- 1Department of Medical and Clinical Microbiology, Faculty of Medicine, Pavol Jozef Šafárik University in Košice, Kosice, Slovakia
- 2Department of Medical Physiology, Faculty of Medicine, Pavol Jozef Šafárik University in Košice, Kosice, Slovakia
- 3Molecular Biology Systems B.V., Goes, Netherlands
The reverse transcription polymerase chain reaction (RT-PCR) is considered the gold standard method for the detection of viruses in a clinic. The aim of this study was to compare the ability of conventional RT-PCR test (FTDTM SARS-CoV-2 Test) and laboratory-developed ultra-fast PCR test (NextGenPCRTM SARS-CoV-2 RT-PCR Reagent Kit) to detect the coronavirus SARS-CoV-2 causing COVID-19. A total of 318 nasopharyngeal swab specimens were collected from people under investigation for COVID-19. Despite the collection of two swab specimens from each patient and their different processing, the analysis showed an overall agreement of 95.9% between the conventional and laboratory-developed tests. The positive percentage agreement was 90.5% (114/126) and the negative percentage agreement was 99.5% (191/192). The ultra-fast NextGenPCR method does not require the isolation of RNA, provides a result of 20–96 specimens within 57–82 min after sampling, and offers a simple procedure of sample processing, analysis, and evaluation. Our results indicate that this method can be considered a potential diagnostic method for the detection of SARS-CoV-2 virus in hospitals, healthcare facilities, and research laboratories.
Introduction
Coronaviruses represent a large group of RNA viruses capable of infecting humans and animals. Of the seven known types of human coronaviruses, four types (229E, NL63, OC43, and KHU1) are common and cause mild to moderate respiratory infections, like the common cold. Coronaviruses SARS-CoV (Severe Acute Respiratory Syndrome coronavirus) and MERS-CoV (Middle East Respiratory Syndrome coronavirus) are known as agents of severe respiratory infections SARS and MERS. The newest coronavirus, SARS-CoV-2, first emerged in the Chinese city of Wuhan in December 2019. The virus is highly contagious and causes mild to severe respiratory symptoms. Since its discovery, SARS-CoV-2 spread worldwide and caused a global pandemic. The infectious respiratory disease caused by this virus was named COVID-19 (Coronavirus disease 2019) (Chen et al., 2020; Araf et al., 2021; Kesheh et al., 2022).
Laboratory testing of the SARS-CoV-2 virus is essential for the appropriate clinical management and effective control measures to reduce transmission in the community. Multiple diagnostic platforms are available, with reverse transcription polymerase chain reaction being presented as the method with high sensitivity, specificity, and a short time interval of virus detection. Nasopharyngeal swabs are considered the gold standard sample type for SARS-CoV-2 diagnostic testing (World Health Organization, 2020; Centers for Disease Control and Prevention, 2021).
Various real-time RT-PCR assays have been developed worldwide, with different genes or regions of the viral genome, most commonly directed at the spike (S), envelope (E), and nucleocapsid (N) genes, or at one or more open reading frames including ORF1a and ORF1b. The aim of this study was to compare the ability of conventional RT-PCR test (FTDTM SARS-CoV-2 Test) and laboratory-developed ultra-fast NextGenPCR test (MBS, The Netherlands) to detect the coronavirus SARS-CoV-2 in clinical specimens. For this purpose, a total of 318 nasopharyngeal swab specimens from patients with suspected COVID-19 were analyzed. Nasopharyngeal swabs were dissolved in Liquid Amies Transport Medium, heat-inactivated, and 4 µL of medium without requiring RNA extraction was used in direct RT-PCR protocol using NextGenPCR ultra-fast thermocycler in order to detect specific sequences of SARS-CoV-2 virus. It follows from the mentioned results that a laboratory-developed ultra-fast NextGenPCR test has potential as a diagnostic method for the detection of SARS-CoV-2 virus in hospitals, healthcare facilities, and research laboratories.
Methods
Clinical samples
For the comparison of two RT-PCR tests, 318 people under investigation for COVID-19 disease were included in this study. Of them, 143 (45%) were men and 175 (55%) women. The age of the patients was in the range from 16 to 92 years. There were 40 (12.6%) patients in the age group of 16–30 years, 87 (27.4%) patients in the age group of 31–50 years, 135 (42.5%) patients in the age group of 51–70 years, and 56 (17.6%) patients in the age group over 71 years.
We collected two nasopharyngeal swab specimens from each patient using Σ-Transwab® nasopharyngeal swabs (Copan Diagnostics, Italy) and transferred them to a microtube containing 2 mL Liquid Amies Transport Medium (#52000, MBS, Netherlands) for the laboratory-developed ultra-fast PCR and with 2 mL InActiv Blue Transport Medium for the conventional FTD SARS-CoV-2 test. Specimens were collected at the mobile collection point of the Louis Pasteur University Hospital (Košice, Slovakia) between August and December 2022 and tested at the Department of Medical and Clinical Microbiology (DMCM) of the Pavol Jozef Šafárik University (Košice, Slovakia) within 48 h of collection. They were held refrigerated at 4°C if all testing could not be completed on the same day. Microtubes containing swabs in Liquid Amies medium were heat-inactivated at 100°C for 10 min, and 4 µL of the medium after cooling was used in a direct RT-PCR test. A total of 650 µL of InActive Blue medium containing the swab was taken for RNA extraction with the Versant SP 1.0 kit (Siemens Healthineers) on Versant kPCR Molecular System (Siemens Healthineers). The total eluate volume was 100 µL. For the conventional RT-PCR test, 10 µL of eluate was used.
Conventional RT-PCR test
By the FTDTM SARS-CoV-2 test, conserved sequences within ORF1ab and N genes of SARS-CoV-2 virus are amplified. Oligonucleotide probes labeled with FAM-89 (fluorescein amidites) are used in this test to detect SARS-CoV-2 gene sequences and a Cy5-labeled oligonucleotide probe to detect a sequence in the genome of equine arteritis virus (EAV). The EAV serves as an internal control (IC) in this test. The IC is extracted, processed, and amplified simultaneously with each sample to monitor the extraction process and to allow the identification of PCR inhibition. The negative control (nuclease free H2O) is processed as a sample (extraction and RT-PCR) to confirm the absence of contamination. The FTD TM SARS-CoV-2 kit contains a positive control (double-stranded synthetic DNA), which is added to each RT-PCR run to monitor the process of RT-PCR and the performance of the primers and probes. The analysis was done according to the manufacturer´s instructions. The reaction mixture with a total volume of 25 μL consisted of 10 µL of nucleic acid and 15 μL of RT PCR master mix (12.5 μL 2x RT PCR Buffer, 1.5 μL Primer/probe mix and 1 μL 25x RT-PCR Enzyme). RT-PCR was performed in a thermocycler Applied Biosystems 7500 Real-Time PCR System (Thermo Fisher Scientific). The thermal cycling program of conventional RT-PCR test is described in Table 1. The results were evaluated and reported as a cycle threshold (CT) value according to the manufacturer’s instructions (CT value ≤36.4 represented a positive and CT >36.4 a negative result).
NextGenPCR direct RT-PCR test
Three target sequences are amplified by multiplex RT-PCR in direct RT-PCR test. The presence of SARS-CoV-2 gRNA and human gDNA material in specimen is determined. For detection of SARS-CoV-2 N1 and ORF1ab gene sequences, FAM-89 labelled (fluorescein amidites) oligonucleotide probes and for detection of a sequence in the human RPP30 gene, Cy5-labeled oligonucleotide probes are used. As an internal control, primers/probes specific for RPP30 are used in the reaction in order to confirm absence of PCR inhibition and indicate the presence of human genomic material. A total volume of 20 μL of the reaction mixture included 4 μL of sample and 16 μL of RT-PCR master mix (10 μL RT-PCR Chemistry 2x, 1.6 μL SARS-CoV-2/hRNaseP Primers and Probes and 4.4 μL nuclease-free H2O). For confirmation of RT-PCR, positive control (Human Positive Control material) and negative control (nuclease free H2O) from the SARS-CoV-2 RT-PCR Reagent Kit (#50007, MBS, Netherlands) were included in each RT-PCR run. After pipetting, the microplate was covered with a transparent Clear Heat Seal (MBS, Netherlands), heat-sealed in a NextGenPCR Semiautomatic Heat Sealer (MBS, Netherlands) and transferred to a NextGenPCR Thermal Cycler (MBS, Netherlands) for PCR reaction. The RT-PCR program is described in the Table 2. After completion of PCR reaction, the sealed microplate was analysed using a FLUOstar Omega Microplate Reader (BMG Labtech, Germany). The fluorescence readout results were exported to Excel, visualized and analyzed.
Results
Description of the results for conventional RT-PCR and NextGenPCR direct RT-PCR test
Nasopharyngeal swab specimens from 318 unique patients were included in this study. 175 (55%) patients were women, 143 (45%) were men and the mean patient age was 53.9 years. The conventional RT-PCR test detecting ORF1ab, and N gene sequences was used to determine the clinical sensitivity and specificity of the laboratory-developed NextGenPCR direct RT-PCR test. The first nasopharyngeal swab was transferred to a microtube with InActiv Blue Transport Medium and always used in conventional FTD SARS-CoV-2 test while the second swab with Liquid Amies Transport Medium was always used in NextGenPCR.
There were 114 (35.8%) specimens in which SARS-CoV-2 gene sequences were detected by both tests (conventional RT-PCR and NextGenPCR). A total of 191 (60.1%) specimens were tested negative. The remaining 13 specimens gave discordant results, 12 (3.8%) specimens were positive in conventional RT-PCR and negative in NextGenPCR, one (0.3%) specimen was negative in conventional RT-PCR and positive in NextGenPCR. Agreement scores of both tests are described in Table 3.
For 126 positive specimens with conventional RT-PCR, the obtained CT values ranged from 11 to 34 (median = 21.1). Of them, 12 specimens gave a negative result with NextGenPCR. The CT values of these specimens ranged from 28 to 33 (median = 30.7). The remaining 112 concordant specimens exhibited CT values from 11 to 34 (median = 20.3). Comparison CT values of positive specimens of conventional RT-PCR and fluorescence levels of NextGenPCR direct RT-PCR test are shown in Figure 1. According to the Pearson Correlation Coefficient test the r-value is −0.7311. This is a moderate negative correlation, which means there is a tendency for high X variable scores (CT value) to go with low Y variable scores (Fluorescence) and vice versa. A negative result with conventional RT-PCR and a positive result with NextGenPCR test was determined in one specimen. From the mentioned results it follows that the clinical sensitivity (positive percentage agreement) was 90.5% (114/126) and the clinical specificity (negative percentage agreement) 99.5% (191/192).
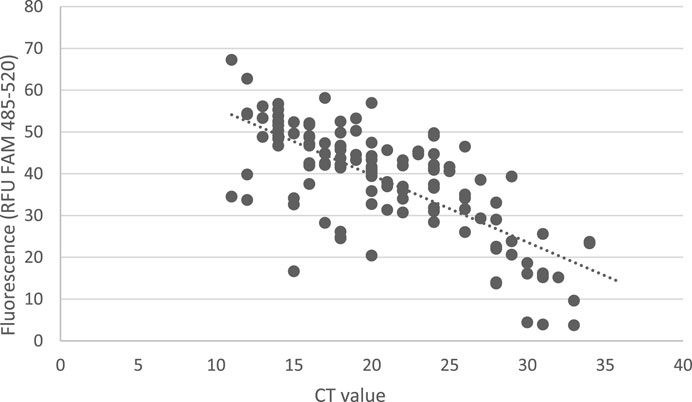
FIGURE 1. Comparison of NextGenPCR direct RT-PCR test and conventional RT-PCR test. Fluorescence levels detected by NextGenPCR direct RT-PCR test show a linear correlation with CT values of specimens determined by conventional RT-PCR as positive. The Y-axis represents measured fluorescence levels x 103.
Description of the time course for conventional RT-PCR and NextGenPCR direct RT-PCR test
The total time of conventional RT-PCR ranged from 209 to 399 min, depending on the number of tested specimens. In the monitored period, 20–96 specimens were processed per run. The DMCM laboratory processed specimens of patients hospitalized at Louis Pasteur University Hospital and outpatients whose nasopharyngeal swabs were performed at the mobile collection point. The first step of this procedure was pipetting collected specimens into special extraction tubes and centrifugation. The time interval of this step varied from 20 to 45 min. The minimum time for RNA extraction with Versant kPCR Molecular System was 80 min (20 samples) and the maximum time was 220 min (96 samples including PC and NC). Preparation of the plate for RT-PCR with 20 specimens took 10 min while preparation of a full plate took 45 min. The time course of RT-PCR was 89 min and evaluation 10–20 min.
The total time of NextGenPCR direct RT-PCR ranged from 57 to 82 min, depending on the number of tested specimens. The DMCM laboratory processed specimens from the mobile collection point only. In the monitored period, 4–21 specimens were processed per run. One full plate was also prepared to determine the total time for this method. Specimens were first inactivated for 10 min. Steps such as pipetting, centrifugation, and extraction were not required. The time of the plate preparation and evaluation of results was identical to the conventional qPCR and the time of RT PCR was 27 min. The time course of all steps for both methods is described in detail in Figure 2.
Discussion
Rapid, reliable, and accurate detection of SARS-CoV-2 virus plays an essential role in the appropriate management of patients, limiting the outbreak of infection and understanding the epidemiology of the virus. Current laboratory tests primarily focus on amplifying and detecting several specific sequences of the virus genome in upper respiratory tract specimens. The results of several studies (Astuti and srafil, 2020; Shen et al., 2021) indicate that ORF1ab and N genes are key targets in the detection of SARS-CoV-2. ORF1ab (RNA-dependent RNA polymerase) is responsible for viral transcription and translation (Astuti and srafil, 2020). The N protein is abundantly produced by infected cells and plays a role in their apoptosis and in viral replication, translation, and transcription (Tai et al., 2020). This study compares two amplification methods using multiple primer/probe pairs directed at the SARS-CoV-2 N and ORF1ab gene sequences in a single reaction mixture. The advantage of such methods is the simultaneous detection of different targets in one reaction. In the presence of the target, primers and probes hybridize to the specific sequence and allow amplification by the polymerase. Both amplicons contribute to the fluorescent signal generated in the specimen and lead to an increased signal amplitude generated for N and ORF1ab targets.
In our cohort, a total of 318 nasopharyngeal swab specimens were tested by two RT-PCR tests. Of them, 13 specimens exhibited discordant results, 12 were positive with conventional RT-PCR and negative with NextGenPCR, one was negative with conventional RT-PCR and positive with NextGenPCR test. The reason for these different results may be the double collection of nasopharyngeal swabs from the patient as the second swab may not always be carried out as thoroughly. We consider this to be the limitation of our study. The concentration factor is different in both tests and this may cause discrepancies between the test outcomes as well. In FTD SARS-CoV-2 test, the volume 650 µL of InActiv Blue Transport Medium for RNA extraction is required. In NextGenPCR test, the volume 4 µL of the heat-inactivated medium after cooling is required (Struijk et al., 2023).
The heat inactivation procedure was introduced in RT-PCR techniques in order to lyse epithelial cells. This process may have a negative effect on virus detection and reduce the sensitivity of the technique as described by several authors (Burton et al., 2021; Cameron et al., 2021; Domnich et al., 2021). In this study, discordance between compared tests was observed in 12 specimens with CT values ≥ 28 while specimens with CT ˂28 were identified correctly. Domnich et al. (2021) compared the sensitivity of conventional extraction-based, heated extraction-free, and unheated extraction-free RT-PCR methods for SARS-CoV-2 detection in 98 nasopharyngeal swabs from symptomatic individuals. All these specimens were positive in the conventional RT-PCR test. Of them, 8.2% of swabs gave negative results with the heated extraction-free method and clinical sensitivity (91.8%) was comparable to our NextGenPCR method (90.5%). They found discordant results in specimens with CT >30. Cameron et al. (2021) observed the sensitivity of the heated extraction-free method in specimens with CT ≤25.
The routine laboratory testing process of the RT-PCR technique in order to determine SARS-CoV-2 virus takes a few hours. In the case of the FTDTM SARS-CoV-2 test used in our study it is 3.5–6.7 h. Many clinical laboratories isolate RNA using various high-throughput robotics and in this way can reduce the total time of testing process to 2.5–3.5 h compared to our conventional test. The average processing time of a heated extraction-free NextGenPCR test is 57–82 min. In the study of Domnich et al. (2021) the average processing times were 270, 163, and 156 min for the conventional extraction-based, heated extraction-free, and unheated extraction-free RT-PCR methods, respectively. Moore et al. (2020) compared the ability of two conventional RT-PCR tests and a laboratory-developed RT-PCR test with RNA extraction to identify the SARS-CoV-2 virus in 200 nasopharyngeal swab specimens. In their research, one swab was collected from each patient. The laboratory-developed isothermal test targeted the RdRP gene, was the easiest to perform, and provided a result in the shortest time (not determined exactly), but overall agreement with a value of 83.5% for positive as well as negative, agreement scores were lower than in our research.
In addition to detecting SARS-CoV-2, the ultra-fast NextGenPCR method has already been used to identify E. coli in urine by molecular analysis of phylogenetic groups (A, B1, B2, D, F, clade V) and three genes specific for uropathogenic E. coli. In this application, PCR amplification takes 16 min and significantly reduces the time required for pathogen identification (Brons et al., 2020). From this point of view the ultra-fast NextGenPCR method with a novel thermal cycler developed for this purpose can be used to analyze the gene sequences of multiple organisms, not only viruses and bacteria.
Conclusion
In our study, ORF1ab and N gene sequences of SARS-CoV-2 virus were analyzed by two RT-PCR tests, laboratory-developed NextGenPCR and conventional RT-PCR test. The obtained data showed that NextGenPCR detected correctly 95.9% of clinical specimens. The positive percentage agreement reached a value 90.5% and the negative percentage agreement was 99.5%. In addition to the promising results, important benefits of NextGenPCR method with a novel thermal cycler include a simple procedure of sample processing, analysis, and evaluation, the use of a heat-inactivated medium containing a nasopharyngeal swab specimen, and a significant time saving for pathogen identification compared to a conventional RT-PCR test. This method has the potential to be used in the routine clinical diagnostics of gene sequences of various organisms.
Data availability statement
The raw data supporting the conclusion of this article will be made available by the authors, without undue reservation.
Ethics statement
The studies involving humans were approved by Ethics Committee of Louis Pasteur University Hospital in Kosice. The studies were conducted in accordance with the local legislation and institutional requirements. Written informed consent for participation in this study was provided by the participants’ legal guardians/next of kin.
Author contributions
KČ: performing experiments, data analysis, co-writing the manuscript. VL: performing experiments, data analysis, review the manurscript. MN: performing experiments and review the manuscript. LS: review the manuscript. VD: review the manuscript. GV: conceptualization and review the manuscript. All authors contributed to the article and approved the submitted version.
Funding
This publication is the result of the project: “Design and implementation of advanced methods of lung ventilation treatment and diagnosis of viral pneumonia including COVID-19 with the possibility of their rapid adoption,” ITMS 2014+: 313011ASX1 supported by the Operational Program Integrated Infrastructure funded by European Regional Development Fund (ERDF).
Conflict of interest
GV is the director of Molecular Biology Systems B.V.
The remaining authors declare that the research was conducted in the absence of any commercial or financial relationships that could be construed as a potential conflict of interest.
References
Araf, Y., Faruqui, N. A., Anwar, S., and Hosen, M. J. (2021). SARS-CoV-2: A new dimension to our understanding of coronaviruses. Int. Microbiol. Jan. 24 (1), 19–24. doi:10.1007/s10123-020-00152-y
Astuti, I., and srafil, Y. (2020). Severe acute respiratory syndrome coronavirus 2 (SARS-CoV-2): an overview of viral structure and host response. Diabetes Metab. Syndr. 14 (4), 407–412. doi:10.1016/j.dsx.2020.04.020
Brons, J. K., Vink, S. N., de Vos, M. G. J., Reuter, S., Dobrindt, U., and van Elsas, J. D. (2020). Fast identification of Escherichia coli in urinary tract infections using a virulence gene based PCR approach in a novel thermal cycler. J. Microbiol. Methods 169, 105799. doi:10.1016/j.mimet.2019.105799
Burton, J., Love, H., Richards, K., Burton, C., Summers, S., Pitman, J., et al. (2021). The effect of heat-treatment on SARS-CoV-2 viability and detection. J. Virol. Methods 290, 114087. doi:10.1016/j.jviromet.2021.114087
Cameron, A., Pecora, N. D., and Pettengill, M. A. (2021). Extraction-free methods for the detection of SARS-CoV-2 by reverse transcription-PCR: A comparison with the cepheid xpert xpress SARS-CoV-2 assay across two medical centers. J. Clin. Microbiol. 59, e02643–e2720. doi:10.1128/JCM.02643-20
Centers for Disease Control and Prevention (2021). Interim guidelines for collecting and handling of clinical specimens for COVID-19 testing. Available at: https://www.cdc.gov/coronavirus/2019-ncov/lab/guidelines-clinical-specimens.html (Accessed July 15, 2022).
Chen, B., Tian, E. K., He, B., Tian, L., Han, R., Wang, S., et al. (2020). Overview of lethal human coronaviruses. Sig Transduct. Target Ther. 5 (1), 89. doi:10.1038/s41392-020-0190-2
Domnich, A., De Pace, V., Pennati, B. M., Caligiuri, P., Varesano, S., Bruzzone, B., et al. (2021). Evaluation of extraction-free RT-qPCR methods for SARS-CoV-2 diagnostics. Arch. Virol. 166, 2825–2828. doi:10.1007/s00705-021-05165-0
Kesheh, M. M., Hosseini, P., Soltani, S., and Zandi, M. (2022). An overview on the seven pathogenic human coronaviruses. Rev. Med. Virol. Mar. 32 (2), e2282. doi:10.1002/rmv.2282
Moore, N. M., Li, H., Schejbal, D., Lindsley, J., and Hayden, M. K. (2020). Comparison of two commercial molecular tests and a laboratory-developed modification of the CDC 2019-nCoV reverse transcriptase PCR assay for the detection of SARS-CoV-2. J. Clin. Microbiol. 58, 009388–e1020. doi:10.1128/JCM.00938-20
Shen, L., Cui, S., Zhang, D., Lin, C., Chen, L., and Wang, Q. (2021). Comparison of four commercial RT-PCR diagnostic kits for COVID-19 in China. J. Clin. Lab. Anal. 35 (1), e23605. doi:10.1002/jcla.23605
Struijk, R., van den Ouden, A., Louwerse, J., Čurová, K., Burggrave, R., McNally, B., et al. (2023). Ultrafast RNA extraction-free SARS-CoV-2 detection by direct RT-PCR using a rapid thermal cycling approach. Diagn Microbiol Inf Dis 107, 115975. doi:10.1016/j.diagmicrobio.2023.115975
Tai, W., He, L., Zhang, X., Pu, J., Voronin, D., Jiang, S., et al. (2020). Characterization of the receptor-binding domain (RBD) of 2019 novel coronavirus: implication for development of RBD protein as a viral attachment inhibitor and vaccine. Cell. Mol. Immunol. 17 (6), 613–620. doi:10.1038/s41423-020-0400-4
World Health Organization (2020) Laboratory testing for coronavirus disease (COVID-19) in suspected human cases: Interim guidance, 19 march 2020. Geneva: World Health Organization. License: CC BY-NC-SA 3.0 IGO. Available at: https://apps.who.int/iris/handle/10665/331501 (Accessed January 17, 2020).
Keywords: SARS-CoV-2, RT PCR, NextGenPCR, detection, time course
Citation: Čurová K, Lovayová V, Nagyová M, Siegfried L, Donič V and de Vos G (2023) Detection of SARS-CoV-2 using a laboratory-developed ultra-fast NextGenPCR test versus a conventional RT-PCR test. Acta Virol. 67:11588. doi: 10.3389/av.2023.11588
Received: 17 May 2023; Accepted: 29 September 2023;
Published: 10 October 2023.
Edited by:
Boris Klempa, Slovak Academy of Sciences, SlovakiaReviewed by:
Peter Sabaka, Comenius University, SlovakiaLukas Radosa, University Hospital Jena, Germany
Copyright © 2023 Čurová, Lovayová, Nagyová, Siegfried, Donič and de Vos. This is an open-access article distributed under the terms of the Creative Commons Attribution License (CC BY). The use, distribution or reproduction in other forums is permitted, provided the original author(s) and the copyright owner(s) are credited and that the original publication in this journal is cited, in accordance with accepted academic practice. No use, distribution or reproduction is permitted which does not comply with these terms.
*Correspondence: Gert de Vos, Z2VydGRldm9zQG1ic3Bjci5jb20=