, collection, analysis, interpretation of data, the writing of this article or the decision to submit it for publication.
Conflict of Interest
The authors declare that the research was conducted in the absence of any commercial or financial relationships that could be construed as a potential conflict of interest.
Acknowledgments
We would like to thank Mrs Judith Kal-van Gestel, MSc and Mrs Tessa Royaards for their updates of regular recipient and living donor data, Mrs Ineke Tieken, MSc, for providing Eurotransplant donor data and Mr Chris Ramakers, MSc, for sample processing and storage.
Abbreviations
AGTX, Alanine glyoxylate aminotransferase; CAPD, Continuous ambulant peritoneal dialysis; DGF, Delayed graft function; DBD, Donation after brain death; DCD, Donation after cardiac death; eGFR, estimated Glomerular filtration rate; GO, Glycolate oxidase; HD, Hemodialysis; HLA, Human leucocyte antibody; IQR, interquartile range; LDH, Lactic dehydrogenase; vPRA, virtual Panel reactive antibodies.
References
1. Redfield, RR, Scalea, JR, Zens, TJ, Muth, B, Kaufman, DB, Djamali, A, et al. Predictors and Outcomes of Delayed Graft Function After Living-Donor Kidney Transplantation. Transpl Int (2016) 29(1):81–7. doi:10.1111/tri.12696
2. Irish, WD, McCollum, DA, Tesi, RJ, Owen, AB, Brennan, DC, Bailly, JE, et al. Nomogram for Predicting the Likelihood of Delayed Graft Function in Adult Cadaveric Renal Transplant Recipients. J Am Soc Nephrol (2003) 14(11):2967–74. doi:10.1097/01.asn.0000093254.31868.85
3. Mannon, RB. Delayed Graft Function: The AKI of Kidney Transplantation. Nephron (2018) 140(2):94–8. doi:10.1159/000491558
4. Jayaram, D, Kommareddi, M, Sung, RS, and Luan, FL. Delayed Graft Function Requiring More Than One-Time Dialysis Treatment Is Associated With Inferior Clinical Outcomes. Clin Transpl (2012) 26(5):E536–43. doi:10.1111/ctr.12029
5. Yarlagadda, SG, Coca, SG, Formica, RN, Poggio, ED, and Parikh, CR. Association Between Delayed Graft Function and Allograft and Patient Survival: A Systematic Review and Meta-Analysis. Nephrol Dial Transpl (2009) 24(3):1039–47. doi:10.1093/ndt/gfn667
6. Olsen, S, Burdick, JF, Keown, PA, Wallace, AC, Racusen, LC, and Solez, K. Primary Acute Renal Failure ("Acute Tubular Necrosis") in the Transplanted Kidney: Morphology and Pathogenesis. Medicine (Baltimore) (1989) 68(3):173–87. doi:10.1097/00005792-198905000-00005
7. Snijders, MLH, Hesselink, DA, Clahsen-van Groningen, MC, and Roodnat, JI. Oxalate Deposition in Renal Allograft Biopsies Within 3 Months After Transplantation Is Associated With Allograft Dysfunction. PLoS One (2019) 14(4):e0214940. doi:10.1371/journal.pone.0214940
8. Palsson, R, Chandraker, AK, Curhan, GC, Rennke, HG, McMahon, GM, and Waikar, SS. The Association of Calcium Oxalate Deposition in Kidney Allografts With Graft and Patient Survival. Nephrol Dial Transpl (2020) 35(5):888–94. doi:10.1093/ndt/gfy271
9. Truong, LD, Yakupoglu, U, Feig, D, Hicks, J, Cartwight, J, Sheikh-Hamad, D, et al. Calcium Oxalate Deposition in Renal Allografts: Morphologic Spectrum and Clinical Implications. Am J Transpl (2004) 4(8):1338–44. doi:10.1111/j.1600-6143.2004.00511.x
10. Roodnat, JI, de Mik-van Egmond, AME, Visser, WJ, Berger, SP, van der Meijden, WAG, Knauf, F, et al. A Successful Approach to Kidney Transplantation in Patients With Enteric (Secondary) Hyperoxaluria. Transpl Direct (2017) 3(12):e331. doi:10.1097/TXD.0000000000000748
11. Robijn, S, Hoppe, B, Vervaet, BA, D'Haese, PC, and Verhulst, A. Hyperoxaluria: A Gut-Kidney Axis? Kidney Int (2011) 80(11):1146–58. doi:10.1038/ki.2011.287
12. Poldelski, V, Johnson, A, Wright, S, Rosa, VD, and Zager, RA. Ethylene Glycol-Mediated Tubular Injury: Identification of Critical Metabolites and Injury Pathways. Am J Kidney Dis (2001) 38(2):339–48. doi:10.1053/ajkd.2001.26099
13. Knauf, F, Asplin, JR, Granja, I, Schmidt, IM, Moeckel, GW, David, RJ, et al. NALP3-Mediated Inflammation Is a Principal Cause of Progressive Renal Failure in Oxalate Nephropathy. Kidney Int (2013) 84(5):895–901. doi:10.1038/ki.2013.207
14. Cochat, P, and Rumsby, G. Primary Hyperoxaluria. N Engl J Med (2013) 369(7):649–58. doi:10.1056/NEJMra1301564
15. Demoulin, N, Aydin, S, Gillion, V, Morelle, J, and Jadoul, M. Pathophysiology and Management of Hyperoxaluria and Oxalate Nephropathy: A Review. Am J Kidney Dis (2022) 79(5):717–27. doi:10.1053/j.ajkd.2021.07.018
16. Witting, C, Langman, CB, Assimos, D, Baum, MA, Kausz, A, Milliner, D, et al. Pathophysiology and Treatment of Enteric Hyperoxaluria. Clin J Am Soc Nephrol (2021) 16(3):487–95. doi:10.2215/CJN.08000520
17. Sakhaee, K. Unraveling the Mechanisms of Obesity-Induced Hyperoxaluria. Kidney Int (2018) 93(5):1038–40. doi:10.1016/j.kint.2018.01.012
18. Waikar, SS, Srivastava, A, Palsson, R, Shafi, T, Hsu, CY, Sharma, K, et al. Association of Urinary Oxalate Excretion With the Risk of Chronic Kidney Disease Progression. JAMA Intern Med (2019) 179(4):542–51. doi:10.1001/jamainternmed.2018.7980
19. Karaolanis, G, Lionaki, S, Moris, D, Palla, VV, and Vernadakis, S. Secondary Hyperoxaluria: A Risk Factor for Kidney Stone Formation and Renal Failure in Native Kidneys and Renal Grafts. Transpl Rev (Orlando) (2014) 28(4):182–7. doi:10.1016/j.trre.2014.05.004
20. Nasr, SH, Valeri, AM, Said, SM, Sethi, S, Nath, KA, Lieske, JC, et al. Clinicopathologic Characteristics, Etiologies, and Outcome of Secondary Oxalate Nephropathy. Mayo Clin Proc (2024) 99:593–606. doi:10.1016/j.mayocp.2023.08.014
21. Tomson, CR, Channon, SM, Ward, MK, and Laker, MF. Plasma Oxalate Concentration, Oxalate Clearance and Cardiac Function in Patients Receiving Haemodialysis. Nephrol Dial Transpl (1989) 4(9):792–9. doi:10.1093/ndt/4.9.792
22. Morgan, SH, Purkiss, P, Watts, RW, and Mansell, MA. Oxalate Dynamics in Chronic Renal Failure. Comparison With Normal Subjects and Patients With Primary Hyperoxaluria. Nephron (1987) 46(3):253–7. doi:10.1159/000184364
23. Groothoff, JW, Metry, E, Deesker, L, Garrelfs, S, Acquaviva, C, Almardini, R, et al. Clinical Practice Recommendations for Primary Hyperoxaluria: An Expert Consensus Statement From ERKNet and OxalEurope. Nat Rev Nephrol (2023) 19(3):194–211. doi:10.1038/s41581-022-00661-1
24. Marangella, M, Petrarulo, M, Mandolfo, S, Vitale, C, Cosseddu, D, and Linari, F. Plasma Profiles and Dialysis Kinetics of Oxalate in Patients Receiving Hemodialysis. Nephron (1992) 60(1):74–80. doi:10.1159/000186708
25. Toth-Manikowski, SM, Sirich, TL, Meyer, TW, Hostetter, TH, Hwang, S, Plummer, NS, et al. Contribution of 'Clinically Negligible' Residual Kidney Function to Clearance of Uremic Solutes. Nephrol Dial Transpl (2020) 35(5):846–53. doi:10.1093/ndt/gfz042
26. Snauwaert, E, Holvoet, E, Van Biesen, W, Raes, A, Glorieux, G, Vande Walle, J, et al. Uremic Toxin Concentrations Are Related to Residual Kidney Function in the Pediatric Hemodialysis Population. Toxins (Basel) (2019) 11(4):235. doi:10.3390/toxins11040235
27. Termorshuizen, F, Korevaar, JC, Dekker, FW, van Manen, JG, Boeschoten, EW, Krediet, RT, et al. The Relative Importance of Residual Renal Function Compared With Peritoneal Clearance for Patient Survival and Quality of Life: An Analysis of the Netherlands Cooperative Study on the Adequacy of Dialysis (NECOSAD)-2. Am J Kidney Dis (2003) 41(6):1293–302. doi:10.1016/s0272-6386(03)00362-7
28. Marquez, IO, Tambra, S, Luo, FY, Li, Y, Plummer, NS, Hostetter, TH, et al. Contribution of Residual Function to Removal of Protein-Bound Solutes in Hemodialysis. Clin J Am Soc Nephrol (2011) 6(2):290–6. doi:10.2215/CJN.06100710
29. Fry, AC, Singh, DK, Chandna, SM, and Farrington, K. Relative Importance of Residual Renal Function and Convection in Determining Beta-2-Microglobulin Levels in High-Flux Haemodialysis and On-Line Haemodiafiltration. Blood Purif (2007) 25(3):295–302. doi:10.1159/000104870
30. Chaumont, M, Racape, J, Broeders, N, El Mountahi, F, Massart, A, Baudoux, T, et al. Delayed Graft Function in Kidney Transplants: Time Evolution, Role of Acute Rejection, Risk Factors, and Impact on Patient and Graft Outcome. J Transpl (2015) 2015:163757. doi:10.1155/2015/163757
31. Jahn, L, Ruster, C, Schlosser, M, Winkler, Y, Foller, S, Grimm, MO, et al. Rate, Factors, and Outcome of Delayed Graft Function After Kidney Transplantation of Deceased Donors. Transpl Proc (2021) 53(5):1454–61. doi:10.1016/j.transproceed.2021.01.006
32. Liao, CT, Chen, YM, Shiao, CC, Hu, FC, Huang, JW, Kao, TW, et al. Rate of Decline of Residual Renal Function Is Associated With All-Cause Mortality and Technique Failure in Patients on Long-Term Peritoneal Dialysis. Nephrol Dial Transpl (2009) 24(9):2909–14. doi:10.1093/ndt/gfp056
33. Kong, J, Davies, M, and Mount, P. The Importance of Residual Kidney Function in Haemodialysis Patients. Nephrology (Carlton) (2018) 23(12):1073–80. doi:10.1111/nep.13427
34. Virzi, GM, Milan Manani, S, Clementi, A, Castegnaro, S, Brocca, A, Riello, C, et al. Eryptosis Is Altered in Peritoneal Dialysis Patients. Blood Purif (2019) 48(4):351–7. doi:10.1159/000501541
35. Menon, MK, Naimark, DM, Bargman, JM, Vas, SI, and Oreopoulos, DG. Long-Term Blood Pressure Control in a Cohort of Peritoneal Dialysis Patients and its Association With Residual Renal Function. Nephrol Dial Transpl (2001) 16(11):2207–13. doi:10.1093/ndt/16.11.2207
36. de Sequera, P, Corchete, E, Bohorquez, L, Albalate, M, Perez-Garcia, R, Alique, M, et al. Residual Renal Function in Hemodialysis and Inflammation. Ther Apher Dial (2017) 21(6):592–8. doi:10.1111/1744-9987.12576
37. Wang, AY, Wang, M, Woo, J, Law, MC, Chow, KM, Li, PKT, et al. A Novel Association Between Residual Renal Function and Left Ventricular Hypertrophy in Peritoneal Dialysis Patients. Kidney Int (2002) 62(2):639–47. doi:10.1046/j.1523-1755.2002.00471.x
38. Yang, PY, Lin, JL, Lin-Tan, DT, Hsu, CW, Yen, TH, Chen, KH, et al. Residual Daily Urine Volume Association With Inflammation and Nutrition Status in Maintenance Hemodialysis Patients. Ren Fail (2009) 31(6):423–30. doi:10.1080/08860220902963566
39. Iwasawa, H, Nakao, T, Matsumoto, H, Okada, T, Nagaoka, Y, and Wada, T. Phosphate Handling by End-Stage Kidneys and Benefits of Residual Renal Function on Phosphate Removal in Patients on Haemodialysis. Nephrology (Carlton) (2013) 18(4):285–91. doi:10.1111/nep.12039
40. Chen, HC, Chou, CY, Jheng, JS, Chen, IR, Liang, CC, Wang, SM, et al. Loss of Residual Renal Function Is Associated With Vascular Calcification in Hemodialysis Patients. Ther Apher Dial (2016) 20(1):27–30. doi:10.1111/1744-9987.12376
41. Rroji, M, Spahia, N, Seferi, S, Barbullushi, M, and Spasovski, G. Influence of Residual Renal Function in Carotid Modeling as a Marker of Early Atherosclerosis in Dialysis Patients. Ther Apher Dial (2017) 21(5):451–8. doi:10.1111/1744-9987.12548
42. Wong, J, Sridharan, S, Berdeprado, J, Vilar, E, Viljoen, A, Wellsted, D, et al. Predicting Residual Kidney Function in Hemodialysis Patients Using Serum Beta-Trace Protein and Beta2-Microglobulin. Kidney Int (2016) 89(5):1090–8. doi:10.1016/j.kint.2015.12.042
43. Koppe, L, and Soulage, CO. Preservation of Residual Kidney Function to Reduce Non-Urea Solutes Toxicity in Haemodialysis. Nephrol Dial Transpl (2020) 35(5):733–6. doi:10.1093/ndt/gfz224
44. Metry, EL, Garrelfs, SF, Peters-Sengers, H, Vaz, FM, Bijlsma, JA, Neradova, A, et al. Plasma Oxalate and Glycolate Concentrations in Dialysis Patients With and Without Primary Hyperoxaluria Type 1. Nephrol Dial Transpl (2023) 38:1773–5. doi:10.1093/ndt/gfad049
45. Lu, H, Sun, X, Jia, M, Sun, F, Zhu, J, Chen, X, et al. Rosiglitazone Suppresses Renal Crystal Deposition by Ameliorating Tubular Injury Resulted From Oxidative Stress and Inflammatory Response via Promoting the Nrf2/HO-1 Pathway and Shifting Macrophage Polarization. Oxid Med Cel Longev (2021) 2021:5527137. doi:10.1155/2021/5527137
Keywords: delayed graft function, kidney transplantation, residual diuresis, accumulated waste products, oxalic acid, glyoxylic acid, deceased donor, living donor
Citation: Post Hospers G, Visser WJ, Verhoeven JGHP, Laging M, Baart SJ, Mertens zur Borg IRAM, Hesselink DA, de Mik-van Egmond AME, Betjes MGH, van Agteren M, Severs D, van de Wetering J, Zietse R, Vos MJ, Kema IP, Kho MML, Reinders MEJ and Roodnat JI (2024) Delayed Graft Function After Kidney Transplantation: The Role of Residual Diuresis and Waste Products, as Oxalic Acid and Its Precursors. Transpl Int 37:13218. doi: 10.3389/ti.2024.13218
Received: 01 May 2024; Accepted: 01 July 2024;
Published: 19 July 2024.
Copyright © 2024 Post Hospers, Visser, Verhoeven, Laging, Baart, Mertens zur Borg, Hesselink, de Mik-van Egmond, Betjes, van Agteren, Severs, van de Wetering, Zietse, Vos, Kema, Kho, Reinders and Roodnat. This is an open-access article distributed under the terms of the Creative Commons Attribution License (CC BY). The use, distribution or reproduction in other forums is permitted, provided the original author(s) and the copyright owner(s) are credited and that the original publication in this journal is cited, in accordance with accepted academic practice. No use, distribution or reproduction is permitted which does not comply with these terms.
*Correspondence: Gideon Post Hospers, g.posthospers@erasmusmc.nl