- 1Functional Biomaterial Research Center, Korea Research Institute of Bioscience and Biotechnology, Jeongeup-si, Jeonbuk, Republic of Korea
- 2College of Veterinary Medicine and BK21 Plus Project Team, Chonnam National University, Gwangju, Republic of Korea
Although vaccines have been developed for rotavirus infections, there is currently no effective therapeutic treatment. Therefore, this study aimed to evaluate the efficacy of 18-hydroxyferruginol (1) and 18-oxoferruginol (2) isolated from Torreya nucifera (T. nucifera) against bovine G8P[7] and porcine G5P[7] rotaviruses using two different assay strategies: 1) reduction of viral infectivity by neutralizing the virus (virucidal assay) and 2) inhibition of viral replication after infection (post-treatment assay). In the post-treatment assay, compounds 1 and 2 exhibited strong anti-rotavirus activity, with 50% effective concentration values of 24.7 μM (selectivity index; SI= 2.52) and 23.2 μM (SI= 1.75) against bovine G8P[7], 21.1 μM (SI= 2.95) and 22.6 μM (SI= 1.80) against porcine G5P[7], respectively. During viral replication, the two compounds demonstrated stronger inhibition of viral RNA synthesis in the late stages (18 h) than in the early stages (6 h). Compounds 1 and 2 also inhibited the synthesis of viral proteins such as VP6, as determined by immunofluorescence assay. Thus, it appears that compounds 1 and 2 isolated from T. nucifera possess strong antiviral activity against rotaviruses, inhibit viral replication, and may be developed into potential plant-derived therapeutic candidates against rotavirus infection.
Introduction
Rotavirus, a member of the Reoviridae family, is a significant pathogen that causes severe acute diarrhea that causes dehydration in young children and a wide variety of young animals (Estes and Greenberg, 2013; Desselberger, 2014). Rotaviruses are large, non-enveloped viruses consisting of triple-layered particles that surround the viral genome and are composed of 11 segments of double-stranded RNA (Estes and Greenberg, 2013). Specifically, rotaviruses cause severe diarrhea in neonatal and post-weaning piglets and calves (Estes and Greenberg, 2013; Desselberger, 2014).
Antiviral drugs, including chemicals, probiotics, immunoglobulins, and natural products, are used to treat rotavirus infections (Grandy et al., 2010; Téllez et al., 2015). The chemical compounds Ribavirin, 3-deazaguanine (3-DG), and inosine pranobex have shown antiviral effects against the simian rotavirus SA11 strain (Smee et al., 1982; Linhares et al., 1989), and immunoglobulins have been used to treat rotavirus-induced diarrhea. However, the use of these drugs is limited due to their high cost, and their potential side effects remain unknown (Yolken et al., 1985; Guarino et al., 1994). Natural products from plants, such as black tea (theaflavin compound) (Clark et al., 1988), Stevia rebaudiana (anionic polysaccharide compound) (Takahashi et al., 2001), Brazilian medicinal plants (Cecilio et al., 2012), Quillaja Saponaria (Tam and Roner, 2011), and dietary plants (Huang et al., 2015), inhibit the absorption or replication of human and animal rotaviruses. Currently, medicinal plants and natural molecules are not widely available for use in human or animal treatments of rotavirus infections. To date, no clinically approved drugs are available specifically for the treatment of rotavirus infections. The gold standard treatment for patients with acute gastroenteritis involves replenishing lost fluids and electrolytes through rehydration therapy (Crawford et al., 2017). Nevertheless, diarrhea caused by rotaviruses remains a significant issue; thus, the development of new drugs is urgently needed to effectively control rotavirus infections.
Torreya nucifera (T. nucifera), a member of the Taxaceae family, is a tree species that grows in snowy regions, such as Jeju Island in Korea. This tree has been used in traditional Asian medicine to treat various diseases, including stomachaches, hemorrhoids, and rheumatoid arthritis (Bae, 2000). Various abietane diterpenoids isolated from the leaves of T. nucifera have been reported to possess antiviral activity against the influenza virus (Bae et al., 2023) and SARS-CoV 3CLpro (Ryu et al., 2010). However, the anti-rotavirus activities of extracts and compounds isolated from T. nucifera remain unknown. Thus, this study identified the in vitro anti-rotavirus activity of abietane diterpenoids and its mechanism of anti-rotavirus activity.
Materials and methods
Materials
The leaves of T. nucifera were collected from Jeju Island, Republic of Korea, in October 2003. A voucher specimen of the plant material was deposited in the author’s laboratory for future reference and authentication purposes (Ryu et al., 2010).
Cells and viruses
African rhesus monkey kidney (MA-104) cells were procured from the American Type Culture Collection (ATCC CRL-2373.1; Manassas, VA, United States). The MA-104 cells were cultivated in Eagle’s minimum essential medium (EMEM) accompanied by a supplementation of 5% fetal bovine serum (FBS), 100 U/mL penicillin, 100 μg/mL streptomycin, and 100 U/mL amphotericin B (Park et al., 2006). The KJ-56 (bovine rotavirus, G8P[7]) and 85A (porcine rotavirus, G5P[7]) viruses isolated from fecal samples of diarrheic Korean calves and piglets were pre-activated with 10 μg/mL trypsin (1:250; GIBCO Invitrogen Corporation, California) for 30 min at 37°C before being inoculated into confluent MA-104 cells. Subsequently, the infected cells were sustained in EMEM with 1 μg/mL trypsin.
Cytotoxicity assay
MA-104 cells (1 × 105 cells/well) were seeded into 96-well plates and incubated for 24 h. The media were removed and substituted with EMEM containing serial dilutions of the extracts (1–200 μg/mL) or compounds (1–100 μM) dissolved in DMSO (with a final concentration at 0.5% DMSO). Control groups were cultured in the presence of 0.5% DMSO. After incubation for 72 h, the media were discarded, and 5 µL of MTT (3-[4,5-dimethylthiozol-2-yl]-2,5-diphenyltetrazolium bromide; Sigma, St. Louis, MO) solution was added to each well. After 4 h at 37°C, the supernatant was removed, and 100 µL of 0.04 M HCl-isopropanol was added to each well to dissolve formazan crystals. The absorbance of each well was assessed at 540 nm by using a microplate reader. After subtracting the background absorbance at 655 nm, the 50% cytotoxic concentration (CC50) of each extract was estimated using regression analysis.
Antiviral assay
The antiviral assays employed in this study have been previously described (Bae et al., 2023), and observation of these assays was carried out using the neutral red method, as described briefly. In the virucidal assay, each extract and compound were mixed with a 0.01 multiplicity of infection (MOI) of rotaviruses at various concentrations (1–100 μg/mL or 1–30 μM) and incubated at 4°C for 1 h. The mixtures were incubated in triplicate onto near-confluent MA-104 cell monolayers for 1 h, accompanied by intermittent rocking. The solution was removed and the replaced by EMEM containing 1 μg/mL trypsin. The cells were incubated for 72 h at 37°C under 5% CO2 atmosphere until the cells in the infected, untreated control showed complete viral CPE by light microscopy. In the post-treatment assay, rotaviruses at a 0.01 MOI were inoculated onto near-confluent MA-104 cell monolayers (1 × 105 cells/well) for 1 h with occasional rocking. The solution was removed and replaced by EMEM containing 1 μg/mL trypsin and various concentrations (1–100 μg/mL or 1–30 μM) of each extract or compound dissolved in DMSO (with a final concentration at 0.5% DMSO) in triplicate. Infected/untreated and uninfected/untreated cells were cultured in the presence of 0.5% DMSO. The cells were incubated for 72 h at 37°C until cells in the infected/untreated group exhibited complete viral CPE as observed under light microscopy. Neutral red solution was added to each well at 0.034% (w/v), and the plates were incubated for 2 h at 37°C in the dark. After discarding the neutral red solution, the cells were washed with PBS (pH 7.4) and followed by the addition of a destaining solution (1% glacial acetic acid, 49% H2O, and 50% ethanol). After being incubated in darkness for 15 min at room temperature, the absorbance of each well was at 540 nm using a microplate reader. The 50% effective concentration (EC50) value was calculated by regression analysis. The selectivity index (SI) was calculated using the following formula: SI = 50% cytotoxic concentration (CC50)/EC50.
Quantitative reverse transcription PCR (qRT-PCR)
MA-104 cells were cultured until 90% confluence, infected with rotavirus at 0.01 MOI, and cultured with 18-hydroxyferruginol (1) and 18-oxoferruginol (2). Infected/untreated cells were cultured with 0.5% DMSO. After 6 and 18 h, the culture medium was removed, and the cells were detached by scraping. Subsequently, the cell washed twice with phosphate-buffered saline (PBS) and collected by centrifugation at 500× g for 3 min. Total RNA was extracted utilizing the Qiagen RNeasy Mini Kit (QIAGEN, Hilden, Germany) following the guidelines provided by the manufacturer. Specific primers were used for the reverse transcription of viral RNA (vRNA; NSP 3 gene, 5′-ACCATCTWCACRTRACCCTCTATGAG-3’ (upstream) and 5′-GGTCAC ATAACGCCCCTATAGC -3′ (downstream). GAPDH was used as an internal control for cellular RNAs, with the primer sequences 5′-TCAACAGCGACACCCACTC-3′ (upstream) and 5′-CTTCCTCTTGTGCTCTTGCTG-3′ (downstream). cDNA was synthesized from total RNA using the cDNA Master Mix (Applied Biosystems, CA, United States). qRT-PCR was conducted using 2 μL cDNA and Power SYBR Green PCR 2 × Master Mix (Applied Biosystems, CA, United States) according to the manufacturer’s recommended thermal cycling conditions (40 cycles of 15 s at 95°C and 60 s at 60°C). qRT-PCR was conducted using the Step One Plus Real-time PCR System and the data were analyzed using StepOne software v2.1 (Applied Biosystems, Foster City, CA, United States). Relative viral RNA expression levels were normalized by GAPDH gene according to the 2-△Ct method, and expressed as a ratio of infected controls.
Immunofluorescence assay (IFA)
MA-104 cells were cultured on 8-well chamber slides (LAB-TEK, NUNC, United States), and the cells were infected with rotavirus at 0.01 MOI for 1 h. The supernatant was removed and replaced with EMEM containing 1 μg/mL trypsin and 25 μM of the compounds. The cells were incubated for 24 h at 37°C in a 5% CO2 atmosphere, washed three times with PBS (pH 7.4), and fixed in 4% paraformaldehyde solution for 15 min at room temperature. After three washes with PBS (pH 7.4), the cells were incubated at 37°C for 1 h with a monoclonal antibody against rotavirus VP6 protein (Santa Cruz, California) diluted 1:50 in PBS (pH 7.4). After washing with PBS (pH 7.4), the cells were incubated at 37°C for 1 h with a FITC-conjugated goat anti-mouse IgG antibody (Santa Cruz) diluted 1:100 in PBS (pH 7.4). Cells were washed with PBS (pH 7.4), stained with 500 nM 4′,6-diamidino-2-phenylindole (DAPI) solution for 10 min at room temperature, and washed thrice with PBS (pH 8.0). Slides were mounted using the SlowFade Gold antifade reagent (Invitrogen, CA, United States). Confocal fluorescence imaging was performed using the Carl Zeiss LSM 510 META con-focal microscope (Carl Zeiss Inc., Jena, Germany). Confocal fluorescence imaging was quantified using ImageJ software 1.8.0.
Statistical analysis
All experiments were performed in triplicate. Data are expressed as mean ± SE. Statistical analysis was performed using Sigma Plot Statistical Analysis software. Differences between group mean values were determined using a one-way analysis of variance, followed by a Tukey’s test, assuming equal variances.
Results
Cytotoxicity of 18-hydroxyferruginol (1) and 18-oxoferruginol (2) isolated from T. nucifera in MA-104 cells
The structures of 18-hydroxyferruginol (1) and 18-oxoferruginol (2) are presented in Figure 1. Diterpenoid compounds were isolated from the ethanol extracts. The cytotoxicity of T. nucifera extracts and compounds was evaluated using the MTT assay at 50% cell cytotoxicity (CC50). Confluent MA-104 cells were incubated with EMEM in the absence or presence of a two-fold diluted EtOH extract (1–200 μg/mL) or compounds (1–100 μM) for 72 h, and MTT reagents were added to the cells. The cytotoxicity of the extracts and compounds differed at different concentrations. The EtOH extract of T. nucifera showed a high CC50 at 133.8 μg/mL (Table 1). The cytotoxicities of compounds 1 and 2 were determined as CC50 values of 62.7 and 40.7 μM (Table 1). Hence, at concentrations of compounds 1 and 2 < 25 μM, no toxic effects were observed on MA-104 cells. Subsequent experiments designed to assess the antiviral efficacy of the compounds were conducted at minimally toxic concentrations, resulting in >95% cell viability.
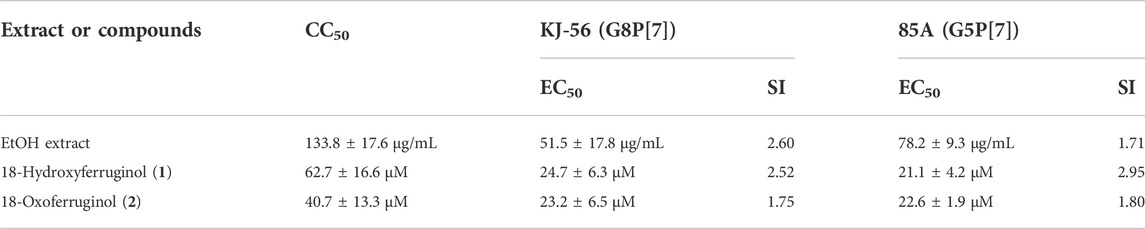
TABLE 1. In vitro anti-rotavirus activities for extract and two compounds isolated from Torreya nucifera against KJ-56 (bovine, G8P[7]) and 85A (porcine, G5P[7]) on MA-104 cells in post-treatment assay.
Antiviral activity of 18-hydroxyferruginol (1) and 18-oxoferruginol (2) on rotavirus replication
Time-of-addition experiments were performed to determine the viral replication stage at which the compounds exerted their maximum inhibitory effects. Our findings showed that 18-hydroxyferruginol (1) demonstrated the most antiviral activity against KJ-56 (G8P[7]) (EC50 = 24.7 μM, SI = 2.52) and 85A (G5P[7]) (EC50 = 21.1 μM, SI = 2.95) in the post-treatment assay. Moreover, 18-oxoferruginol (2) exerted antiviral activity against all strains, with KJ-56 (G8P[7]) (EC50 = 23.2 μM, SI = 1.75) and 85A (G5P[7]) (EC50 = 22.6 μM, SI = 1.80) (Table 1). These results indicate that both compounds 1 and 2 can effectively inhibit rotavirus during the post-treatment stage.
Inhibition of rotavirus viral RNA synthesis and viral protein
As rotavirus viral RNA is synthesized in the early (6 h) and late (18 h) stages, we tested the effects of these compounds on both synthesis stages in infected cells using qRT-PCR. As shown in Figure 2, viral RNA levels (NSP3 gene) were decreased by 76.6% (KJ-56) and 63.15% (85A) by compound 1 and by 13.25% (KJ-56) and 15.91% (85A) by compound 2 at 6 h. We showed that rotavirus viral RNA levels were markedly decreased by 81.5%–86.3% by compound 1% and 77.2%–95.0% by compound 2 (25 μM) in all virus strains at 18 h compared with infected/untreated cells (0.5% DMSO) (Figure 2). These results indicate that compounds 1 and 2 exerted a stronger inhibitory effect against KJ-56 (Bovine, G8P[7]) and 85A (Porcine, G5P[7]) on the late stage of viral replication than on the early stage. Notably, we examined the intracellular trafficking of the rotavirus VP6 protein in virus-infected cells using immunofluorescence. The VP6 protein was found mainly to show green fluorescence in the cytoplasm of rotavirus-infected cells (Figures 3B, F) but not in mock-infected MA-104 cells (Figures 3A, E). However, cell treatment with 25 μM of compounds 1 (Figures 3C, G) or 2 (Figures 3D, H) after infection reduced the number of fluorescence-positive cells in the cytoplasm, indicating that these compounds disturbed viral protein production (Figure 3I).
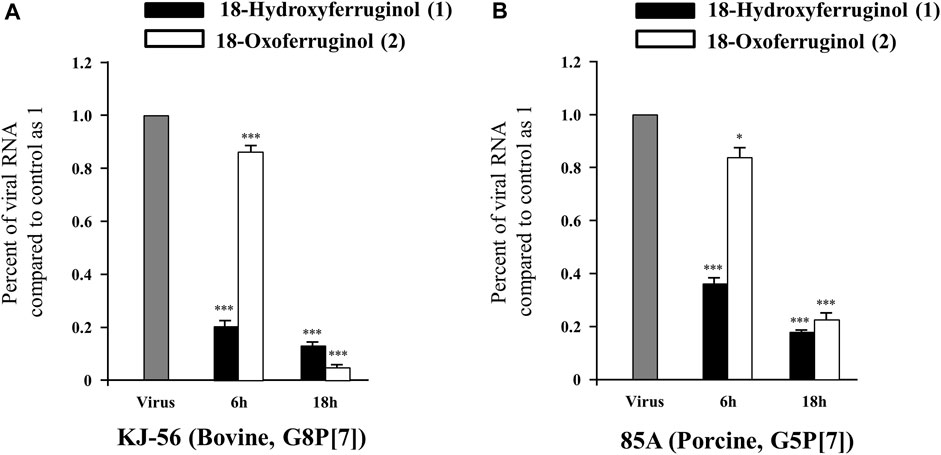
FIGURE 2. Effects of 18-hydroxyferruginol (1) and 18-oxoferruginol (2) on rotavirus viral RNA levels (NSP3 gene) by quantitative reverse transcription PCR. MA-104 cells were infected with rotavirus (0.01 MOI) for 1 h, viruses were removed, and cells were treated with 0.5% DMSO, 25 μM 18-hydroxyferruginol (1), or 25 μM 18-oxoferruginol (2) for 6 and 18 h after (A) KJ-56 (bovine rotavirus, G8P[7]) and (B) 85A (porcine rotavirus, G5P[7]) strain rotavirus infection. Total RNA was extracted after virus infection, and the level of the NSP3 gene was measured. Rotavirus RNA levels normalized to GAPDH. * p < 0.05; *** p < 0.001.
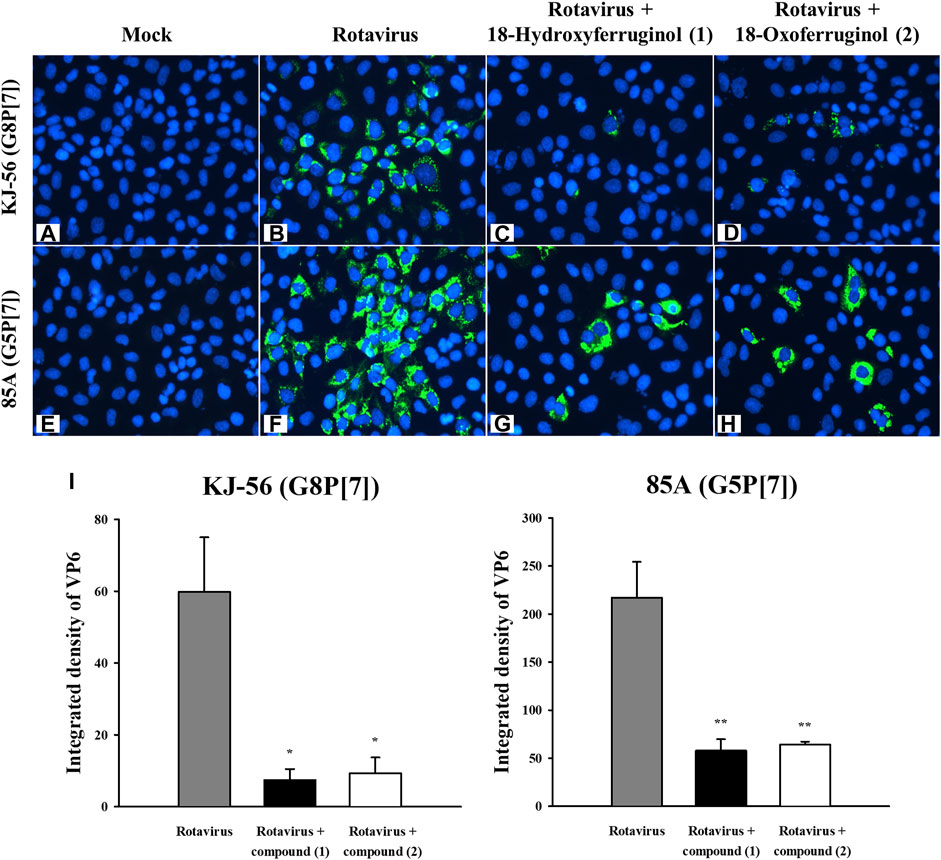
FIGURE 3. Effects of 18-hydroxyferruginol (1) and 18-oxoferruginol (2) on viral protein production (VP6) by immunofluorescence assay. MA-104 cells were infected with rotavirus at 0.01 MOI in the presence of (B,F) 0.5% DMSO, (C,G) 25 μM 18-hydroxyferruginol (1), or (D,H) 25 μM 18-oxoferruginol (2), or (A,E) mock-infected. After 24 h, the cells were fixed in 4% paraformaldehyde. After blocking, the cells were incubated with the anti-VP6 antibody (green). DAPI was used as a nuclear counterstain (blue). (I) Green fluorescence imaging of VP6 protein was quantified using ImageJ software 1.8.0. * p < 0.05; ** p < 0.01.
Discussion
Over the past few decades, medicinal plants have been increasingly studied and investigated as potential alternative antiviral agents. Moreover, a growing number of medicinal plants have been investigated for their potential antiviral efficacy in experimental and/or clinical settings and have been shown to affect different stages of the cycle of rotavirus infection (Takahashi et al., 2001; Tam and Roner, 2011; Huang et al., 2015; Jiang et al., 2016). In a previous study, abietane diterpenoids isolated from T. nucifera were reported to have antiviral activity against SARS-CoV 3CLpro and the influenza virus (Ryu et al., 2010; Bae et al., 2023). However, the anti-rotavirus activity of 18-hydroxyferruginol (1) and 18-oxoferruginol (2) are currently unclear. To our knowledge, this study demonstrates that compounds 1 and 2 isolated from T. nucifera inhibit the replication cycle of rotaviruses.
The targets of anti-rotavirus agents can be different stages of viral replication, including adsorption, cell penetration, uncoating, transcription, translation, assembly, and viral release from infected cells (Estes and Greenberg, 2013). We hypothesized that the antiviral effects of compounds 1 and 2 would act at two distinct time steps: 1) viral neutralization (virucidal) and/or 2) viral replication after entry into cells (post-treatment). In this study, the two compounds showed inhibitory effects against rotaviruses in a post-treatment assay against all strains (KJ-56 and 85A). We investigated the effects of compounds 1 and 2 on viral RNA synthesis and protein expression. We found that compounds 1 and 2 decreased rotavirus RNA synthesis. The IFA results also confirmed that compounds 1 and 2 suppressed the viral protein (VP6) synthesis in MA-104 cells (Figure 3). This suggests that these compounds are more effective at targeting rotavirus replication, potentially by disrupting the processes of viral RNA and protein synthesis.
Viral survival depends on the ability to evolve strategies that modulate host cell signaling pathways, especially those involved in apoptosis and cell survival (Cooray, 2004). Activation of the PI3K signaling pathway has been identified as a critical factor in boosting the production of infectious rotaviruses because it enhances the adhesion and survival of infected cells (Halasz et al., 2008). The activation of PI3K-dependent Akt signaling induces rotavirus infection, as observed in rotavirus-induced early apoptosis and rotavirus protein synthesis (Dutta et al., 2009; Bagchi et al., 2010; Bagchi et al., 2013). In a previous study, we reported that 18-hydroxyferruginol (1) and 18-oxoferruginol (2) from T. nucifera inhibited influenza virus infection by modulating the PI3K-Akt and ERK signaling pathways (Bae et al., 2023). The consequence of these events is the inhibition of viral RNP export from the nucleus and disruption of influenza virus replication (Bae et al., 2023). Therefore, we are currently attempting to determine the specific mechanisms through which these compounds act against rotaviruses.
In conclusion, this study showed that compounds 1 and 2 inhibited both G8P[7] bovine rotavirus and G5P[7] porcine rotavirus infections, probably by blocking viral replication after viral entry into the host cell. Therefore, we suggest that 18-hydroxyferruginol (1) and 18-oxoferruginol (2) have good potential as candidates for developing natural therapeutic drugs against rotaviruses.
Data availability statement
The datasets presented in this study can be found in online repositories. The names of the repository/repositories and accession number(s) can be found in the article/supplementary material.
Ethics statement
Ethical approval was not required for the studies on animals in accordance with the local legislation and institutional requirements because only commercially available established cell lines were used.
Author contributions
JP, JB, JJ, J-SK, and S-JP contributed to the research idea and design. J-SK and S-JP created the search strategy. J-SK and S-JP screened titles, abstracts, and full texts. JP, JB, JJ, J-SK, and S-JP contributed to data extraction and quality assessment. JP and JB contributed to the statistical analysis and interpretation of data. JB wrote the first draft of the manuscript. J-SK and S-JP edited the draft of the manuscript. All authors contributed to the article and approved the submitted version.
Funding
This research was supported by National Research Foundation grant funded by KRIBB Research Initiative Program (KGM5242322), Republic of Korea; and the National Research Foundation of Korea (NRF) grant funded by the Korea government (MSIT) (NRF-2021R1C1C2095006).
Conflict of interest
The authors declare that the research was conducted in the absence of any commercial or financial relationships that could be construed as a potential conflict of interest.
References
Bae, J., Kwon, H. J., Park, J. S., Jung, J., Ryu, Y. B., Kim, W. S., et al. (2023). Abietane diterpenoids isolated from torreya nucifera disrupt replication of influenza virus by blocking the phosphatidylinositol-3-kinase (PI3K)-Akt and ERK signaling pathway. Curr. issues Mol. Biol. 45, 2284–2295. doi:10.3390/cimb45030147
Bae, K. H. (2000). The medicinal plants of korea. Seoul, Republic of Korea: Kyo-Hak Publishing Co, Ltd, 45.
Bagchi, P., Dutta, D., Chattopadhyay, S., Mukherjee, A., Halder, U. C., Sarkar, S., et al. (2010). Rotavirus nonstructural protein 1 suppresses virus-induced cellular apoptosis to facilitate viral growth by activating the cell survival pathways during early stages of infection. J. virology 84, 6834–6845. doi:10.1128/JVI.00225-10
Bagchi, P., Nandi, S., Nayak, M. K., and Chawla-Sarkar, M. (2013). Molecular mechanism behind rotavirus NSP1-mediated PI3 kinase activation: interaction between NSP1 and the p85 subunit of PI3 kinase. J. virology 87, 2358–2362. doi:10.1128/JVI.02479-12
Cecílio, A. B., de Faria, D. B., Oliveira, P. D. C., Caldas, S., de Oliveira, D. A., Sobral, M. E., et al. (2012). Screening of brazilian medicinal plants for antiviral activity against rotavirus. J. Ethnopharmacol. 141, 975–981. doi:10.1016/j.jep.2012.03.031
Clark, K. J., Grant, P. G., Sarr, A. B., Belakere, J. R., Swaggerty, C. L., Phillips, T. D., et al. (1998). An in vitro study of theaflavins extracted from black tea to neutralize bovine rotavirus and bovine coronavirus infections. Veterinary Microbiol. 63, 147–157. doi:10.1016/s0378-1135(98)00242-9
Cooray, S. (2004). The pivotal role of phosphatidylinositol 3-kinase-Akt signal transduction in virus survival. J. general virology 85, 1065–1076. doi:10.1099/vir.0.19771-0
Crawford, S. E., Ramani, S., Tate, J. E., Parashar, U. D., Svensson, L., Hagbom, M., et al. (2017). Rotavirus infection. Nat. Rev. Dis. Prim. 3, 17083. doi:10.1038/nrdp.2017.83
Dutta, D., Bagchi, P., Chatterjee, A., Nayak, M. K., Mukherjee, A., Chattopadhyay, S., et al. (2009). The molecular chaperone heat shock protein-90 positively regulates rotavirus infection. Virology 391, 325–333. doi:10.1016/j.virol.2009.06.044
Estes, M. K., and Greenberg, H. B. (2013). Rotaviruses. Fields virology. 6th ed. (Philadelphia: Lippincott Williams & Wilkins), 1917–1974.
Grandy, G., Medina, M., Soria, R., Terán, C. G., and Araya, M. (2010). Probiotics in the treatment of acute rotavirus diarrhoea. A randomized, double-blind, controlled trial using two different probiotic preparations in Bolivian children. BMC Infect. Dis. 10, 253. doi:10.1186/1471-2334-10-253
Guarino, A., Canani, R. B., Russo, S., Albano, F., Canani, M. B., Ruggeri, F. M., et al. (1994). Oral immunoglobulins for treatment of acute rotaviral gastroenteritis. Pediatrics 93, 12–16. doi:10.1542/peds.93.1.12
Halasz, P., Holloway, G., Turner, S. J., and Coulson, B. S. (2008). Rotavirus replication in intestinal cells differentially regulates integrin expression by a phosphatidylinositol 3-kinase-dependent pathway, resulting in increased cell adhesion and virus yield. J. virology 82, 148–160. doi:10.1128/JVI.01980-07
Huang, H., Liao, D., Liang, L., Song, L., and Zhao, W. (2015). Genistein inhibits rotavirus replication and upregulates AQP4 expression in rotavirus-infected caco-2 cells. Archives virology 160, 1421–1433. doi:10.1007/s00705-015-2404-4
Jiang, Y., Yu, B., Yang, H., and Ma, T. (2016). Shikonin inhibits intestinal calcium-activated chloride channels and prevents rotaviral diarrhea. Front. Pharmacol. 7, 270. doi:10.3389/fphar.2016.00270
Linhares, R. E., Wigg, M. D., Lagrota, M. H., and Nozawa, C. M. (1989). The in vitro antiviral activity of isoprinosine on simian rotavirus (SA-11). Braz J Med Biol Res 22, 1095–1103.
Park, S. H., Saif, L. J., Jeong, C., Lim, G. K., Park, S. I., Kim, H. H., et al. (2006). Molecular characterization of novel G5 bovine rotavirus strains. J. Clin. Microbiol. 44, 4101–4112. doi:10.1128/JCM.01196-06
Ryu, Y. B., Jeong, H. J., Kim, J. H., Kim, Y. M., Park, J. Y., Kim, D., et al. (2010). Biflavonoids from torreya nucifera displaying SARS-CoV 3CL(pro) inhibition. Bioorg. Med. Chem. 18, 7940–7947. doi:10.1016/j.bmc.2010.09.035
Smee, D. F., Sidwell, R. W., Clark, S. M., Barnett, B. B., and Spendlove, R. S. (1982). Inhibition of rotaviruses by selected antiviral substances: mechanisms of viral inhibition and in vivo activity. Antimicrob. agents Chemother. 21, 66–73. doi:10.1128/AAC.21.1.66
Takahashi, K., Matsuda, M., Ohashi, K., Taniguchi, K., Nakagomi, O., Abe, Y., et al. (2001). Analysis of anti-rotavirus activity of extract from stevia rebaudiana. Antivir. Res. 49, 15–24. doi:10.1016/s0166-3542(00)00134-0
Tam, K. I., and Roner, M. R. (2011). Characterization of in vivo anti-rotavirus activities of saponin extracts from quillaja saponaria molina. Antivir. Res. 90, 231–241. doi:10.1016/j.antiviral.2011.04.004
Téllez, M. A., Téllez, A. N., Vélez, F., and Ulloa, J. C. (2015). In vitro antiviral activity against rotavirus and astrovirus infection exerted by substances obtained from achyrocline bogotensis (Kunth) DC. (Compositae). BMC complementary Altern. Med. 15, 428. doi:10.1186/s12906-015-0949-0
Keywords: abietane diterpenoids, Torreya nucifera, antiviral, rotavirus, viral replication
Citation: Park JS, Bae J, Jung J, Kim J-S and Park S-J (2023) In vitro antiviral activity of abietane diterpenoids isolated from Torreya nucifera against rotavirus infection. Acta Virol. 67:11630. doi: 10.3389/av.2023.11630
Received: 31 May 2023; Accepted: 10 August 2023;
Published: 22 August 2023.
Edited by:
Ivana Nemčovičová, Slovak Academy of Sciences, SlovakiaReviewed by:
Katarína Briestenská, Slovak Academy of Sciences, SlovakiaAyalew Mergia, University of Florida, United States
Copyright © 2023 Park, Bae, Jung, Kim and Park. This is an open-access article distributed under the terms of the Creative Commons Attribution License (CC BY). The use, distribution or reproduction in other forums is permitted, provided the original author(s) and the copyright owner(s) are credited and that the original publication in this journal is cited, in accordance with accepted academic practice. No use, distribution or reproduction is permitted which does not comply with these terms.
*Correspondence: Joong-Sun Kim, Y2VudHJhbGluZUBqbnUuYWMua3I=; Su-Jin Park, c2pwYXJrQGtyaWJiLnJlLmty
†These authors have contributed equally to this work