- 1Laboratorio de Virología, Departamento de Química Biológica, Facultad de Ciencias Exactas y Naturales, Universidad de Buenos Aires (UBA), Buenos Aires, Argentina
- 2Instituto de Química Biológica de la Facultad de Ciencias Exactas y Naturales, Consejo Nacional de Investigaciones Científicas y Técnicas (CONICET)-UBA, Ciudad de Universitaria, Buenos Aires, Argentina
Berberine (BBR) is a plant derived isoquinoline alkaloid that displays a great variety of biological activities. In this study we showed that BBR exhibits a strong antiviral activity with broad spectrum of inhibition against different strains of Zika virus (ZIKV) and the four serotypes of dengue virus (DENV) in Vero cells. The inhibitory effect of BBR on ZIKV and DENV-2 growth was also demonstrated in human cell lines. The analysis of BBR mode of action showed that the compound did not affect early events of viral multiplication cycle or viral protein expression. By contrast, BBR strongly impaired the formation of intracellular and extracellular infectious viral particles. Furthermore, BBR was able to inhibit ZIKV induced activation of ERK1/2 and p38 cell signaling pathways and also reduced p38 phosphorylation in uninfected cells. Like BBR, the p38 inhibitor SB202190 reduced p38 phosphorylation in both uninfected and ZIKV infected cultures and caused a significant diminishment of viral yields even when it was added late during the infection. These results suggest for the first time that the inhibitory effect of BBR on p38 signaling might contribute to the antiviral action of the compound against flaviviruses.
Introduction
Dengue (DENV) and Zika (ZIKV) are arthropod-borne viruses that belong to the family Flaviviridae, genus Flavivirus. There are four DENV serotypes (1–4) that cause frequent outbreaks in tropical and subtropical regions worldwide. DENV infection may be asymptomatic or may cause a mild or an acute flu-like illness called dengue fever, which can occasionally result in severe dengue with lethal complications. ZIKV is associated with neurological manifestations including encephalitis, meningitis and congenital malformations such as microcephaly in newborns (Jayachandran et al., 2021) The development of antiviral compounds against these viruses represents a major challenge for virology researchers.
Berberine (BBR) is an isoquinoline alkaloid extracted from roots, rhizome and outer bark of traditional medicine herbs belonging to the families Berberidaceae and Ranunculaceae. This compound presents promissory biological properties for the treatment of tumors, hyperlipidemia, inflammatory disorders and microbial infections (Singh et al., 2021; Majdalawieh et al., 2022).
It has been described that BBR displays antiviral activity against a great variety of human viruses, such as herpes simplex virus (HSV-1) (Chin et al., 2010), human cytomegalovirus (HCMV) (Luganini et al., 2019), human papilloma virus (HPV) (Mahata et al., 2011), influenza A virus (IAV) (Cecil et al., 2011; Wu et al., 2011; Yan et al., 2018; Botwina et al., 2020), respiratory syncytial virus (RSV) (Shin et al., 2015), Chikungunya virus (CHIKV) and other alphaviruses (Varghese et al., 2016a, 2016b; Wan et al., 2020), Coxsackievirus B Type 3 (CVB3) (Dai et al., 2017), enterovirus 71 (EV71) (Wang et al., 2017) and severe acute respiratory syndrome coronavirus 2 (SARS-CoV-2) (Pizzorno et al., 2020; Rodriguez-Rodriguez et al., 2021; Varghese et al., 2021). In addition, the in vitro inhibitory effect of BBR against members of the Flaviviridae family has been reported for ZIKV (Robinson et al., 2018), hepatitis C virus (HCV) (Hung et al., 2019) and DENV-2 (Ratanakomol et al., 2021). Moreover, for CHIKV (Wan et al., 2020), SARS-CoV-2 (Rodriguez-Rodriguez et al., 2021) DENV-2 (Ratanakomol et al., 2021) and ZIKV (Robinson et al., 2018; Batista et al., 2019; Ratanakomol et al., 2021) BBR also exhibited a direct inactivating activity against viral particles in the extracellular medium.
Different mechanisms for BBR antiviral action have been proposed, namely the inhibition of viral entry (Hung et al., 2019), the blockage of RNA or protein synthesis (Chin et al., 2010; Mahata et al., 2011; Shin et al., 2015; Dai et al., 2017; Wang et al., 2017; Luganini et al., 2019) or an adverse effect on late stages of viral multiplication cycle (Cecil et al., 2011; Varghese et al., 2016a, Varghese et al., 2016b; Wan et al., 2020; Varghese et al., 2021). Whereas the inhibitory mode of action of BBR against ZIKV has not been explored, it was proposed that the compound would inhibit the formation of DENV-2 viral particles by affecting cellular lipid metabolism (Ratanakomol et al., 2021).
The ability of BBR to modulate cellular signaling has been also investigated in an attempt to understand the molecular basis of BBR biological properties. In fact, several reports described the relation between the antiviral effect of BBR and nuclear factor κB (Song et al., 2014), mitogen activated protein kinases (MAPKs) (Shin et al., 2015; Varghese et al., 2016b; Dai et al., 2017; Wang et al., 2017; Botwina et al., 2020; Ratanakomol et al., 2021; Cui et al., 2022) and PI3K-Akt (Varghese et al., 2016b; Wang et al., 2017) pathways. Furthermore, BBR is a potent immunomodulatory and anti-inflammatory agent, which may prove to be useful for the treatment of viral infections that promote an excessive release of pro-inflammatory cytokines (Majdalawieh et al., 2022).
Here we first investigated the efficacy of BBR against the four DENV serotypes in comparison with different ZIKV strains and we further analyzed the mode of antiviral action of BBR also exploring whether ERK1/2 and p38 MAPKs are involved in the antiviral effect of the compound.
Materials and methods
Cells and viruses
Vero (ATCC CCL 81), A549 (ATCC CL-185) and HepG2 (ATCC CRL-10741) cells were grown in Eagle’s minimum essential medium (MEM, GIBCO) containing 5% inactivated calf serum and 50 μg/mL gentamycin. Maintenance medium (MM), pH 7.5, consisted of MEM supplemented with 1.5% calf serum and gentamycin. C6/36 mosquito cells (ATCC CRL-1660) were grown in L-15 medium (Leibovitz, GIBCO) supplemented with 10% fetal bovine serum, 0.3% tryptose phosphate broth, 1% MEM non-essential amino acids and 0.02% glutamine.
Stocks of DENV-1 (strain Hawaii, GenBank KM204119.1), DENV-2 (strain NGC, GenBank MK506264.1), DENV-3 (strain H87, GenBank KU050695), DENV-4 (strain 8124, provided by the Hospital de Niños Dr. Ricardo Gutierrez, Buenos Aires, Argentina), ZIKV (strains DAK-AR-41524, GenBank KX198134.2) and PRVABC59, GenBank MK713748.1) and the clinical ZIKV isolate INEVH116141 (provided by the Instituto Nacional de Enfermedades Virales Humanas, Pergamino, Argentina) were obtained in C6/36 cells and further titrated in Vero cells by plaque formation.
Cytotoxicity assay
Vero, A549 and HepG2 cells grown in 96 well microplates were treated with MM containing 0.5% dimethyl sulfoxide (DMSO) used as drug diluent (control) or different concentrations of BBR (Sigma-Aldrich, USA) for 24 h at 37°C and cell viability was then determined by the 3-(4,5-dimethylthiazol-2-yl)-2,5-diphenyl tetrazolium bromide (MTT, Sigma- Aldrich, USA) method. The 50% cytotoxic concentration (CC50), the concentration of BBR that reduced cell viability by 50% respect to control cultures, was determined. Similar cytotoxicity assays were performed for the synthetic compounds U0126 (#9903 Cell Signaling, USA), an inhibitor of ERK1/2 activation, and SB202190 (#8158, Cell Signaling, USA), a compound that impairs p38 phosphorylation, to determine the range of non-cytotoxic concentrations for each inhibitor.
Virus yield inhibition assay
Vero, A549 or HepG2 cells were infected with DENV or ZIKV (MOI = 1). After 1 h of incubation at 37°C, cells were incubated with MM containing DMSO 0.5% (control) or different concentrations of BBR, U0126 (15 µM) (Cell Signaling, USA) or SB202190 (40 µM) (Cell Signaling, USA) for 24 h at 37°C. Then, cell supernatants were harvested and extracellular virus yield was determined by plaque assay in Vero cells. The 50% effective concentration (EC50) of BBR, the concentration of compound that reduced viral yield by 50% respect to control cultures, was determined.
Other Vero cell cultures were infected with ZIKV (INEVH116141) or DENV-2 (MOI = 1) and after 1 h at 37°C, cells were treated with MM containing DMSO 0.5% (control), BBR (40 µM) or SB202190 (40 µM). After 24 h, cell supernatants were harvested to quantify extracellular viral titer whereas cells were covered with MM and disrupted by two cycles of freezing and thawing. After removing cell debris by low-speed centrifugation intracellular virus titer was determined by plaque assay in Vero cells.
Time of addition experiments
Vero cells were infected with ZIKV (INEVH116141) or DENV-2 (MOI = 1) and DMSO 0.5% (control) or BBR (40 µM) was added at 1, 2, 4, 6 or 8 h post- infection (p.i.). In other ZIKV (INEVH116141) (MOI = 1) infected cultures the inhibitor SB202190 (40 µM) was added at 1 or 8 h p.i. In both cases, at 24 h p.i. extracellular virus yield was determined by plaque assay in Vero cells. In other experiments Vero cells infected with ZIKV (INEVH116141) or DENV-2 (MOI = 1) were treated with DMSO 0.5% (control) or BBR (40 µM) during different intervals (1–24 h p.i, 1–8 h p.i. or 8–24 h p.i.). At 24 h p.i. extracellular virus yield was determined by plaque assay in Vero cells.
Western blot assay
Vero cells infected with ZIKV (INEVH116141) or DENV-2 (MOI = 1) were treated with DMSO 0.5% (control) or BBR (40 µM) during different intervals: 1–8 h p.i. or 8–24 h p.i. Other set of Vero cell cultures were infected with ZIKV (INEVH116141) (MOI = 1) and after 1 h of incubation at 37°C cells were treated with DMSO 0.5% (control), BBR (40 µM), U0126 (15 µM) or SB202190 (40 µM) for 24 h. In all cases cells were lysed and Western blot assay was performed as previously described (Quintana et al., 2016). The detection of viral E glycoprotein was performed using a mouse monoclonal antibody anti-flavivirus group antigen antibody, clone D1-4G2-4-15 (MAB10216, Millipore, USA). The relative protein expression level was normalized against glyceraldehyde 3-phosphate dehydrogenase (GAPDH) using a mouse anti-GAPDH (ab-9484, Abcam, UK) as primary antibody. Peroxidase conjugated anti-mouse immunoglobulins (Sigma-Aldrich, USA) were employed as secondary antibody. The analysis of ERK1/2 and p38 activation was done as previously described (Brunetti et al., 2018; Quintana et al., 2020; Brunetti et al., 2022). To examine ERK1/2 activation the primary antibodies employed were rabbit anti-phospho-ERK1/2 (p-ERK) (#9101, Cell Signaling, USA) and rabbit anti-ERK1/2 (ERK) (sc-514302, Santa Cruz Biotechnology, USA), whereas p38 activation was determined using rabbit anti-phospho-p38 (p-p38) (# 9211, Cell Signaling, USA) and rabbit anti-p38 (#8690, Cell Signaling, USA) as primary antibodies. In both cases, peroxidase-conjugated anti-rabbit IgGs (Promega, USA) were employed as secondary antibody. Protein bands were detected by chemiluminescence and quantified using ImageJ software.
Virucidal assay
Aliquots of ZIKV (INEVH116141) or DENV-2 suspensions were incubated for 2 h at 37°C with MM containing DMSO 0.5% (control) or different concentrations of BBR. Then, samples were diluted in MM, in order to achieve a low BBR concentration (0.1 µM) that is below the active antiviral concentrations, and remaining infectivity was determined by plaque assay in Vero cells.
Statistical analyses
Data represent the mean values obtained from three independent experiments. Duplicate samples were performed in each experiment. Statistical significance of the differences between control and drug-treated cultures was determined either by 2-tailed paired Student’s t-test or one-way ANOVA followed by Dunnett’s multiple comparison tests (Prism 7, GraphPad Software, Inc.). A p-value <0.05 was considered to be statistically significant; *p < 0.05, **p < 0.01 and ***p < 0.001.
Results
Inhibitory effect of BBR against different ZIKV strains and DENV serotypes
In order to assess the antiviral activity of BBR against ZIKV and DENV we first tested the effect of the compound on the viability of Vero cell monolayers. The incubation of cell cultures with BBR, within the concentration range 6.5–200 µM for 24 h did not exert cytotoxic effects (Figure 1A). By contrast, BBR treatment caused a dose dependent inhibition of virus yield in cells infected with the ZIKV clinical isolate INEVH116141 (Figure 1B) or the ZIKV strains PRVABC59 (Figure 1C) and DAK-AR-41524 (Figure 1D). The lowest value of EC50 was obtained against PRVABC59 strain, whereas similar susceptibility to BBR was observed for ZIKV (INEVH116141) and ZIKV (DAK-AR-41524) (Table 1). More than 99.9% of virus titer inhibition was achieved after treatment with 40 µM BBR for all the ZIKV strains assayed. On the other hand, BBR exhibited effectiveness against all DENV serotypes (Figures 2A–D), with EC50 values between 2.4 and 11.6 µM (Table 1). Treatment with the maximal concentration of BBR tested (40 µM) reduced virus yield by 98.0%, 99.1%, 96.4% and 92.4% for DENV-1, DENV-2, DENV-3 and DENV-4, respectively. We next examined the cytotoxic and antiviral effects of BBR in human cell lines. The inhibitory effect of BBR against ZIKV (INEVH116141) and DENV-2 was evident in A549 and HepG2 cells at non-cytotoxic concentrations of the compound and with similar EC50 values for each virus in both cell types (Figure 3; Table 1). At the maximal concentration of BBR assayed, the antiviral effect was stronger in HepG2 than in A549 cells, with 99.3% and 99.9% inhibition of virus production for DENV-2 and ZIKV, respectively (Figures 3D, F).
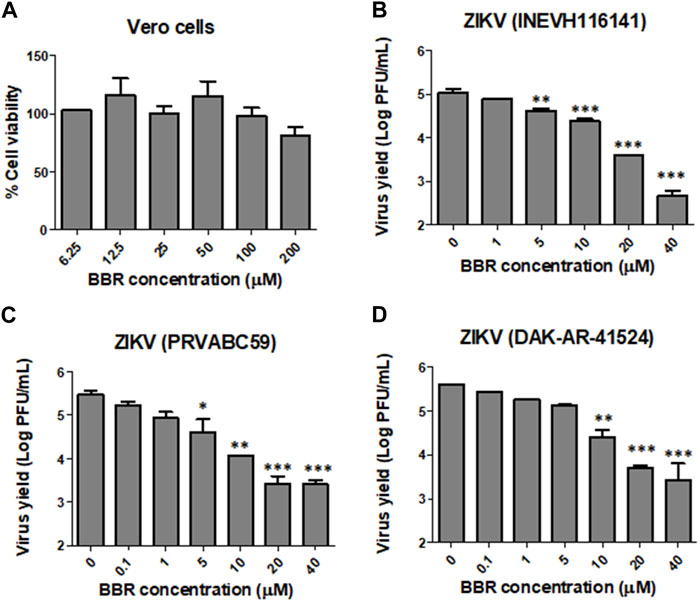
FIGURE 1. Inhibitory effect of BBR against ZIKV in Vero cells (A) Vero cells were treated with different concentrations of BBR for 24 h and the percentage of cell viability was determined by the MTT method. (B–D) Vero cells infected with ZIKV (B) INEVH116141, (C) PRVABC59 or (D) DAK-AR-41524 were treated with different concentrations of BBR for 24 h and extracellular virus titer was determined by plaque assay. Data are expressed as mean values of viral titer ±standard deviation (SD).
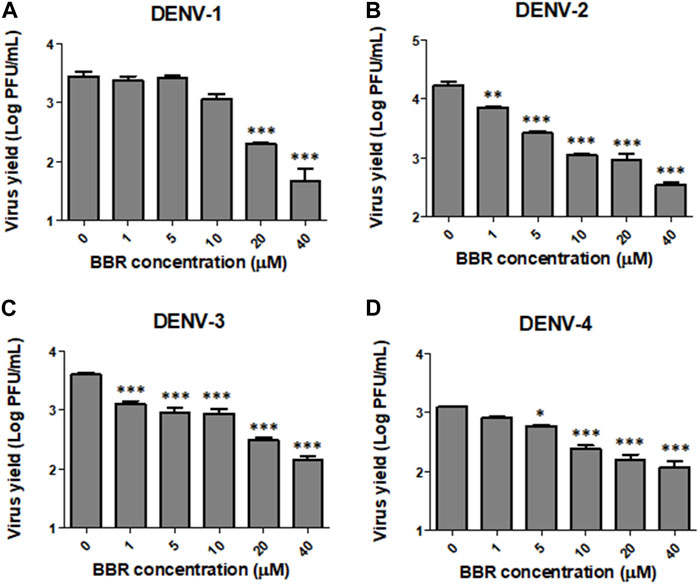
FIGURE 2. Inhibitory effect of BBR against DENV in Vero cells. Vero cells infected with (A) DENV-1, (B) DENV-2, (C) DENV-3 or (D) DENV-4 were treated with different concentrations of BBR for 24 h and extracellular virus titer was determined by plaque assay. Data are expressed as mean values of viral titer ±SD.
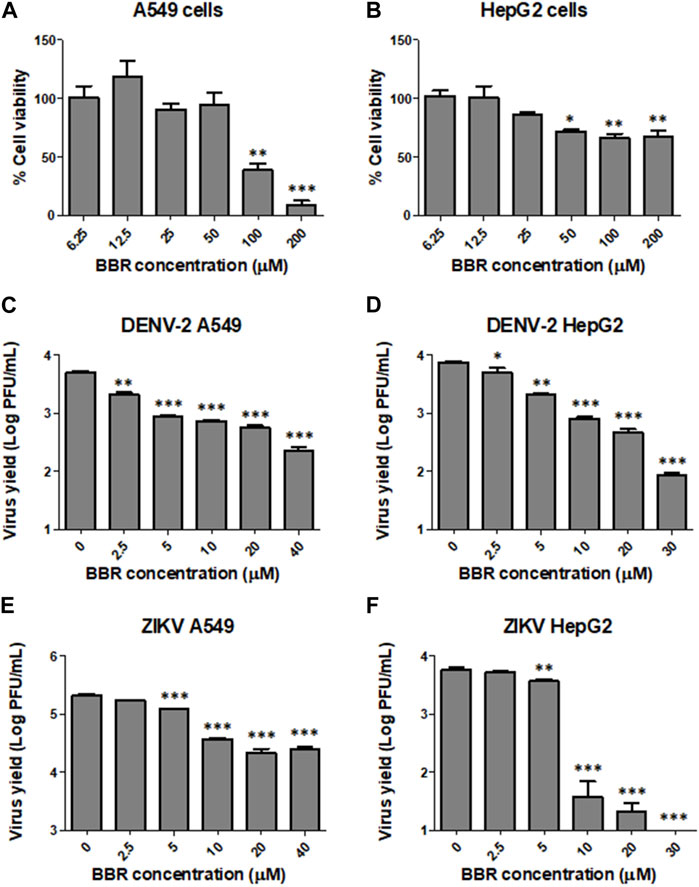
FIGURE 3. Inhibitory effect of BBR against ZIKV and DENV-2 in A549 and HepG2 cells (A) A549 and (B) HepG2 cells were treated with different concentrations of BBR for 24 h and the percentage of cell viability was determined by the MTT method. (C–D) DENV-2 infected (C) A549 or (D) HepG2 cells were treated with different concentrations of BBR for 24 h and extracellular virus titer was determined by plaque assay. (E–F) ZIKV (INEVH116141) infected (E) A549 or (F) HepG2 cells were treated with different concentrations of BBR for 24 h and extracellular virus titer was determined by plaque assay. Data are expressed as mean values of viral titer ±SD.
BBR antiviral mode of action
Next we decided to investigate the mode of antiviral action of BBR on ZIKV and DENV infections. Taking into account that BBR was similarly effective against the four DENV serotypes, DENV-2 was chosen as a representative serotype to further analyze the antiviral activity of the alkaloid against DENV infection. In order to assess whether BBR affects early or late stages of viral multiplication cycle we investigated the effect of the time of addition of BBR on extracellular ZIKV and DENV-2 production in Vero cells. For these experiments we used the 40 µM concentration of BBR since this compound concentration exerted a significant inhibitory effect on viral yield (Figures 1B, 2B). Figure 4A shows that similar high levels of viral yield inhibition were achieved when BBR was added up to 8 h p.i. Additionally, treatment with the compound only during the first 8 h of infection did not significantly affect ZIKV or DENV-2 infectious titers measured at 24 h p.i. indicating that the compound would not affect early events of viral multiplication cycle. By contrast, the presence of BBR from 8 to 24 h p.i. markedly reduced ZIKV and DENV-2 yields (Figure 4B). To determine whether BBR impairs viral protein production we analyzed the expression of E glycoprotein by Western blot. After treatment of ZIKV or DENV-2 infected Vero cells with BBR, either from 1 to 8 h p.i. or from 8 to 24 h p.i. non-significant changes in the level of E glycoprotein expression was detected indicating that the inhibitory effect of the compound would be exerted after viral protein synthesis (Figures 4C, D). Therefore, BBR would impair either the formation of progeny infectious viral particles or the release of infectious virions to the extracellular medium. To explore these possibilities we investigated the effect of BBR on intracellular and extracellular infectivity. For this purpose, ZIKV or DENV-2 infected cells were treated with BBR for 24 h and after harvesting culture supernatants to quantify released virions, cells were treated with low pH to inactivate viral particles adsorbed to cell membrane and then cell monolayers were disrupted to determine the number of intracellular infectious viral particles. Treatment with BBR caused 89.8% (for ZIKV) and 89.2% (for DENV-2) of inhibition of intracellular viral titer indicating that the alkaloid impairs the formation of mature viral particles. On the other hand, a higher level of inhibition was detected in extracellular titers in comparison with intracellular ones. Extracellular viral titer was reduced by 99.1% and 98.9% in ZIKV and DENV-2 infected cells, respectively (Figures 5A, B). These results may suggest that the release of mature virions might be also affected. As it was mentioned in section 1, it has been described that BBR exhibits a direct inactivating effect on ZIKV and DENV-2 particles (Robinson et al., 2018; Batista et al., 2019; Ratanakomol et al., 2021) and this property may contribute to the strong reduction of extracellular viral titers. Therefore, we examined whether BBR displays virucidal action against ZIKV (INEVH116141) and DENV-2 under our experimental conditions. As it is shown in Figure 5C, the incubation of ZIKV or DENV-2 particles with different concentrations of BBR for 2 h at 37°C caused the inactivation of viral particles in a dose dependent manner corroborating that besides its inhibitory action, BBR exhibits inactivating properties mainly at high compound concentrations. Maximal virucidal action, 95.7% for ZIKV and 67.3% for DENV-2, was observed at 160 µM of BBR (Figure 5C), whereas at 40 µM of BBR, the compound concentration used in the mechanistic studies, the reduction of viral particle infectivity was 60.7% and 54.6% for ZIKV and DENV-2, respectively.
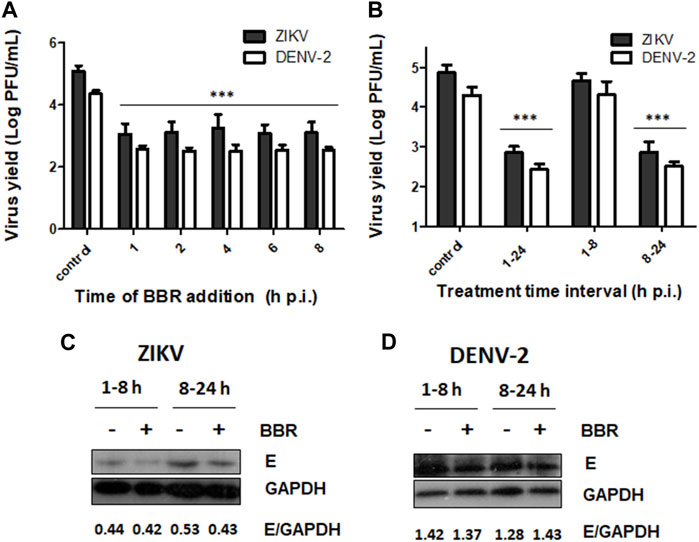
FIGURE 4. Effect of time of BBR treatment on ZIKV and DENV-2 yields and protein expression (A) BBR (40 µM) was added to Vero cells at different time points after ZIKV (INEVH116141) or DENV-2 infection. At 24 h p.i. extracellular virus titers were determined by plaque assay. (B) Vero cells infected with ZIKV (INEVH116141) or DENV-2 were treated with BBR (40 µM) from 1 to 8 h p.i. or from 8 to 24 h p.i. At 24 h p.i., extracellular virus titers were determined by plaque assay. In (A) and (B) data are expressed as mean values of viral titer ±SD. (C–D) Vero cells infected with (C) ZIKV (INEVH116141) or (D) DENV-2 were treated with BBR (40 µM) from 1 to 8 h p.i. or from 8 to 24 h p.i. At 24 h p.i., the expression of E glycoprotein and GAPDH was assessed by Western blot. The mean value of the ratio E/GAPDH obtained from densitometry is indicated below the respective protein panels.
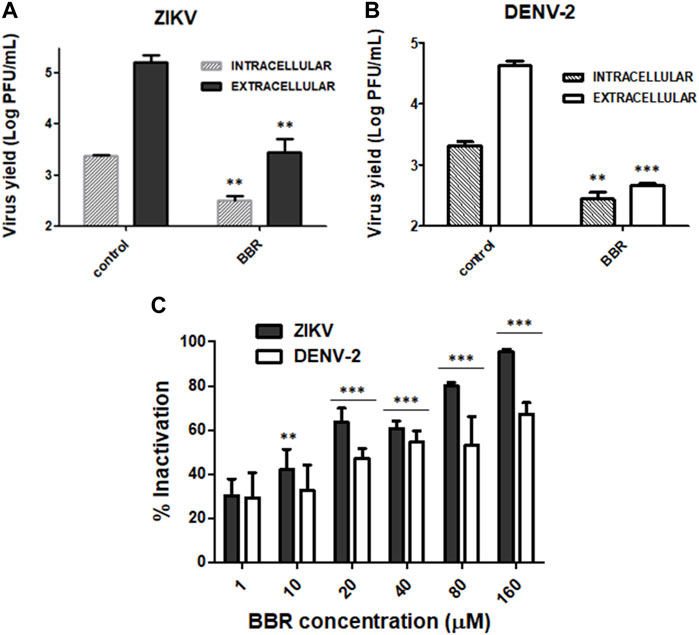
FIGURE 5. Effects of BBR on intracellular and extracellular viral production and infectivity of viral particles (A) ZIKV (INEVH116141) or (B) DENV-2 infected Vero cells were treated with BBR (40 µM). At 24 h p.i. extracellular viral infectious titer was determined from culture medium whereas intracellular infectious viral particles were quantified after disruption of cells by freezing and thawing cycles. Data are expressed as mean values of viral titer ±SD. (C) ZIKV (INEVH116141) or DENV-2 suspensions were incubated with MM containing different concentrations of BBR for 2 h at 37°C. After diluting with MM, the remaining infectivity was measured by plaque assay. Data represent the mean values ±SD of the percentage of inactivated viral particles with respect to control viral suspensions.
Modulation of ERK1/2 and p38 MAPKs mediated by BBR in ZIKV infected cells
Finally we examined the effect of BBR on the activation state of ERK1/2 and p38 MAPK pathways. In these assays we also employed two well-known MAPK inhibitors: the compound U0126, which is a potent non-ATP competitive inhibitor of MEK, the upstream kinase that phosphorylates ERK1/2 and the compound SB202190 that inhibits the phosphorylation of p38 (Davies et al., 2000). In a previous report we demonstrated that DENV-2 was able to induce p38 activation in Vero cells and we also confirmed that treatment with SB202190 inhibited viral multiplication and p38 phosphorylation (Quintana et al., 2020). Therefore, we decided to analyze the effect of BBR and the inhibitors of ERK1/2 and p38 kinases on MAPK activation and viral production in the context of ZIKV infection. To this end, Vero cells infected or not with ZIKV (INEVH116141) were treated with BBR (40 µM), U0126 (15 µM) or SB202190 (40 µM). ZIKV infection promoted the activation of both ERK1/2 and p38 signaling pathways (Figures 6A, B). While BBR treatment did not alter basal levels of ERK1/2 phosphorylation in uninfected cultures the alkaloid produced a significant inhibition of p-ERK1/2 in ZIKV infected cells. On the contrary, the inhibitor U0126 caused a significant diminishment in p-ERK1/2 level both in ZIKV infected and uninfected cells (Figure 6A). On the other hand, the presence of BBR or SB202190 reduced p-p38 levels both in uninfected and ZIKV infected cells (Figure 6B) suggesting that BBR might affect p38 activation in a virus independent manner. In addition, the three assessed compounds markedly reduced viral yield after treatment for 24 h. Whereas BBR and SB202190 inhibited extracellular viral production by 99.7% and 99.8%, respectively, treatment with U0126 reduced viral yield by 94.0% (Figure 6C). In line with the results obtained with BBR (Figure 5A), besides the strong inhibition on extracellular virus production, treatment with SB202190 from 1 to 24 h p.i. also exerted a significant diminishment (97.8%) of intracellular virus titer with respect to untreated infected cells (Figure 6D). Furthermore, as it was observed in BBR treated cultures (Figure 4A) late addition of SB202190 at 8 h p.i. still caused a high reduction (98.5%) of viral yield respect to the control (Figure 6E).
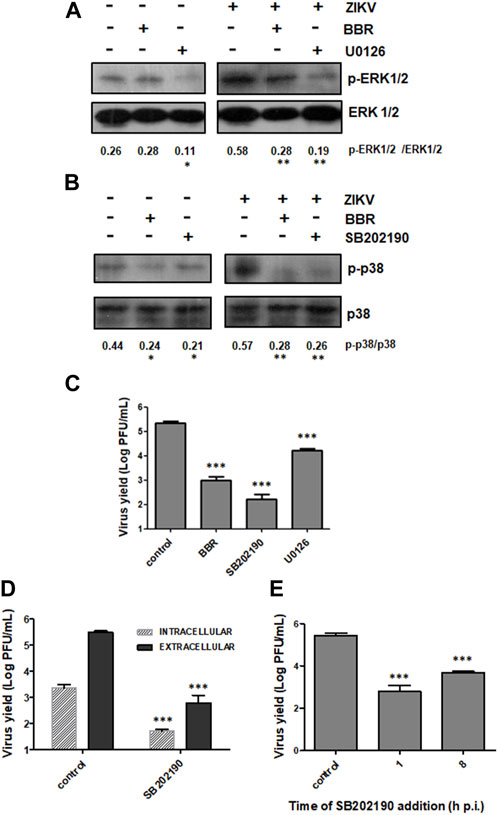
FIGURE 6. Effect of BBR and MAPK inhibitors on ZIKV production and modulation of signaling pathways (A–C) Vero cells uninfected or infected with ZIKV (INEVH116141) were treated or not with BBR (40 µM), U0126 (15 µM) or SB202190 (40 µM) for 24 h. (A) p-ERK1/2 and total ERK1/2 levels of expression were determined by Western blot. The mean value of the ratio p-ERK1/2/ERK1/2 obtained from densitometry is indicated below the protein panel. (B) p-p38 and p38 levels of expression were determined by Western blot. The mean value of the ratio p-p38/p38 obtained from densitometry is indicated below the protein panel. (C) Extracellular viral titers were determined by plaque assay. (D) ZIKV infected Vero cells were treated or not with SB202190 (40 µM) for 24 h and extracellular and intracellular viral titers were determined by plaque assay. (E) Vero cells were infected with ZIKV and at 1 or 8 h p.i. cells were treated or not with SB202190 (40 µM). At 24 h p.i., extracellular viral yield was determined by plaque assay. In (C D, E) data are expressed as mean values of viral titer ±SD.
Discussion
Despite the fact that DENV, ZIKV and other members of the Flavivirus genus are the arboviruses with the highest impact on human health there are currently no approved antiviral drugs to face these viral infections. In the last years, the potential of the plant derived alkaloid BBR as an antiviral agent against several DNA and RNA human viruses has been explored. A previous study demonstrated that BBR inhibits the replication of ZIKV strain PRVABC59 in mouse germ cells and ZIKV strain MR766 in mouse germ cells and primary human testicular cells (Robinson et al., 2018). The inhibitory effect of BBR against DENV-2 (strain 16681) growth in BHK-21 cells was also recently reported (Ratanakomol et al., 2021). In both cases, and in agreement with our results obtained in Vero, HepG2 and A549 cells, the EC50 values for BBR against ZIKV and DENV-2 were in the low micromolar range. On the other hand, here we report that BBR is able to inhibit the multiplication of all DENV serotypes, the two ZIKV strains tested and a local clinical ZIKV isolate. All together these results indicate that the compound is effective independently of the virus serotype or strain assayed and exhibits strong antiviral effects in different cell types.
One-step growth curves previously reported for DENV-2 and ZIKV in Vero cells showed a replication cycle duration of approximately 24 h (Mukherjee et al., 2016; Moser et al., 2018). Time of addition experiments performed in the present study showed that the delayed addition of BBR at 8 h p.i. caused a high inhibition of ZIKV and DENV- 2 production. Moreover, treatment with the compound only during the first hours of infection did not significantly affect extracellular virus production. Therefore, BBR would not affect early stages of ZIKV and DENV-2 infection. In addition, even though BBR markedly reduced virus titers in cultures incubated with the compound from 8 to 24 h p.i., this treatment did not affect the expression of the structural protein E in ZIKV and DENV-2 infected cultures as determined by Western blot analysis. Studies performed with different viruses such as HSV, HCMV, HPV, RSV, CVB3 and EV71, showed that BBR inhibits viral protein or RNA synthesis (Chin et al., 2010; Mahata et al., 2011; Shin et al., 2015; Dai et al., 2017; Wang et al., 2017; Luganini et al., 2019). However, our findings indicate that BBR would exert its antiviral effect against ZIKV and DENV-2 after the synthesis of viral components, either affecting the formation of mature viral particles or their release to the extracellular medium. It is interesting to note that it has been described that treatment of DENV-2 infected BHK-21 cells with BBR exhibited an augmented expression of E protein in comparison to untreated cells (Ratanakomol et al., 2021). Our experiments did not reveal significative changes in ZIKV or DENV-2 E protein expression in the presence of BBR with respect to untreated Vero cells. Differences in the DENV-2 strain tested or the cell system employed might account for the discrepancy between our experiments and those reported by Ratanakomol et al. (2021). In accordance with our findings, BBR did not affect the production of viral proteins in Raw 264.7 cells infected with IAV (Cecil et al., 2011) or BHK-21 cells infected with the alphavirus Semliki Forest (SFV) (Wan et al., 2020).
On the other hand, our findings showed that BBR was able to strongly reduce intracellular infectious viral titers, thus the compound seems to target the assembly or the maturation of viral particles. Consistent with these results, Ratanakomol et al. (2021) provided evidence that BBR alters cellular lipid metabolism and suggested that this effect would result in an altered viral particle assembly. Furthermore, BBR significantly inhibited the production of infectious virus in BHK-21 cells infected with SFV or CHIKV (Varghese et al., 2016a) and Vero E6 cells infected with SARS-CoV-2 (Varghese et al., 2021) even when the compound was added at late stages of infection, and experimental evidence pointed out viral assembly as the target of BBR inhibitory action (Wan et al., 2020; Varghese et al., 2021).
In addition, in comparison to intracellular viral titers, we found a higher inhibition of extracellular viral titers in ZIKV and DENV-2 infected cells treated with BBR. The inactivating effect of BBR on free extracellular viral particles might contribute to this result. However, we cannot exclude an additional adverse effect of BBR on the egress of mature infectious viral particles.
We also studied the effect of BBR on ERK1/2 and p38 MAPK pathways. Several studies revealed that ZIKV and DENV induce the activation of ERK1/2 and p38 MAPK signaling in different cell systems (Zhu et al., 2017; Sreekanth et al., 2018; Rashid et al., 2020; Muthuraj et al., 2021; Sabino et al., 2021). Other studies showed that inhibition of ERK 1/2 or p38 MAPKs reduced levels of viral yields in DENV-2 (Sreekanth et al., 2018; Quintana et al., 2020) and ZIKV (Cheng et al., 2018; Ye et al., 2022) in infected cells. In agreement with the abovementioned reports, our study showed that ZIKV promoted the activation of both ERK1/2 and p38 MAPKs in Vero cells and treatment with chemical compounds that specifically inhibit these pathways significantly reduced virus yield. Unlike U0126, which is a selective inhibitor of ERK1/2 pathway, BBR inhibited ERK1/2 phosphorylation in ZIKV infected cells without affecting p-ERK1/2 basal levels in uninfected ones. These results suggest that inhibition of ERK1/2 activation in BBR treated infected cells might be a consequence of the antiviral effect of BBR on viral replication rather than an adverse effect of the compound on ERK1/2 cascade itself. Furthermore, it has been described that ERK1/2 MAPK inhibitors would target the entry of ZIKV into the host cell (Sabino et al., 2021). On the other hand, basal and ZIKV induced p38 phosphorylation was reduced by treatment with either BBR or the p38 inhibitor SB202190 indicating that BBR would be able to block p38 MAPK activity. Furthermore, similarly to BBR, the inhibitor SB20219 effectively impaired ZIKV multiplication when the compound was added late during infection and markedly blocked the formation of progeny infectious virus. Collectively, these results suggest that the inhibitory effect of BBR on p38 MAPK might contribute to the antiviral action of the compound. The involvement of p38 signaling in the antiviral activity of BBR has been also proposed for RSV (Shin et al., 2015), CVB3 (Dai et al., 2017) and CHIKV (Varghese et al., 2016b) in human cell lines, but the accomplishment of further studies is needed for the better understanding of this connection. It has been proved that MAPK inhibitors mitigate DENV-2 pathogenesis by downregulating cytokine production (Sreekanth et al., 2018). BBR not only affects the activation of MAPK signaling but also reduces the production of proinflammatory cytokines induced by different viruses (Chin et al., 2010; Cecil et al., 2011; Wu et al., 2011; Varghese et al., 2016b; Yan et al., 2018). Therefore, it can be speculated that BBR would restrain DENV induced inflammatory response improving the treatment of dengue disease.
It is noteworthy that besides its wide range of antiviral activity in cell cultures, BBR has shown antiviral efficacy in animal models interfering with the replication of ZIKV (Robinson et al., 2018) and other viruses like IAV (Wu et al., 2011; Yan et al., 2018), CHIKV (Varghese et al., 2016b) and CVB3 (Dai et al., 2021). Moreover, in order to improve BBR bioavailability, an oral immunotherapeutic BBR nanomedicine for treating food allergy has been developed (Wang et al., 2021). Given that BBR displays a great variety of biological properties, novel strategies for drug administration will be relevant for the potential use of BBR in the treatment of other human pathologies. Additionally, several synthetic BBR derivatives also showed antiviral action against different viruses (Enkhtaivan et al., 2017; Wang et al., 2018; Zeng et al., 2020).
In conclusion, we have shown that BBR, a promising bioactive molecule, has a strong and wide range antiviral activity against ZIKV strains of both historical African and recent Asian lineages and the four DENV serotypes. BBR mainly affects a late stage of viral multiplication cycle impairing the formation of infectious viral particles and our results also suggest for the first time that p38 MAPK inhibition mediated by BBR would be relevant for the antiviral action of this alkaloid against flaviviruses.
Data availability statement
The original contributions presented in the study are included in the article/supplementary material, further inquiries can be directed to the corresponding author.
Author contributions
Conducted experiments: DG, LP, JB, and VQ; Participated in research conception and design: LP and VC; Wrote and drafted the manuscript: VC; Critical revision of the article: ED; Performed data analysis: DG and LP; Contributed to writing the manuscript: LP. All authors contributed to the article and approved the submitted version.
Funding
This work was supported by a grant from the Universidad de Buenos Aires (20020190100211BA).
Acknowledgments
ED is a member of the Research Career from Consejo Nacional de Investigaciones Científicas y Técnicas (CONICET).
Conflict of interest
The authors declare that the research was conducted in the absence of any commercial or financial relationships that could be construed as a potential conflict of interest.
Abbreviations
BBR, berberine; CC50, 50% cytotoxic concentration; CHIKV, Chikungunya virus; CVB3, Coxsackievirus B Type 3; DENV, dengue virus; DMSO, dimethyl sulfoxide; EC50, 50% effective concentration; EV71, enterovirus 71; ERK, extra-cellular regulated kinase; HSV, herpes simplex virus; HCMV, human cytomegalovirus; HPV, human papilloma virus; IAV, influenza A virus; MAPK, mitogen activated protein kinase; MEM, Eagle’s minimum essential medium; MM, maintenance medium; MTT, 3-(4,5-dimethylthiazol-2-yl)-2,5-diphenyl tetrazolium bromide; p.i., post-infection; RSV, respiratory syncytial virus; SARS-CoV-2, severe acute respiratory syndrome coronavirus 2; SFV, Semliki Forest virus; SI, selectivity index; ZIKV, Zika virus.
References
Batista, M. N., Braga, A. C. S., Campos, G. R. F., Souza, M. M., Matos, R. P. A., Lopes, T. Z., et al. (2019). Natural products isolated from oriental medicinal herbs inactivate zika virus. Viruses 11 (1), 49. doi:10.3390/v11010049
Botwina, P., Owczarek, K., Rajfur, Z., Ochman, M., Urlik, M., Nowakowska, M., et al. (2020). Berberine hampers influenza a replication through inhibition of MAPK/ERK pathway. Viruses 12 (3), 344. doi:10.3390/v12030344
Brunetti, J. E., Foscaldi, S., Quintana, V. M., Scolaro, L. A., López, N., and Castilla, V. (2018). Role of the ERK1/2 signaling pathway in the replication of junín and tacaribe viruses. Viruses 10 (4), 199. doi:10.3390/v10040199
Brunetti, J. E., Quintana, V. M., Scolaro, L. A., and Castilla, V. (2022). Inhibitors of the p38 cell signaling pathway as antiviral compounds against junín virus. Arch. Virol. 167 (3), 935–940. doi:10.1007/s00705-022-05388-9
Cecil, C. E., Davis, J. M., Cech, N. B., and Laster, S. M. (2011). Inhibition of H1N1 influenza a virus growth and induction of inflammatory mediators by the isoquinoline alkaloid berberine and extracts of goldenseal (hydrastis canadensis). Int. Immunopharmacol. 11 (11), 1706–1714. doi:10.1016/j.intimp.2011.06.002
Cheng, F., Ramos da Silva, S., Huang, I. C., Jung, J. U., and Gao, S. J. (2018). Suppression of zika virus infection and replication in endothelial cells and astrocytes by PKA inhibitor PKI 14-22. J. Virol. 92 (4), e02019–17. doi:10.1128/jvi.02019-17
Chin, L. W., Cheng, Y. W., Lin, S. S., Lai, Y. Y., Lin, L. Y., Chou, M. Y., et al. (2010). Anti-herpes simplex virus effects of berberine from coptidis rhizoma, a major component of a chinese herbal medicine, Ching-Wei-San. Arch. Virol. 155 (12), 1933–1941. doi:10.1007/s00705-010-0779-9
Cui, Y., Zhang, L., Hu, D., and Yang, Y. (2022). Berberine inhibits herpes simplex virus 1 replication in HEK293T cells. Comput. Math. Methods Med. 2022, 7137401–7137407. doi:10.1155/2022/7137401
Dai, Q., He, X., Yu, H., Bai, Y., Jiang, L., Sheng, H., et al. (2021). Berberine impairs coxsackievirus B3-induced myocarditis through the inhibition of virus replication and host pro-inflammatory response. J. Med. Virol. 93 (6), 3581–3589. doi:10.1002/jmv.26747
Dai, Q., Zhang, D., Yu, H., Xie, W., Xin, R., Wang, L., et al. (2017). Berberine restricts coxsackievirus B type 3 replication via inhibition of c-jun N-terminal kinase (JNK) and p38 MAPK activation in vitro. Med. Sci. Monit. 23, 1448–1455. doi:10.12659/msm.899804
Davies, S. P., Reddy, H., Caivano, M., and Cohen, P. (2000). Specificity and mechanism of action of some commonly used protein kinase inhibitors. Biochem. J. 351 (1), 95–105. doi:10.1042/bj3510095
Enkhtaivan, G., Muthuraman, P., Kim, D. H., and Mistry, B. (2017). Discovery of berberine based derivatives as anti-influenza agent through blocking of neuraminidase. Bioorg Med. Chem. 25 (20), 5185–5193. doi:10.1016/j.bmc.2017.07.006
Hung, T. C., Jassey, A., Liu, C. H., Lin, C. J., Lin, C. C., Wong, S. H., et al. (2019). Berberine inhibits hepatitis C virus entry by targeting the viral E2 glycoprotein. Phytomedicine 53, 62–69. doi:10.1016/j.phymed.2018.09.025
Jayachandran, B., Chanda, K., and Balamurali, M. M. (2021). Overview of pathogenesis, diagnostics, and therapeutics of infectious diseases: dengue and zika. ACS Omega 6 (35), 22487–22496. doi:10.1021/acsomega.1c03536
Luganini, A., Mercorelli, B., Messa, L., Palù, G., Gribaudo, G., and Loregian, A. (2019). The isoquinoline alkaloid berberine inhibits human cytomegalovirus replication by interfering with the viral Immediate Early-2 (IE2) protein transactivating activity. Antivir. Res. 164, 52–60. doi:10.1016/j.antiviral.2019.02.006
Mahata, S., Bharti, A. C., Shukla, S., Tyagi, A., Husain, S. A., and Das, B. C. (2011). Berberine modulates AP-1 activity to suppress HPV transcription and downstream signaling to induce growth arrest and apoptosis in cervical cancer cells. Mol. Cancer 10, 39. doi:10.1186/1476-4598-10-39
Majdalawieh, A. F., Yousef, S. M., Abu-Yousef, I. A., and Nasrallah, G. K. (2022). Immunomodulatory and anti-inflammatory effects of berberine in lung tissue and its potential application in prophylaxis and treatment of COVID-19. Front. Biosci. (Landmark Ed). 27 (5), 166. doi:10.31083/j.fbl2705166
Moser, L. A., Boylan, B. T., Moreira, F. R., Myers, L. J., Svenson, E. L., Fedorova, N. B., et al. (2018). Growth and adaptation of zika virus in mammalian and mosquito cells. PLoS Negl. Trop. Dis. 12 (11), e0006880. doi:10.1371/journal.pntd.0006880
Mukherjee, S., Sirohi, D., Dowd, K. A., Chen, Z., Diamond, M. S., Kuhn, R. J., et al. (2016). Enhancing dengue virus maturation using a stable furin over-expressing cell line. Virology 497, 33–40. doi:10.1016/j.virol.2016.06.022
Muthuraj, P. G., Sahoo, P. K., Kraus, M., Bruett, T., Annamalai, A. S., Pattnaik, A., et al. (2021). Zika virus infection induces endoplasmic reticulum stress and apoptosis in placental trophoblasts. Cell Death Discov. 7 (1), 24. doi:10.1038/s41420-020-00379-8
Pizzorno, A., Padey, B., Dubois, J., Julien, T., Traversier, A., Dulière, V., et al. (2020). In vitro evaluation of antiviral activity of single and combined repurposable drugs against SARS-CoV-2. Antivir. Res. 181, 104878. doi:10.1016/j.antiviral.2020.104878
Quintana, V. M., Piccini, L. E., Panozzo Zénere, J. D., Damonte, E. B., Ponce, M. A., and Castilla, V. (2016). Antiviral activity of natural and synthetic β-carbolines against dengue virus. Antivir. Res. 134, 26–33. doi:10.1016/j.antiviral.2016.08.018
Quintana, V. M., Selisko, B., Brunetti, J. E., Eydoux, C., Guillemot, J. C., Canard, B., et al. (2020). Antiviral activity of the natural alkaloid anisomycin against dengue and zika viruses. Antivir. Res. 176, 104749. doi:10.1016/j.antiviral.2020.104749
Rashid, M. U., Zahedi-Amiri, A., Glover, K. K. M., Gao, A., Nickol, M. E., Kindrachuk, J., et al. (2020). Zika virus dysregulates human sertoli cell proteins involved in spermatogenesis with little effect on tight junctions. PLoS Negl. Trop. Dis. 14 (6), e0008335. doi:10.1371/journal.pntd.0008335
Ratanakomol, T., Roytrakul, S., Wikan, N., and Smith, D. R. (2021). Berberine inhibits dengue virus through dual mechanisms. Molecules 26 (18), 5501. doi:10.3390/molecules26185501
Robinson, C. L., Chong, A. C. N., Ashbrook, A. W., Jeng, G., Jin, J., Chen, H., et al. (2018). Male germ cells support long-term propagation of zika virus. Nat. Commun. 9 (1), 2090. doi:10.1038/s41467-018-04444-w
Rodriguez-Rodriguez, B. A., Noval, M. G., Kaczmarek, M. E., Jang, K. K., Thannickal, S. A., Cifuentes Kottkamp, A., et al. (2021). Atovaquone and berberine chloride reduce SARS-CoV-2 replication in vitro. Viruses 13 (12), 2437. doi:10.3390/v13122437
Sabino, C., Bender, D., Herrlein, M. L., and Hildt, E. (2021). The epidermal growth factor receptor is a relevant host factor in the early stages of the zika virus life cycle in vitro. J. Virol. 95 (20), e0119521. doi:10.1128/jvi.01195-21
Shin, H. B., Choi, M. S., Yi, C. M., Lee, J., Kim, N. J., and Inn, K. S. (2015). Inhibition of respiratory syncytial virus replication and virus-induced p38 kinase activity by berberine. Int. Immunopharmacol. 27 (1), 65–68. doi:10.1016/j.intimp.2015.04.045
Singh, S., Pathak, N., Fatima, E., and Negi, A. S. (2021). Plant isoquinoline alkaloids: advances in the chemistry and biology of berberine. Eur. J. Med. Chem. 226, 113839. doi:10.1016/j.ejmech.2021.113839
Song, S., Qiu, M., Chu, Y., Chen, D., Wang, X., Su, A., et al. (2014). Downregulation of cellular c-Jun N-terminal protein kinase and NF-κB activation by berberine may result in inhibition of herpes simplex virus replication. Antimicrob. Agents Chemother. 58 (9), 5068–5078. doi:10.1128/aac.02427-14
Sreekanth, G. P., Yenchitsomanus, P. T., and Limjindaporn, T. (2018). Role of mitogen-activated protein kinase signaling in the pathogenesis of dengue virus infection. Cell Signal 48, 64–68. doi:10.1016/j.cellsig.2018.05.002
Varghese, F. S., Kaukinen, P., Gläsker, S., Bespalov, M., Hanski, L., Wennerberg, K., et al. (2016a). Discovery of berberine, abamectin and ivermectin as antivirals against chikungunya and other alphaviruses. Antivir. Res. 126, 117–124. doi:10.1016/j.antiviral.2015.12.012
Varghese, F. S., Thaa, B., Amrun, S. N., Simarmata, D., Rausalu, K., Nyman, T. A., et al. (2016b). The antiviral alkaloid berberine reduces chikungunya virus-induced mitogen-activated protein kinase signaling. J. Virol. 90 (21), 9743–9757. doi:10.1128/jvi.01382-16
Varghese, F. S., van Woudenbergh, E., Overheul, G. J., Eleveld, M. J., Kurver, L., van Heerbeek, N., et al. (2021). Berberine and obatoclax inhibit SARS-cov-2 replication in primary human nasal epithelial cells in vitro. Viruses 13 (2), 282. doi:10.3390/v13020282
Wan, J. J., Brown, R. S., and Kielian, M. (2020). Berberine chloride is an alphavirus inhibitor that targets nucleocapsid assembly. mBio 11 (3), e01382–20. doi:10.1128/mbio.01382-20
Wang, H., Li, K., Ma, L., Wu, S., Hu, J., Yan, H., et al. (2017). Berberine inhibits enterovirus 71 replication by downregulating the MEK/ERK signaling pathway and autophagy. Virol. J. 14 (1), 2. doi:10.1186/s12985-016-0674-4
Wang, Y. X., Yang, L., Wang, H. Q., Zhao, X. Q., Liu, T., Li, Y. H., et al. (2018). Synthesis and evolution of berberine derivatives as a new class of antiviral agents against enterovirus 71 through the MEK/ERK pathway and autophagy. Molecules 23 (8), 2084. doi:10.3390/molecules23082084
Wang, Z. Z., Li, K., Maskey, A. R., Huang, W., Toutov, A. A., Yang, N., et al. (2021). A small molecule compound berberine as an orally active therapeutic candidate against COVID-19 and sars: a computational and mechanistic study. FASEB J. 35 (4), e21360. doi:10.1096/fj.202001792r
Wu, Y., Li, J. Q., Kim, Y. J., Wu, J., Wang, Q., and Hao, Y. (2011). In vivo and in vitro antiviral effects of berberine on influenza virus. Chin. J. Integr. Med. 17 (6), 444–452. doi:10.1007/s11655-011-0640-3
Yan, Y. Q., Fu, Y. J., Wu, S., Qin, H. Q., Zhen, X., Song, B. M., et al. (2018). Anti-influenza activity of berberine improves prognosis by reducing viral replication in mice. Phytother. Res. 32 (12), 2560–2567. doi:10.1002/ptr.6196
Ye, H., Kang, L., Yan, X., Li, S., Huang, Y., Mu, R., et al. (2022). MiR-103a-3p promotes zika virus replication by targeting OTU deubiquitinase 4 to activate p38 mitogen-activated protein kinase signaling pathway. Front. Microbiol. 13, 862580. doi:10.3389/fmicb.2022.862580
Zeng, Q. X., Wang, H. Q., Wei, W., Guo, T. T., Yu, L., Wang, Y. X., et al. (2020). Synthesis and biological evaluation of berberine derivatives as a new class of broad-spectrum antiviral agents against coxsackievirus B. Bioorg Chem. 95, 103490. doi:10.1016/j.bioorg.2019.103490
Keywords: flavivirus, zika, dengue, Berberine, antiviral
Citation: Giannone DA, Piccini LE, Brunetti JE, Quintana VM, Damonte EB and Castilla V (2023) Berberine inhibitory action against zika and dengue viruses in cell cultures. Acta Virol. 67:11931. doi: 10.3389/av.2023.11931
Received: 24 August 2022; Accepted: 07 July 2023;
Published: 01 September 2023.
Edited by:
Katarina Polcicova, Slovak Academy of Sciences, SlovakiaCopyright © 2023 Giannone, Piccini, Brunetti, Quintana, Damonte and Castilla. This is an open-access article distributed under the terms of the Creative Commons Attribution License (CC BY). The use, distribution or reproduction in other forums is permitted, provided the original author(s) and the copyright owner(s) are credited and that the original publication in this journal is cited, in accordance with accepted academic practice. No use, distribution or reproduction is permitted which does not comply with these terms.
*Correspondence: Viviana Castilla, viviana@qb.fcen.uba.ar
†Present address: Denise A. Giannone, Instituto de Investigaciones Biomédicas en Retrovirus y SIDA (INBIRS), UBA-CONICET, Buenos Aires, Argentina; Luana E. Piccini, Servicio Nacional de Sanidad y Calidad Agroalimentaria (SENASA), Buenos Aires, Argentina; Jesús E. Brunetti, Virus Immunology Group, Bernhard Nocht Institute for Tropical Medicine, Hamburg, Germany; Verónica M. Quintana, Instituto de Investigaciones Biotecnológicas, Universidad Nacional de San Martín, Buenos Aires, Argentina
‡These authors have contributed equally to this work