- 1Department of Physiology, Louisiana State University Health Sciences Center, New Orleans, LA, United States
- 2Neuroscience Center of Excellence, Louisiana State University Health Sciences Center, New Orleans, LA, United States
- 3Alcohol and Drug Abuse Center of Excellence, Louisiana State University Health Sciences Center, New Orleans, LA, United States
- 4Southeast Louisiana VA Healthcare System, New Orleans, LA, United States
Traumatic stress disorders are defined in part by persistent avoidance of trauma-related contexts. Our lab uses a preclinical model of traumatic stress using predator odor (i.e., bobcat urine) in which some but not all rats exhibit persistent avoidance of odor-paired stimuli, similar to what is seen in humans. Bobcat urine exposure increases alcohol consumption in male Avoider rats, but it has not been tested for its effects on intake of other drugs. Here, we tested the effect of bobcat urine exposure on cocaine self-administration in adult male and female Wistar rats. We did not observe any effect of bobcat urine exposure on cocaine self-administration in male or female rats. We observed that (1) female rats with long access (6 h) to cocaine self-administer more cocaine than long-access males, (2) long-access males and females exhibit escalation of cocaine intake over time, (3) stressed rats gain less weight than unstressed rats following acute predator odor exposure, (4) baseline cocaine self-administration is predictive of subsequent cocaine self-administration. The results of this study may inform future work on predator odor effects on cocaine self-administration.
Introduction
Traumatic stress disorders have a lifetime prevalence around 8% in the general population (1) with women being twice as likely as men to develop PTSD after experiencing a traumatic event (2-4). Traumatic stress disorder symptoms are classified in four clusters that include negative affect, intrusive thoughts and memories of the traumatic event, hyperarousal, and avoidance of contexts and stimuli associated with the trauma (5). Patients with traumatic stress disorders are at higher risk for developing comorbid conditions such as substance use disorders (SUDs). Patients with SUDs, particularly cocaine use disorder (CUD), present with more severe traumatic stress disorder symptoms and respond more poorly to treatment (6). The rate of traumatic stress disorders among cocaine users is approximately twice that of the general population (7–9). In patients with comorbid traumatic stress disorder and CUD, cocaine craving severity is correlated with traumatic stress disorder symptom severity (10, 11). Currently, the specific mechanisms underlying the high prevalence of comorbid traumatic stress disorders and CUD are unclear.
Cocaine has high abuse potential. Over 1.3 million people meet criteria for CUD and over 19,000 people died from cocaine-related overdoses in 2020 (12). Women exhibit higher rates of cocaine dependence (13). Although men use more cocaine than women, women experience a phenomenon in drug use known as “telescoping” in which women progress from casual use to problematic use and dependence at a faster rate than men (14, 15). In rat models of cocaine self-administration, females display more cocaine self-administration and drug seeking than males during acquisition, escalation, and reinstatement (16). Long-access (LgA) cocaine self-administration (6–12 h/day sessions) but not short-access (ShA) (1–3 h/day sessions) produces escalation of cocaine intake (17).
Patients with a history of cocaine misuse are more likely to relapse following periods of stress, and stress potentiates cue-induced cravings in cocaine-experienced people (18). However, stress exposure does not predict the severity of cocaine cravings (19). In cocaine-experienced rats, stressors (i.e., footshock, restraint, yohimbine) are highly effective at promoting reinstatement of cocaine seeking after extinction (20–25), and there are sex differences in this phenomenon. For example, female rats are more susceptible to cocaine reinstatement after yohimbine administration than males (26); and relative to male rats, female rats exhibit greater reinstatement of cocaine seeking induced by the combination of a priming dose of cocaine and restraint stress (27).
History of cocaine use influences subsequent stress effects on cocaine seeking in rats. For example, male rats with a history of LgA self-administration (6–12 h/day) exhibit footshock-induced reinstatement to cocaine seeking, whereas rats with ShA self-administration (1–2 h/day) do not (28). Similarly, social defeat causes increases in cocaine self-administration and cocaine “binge” intake in rats (29, 30). Additionally, acute- and intermittent-socially defeated male rats more readily acquire cocaine self-administration and self-administer more cocaine than non-defeated rats (31–33). Interestingly, social defeat-stressed females exhibit enhanced locomotor sensitization to cocaine relative to stressed males (34).
Previous work examining predator odor stress on cocaine self-administering rats has shown that male rats that avoid Trimethylthiazoline (TMT; fox feces extract) exhibit greater sensitization to the locomotor-stimulating effects of cocaine and greater motivation to consume cocaine than non-susceptible and control rats (35). Another research group showed that adult male Sprague Dawley rats exposed to TMT one time exhibit increases in cocaine self-administration over time during ShA (1 h/day) and LgA (6 h/day) cocaine sessions (36). Prior work examining the effects of stressful odors (e.g., feline urine, predator feces, TMT isolate) or other stressors (i.e., footshock, social defeat, restraint stress) on cocaine self-administration has been performed almost exclusively in male subjects—here, we tested predator stress effects on escalation of cocaine self-administration in male and female adult Wistar rats with long and short access to cocaine.
To do this, we used a model of traumatic stress in which rats are indexed for avoidance of a predator odor (i.e., bobcat urine)-paired context (37, 38). In humans, avoidance severity correlates with likelihood of diagnosis with a traumatic stress disorder, is associated with greater psychological distress after trauma, and is predictive of traumatic stress disorder symptom duration (39–41). Furthermore, avoidance-related symptoms predict drug craving in stimulant users, suggesting that individuals with high levels of avoidance may self-medicate with psychostimulants (42). To this point, our lab has primarily used this model to examine stress effects on alcohol self-administration in animals that do or do not exhibit persistent avoidance of predator odor-paired stimuli. In those studies, we have repeatedly observed that bobcat urine exposure produces reliable and lasting increases in alcohol self-administration in adult male Wistar rats (37, 43). Prior work from our group also shows that this procedure produces reliable increases in anxiety-like behavior (44) and HPA axis activation (44) in rats. Here, using the same model, we tested predator odor stress effects on cocaine self-administration in male and female adult Wistar rats. We hypothesized that predator odor exposure would lead to higher long-access cocaine self-administration in Avoider rats, and that this effect would be greater in females than in males.
Methods
Subjects
Male and female adult Wistar rats (n = 64 per sex) were obtained from Charles River Laboratories aged 8 weeks old (Raleigh, NC, USA) and were single-housed in a 12-hr reverse light cycle humidity- and temperature-controlled (22°C) colony room. Rats were allowed to acclimate to the colony room for 4 days prior to the start of surgeries. Animals were handled daily following surgery. Testing occurred during the dark period (between 8:00 am and 8:00 pm). All animals were provided with additional enrichment (Nestlet, BedderNest, and Nylabone) in their home cages throughout the study. Food was available ad libitum except during the first 4 days of the experiment while lever training. Water was always available. All procedures were approved by the Institutional Animal Care and Use Committee of the Louisiana State University Health Sciences Center and were in accordance with the National Institute of Health guidelines. For final analyses, 39 total rats were excluded due to either loss of catheter patency, post-surgical complications, or outlier behavioral responses.
Jugular catheter implantation surgery
Rats were anesthetized with 4% isoflurane gas and maintained on 2.5% isoflurane gas throughout the surgery. They received an implanted chronic indwelling catheter into the right jugular vein using catheters made in house. The catheters were inserted under the skin directly posterior to the shoulder blades and the Silastic tubing was threaded over the right shoulder and inserted into the right jugular vein. Catheter cannulas were covered with a small piece of clean plastic tubing and a metal screw top to prevent debris from entering the catheter. Rats were allowed to recover from surgery for 1 h on a heating pad before being returned to a clean home cage. 3 mL of sterile saline was administered subcutaneously to aid in recovery time. Flunixin meglumine (Covetrus; Portland, Maine, USA) was administered at the start of the surgery subcutaneously (2.5 mg/kg). After the sterilized (Maxicide supplied by Henry Schein; Mandeville, LA, USA) catheter was set in place, the catheter was flushed with 0.2 mL of cefazolin/heparin (1,000 USP units per mL) solution to prevent infections and catheter clogging. Heparin was supplied by Sagent Pharmaceuticals; Schaumburg, IL, USA, and cefazolin was supplied by WG Critical Care LLC.; Paramus, New Jersey, USA. Post-operative care included weighing and visually inspecting animals daily after surgery and a catheter flush with 0.2 mL cefazolin/heparin solution once daily until the end of the experiment. Rats were allowed to recover from surgery for at least 5 days before starting experiments.
Drugs
Cocaine (-) HCl was generously provided by the National Institute for Drug Abuse (NIDA) Drug Supply Program and Research Triangle Institute (RTI). Cocaine was dissolved in sterile saline and delivered at a dose and volume of 0.5 mg/0.1 mL/infusion. Rats were weighed daily before the start of each session and unit cocaine dose for each rat was calculated based on body weight.
Drug acquisition
To establish a baseline of cocaine intake per animal and facilitate lever-response training, rats were food restricted (20 g/day for males and 15 g/day for females) 1 day prior to the start of acquisition sessions and during the first 3 days of acquisition. Rats were placed into sound- and light-attenuated operant-conditioning chambers (30 cm × 20 cm × 24 cm; Med Associates Inc., St. Albans, VT) for 2 h/day for 10 consecutive days beginning at the same time each day. Rats began sessions 1–3 h into the dark cycle every day for 7 days/week. Each chamber contained two retractable levers, a stimulus light above each lever, a food trough between the levers, a fan, and a speaker connected to a tone generator (ANL-926, Med Associates). A single lever press on the active lever (right lever) resulted in the delivery of a single cocaine infusion (FR1; 0.5 mg/kg/0.1 mL) along with a 20 s presentation of 1) light above the active lever, 2) tone cue, and 3) 20 s timeout period during which time no subsequent cocaine could occur. Presses of the left lever had no consequence but were recorded. To meet criteria for progression to the next phase of the study, rats were required to press the active lever at least 10 times during a 2-hr session for the final three consecutive days of acquisition (days 8–10)—most animals achieved this during the 10-day baseline period.
Stress exposure and conditioned place aversion
One day after the final day of the acquisition/baseline period, predator odor place conditioning began. No cocaine was given during this part of the experiment. All rats completed conditioned place aversion (CPA) procedure as previously described (38, 45) using a 3-chamber apparatus. Briefly, on day 1 of this procedure, each rat is given 5 minutes to freely explore the three connected chambers in the apparatus (3-chamber pre-test), each with unique visual cues on the walls and tactile cues on the floor. To maintain an unbiased design, the chamber each rat preferred most or least relative to the other two was excluded for the remainder of the experiment. On day 2, each rat explored the two chambers it spent the most similar amounts of time in during day 1 for 5 minutes (2-chamber pre-test). Rats were then separated into predator odor-exposed or unstressed control groups. Again, to maintain an unbiased design, the chamber in which odor exposure would occur was assigned in a counterbalanced fashion based on chamber preference. On day 3 of the procedure, rats were confined to the “no odor” chamber in the absence of any odor for 15 min. On day 4 of the procedure, rats were confined to the “odor” chamber for 15 min, during which time rats in the odor group were exposed to bobcat urine odor and rats in the control group were exposed to no odor (same as day 3). On day 5 of the procedure, rats were once again allowed to freely move about the two chambers (2-chamber post-test). Rats were classified as “Avoiders,” or “Non-avoiders” based on the differences in time spent in the “odor” chamber between the 2-chamber pre-test and post-test. Rats with greater than a 10 s decrease in time spent in the “odor” chamber from pre-test to post-test were categorized as “Avoiders,” and all other odor-exposed animals were categorized as “Non-avoiders.” Unstressed controls were never exposed to bobcat urine.
Post-stress cocaine self-administration
Rats were assigned to ShA or LgA self-administration groups based on their avoidance score in a counterbalanced fashion, such that unstressed controls, Non-avoiders, and Avoiders were equally represented in the two cocaine access groups. One day following the 2-chamber post-test of the place conditioning procedure, rats were once again allowed to self-administer cocaine (0.5 mg/0.1 mL/infusion) on an FR1 schedule of reinforcement for either 1 h/day (ShA) or 6 h/day (LgA) for 10 consecutive days in the same operant chambers used for acquisition and baseline. Specific operant boxes were used for males only, and others were used for females only during the entirety of the experiment. At the end of the study, 1 day after the 10th day of self-administration, rats were allowed to self-administer cocaine for 1 h and then anesthetized with 4% isoflurane gas, decapitated, and the brains were flash frozen in 2-methylbutane for future analysis.
Catheter patency
Catheter patency was checked once per week by flushing catheters with 0.2 mL Brevitol Sodium (distributed by Henry Schein; Mandeville, LA, USA) and observing body response. Rats that failed to go limp after 3 s were excluded from the study.
Statistical analysis
All data was analyzed using Prism Graph Pad version 9.0 (GraphPad Software, Inc., La Jolla, CA, USA). Data were analyzed using ANOVAs with stress groups, sex, and self-administration access groups as between-subjects factors and session days as a within-subjects factor when appropriate. Significant effects were followed up with Tukey’s or Bonferonni post hoc tests where appropriate (Bonferonni post hoc tests were used for all repeated measures ANOVAs). Outliers were detected using the interquartile range (IQR) rule, and data analyzed after outliers were detected were analyzed using Mann-Whitney test. Correlations were performed using Pearson correlations. Statistical significance was set at p < 0.05.
Results
Avoidance of predator odor context is not associated with cocaine self-administration
Adult male and female Wistar rats were exposed to bobcat urine and indexed for avoidance behavior of the odor-paired chamber (see Figure 1), and 62% of males and 46% of females were categorized as Avoiders. A Pearson correlation revealed no association between avoidance and cocaine deliveries in ShA stressed males (r = 0.30; p = 0.37), LgA stressed males (r = 0.25; p = 0.41), ShA stressed females (r = −0.05; p = 0.89), or LgA stressed females (r = −0.07; p = 0.83). We analyzed the effects of avoidance phenotype and time on cocaine deliveries using separate two-way repeated measures (RM) ANOVAs for LgA males, LgA females, ShA males, and ShA females. There was no effect of avoidance phenotype on post-stress cocaine deliveries in any of these groups (p > 0.05 in all cases). Because we did not find differences in cocaine intake between Avoiders and Non-avoiders, we collapsed Avoiders and Non-avoiders into a single group of “Stressed” rats for each sex, and we compared stressed rats to unstressed controls in all subsequent analyses.
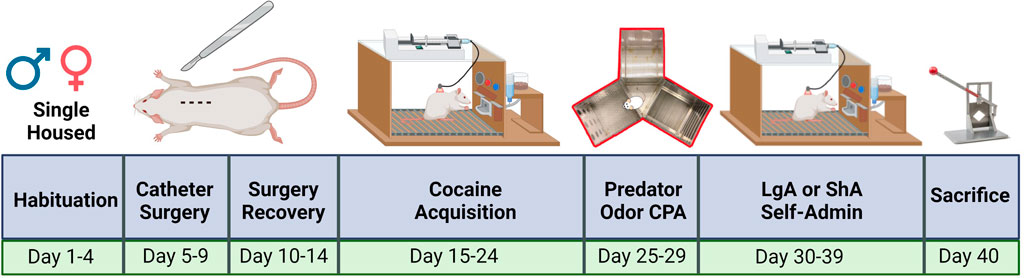
FIGURE 1. Experiment timeline. Each cohort was treated identically upon arrival to the LSUHSC animal facility. The experiment lasted 26 days from the start of cocaine exposure to sacrifice. All rats acquired cocaine operant conditioning. Then rats underwent place aversion conditioning with bobcat urine. Rats in the control group never experienced bobcat urine. Avoidance was measured and subjects were divided into ShA or LgA groups. The ShA and LgA groups completed 10 days of post-CPA self-administration. created with biorender.com
Predator odor exposure does not alter cocaine self-administration
A 2-way RM ANOVA showed that LgA males escalated cocaine drug deliveries regardless of stress history (F [2.41, 55.64] = 6.29; p < 0.01), but there was no effect of stress (F [1.23] = 0.02; p = 0.88) (Figure 2A). Neither stress (F [1.20] = 2.11; p = 0.16) nor day (F [1.93, 38.53] = 1.92; p = 0.16) had effects on ShA males’ cocaine deliveries (Figure 2A). Figure 2B highlights that LgA males exhibit greater increases in cocaine deliveries over time relative to ShA males (F [1.43] = 27.94; p < 0.0001) in the absence of stress effects (F [1.43] = 1.90; p = 0.18).
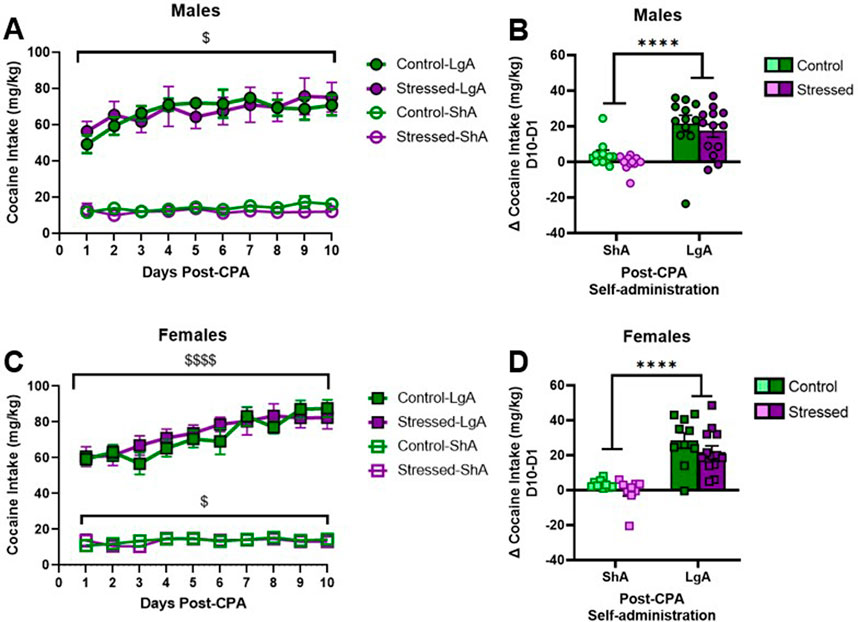
FIGURE 2. Long-access males and females escalate self-administration; no effect of stress. (A,B) LgA males escalated cocaine intake (p = 0.01), but ShA males did not change intake over time. (C,D) LgA and ShA females escalated cocaine intake. Data presented as mean ± SEM. Dollar signs denote a main effect of time ($ denotes p < 0.05 and denotes p < 0.0001). Asterisks denote a main effect of access condition (**** denotes p < 0.0001).
A separate 2-way RM ANOVA showed that LgA females escalated cocaine deliveries regardless of stress history (F [2.15, 45.22] = 26.18; p < 0.0001; Figure 2C), and again there was no effect of stress (F [1.21] = 0.21; p = 0.65). A separate 2-way RM ANOVA in ShA females revealed a main effect of day (F [2.48, 42.13] = 3.29; p = 0.04) but not of stress (F [1.17] = 0.05; p = 0.83) (Figure 2C). Figure 2D highlights LgA females exhibit greater increases in cocaine intake over time relative to ShA females (F [1.38] = 54.74; p < 0.0001) in the absence of stress effects (F [1.38] = 2.738; p = 0.11).
Males and females escalate cocaine deliveries
In separate analyses, we compared self-administration across sexes in animals with the same access conditions (LgA or ShA) collapsed across stress history. In a 2-way RM ANOVA analysis of cocaine delivery data in LgA animals, we observed that all rats escalate cocaine deliveries over 10 days (F [2.50, 115.0] = 22.93; p < 0.0001; Figure 3A). There was no main effect of sex (F [1.46] = 3.35; p = 0.07; Figure 3A). ShA males and females did not differ in intake (F [1.39] = 0.23; p = 0.64), but there was a main effect of day (F [2.46, 95.82] = 3.81; p = 0.02) on ShA cocaine delivery showing all rats escalated cocaine deliveries over time. A 2-way ANOVA on cocaine deliveries in LgA rats showed LgA males and females take more cocaine during the second half of post-CPA self-administration (days 6–10) than during days 1–5 (D1-5) of post-CPA self-administration (main effect of access days: F [1.46] = 21.52; p < 0.0001; Figure 3B). In LgA rats, females take more cocaine than males during self-administration days 6–10 (D6-10) (days x sex interaction: F [1.46] = 2.750; p < 0.01; Figure 3B).
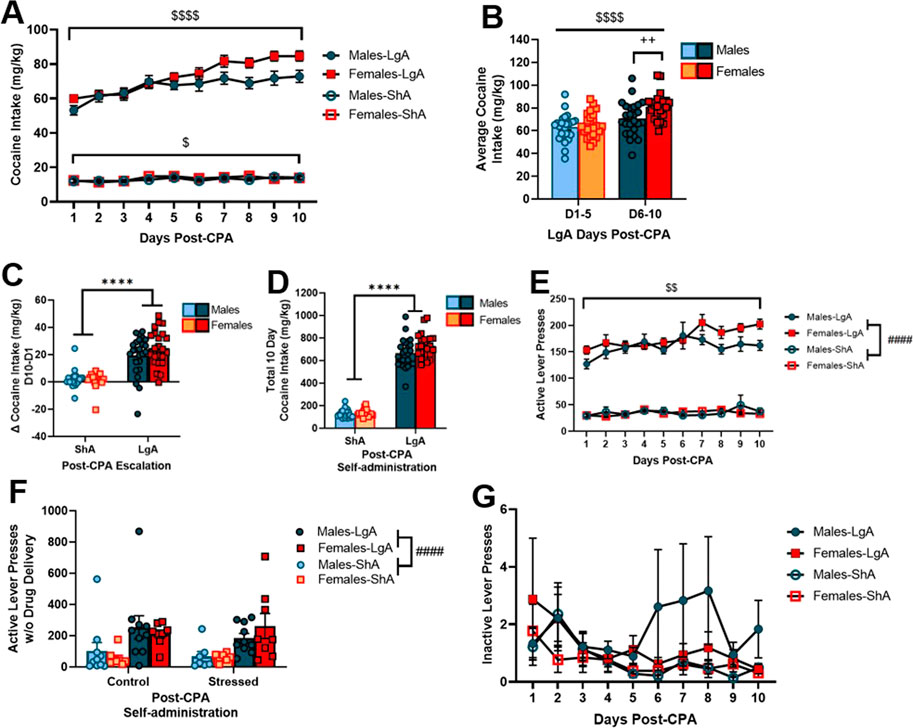
FIGURE 3. LgA females self-administer more cocaine than LgA males on days 6–10. (A) All LgA males and females (combined control and stressed groups) escalated cocaine intake over 10 days post-CPA self-administration. (B) LgA males and females received more cocaine deliveries during the second half of post-CPA self-administration, and females received more cocaine deliveries than males on days 6–10. (C) LgA males and females escalated cocaine intake, but ShA rats maintained similar intake levels throughout the 10-day testing period. LgA males and females escalated intake similar amounts across all 10 days. (D) All LgA rats received more cocaine deliveries than ShA rats. (E) LgA males and females increased total active lever presses (with and without drug delivery) over time. (F) LgA males and females pressed the active lever more during 20 s time-outs than ShA males and females; there was no effect of stress. (G) There was no difference between how many inactive lever presses males and females made during post-CPA self-administration sessions. Data presented as mean ± SEM. Asterisks denote a main effect of access condition (**** denotes p < 0.0001). Dollar signs denote a main effect of day ($$ denotes p < 0.01; denotes p < 0.0001). Pound signs denote a main effect of sex (#### denotes p < 0.0001). Plus signs denote an interaction (++ denotes p < 0.01).
A follow-up 2-way ANOVA highlights that LgA rats display greater escalation of cocaine deliveries than ShA rats (F [1.85] = 76.46; p < 0.0001; Figure 3C). LgA males and females escalated drug deliveries similar amounts across all 10 days post-CPA self-administration (F [1.85] = 1.06; p = 0.31). When comparing total cocaine deliveries, we found all LgA rats received more cocaine deliveries than ShA rats (F [1.85] = 876.9; p < 0.0001; Figure 3D). There was not a significant difference between sexes in total deliveries in 10 days, but there was a trend that showed females self-administered more than males (F [1.85] = 3.53; p = 0.06; Figure 3D). Figure 3E illustrates LgA males and females increase total active lever presses (with and without drug delivery) over time (F [3.20, 107.5] = 4.21; p < 0.01) with no sex differences (F [1.34] = 2.96; p = 0.09). There was no change in active lever presses across days (F [1.81, 51.56] = 1.07; p = 0.34) nor sex (F [1.30] = 0.04; p = 0.84) in ShA rats (Figure 3E). A 3-way ANOVA on total active lever presses during the time-out period, when no cocaine was available, revealed that LgA rats press the active lever more during 20 s timeouts than ShA rats (F [1.60] = 18.70; p < 0.001; Figure 3F); there was no effect of stress (F [1.60] = 0.11; p = 0.74) nor sex (F [1.60] = 0.013; p = 0.91) on responding during time-out periods.
Figure 3G shows inactive lever presses (lever presses resulting in no reinforcer delivery) for all LgA and ShA males and females during post-CPA sessions. We used a 2-way RM ANOVAs to analyze sex and time effects on inactive lever presses in LgA and ShA animals. After removing outliers (1 male) identified using the IQR test, a Mann-Whitney test revealed that there were no differences in inactive lever pressing during post-CPA self-administration between any group. There was no time x sex interactions between LgA males and LgA females (F [9, 288] = 0.82; p = 0.60), and no time x sex interactions between ShA males and females F [9, 225] = 0.87; p = 0.56). There was no difference in inactive lever presses between ShA and LgA animals (F [27, 513] = 0.65; p = 0.91). Outlier data appeared to be associated with traditional stimulant-induced stereotypy behavior.
Baseline cocaine intake correlates with post-CPA cocaine self-administration
Collapsing across stress groups, in the ShA male, ShA female, and LgA female groups, baseline cocaine intake (average cocaine intake [mg/kg] during acquisition days 8, 9, and 10) was positively correlated with post-CPA cocaine intake (ShA males [r = 0.66; p < 0.001; Figure 4A]; ShA females [r = 0.57; p = 0.01; Figure 4C]; LgA females [r = 0.50; p = 0.01; Figure 4D]). LgA males exhibited a trend toward the same correlation (r = 0.40; p = 0.058; Figure 4B).
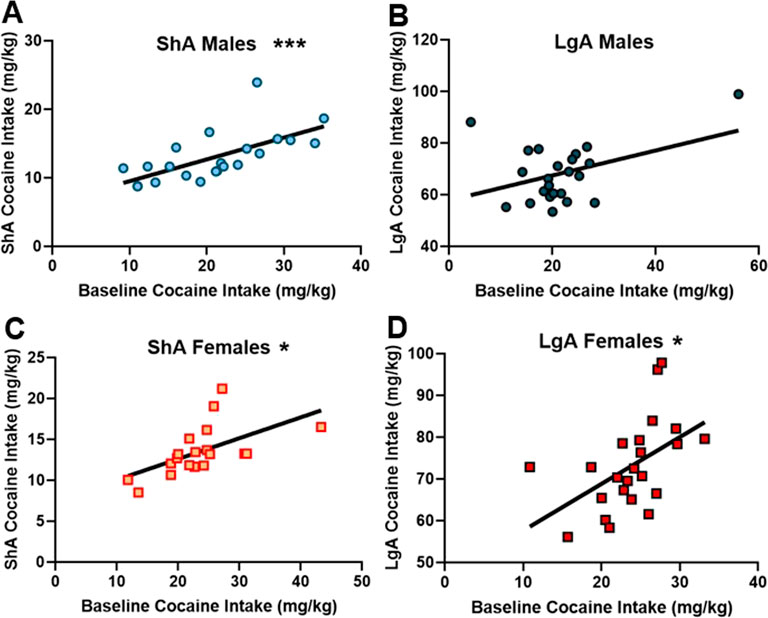
FIGURE 4. Baseline cocaine intake predicts future cocaine intake. (A–D) Male and female ShA and LgA rats that were low responders during acquisition continued to be low responders and high responders continued to be high responders after the conditioned place aversion test regardless of stress exposure group and access group. *denotes p < 0.05; *** denotes p < 0.001.
Weight gain during self-administration and stress exposure
Rats were weighed daily throughout the experiment. We analyzed percent change in body weight during the pre-CPA cocaine acquisition period (2 h/day cocaine access), and during the post-CPA LgA (6hrs/day cocaine access) and ShA (1 h/day cocaine access) period. Males and females increase body weight similarly during acquisition (unpaired t-test: p = 0.59; Figure 5A). During post-stress self-administration, all (control and stressed combined) LgA males gained less weight than all ShA males (F [1.85] = 7.04; p < 0.01); and there was a sex x access condition interaction effect on body weight gain (F [1.85) = 8.38; p < 0.01; Figure 5B).
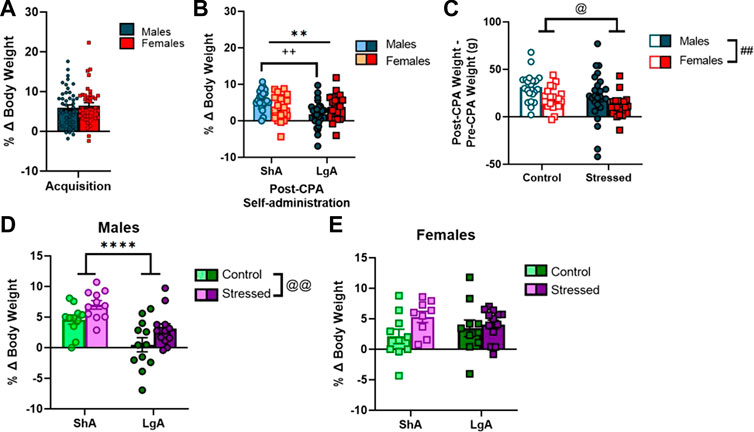
FIGURE 5. Weight gain during acquisition, CPA, and post-CPA ShA and LgA. (A) Males and females increased body weight similarly during acquisition. (B) LgA males gained less weight than ShA males while females were unaffected. (C) Stressed males and females gained less weight than controls following acute predator odor exposure across the 5-day CPA procedure. (D) LgA males gained less weight than ShA males, and stressed males gained more weight than control males after predator odor exposure during post-CPA 10-day self-administration. (E) There was no difference in post-CPA weight gain between LgA and ShA females nor control and stressed females during 10-days post-CPA self-administration. Data presented as mean ± SEM. Asterisks denote a main effect of access condition (** denotes p < 0.01; **** denotes p < 0.0001). Pound signs denote a main effect of sex (## denotes p < 0.01). @ symbols denote a main effect of stress (@ denotes p < 0.05; @@ denotes p < 0.01). Plus signs denote an interaction (++ denotes p < 0.01).
Next, we collapsed data across LgA and ShA conditions to examine acute effects of predator odor exposure (i.e., during the 5-day conditioning procedure) on body weight gain in male and female rats. When comparing body weight (g) 48 h after the CPA test (i.e., day 1 of post-CPA self-administration prior to the start of the operant session) to body weight prior to day 1 of the conditioning procedure, we observed that stressed males and females gain less weight than controls (F [1.85] = 5.59; p = 0.02; Figure 5C), and females gained less weight overall than males (F [1.85] = 8.16; p < 0.01; Figure 5C) during the 5 days of the conditioning procedure, presumably due to acute stress effects of the predator odor exposure.
Finally, we used a 2-way ANOVA to analyze percent body weight gain in male rats during the 10 days of post-CPA self-administration. LgA males gain less weight than ShA males (F [1.43] = 20.85; p < 0.0001; Figure 5D), and stressed males gain more weight than control males during the 10 days of post-CPA self-administration (F [1.43] = 8.41; p < 0.01; Figure 5D). A separate 2-way ANOVA of percent body weight gain in female rats during the 10 days of post-CPA self-administration revealed there was no difference in post-CPA body weight gain between LgA and ShA females (F [1.38] = 0.002; p = 0.97), nor was there a stress effect (F [1.38] = 3.28; p = 0.08; Figure 5E) on body weight gain in females during the 10-day post-CPA self-administration period.
Discussion
The main results of this experiment demonstrate that 1) acute predator odor stress does not affect ShA or LgA cocaine self-administration in adult male and female Wistar rats; 2) male and female rats exhibit greatest escalation of cocaine intake under long-access conditions; 3) females self-administer more cocaine than males; and 4) early cocaine intake during 2-hr acquisition periods is positively associated with subsequent levels of cocaine intake in males and females under LgA and ShA conditions. We also report that 1) stressed rats gain less weight in the days immediately following bobcat urine exposure than unstressed controls; 2) LgA males gain less weight than ShA males, and surprisingly, 3) stressed males (regardless of access condition) gain more weight than unstressed control males during 10 days of post-CPA self-administration. Interestingly, cocaine access condition and stress exposure did not affect body weight gain in females.
In this study, females acquired cocaine at a similar rate to males; then they self-administered more under long-access conditions, but this was only seen in the later session (LgA D6-10). We originally hypothesized that Avoider rats would take more cocaine than Non-avoiders and unstressed rats and that females would take more than males. Our data indicated that Avoiders did not differ in their cocaine intake from Non-avoiders nor unstressed rats. Furthermore, all stressed rats (Avoiders + Non-avoiders) did not self-administer more cocaine than unstressed rats. This differs from previous results from our lab in which bobcat urine exposure produces long lasting increases in alcohol self-administration in Avoiders (37, 43) and blunts alcohol aversion (46). It is possible that a single odor exposure was not sufficient to change cocaine self-administration due to the strong reinforcing properties of cocaine.
Prior work from another research group also reported that a one-time exposure to predator odor (TMT in this case) failed to alter LgA cocaine self-administration (FR1; 0.33 mg/infusion) (36). That work differed from ours in that their animals were exposed to predator odor without prior cocaine experience, subjects were Sprague Dawley rats, and LgA sessions began 23 days after a 10 min TMT exposure. That prior study reported that TMT-stress resilient rats, identified according to anxiety-like behavior and startle reactivity, self-administered fewer cocaine infusions during ShA sessions compared to controls and TMT-stress susceptible rats. Comparing between experiments, Sprague-Dawley rats in the (36) study responded less for cocaine and received fewer cocaine infusions during ShA (1 h/day) and LgA (6 h/day) sessions than Wistar rats in the current study (2-to-4 fold higher responding in the current study). These differences may be attributable to strain and/or procedural differences between the studies (timing of self-administration acquisition, type of odor, etc.). Future work may benefit from utilizing repeated stress exposures and/or fewer baseline acquisition sessions prior to odor exposure.
Bobcat urine is an innately stressful stimulus that triggers the release of pro-stress hormones (e.g., corticosterone) and increases anxiety-like behaviors in rats (44, 47–50). A subset of rats exposed to bobcat urine exhibit long-lasting and extinction-resistant avoidance of stimuli paired with that odor (37). Other work reported that cat urine-exposed rats exhibit less habituation in the acoustic startle response than unstressed controls (51). Interestingly, female rats exposed to bobcat urine exhibit lower acoustic startle reactivity relative to unstressed control females, unlike what is seen in males, highlighting sex differences in the behavioral response to bobcat urine exposure in male and female rats (49).
Previous work in our lab reported that bobcat urine exposure reduces body weight gain in Avoiders and Non-avoiders relative to unstressed controls (43, 49). Here, we confirmed those findings such that stressed animals gained less weight during the week in which bobcat urine exposure and place conditioning occurred. Interestingly, however, predator odor-exposed males subsequently gained more weight than unstressed controls during the following 10 days of cocaine self-administration, regardless of access condition. Cocaine is frequently used as a weight control strategy, more so in women than in men (52, 53). One study reported that cocaine-dependent men have lower body mass index than non-dependent men, but report more compulsive eating and consume more calorie-dense food indicating cocaine’s weight loss effects may be due to changes in nutrient metabolism rather than changes in feeding behavior (54).
Preclinical work has reported that cocaine does not change long-term food intake in rats, but rather cocaine has acute anorexigenic effects (55). It has been suggested that increases in mesolimbic dopamine may suppress food consumption, similar to what is seen following administration of the dopamine type-2 receptor agonist, bromocriptine (56). However, following acute cocaine-induced appetite suppression, rats display increased motivation for high-calorie food which may lead to “binge eating” in cocaine-experienced rats (57). Clinical and preclinical work suggests that subjects with a history of cocaine use consume more calories and more high-fat food, but they do not gain more weight than non-cocaine users (54, 58). It has been speculated that these effects may be due to cocaine stimulation of cocaine and amphetamine-regulated transcript (CART) release and activation of CART-expressing nucleus accumbens neurons, as well as subsequent reductions in leptin production (59–61). Furthermore, cocaine’s ability to antagonize the serotonin transporter and norepinephrine transporter (resulting in a decrease in pre-synaptic serotonin and norepinephrine reuptake) likely result in increased appetite, decreased leptin production, and increased thermogenesis (62, 63). Here, we report that males with extended access (6 h/day) to cocaine exhibit attenuated weight gain across 10 days of long-access cocaine self-administration relative to males with shorter access (1 h/day) to cocaine. One limitation of this study is that we did not measure food intake over time.
Intravenous self-administration experiments report that female rats respond more for cocaine than males at all stages of access (i.e., acquisition, escalation) (16, 64). Female rodents also more readily reinstate drug use after a period of abstinence in the absence of any reinforcing cues when compared to males (65, 66). Here, in adult male and female Wistar rats, we confirmed prior work showing that LgA cocaine self-administration but not ShA produces escalation of cocaine intake (17). Women begin using cocaine earlier in life (67), develop CUD faster than men (13, 68, 69), experience more cocaine-related stress, paranoia, and hyperalgesia than men (70), and seek treatment for CUD more times in their lives than men (71). It is possible that different strategies will be effective at reducing cocaine use in men versus women. Furthermore, sex/gender differences in cocaine reward may depend on menstrual cycles and circulating/brain estradiol and progesterone in women (72–79). Unfortunately, we did not monitor the estrous cycles of females, which is a limitation of this study considering that female rodents self-administer more cocaine during estrus/proestrus than diestrus and when given estradiol (80, 81).
In conclusion, this study confirms various prior findings in the cocaine self-administration and predator odor stress fields. Prior findings showing that bobcat urine exposure produces escalation of alcohol self-administration did not generalize to cocaine self-administration in this study regardless of sex and cocaine access condition. Strategies for future work in this area may consider exposing animals to repeated stress, changing the timing of stress relative to cocaine self-administration, or exploring other predator odors.
Data availability statement
The raw data supporting the conclusion of this article will be made available by the authors, without undue reservation.
Ethics statement
The animal study was reviewed and approved by Institutional Animal Care and Use Committee of the Louisiana State University Health Sciences Center.
Author contributions
TT-J and NG conceived and planned the experiment. TT-J carried out the experiment, analyzed the data, and wrote the manuscript. NG supervised the project and revised and guided the manuscript. SD recorded, sorted, and entered the data. All authors contributed to the article and approved the submitted version.
Funding
This work was supported by NIH grants AA023305 (NG) and AA026531 (NG). This work was supported in part by a Merit Review Award #I01 BX003451 (NG) from the United States (US) Department of Veterans Affairs, Biomedical Laboratory Research and Development Service.
Conflict of interest
NG is a consultant for Glauser Life Sciences, Inc.
The remaining authors declare that the research was conducted in the absence of any commercial or financial relationships that could be construed as a potential conflict of interest.
Acknowledgments
We thank Dr. Mary Secci, Chuck Jager, and Kim Edwards for their technical assistance.
References
1.American Psychiatric Association. Diagnostic and statistical manual of mental disorders. 4th ed. (2000). p. DSM4. Available from: https://dsm.psychiatryonline.org/doi/epdf/10.1176/appi.books.9780890420249.dsm-iv-tr (Accessed February 1, 2023).
2. Kessler, RC, Sonnega, A, Bromet, E, Hughes, M, and Nelson, C. Posttraumatic stress disorder in the national comorbidity survey. Arch Gen Psychiatry (1995) 52(12):1048–60. doi:10.1001/archpsyc.1995.03950240066012
3. Christiansen, D, Elklit, A, Christiansen, D, and Elklit, A. Sex differences in PTSD. In: Post traumatic stress disorders in a global context. Rijeka, Croatia: IntechOpen (2012). doi:10.5772/28363
4. Blain, LM, Galovski, TE, and Robinson, T. Gender differences in recovery from posttraumatic stress disorder: A critical review. Aggression Violent Behav (2010) 15(6):463–74. doi:10.1016/j.avb.2010.09.001
5.American Psychiatric Association. Diagnostic and statistical manual of mental disorders. 5th ed. (2013). p. DSM5. Available from: https://dsm.psychiatryonline.org/doi/epdf/10.1176/appi.books.9780890425596 (Accessed February 1, 2023).
6. Najavits, LM, Harned, MS, Gallop, RJ, Butler, SF, Barber, JP, Thase, ME, et al. Six-month treatment outcomes of cocaine-dependent patients with and without PTSD in a multisite national trial. J Stud Alcohol Drugs (2007) 68(3):353–61. doi:10.15288/jsad.2007.68.353
7. Cottler, LB, Compton, WM, Mager, DM, Spitznagel, EL, and Janca, A. Posttraumatic stress disorder among substance users from the general population. Am J Psychiatry (1992) 149(5):664–70. doi:10.1176/ajp.149.5.664
8. Najavits, LM, Gastfriend, DR, Barber, JP, Reif, S, Muenz, LR, Blaine, J, et al. Cocaine dependence with and without PTSD among subjects in the national Institute on drug abuse collaborative cocaine treatment study. Am J Psychiatry (1998) 155(2):214–9. doi:10.1176/ajp.155.2.214
9. Back, S, Dansky, BS, Coffey, SF, Saladin, ME, Sonne, S, and Brady, KT. Cocaine dependence with and without post-traumatic stress disorder: A comparison of substance use, trauma history and psychiatric comorbidity. Am J Addict (2000) 9(1):51–62. doi:10.1080/10550490050172227
10. Coffey, SF, Saladin, ME, Drobes, DJ, Brady, KT, Dansky, BS, and Kilpatrick, DG. Trauma and substance cue reactivity in individuals with comorbid posttraumatic stress disorder and cocaine or alcohol dependence. Drug and Alcohol Dependence (2002) 65(2):115–27. doi:10.1016/S0376-8716(01)00157-0
11. Saladin, ME, Drobes, DJ, Coffey, SF, Dansky, BS, Brady, KT, and Kilpatrick, DG. PTSD symptom severity as a predictor of cue-elicited drug craving in victims of violent crime. Addict Behaviors (2003) 28(9):1611–29. doi:10.1016/j.addbeh.2003.08.037
12.Substance Abuse and Mental Health Services Administration. (2021). Key substance use and mental health indicators in the United States: Results from the 2020 national survey on drug use and health. (HHS Publication No. PEP21-07-01-003, NSDUH Series H-56). Rockville, MD: Center for Behavioral Health Statistics and Quality, Substance Abuse and Mental Health Services Administration. Available from: https://www.samhsa.gov/data/ (Accessed December 12, 2022).
13. Brady, K, Grice, D, Dustan, L, and Randall, C. Gender differences in substance use disorders. Am J Psychiatry (1993) 150(11):1707–11. doi:10.1176/ajp.150.11.1707
14. Brady, K, and Randall, C. Gender differences in substance use disorders. Psychiatr Clin North Am (1999) 22(2):241–52. doi:10.1016/S0193-953X(05)70074-5
15. Kosten, TR, Schottenfeld, R, Ziedonis, D, and Falcioni, J. Buprenorphine versus methadone maintenance for opioid dependence. J Nervous Ment Dis (1993) 181(6):358–64. doi:10.1097/00005053-199306000-00004
16. Roth, ME, and Carroll, ME. Sex differences in the escalation of intravenous cocaine intake following long- or short-access to cocaine self-administration. Pharmacol Biochem Behav (2004) 78(2):199–207. doi:10.1016/j.pbb.2004.03.018
17. Wee, S, Specio, SE, and Koob, GF. Effects of dose and session duration on cocaine self-administration in rats. J Pharmacol Exp Ther (2007) 320(3):1134–43. doi:10.1124/jpet.106.113340
18. Preston, KL, Kowalczyk, WJ, Phillips, KA, Jobes, ML, Vahabzadeh, M, Lin, J-L, et al. Exacerbated craving in the presence of stress and drug cues in drug-dependent patients. Neuropsychopharmacology (2018) 43(4):859–67. doi:10.1038/npp.2017.275
19. Preston, KL, and Epstein, DH. Stress in the daily lives of cocaine and heroin users: Relationship to mood, craving, relapse triggers, and cocaine use. Psychopharmacology (2011) 218(1):29–37. doi:10.1007/s00213-011-2183-x
20. Buffalari, DM, and See, RE. Footshock stress potentiates cue-induced cocaine-seeking in an animal model of relapse. Physiol Behav (2009) 98(5):614–7. doi:10.1016/j.physbeh.2009.09.013
21. Buffalari, DM, and See, RE. Inactivation of the bed nucleus of the stria terminalis in an animal model of relapse: Effects on conditioned cue-induced reinstatement and its enhancement by yohimbine. Psychopharmacology (2011) 213(1):19–27. doi:10.1007/s00213-010-2008-3
22. Graf, EN, Wheeler, RA, Baker, DA, Ebben, AL, Hill, JE, McReynolds, JR, et al. Corticosterone acts in the nucleus accumbens to enhance dopamine signaling and potentiate reinstatement of cocaine seeking. J Neurosci (2013) 33(29):11800–10. doi:10.1523/JNEUROSCI.1969-13.2013
23. McReynolds, JR, Doncheck, EM, Li, Y, Vranjkovic, O, Graf, EN, Ogasawara, D, et al. Stress promotes drug seeking through glucocorticoid-dependent endocannabinoid mobilization in the prelimbic cortex. Biol Psychiatry (2018) 84(2):85–94. doi:10.1016/j.biopsych.2017.09.024
24. Ahmed, SH, and Koob, GF. Cocaine- but not food-seeking behavior is reinstated by stress after extinction. Psychopharmacology (1997) 132(3):289–95. doi:10.1007/s002130050347
25. Erb, S, Shaham, Y, and Stewart, J. Stress reinstates cocaine-seeking behavior after prolonged extinction and a drug-free period. Psychopharmacology (1996) 128(4):408–12. doi:10.1007/s002130050150
26. Feltenstein, MW, Henderson, AR, and See, RE. Enhancement of cue-induced reinstatement of cocaine-seeking in rats by yohimbine: Sex differences and the role of the estrous cycle. Psychopharmacology (2011) 216(1):53–62. doi:10.1007/s00213-011-2187-6
27. Doncheck, EM, Liddiard, GT, Konrath, CD, Liu, X, Yu, L, Urbanik, LA, et al. Sex, stress, and prefrontal cortex: Influence of biological sex on stress-promoted cocaine seeking. Neuropsychopharmacology (2020) 45(12):1974–85. doi:10.1038/s41386-020-0674-3
28. Mantsch, JR, Baker, DA, Francis, DM, Katz, ES, Hoks, MA, and Serge, JP. Stressor- and corticotropin releasing factor-induced reinstatement and active stress-related behavioral responses are augmented following long-access cocaine self-administration by rats. Psychopharmacology (2007) 195(4):591–603. doi:10.1007/s00213-007-0950-5
29. Covington, HE, and Miczek, KA. Repeated social-defeat stress, cocaine or morphine. Effects on behavioral sensitization and intravenous cocaine self-administration “binges. Psychopharmacology (2001) 158(4):388–98. doi:10.1007/s002130100858
30. Quadros, IMH, and Miczek, KA. Two modes of intense cocaine bingeing: Increased persistence after social defeat stress and increased rate of intake due to extended access conditions in rats. Psychopharmacology (2009) 206(1):109–20. doi:10.1007/s00213-009-1584-6
31. Tidey, JW, and Miczek, KA. Acquisition of cocaine self-administration after social stress: Role of accumbens dopamine. Psychopharmacology (1997) 130(3):203–12. doi:10.1007/s002130050230
32. Yap, JJ, Chartoff, EH, Holly, EN, Potter, DN, Carlezon, WA, and Miczek, KA. Social defeat stress-induced sensitization and escalated cocaine self-administration: the role of ERK signaling in the rat ventral tegmental area. Psychopharmacology (Berl) (2015) 232(9):1555–69. doi:10.1007/s00213-014-3796-7
33. Covington, HE, Kikusui, T, Goodhue, J, Nikulina, EM, Hammer, RP, and Miczek, KA. Brief social defeat stress: Long lasting effects on cocaine taking during a binge and Zif268 mRNA expression in the amygdala and prefrontal cortex. Neuropsychopharmacology (2005) 30(2):310–21. Article 2. doi:10.1038/sj.npp.1300587
34. Holly, EN, Shimamoto, A, DeBold, JF, and Miczek, KA. Sex differences in behavioral and neural cross-sensitization and escalated cocaine taking as a result of episodic social defeat stress in rats. Psychopharmacology (2012) 224(1):179–88. doi:10.1007/s00213-012-2846-2
35. Brodnik, ZD, Black, EM, Clark, MJ, Kornsey, KN, Snyder, NW, and España, RA. Susceptibility to traumatic stress sensitizes the dopaminergic response to cocaine and increases motivation for cocaine. Neuropharmacology (2017) 125:295–307. doi:10.1016/j.neuropharm.2017.07.032
36. Schwendt, M, Shallcross, J, Hadad, NA, Namba, MD, Hiller, H, Wu, L, et al. A novel rat model of comorbid PTSD and addiction reveals intersections between stress susceptibility and enhanced cocaine seeking with a role for mGlu5 receptors. Translational Psychiatry (2018) 8:209. doi:10.1038/s41398-018-0265-9
37. Edwards, S, Baynes, BB, Carmichael, CY, Zamora-Martinez, ER, Barrus, M, Koob, GF, et al. Traumatic stress reactivity promotes excessive alcohol drinking and alters the balance of prefrontal cortex-amygdala activity. Translational Psychiatry (2013) 3(8):e296. doi:10.1038/tp.2013.70
38. Albrechet-Souza, L, and Gilpin, NW. The predator odor avoidance model of post-traumatic stress disorder in rats. Behav Pharmacol (2019) 30(2):105–14. doi:10.1097/FBP.0000000000000460
39. Hendrix, CC, Anelli, LM, Gibbs, JP, and Fournier, DG. Validation of the purdue post-traumatic stress scale on a sample of vietnam veterans. J Traumatic Stress (1994) 7(2):311–8. doi:10.1007/BF02102951
40. Trappler, B, Braunstein, JW, Moskowitz, G, and Friedman, S. Holocaust survivors in a primary care setting: Fifty years later. Psychol Rep (2002) 91(2):545–52. doi:10.2466/pr0.2002.91.2.545
41. Shin, KM, Chang, HY, Cho, S-M, Kim, NH, Kim, KA, and Chung, YK. Avoidance symptoms and delayed verbal memory are associated with post-traumatic stress symptoms in female victims of sexual violence. J Affective Disord (2015) 184:145–8. doi:10.1016/j.jad.2015.05.051
42. Somohano, VC, Rehder, KL, Dingle, T, Shank, T, and Bowen, S. PTSD symptom clusters and craving differs by primary drug of choice. J Dual Diagn (2019) 15(4):233–42. doi:10.1080/15504263.2019.1637039
43. Weera, MM, Schreiber, AL, Avegno, EM, and Gilpin, NW. The role of central amygdala corticotropin-releasing factor in predator odor stress-induced avoidance behavior and escalated alcohol drinking in rats. Neuropharmacology (2020) 166:107979. doi:10.1016/j.neuropharm.2020.107979
44. Whitaker, AM, and Gilpin, NW. Blunted hypothalamo-pituitary adrenal Axis response to predator odor predicts high stress reactivity. Physiol Behav (2015) 147:16–22. doi:10.1016/j.physbeh.2015.03.033
45. Schreiber, AL, Lu, YL, Baynes, BB, Richardson, HN, and Gilpin, NW. Corticotropin-releasing factor in ventromedial prefrontal cortex mediates avoidance of a traumatic stress-paired context. Neuropharmacology (2017) 113:323–30. doi:10.1016/j.neuropharm.2016.05.008
46. Schreiber, AL, McGinn, MA, Edwards, S, and Gilpin, NW. Predator odor stress blunts alcohol conditioned aversion. Neuropharmacology (2019) 144:82–90. doi:10.1016/j.neuropharm.2018.10.019
47. Kondoh, K, Lu, Z, Ye, X, Olson, DP, Lowell, BB, and Buck, LB. A specific area of olfactory cortex involved in stress hormone responses to predator odours. Nature (2016) 532(7597):103–6. doi:10.1038/nature17156
48. Takahashi, LK, Nakashima, BR, Hong, H, and Watanabe, K. The smell of danger: A behavioral and neural analysis of predator odor-induced fear. Neurosci Biobehavioral Rev (2005) 29(8):1157–67. doi:10.1016/j.neubiorev.2005.04.008
49. Albrechet-Souza, L, Schratz, CL, and Gilpin, NW. Sex differences in traumatic stress reactivity in rats with and without a history of alcohol drinking. Biol Sex Differences (2020) 11(1):27. doi:10.1186/s13293-020-00303-w
50. Hegab, I, and Wei, W. Neuroendocrine changes upon exposure to predator odors. Neuroendocrine Changes Upon Exposure Predator Odors Physiol Behav (2014) 131:149–55. doi:10.1016/j.physbeh.2014.04.041
51. Hadad, NA, Wu, L, Hiller, H, Krause, EG, Schwendt, M, and Knackstedt, LA. Conditioned stress prevents cue-primed cocaine reinstatement only in stress-responsive rats. Stress (2016) 19(4):406–18. doi:10.1080/10253890.2016.1189898
52. Cochrane, C, Malcolm, R, and Brewerton, T. The role of weight control as a motivation for cocaine abuse. Addict Behaviors (1998) 23(2):201–7. doi:10.1016/S0306-4603(97)00046-4
53. Bruening, AB, Perez, M, and Ohrt, TK. Exploring weight control as motivation for illicit stimulant use. Eat Behaviors (2018) 30:72–5. doi:10.1016/j.eatbeh.2018.06.002
54. Ersche, KD, Stochl, J, Woodward, JM, and Fletcher, PC. The skinny on cocaine: Insights into eating behavior and body weight in cocaine-dependent men. Appetite (2013) 71:75–80. doi:10.1016/j.appet.2013.07.011
55. Balopole, DC, Hansult, CD, and Dorph, D. Effect of cocaine on food intake in rats. Psychopharmacology (1979) 64(1):121–2. doi:10.1007/BF00427356
56. Thanos, PK, Cho, J, Kim, R, Michaelides, M, Primeaux, S, Bray, G, et al. Bromocriptine increased operant responding for high fat food but decreased chow intake in both obesity-prone and resistant rats. Behav Brain Res (2011) 217(1):165–70. doi:10.1016/j.bbr.2010.10.027
57. Laque, A, Wagner, GE, Matzeu, A, De Ness, GL, Kerr, TM, Carroll, AM, et al. Linking drug and food addiction via compulsive appetite. Br J Pharmacol (2022) 179(11):2589–609. doi:10.1111/bph.15797
58. Bane, AJ, McCoy, JG, Stump, BS, and Avery, DD. The effects of cocaine on dietary self-selection in female rats. Physiol Behav (1993) 54(3):509–13. doi:10.1016/0031-9384(93)90244-A
59. Wortley, KE, Chang, G-Q, Davydova, Z, Fried, SK, and Leibowitz, SF. Cocaine- and amphetamine-regulated transcript in the arcuate nucleus stimulates lipid metabolism to control body fat accrual on a high-fat diet. Regul Peptides (2004) 117(2):89–99. doi:10.1016/j.regpep.2003.08.005
60. Vicentic, A, and Jones, DC. The CART (cocaine- and amphetamine-regulated transcript) system in appetite and drug addiction. J Pharmacol Exp Ther (2007) 320(2):499–506. doi:10.1124/jpet.105.091512
61. Rogge, G, Jones, D, Hubert, GW, Lin, Y, and Kuhar, MJ. CART peptides: Regulators of body weight, reward and other functions. Nat Rev Neurosci (2008) 9(10):747–58. doi:10.1038/nrn2493
62. Yadav, VK, Oury, F, Suda, N, Liu, ZW, Gao, XB, Confavreux, C, et al. A serotonin-dependent mechanism explains the leptin regulation of bone mass, appetite, and energy expenditure. Cell (2009) 138(5):976–89. doi:10.1016/j.cell.2009.06.051
63. Rayner, DV, and Trayhurn, P. Regulation of leptin production: sympathetic nervous system interactions. J Mol Med (Berl) (2001) 79(1):8–20. doi:10.1007/s001090100198
64. Lynch, WJ, and Carroll, ME. Sex differences in the acquisition of intravenously self-administered cocaine and heroin in rats. Psychopharmacology (1999) 144(1):77–82. doi:10.1007/s002130050979
65. Anker, JJ, and Carroll, ME. Sex differences in the effects of allopregnanolone on yohimbine-induced reinstatement of cocaine seeking in rats. Drug and Alcohol Dependence (2010) 107(2–3):264–7. doi:10.1016/j.drugalcdep.2009.11.002
66. Buffalari, DM, Baldwin, CK, Feltenstein, MW, and See, RE. Corticotrophin releasing factor (CRF) induced reinstatement of cocaine seeking in male and female rats. Physiol Behav (2012) 105(2):209–14. doi:10.1016/j.physbeh.2011.08.020
67. Griffin, ML, Weiss, RD, Mirin, SM, and Lange, U. A comparison of male and female cocaine abusers. Arch Gen Psychiatry (1989) 46(2):122–6. doi:10.1001/archpsyc.1989.01810020024005
68.Substance Abuse and Mental Health Services Administration. (2017). Key substance use and mental health indicators in the United States: Results from the 2016 national survey on drug use and health. (HHS Publication No. SMA 17-5044, NSDUH Series H-52). Rockville, MD: Center for Behavioral Health Statistics and Quality, Substance Abuse and Mental Health Services Administration. Available from: https://www.samhsa.gov/data/ (Accessed December 12, 2022).
69. Quiñones-Jenab, V, and Jenab, S. Influence of sex differences and gonadal hormones on cocaine addiction. ILAR J (2012) 53(1):14–22. doi:10.1093/ilar.53.1.14
70. Back, SE, Brady, KT, Jackson, JL, Salstrom, S, and Zinzow, H. Gender differences in stress reactivity among cocaine-dependent individuals. Psychopharmacology (2005) 180(1):169–76. doi:10.1007/s00213-004-2129-7
71. Becker, JB, and Hu, M. Sex differences in drug abuse. Front Neuroendocrinology (2008) 29(1):36–47. doi:10.1016/j.yfrne.2007.07.003
72. Evans, SM, Haney, M, and Foltin, RW. The effects of smoked cocaine during the follicular and luteal phases of the menstrual cycle in women. Psychopharmacology (2002) 159(4):397–406. doi:10.1007/s00213-001-0944-7
73. Evans, SM, and Foltin, RW. Exogenous progesterone attenuates the subjective effects of smoked cocaine in women, but not in men. Neuropsychopharmacology (2006) 31(3):659–74. doi:10.1038/sj.npp.1300887
74. Reed, BG, and Carr, BR. The normal menstrual cycle and the control of ovulation. [Updated 2018 Aug 5]. In: KR Feingold, B Anawalt, and MR Blackman, editors. Endotext [internet]. South Dartmouth (MA: MDText.com, Inc. (2000). Available from: https://www.ncbi.nlm.nih.gov/books/NBK279054/ (Accessed January 21, 2023).
75. Moran-Santa Maria, MM, Sherman, BJ, Brady, KT, Baker, NL, Hyer, JM, Ferland, C, et al. Impact of endogenous progesterone on reactivity to yohimbine and cocaine cues in cocaine-dependent women. Pharmacol Biochem Behav (2018) 165:63–9. doi:10.1016/j.pbb.2017.11.001
76. Sinha, R, Fox, H, Hong, K-I, Sofuoglu, M, Morgan, PT, and Bergquist, KT. Sex steroid hormones, stress response, and drug craving in cocaine-dependent women: Implications for relapse susceptibility. Exp Clin Psychopharmacol (2007) 15(5):445–52. doi:10.1037/1064-1297.15.5.445
77. Fox, HC, Sofuoglu, M, Morgan, PT, Tuit, KL, and Sinha, R. The effects of exogenous progesterone on drug craving and stress arousal in cocaine dependence: Impact of gender and cue type. Psychoneuroendocrinology (2013) 38(9):1532–44. doi:10.1016/j.psyneuen.2012.12.022
78. Milivojevic, V, Fox, HC, Sofuoglu, M, Covault, J, and Sinha, R. Effects of progesterone stimulated allopregnanolone on craving and stress response in cocaine dependent men and women. Psychoneuroendocrinology (2016) 65:44–53. doi:10.1016/j.psyneuen.2015.12.008
79. Sofuoglu, M, Mitchell, E, and Kosten, T. Effects of progesterone treatment on cocaine responses in male and female cocaine users. Pharmacol Biochem Behav (2004) 78(4):699–705. doi:10.1016/j.pbb.2004.05.004
80. Lacy, RT, Austin, BP, and Strickland, JC. The influence of sex and estrous cyclicity on cocaine and remifentanil demand in rats. Addict Biol (2020) 25(1):e12716. doi:10.1111/adb.12716
Keywords: cocaine, self-administration, predator odor, stress, sex differences
Citation: Templeton-Jager TJ, Diarra S and Gilpin NW (2023) Sex differences in cocaine self-administration by Wistar rats after predator odor exposure. Adv. Drug Alcohol Res. 3:11245. doi: 10.3389/adar.2023.11245
Received: 02 February 2023; Accepted: 28 February 2023;
Published: 22 March 2023.
Edited by:
Emmanuel Onaivi, William Paterson University, United StatesReviewed by:
Adrienne McGinn, National Institutes of Health (NIH), United StatesHoward Becker, Medical University of South Carolina, United States
Copyright © 2023 Templeton-Jager, Diarra and Gilpin. This is an open-access article distributed under the terms of the Creative Commons Attribution License (CC BY). The use, distribution or reproduction in other forums is permitted, provided the original author(s) and the copyright owner(s) are credited and that the original publication in this journal is cited, in accordance with accepted academic practice. No use, distribution or reproduction is permitted which does not comply with these terms.
*Correspondence: Taylor J. Templeton-Jager, ttemp2@lsuhsc.edu; Nicholas W. Gilpin, ngilpi@lsuhsc.edu