- 1Department of Physiology, Louisiana State University Health Sciences Center, New Orleans, LA, United States
- 2Department of Cell Biology and Anatomy, Louisiana State University Health Sciences Center, New Orleans, LA, United States
- 3Alcohol and Drug Center of Excellence, School of Medicine, Louisiana State University Health Sciences Center, New Orleans, LA, United States
- 4Neuroscience Center of Excellence, School of Medicine, Louisiana State University Health Sciences Center, New Orleans, LA, United States
- 5Southeast Louisiana VA Healthcare System (SLVHCS), New Orleans, LA, United States
Alcohol withdrawal is characterized by various symptoms that include pain and negative affect in the absence of the drug. The neural underpinnings of these behaviors are not entirely understood, but orexin has emerged as a candidate target for the treatment of substance use disorders. Here, we explored changes in orexin system-related gene expression in brain regions important for mediating reward and stress, including the ventral tegmental area (VTA) and extended amygdala (including the central amygdala, nucleus accumbens shell, and bed nucleus of the stria terminalis), in adolescent and adult female Wistar rats following chronic alcohol exposure. We observed higher numbers of Hcrtr1- (orexin receptor 1)-expressing neurons in the VTA of adolescent and adult female rats during withdrawal from chronic alcohol exposure. The number of Hcrt1+ VTA neurons was negatively correlated with thermal sensitivity in adolescent female rats and anxiety-like behavior in adult female rats. These data suggest that chronic alcohol effects on orexin receptor expression in the VTA are related to specific behaviors that manifest during withdrawal, highlighting potential avenues for targeting alcohol withdrawal-associated behaviors across development.
Introduction
Alcohol use disorder (AUD) is a prevalent condition, affecting over 29 million Americans [1]. Among the clinical diagnostic criteria for AUD are physiological adaptations to alcohol use (e.g., presence of tolerance or withdrawal symptoms [2]), and these can drive continued alcohol use in dependent individuals in an effort to reduce symptomatology. Alcohol misuse and AUD prevalence has increased among women in recent years, reducing the size of the gap between women and men in these statistics, and stimulating interest in sex differences in AUD biology and behavior [3–5]. Of particular concern is adolescent drinking, which is a significant risk factor for later-life AUD development [6]. Recent work points to an increase in initiation of drinking [7], faster progression to heavy drinking episodes [8], and higher rate of alcohol misuse-related emergency department visits [9] among females during early adolescence compared to males. Collectively, these trends highlight the importance of including females in preclinical studies. Here, we investigated the effects of chronic alcohol exposure during two time points (adolescence and adulthood) on behavioral outcomes and region-specific gene expression in female Wistar rats.
Withdrawal symptoms, including negative affect and hyperalgesia, can facilitate relapse in individuals with AUD. While the neurobiology underlying alcohol withdrawal-related behaviors is not entirely understood, brain regions thought to be key for mediating those behaviors include the ventral tegmental area (VTA) in the midbrain, as well as the central amygdala (CeA), nucleus accumbens shell (NAcshell), and bed nucleus of the stria terminalis (BNST) [10] in the forebrain. Interestingly, there are robust reciprocal connections between the VTA and extended amygdala subregions, neurons in both regions are activated by acute and chronic alcohol exposure, and VTA-extended amygdala circuits display increased activity following acute/sub-chronic (e.g., VTA→NAc, BNST↔VTA, and CeA→VTA) and chronic (e.g., VTA→CeA) alcohol exposure [11].
Subregions of the extended amygdala are critical for mediating anxiety-like behavior and hyperalgesia during alcohol withdrawal in rodents [12]. For example, chronic alcohol exposure leads to anxiety-like behavior during withdrawal, an effect attenuated by pharmacological manipulation of NAcshell signaling in adolescent or adult male mice [13, 14], BNST signaling in adult male rats [15] and mice [16], and CeA signaling in adult male rats [17, 18]. Similarly, chronic alcohol exposure produces thermal and mechanical hyperalgesia during withdrawal, which is reversed by pharmacological and optogenetic manipulation of CeA neurons in adult male rats [19], as well as pharmacological alteration of BNST [20] and NAcshell [21] signaling in male and female mice and rats following adolescent alcohol exposure. Here, we analyzed expression patterns of genes within each region after chronic alcohol exposure during adolescence and adulthood.
We have previously demonstrated increased activity in a subset of VTA neurons of adult male rats during withdrawal from chronic alcohol exposure, as measured by Fos expression and spontaneous neural activity [22]. The mechanism by which this cellular activation occurs is unknown, but one possibility is via signaling of orexin (also known as hypocretin), a neuropeptide that is released into the VTA from lateral hypothalamus (LH) inputs, has a key role in numerous homeostatic and reward functions [23], and has an excitatory effect on VTA DA neurons when bath applied in vitro [24]. Orexin can therefore modulate the activity of projections (e.g., dopaminergic) from the VTA to regions in the extended amygdala; additionally, regions within the extended amygdala express orexin receptors and receive innervation directly from the hypothalamus [25]. Orexin signals through specific receptors, including the orexin-1 receptor (OX1R) and orexin-2 receptor (OX2R). OX1R activation has an excitatory effect on VTA neurons via CB1R-mediated disinhibition (i.e., 2-AG synthesis occurs downstream of OX1R activation, which then activates CB1Rs on presynaptic GABAergic neurons, thereby reducing inhibitory tone onto VTA projection neurons) [24]. In humans, blood orexin concentrations are increased in alcohol-dependent men and women during early withdrawal, and these levels gradually decrease over time during abstinence [26, 27]. Orexin levels are positively correlated with alcohol craving, depression, and anxiety in humans [28], and administration of orexin directly into the brain increases anxiety-like behaviors in mice, rats, and hamsters [29–32]. Traditionally, OX1Rs have been considered to play a role in reward seeking, with OX2Rs playing more of a functional role in arousal [33, 34]. More recent work utilizing systemic or whole-brain administration of OX2R antagonists report reduced alcohol self-administration, but not cue-induced reinstatement, in mice and rats [35–37], suggesting that both receptors may be involved in voluntary alcohol consumption, although inhibition of VTA OX2Rs specifically does not significantly impact drinking in mice [38]. Because several studies implicate VTA OX1R signaling specifically in behavioral response to drugs of misuse (e.g., [39–42]), the focus of the current project was to examine Hcrtr1 (OX1R) expression in the VTA and subregions of the extended amygdala during withdrawal from chronic alcohol exposure in adolescent and adult female rats.
Methods
Animals
All procedures were approved by the Institutional Animal Care and Use Committee of the Louisiana State University Health Sciences Center and were carried out in accordance with the National Institutes of Health guidelines. Female Wistar rats were obtained from Charles River Laboratories (Raleigh, NC, United States). Adolescent females were received at postnatal day 23 and triple-housed; adult females were received at postnatal day ∼56 and double-housed. In Experiment 3, we performed pilot studies in adult female Long Evans rats (Charles River Laboratories); because no differences between Wistar and Long Evans data were found (Figure 4; Supplementary Table S3), combined Long Evans and Wistar data are shown. All rats were maintained in a humidity- and temperature-controlled (22°C) vivarium on a 12-hour light/dark cycle (lights off at 7 AM), with ad libitum access to food and water. All animals were acclimated to housing conditions and light/dark cycles for 1 week before the start of experiments. A total of 78 rats (adolescent: n = 38; adults: n = 40) were used across experiments.
Chronic intermittent exposure to alcohol vapor
In adolescent and adult female rats, a chronic intermittent exposure to alcohol vapor model was used to induce alcohol dependence. Rats were triple-housed (adolescent) or pair-housed (adult) in sealed chambers (La Jolla Alcohol Research, La Jolla, CA, United States) and exposed to alcohol vapor for 14 h/day. Blood alcohol levels (BALs) were measured 1–2 times weekly and analyzed using an Analox AM1 analyzer (Analox Instruments, Lunenberg, MA, United States). Adolescent females were exposed to vapor (AIE; adolescent intermittent exposure) for 4 weeks, and adult females were exposed to CIE for 6 weeks. The exposure time for adolescent rats was limited in order to restrict alcohol exposure to the adolescent time period. All rats were maintained within a BAL range of 150–250 mg/dL over the experimental time course (adolescents: BAL = 222 ± 9.1 mg/dL; adults: 164 ± 5.8 mg/dL; mean ± SEM). Behavioral testing and sacrifice time point all occurred during acute withdrawal (6–8 h after vapor cessation, while BALs were at or near 0 mg/dL).
Surgeries
In Experiment 3, adult rats were anesthetized with isoflurane and mounted into a stereotaxic frame (Kopf Instruments, Tujunga, CA, United States). Custom 26 Ga bilateral cannulae (1.5 mm spacing, cut 9 mm below pedestal) were obtained from Plastics One (Roanoke, VA, United States). Cannulae were implanted above the VTA using the following coordinates from Bregma: −5.75 mm AP, +0.75 mm ML, −7.2 mm DV; injectors extended 1 mm below the pedestal for a final DV depth of −8.2 mm. Cannulae were secured to the skull with cranioplastic cement and stainless-steel anchor screws. After all surgical procedures, rats were monitored to ensure full recovery from anesthesia before being singly housed for one night. Rats were treated with the analgesic Meloxicam SR (4 mg/kg, s.c.) and the antibiotic cefazolin (20 mg/kg, i.m.) just prior to surgery. Weight and appearance were also monitored for a period of 7 d following surgery to detect any signs of distress or postoperative complications. Rats used for behavioral experiments were allowed 1 week to recover from any surgical procedure before the resumption of behavioral testing.
Behavior
Hargreaves test for thermal nociception
Hargreaves testing was performed in adolescent females during the withdrawal period (6–8 h after vapor turned off). Briefly, rats were allowed to acclimate for 30 min prior to each testing session, then placed in a Plexiglas enclosure (4 × 8 × 5″) on top of a glass panel raised 8″ above the tabletop. Each hindpaw was stimulated by a halogen light heat source from an IITC model 309 Hargreaves apparatus (75% of maximum light intensity; IITC Life Sciences, Woodland Hills, CA, United States). Latency to withdraw the hindpaw was measured twice for each hindpaw in alternating order, with a minimum of 1 min between measurements. A 20-s cutoff was used to prevent tissue damage in non-responsive subjects. Measurements across the 4 trials were averaged as the thermal nociception score for each rat. We have previously published Hargreaves behavioral data shown in adolescent females in Experiment 1 [43]; in the present study, we examined the brains of these experimental animals.
Elevated plus maze
Elevated plus maze (EPM) testing was performed in adult females during the withdrawal period (6–8 h after vapor turned off) in Experiment 2. Rats were allowed to acclimate for 30 min prior to testing, then placed in a black Plexiglas EPM apparatus, raised 50 cm from the floor and consisting of 10 × 25 cm arms, two of which were enclosed by walls 20 cm high. The lighting measured at the center of the maze was adjusted to ∼100 lux. Rats were placed in the center of the maze and allowed 5 min to explore the apparatus. Time spent in open arms was calculated as a percentage of the time spent in the open arms divided by the total time in the open and closed arms (omitting center time).
In a separate cohort of alcohol-naïve rats, anxiety-like behavior was evaluated following intra-VTA administration of orexin A (Tocris Bioscience, Minneapolis, MN, United States) or vehicle (Experiment 3). Orexin A (50 nM in aCSF) or vehicle (aCSF) was administered into the VTA through 33-gauge injectors over 2.5 min at a rate of 0.2 μL/min, and 1 additional minute was allowed for diffusion before injector removal. Animals underwent sham infusions on days preceding drug infusion to acclimate them to the infusion procedure. Behavioral testing occurred 5 min after VTA injection. Prior to sacrifice, rats received intra-VTA injections 200 nL Evans Blue, and brains were sectioned to verify proper injection site.
RT qPCR analysis of Hcrt
Lateral hypothalamus (LH)-containing tissue punches were taken from a separate cohort of AIE-exposed female Wistar rats, and total RNA was isolated using the miRNeasy Micro Kit (Qiagen, Hilden, Germany; Cat# 217084). Traces of contaminating DNA were removed using the DNA-free™ Kit following the manufacturer’s protocol (Invitrogen, Waltham, MA; Cat# AM1906). RNA concentration was measured with the NanoDrop® ND-1000 UV/Vis Spectrophotometer (ThermoFisher Scientific, Carlsbad, California). cDNA synthesis was performed using the High-Capacity cDNA Reverse Transcription Kit (ThermoFisher Scientific, Cat# 4368814). Briefly, 10 µL containing 40 ng of RNA were mixed with 10 µL of 2X Reverse Transcription Buffer without RNAse inhibitor and incubated in a thermocycler (PTC-100, Bio-Rad, Hercules, CA, United States) at 25°C for 10 min, 37°C for 120 min, and 85°C for 5 min. TaqMan™ Gene Expression Assay (FAM, ThermoFisher Scientific, Cat# 4331182) was used for quantitative real-time PCR analysis (qPCR) using 20 µL of a reaction containing 10 µL of TaqMan™ Universal Master Mix II, 1 µL of the specific primer targeting Hypocretin neuropeptide precursor (Hcrt, Rn00565995), 2 µL of the cDNA, and 7 µL of RNase-free water. Cycling conditions were 50°C for 2 min and 95°C for 10 min, followed by 44 cycles of 95°C for 15 s and 60°C for 1 min. A melt curve analysis was conducted by heating to 95°C, followed by gradual 0.5°C decrements down to 65°C to confirm the specificity of amplification. Each reaction was duplicated without template controls (NTC) or reverse transcriptase controls. Peptidylprolyl isomerase A (Ppia, Rn00690933) served as the housekeeping gene for normalization. PCR data was analyzed using the 2−ΔΔCT method, and results are presented as relative mRNA expression levels.
In situ hybridization
RNAscope was performed in fresh frozen tissue from AIE or CIE-exposed tissue (Experiments 1 and 2) to evaluate gene expression patterns in the VTA, CeA, BNST, and NAcshell. Rats were sacrificed during acute withdrawal from alcohol (6–8 h after vapor turned off), and brains were snap-frozen is isopentane and stored at −80°C until sectioning. A randomized strategy was used to select a subset of AIE/CIE and alcohol-naive brains for RNAscope analysis. Ten µm-thick coronal sections containing the NAcshell, BNST, CeA, and VTA were collected using a cryostat, mounted onto Superfrost glass slides, and stored at −80°C until the assay was performed. Each brain region was analyzed at the same approximate depth along the anterior-posterior axis for each rat, which are as follows: NAcshell, Bregma +1.20 mm; BNST, Bregma +0.00 mm; CeA, Bregma −2.40 mm; VTA, Bregma −5.88 mm.
In situ hybridization was performed using the RNAscope® Fluorescent Multiplex Reagent kit v1 following manufacturer’s recommendations (ACDBio, Newark, CA, United States) and as previously described [43, 44]. Briefly, slides were fixed in chilled 4% PFA then dehydrated using progressive ethanol washes. Sections were incubated for 30 min with Protease III at room temperature, washed in PBS, then incubated with probes for 2 h at 40°C. NAcshell-, BNST-, and CeA-containing tissue sections were incubated with probes targeting Hcrtr1 (Rn-Hcrtr1, Cat#. 444761), Drd1 (Rn-Drd1a-C2, Cat# 317031-C2), and Fos (Rn-Fos-C3, Cat# 403591-C3). VTA-containing sections were incubated with probes targeting Hcrtr1 (Rn-Hcrtr1, Cat#. 444761), Th (Rn-Th-C2, Cat# 314651-C2), and Fos (Rn-Fos-C3, Cat# 403591-C3). Sections were then washed and incubated with amplification reagents, per manufacturer instructions. Each time RNAscope was performed, a separate tissue section was incubated with a negative control probe (Cat# 320871), which was analyzed in order to determine the threshold for counting a positive signal in each channel [44]. Sections were coverslipped with Fluoro-Gel II with DAPI (Electron Microscopy Sciences, Hatfield, PA, United States; Cat# 17985-51) and imaged using a Zeiss AxioScan Z.1 slide scanner (Oberkochen, Germany) with a ×20 objective yielding images with a 0.22 μm/pixel resolution.
Images were analyzed using Qupath 0.4.1 [45] by quantifying the total number of puncta in each cell for each channel within a selected region of interest. Negative control probe-processed tissue was used to establish a threshold (defined as the sum of the mean number of puncta per cell and the standard deviation) for each channel. Only cells exceeding this threshold in processed tissue were counted as expressing mRNA of interest.
Statistical analysis
Statistical analysis was performed in Prism version 9.4.1 (GraphPad Software, La Jolla, CA, United States). Data were analyzed using two-tailed t-tests and linear regression, except where otherwise indicated. In Figure 2, a Mann-Whitney U-test was performed due to non-normal distribution of data. A p value of <0.05 was considered significant.
Results
Adolescent intermittent exposure (AIE) to ethanol
In Experiment 1, adolescent female rats were exposed to AIE from postnatal day 30 (p30)-p58, as previously reported [43]. Hargreaves testing for thermal nociception occurred during acute withdrawal from alcohol (6–8 h after vapor turned off); AIE-exposed females demonstrated a significantly lower latency to withdraw their hindpaw compared to air-exposed controls after 4 weeks of vapor exposure (t22 = 6.0, p < 0.0001, two-tailed t-test; Figure 1A). VTA-containing sections were used to evaluate expression of Hcrtr1, Fos, and Th (tyrosine hydroxylase, the enzyme involved in the rate limiting step of dopamine synthesis, and marker of dopamine neurons in the VTA; Figure 1B), and NAcshell-, BNST-, and CeA-containing sections were used to evaluate expression of Hcrtr1, Fos, and Drd1 (dopamine receptor 1; data not shown). When comparing tissue from AIE females to naïve controls in each brain region, we did not observe a significant increase in total Hcrtr1+ cells in the VTA of AIE rats compared to alcohol-naïve controls (expressed as a percentage of all DAPI+ neurons; Figure 1C), although we found a significantly greater number of Hcrtr1+ cells (expressed as a percentage of all Th+ neurons in the VTA; t14 = 2.9, p = 0.012, two-tailed t-test; Figure 1D). Approximately 25% of Hcrtr1+ VTA neurons expressed Th+, with no significant difference between AIE and alcohol-naïve control rats (25.1% ± 5.8% in alcohol-naïve controls; 24.5% ± 1.8% AIE; t14 = 0.11, p = 0.92, two-tailed t-test). No significant difference in Fos expression was observed in the VTA (t14 = 0.38, p = 0.71, two-tailed t-test), and no significant difference in expression of any markers analyzed in the extended amygdala brain regions was found (Supplementary Table S1), indicating region-specific changes in Hcrtr1 expression in AIE rats.
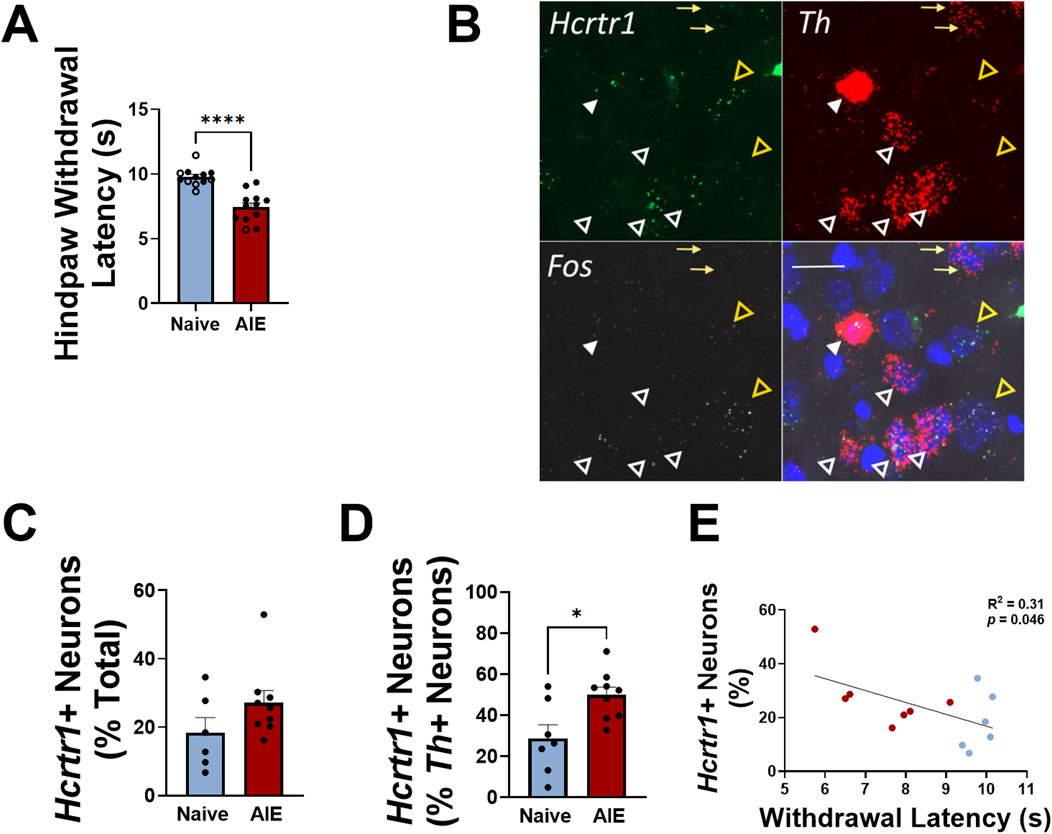
Figure 1. Adolescent females exhibit thermal hypersensitivity during alcohol withdrawal that is positively correlated with VTA Hcrtr1 expression. (A) Hindpaw withdrawal latency during Hargreaves testing in alcohol-naïve (blue) or AIE (red) female rats tested during acute withdrawal. Closed circles indicate rats used for tissue analysis; open circles indicate rats used for behavioral testing, but not tissue analysis. Reproduced with permission from “Figure 1. Intermittent alcohol exposure during adolescence produces sex-dependent long-lasting changes in pain sensitivity. (A) Timeline of the experiment including adolescence intermittent alcohol exposure (AIE), chronic withdrawal and pain-like behaviors from adolescent to early adulthood. Hargreaves (B) and Von Frey (C) thresholds in male rats during acute (∼7 hours after each daily exposure) and chronic alcohol withdrawal intervals. Control animals (AIR) are shown in open circles while exposed animals (AIE) are shown in closed purple circles. Hargreaves (D) and Von Frey (E) thresholds in female rats during acute (∼7 hours after each daily exposure) and chronic alcohol withdrawal intervals. Control animals (AIR) are shown in open circles while exposed animals (AIE) are shown in closed green circles. The data are represented as the mean ± SEM (males: n = 96; females: n = 36): *p < 0.05 AIR vs. AIE was assessed by two-way analysis of variance with repeated measures followed by Sidak’s post hoc test.” by Maria E. Secci, Leslie K. Kelley, Elizabeth M. Avegno, Eleanor B. Holmgren, Lily Chen, Sydney L. Rein, Sheila A. Engi, Virginia Quinlan, LisaWilson, Nicholas W. Gilpin, Tiffany A. Wills, licensed under CC BY-NC-ND. (B) Representative images of VTA Hcrtr1 (green), Fos (white), and Th (red) expression. Bottom right panel shows merged image with DAPI (blue). Yellow arrows indicate Th+, Hcrtr1-, Fos- neurons; white closed arrowheads indicate Th+, Hcrtr1+, Fos- neurons; white open arrowheads indicate Th+, Hcrtr1+, Fos+ neurons; open yellow arrowheads indicate Th-, Hcrtr1+, Fos+ neurons. Scale bar, 20 µm. (C) Number of VTA Hcrtr1+ neurons, expressed as a percentage of all neurons (DAPI+) in alcohol-naïve and AIE rats. (D) Number of VTA Hcrtr1+ neurons, expressed as a percentage of all Th+ neurons, in alcohol-naïve and AIE rats. (E) Number of VTA Hcrtr1 neurons plotted against hindpaw withdrawal latency for individual rats. Data in (A, C, D) shown as mean ± SEM; *p < 0.05; ****p < 0.0001; two-tailed t-test. Data in (E) analyzed using linear regression; R2 = 0.31; p = 0.046.
We next plotted the number of VTA Hcrtr1+ cells against hindpaw withdrawal latency and found a significant negative correlation between the two (R2 = 0.31, p = 0.046, linear regression analysis; Figure 1E). In tissue samples taken from a separate cohort of AIE or alcohol-naïve females, we performed qPCR analysis of Hcrt (hypocretin; orexin) expression in the lateral hypothalamus (LH) and found significantly higher expression of Hcrt in the LH of AIE females compared to alcohol-naïve controls (U = 5, p = 0.0059, Mann-Whitney U-test; Figure 2). Collectively, these data indicate region-specific alterations within the orexin signaling system following adolescent alcohol exposure, as well as a relationship between VTA Hcrtr1 expression and thermal hyperalgesia that manifests during alcohol withdrawal.
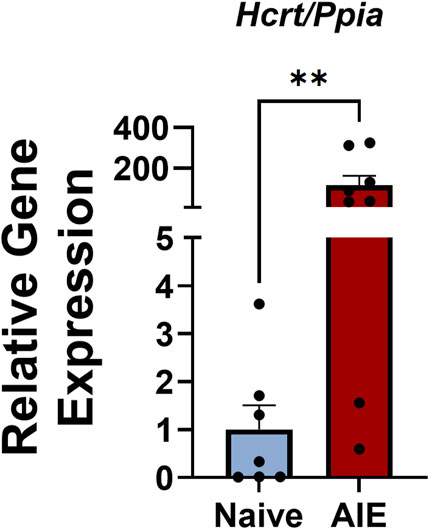
Figure 2. Adolescent females exhibit higher Hcrt expression in the LH during alcohol withdrawal relative to alcohol-naïve controls. Expression levels of Hcrt (relative to the housekeeping gene Ppia) in LH samples from alcohol-naïve (blue) and AIE (red) rats. Data shown as mean ± SEM; **p = 0.0059; Mann-Whitney U-test.
Chronic intermittent exposure (CIE) to ethanol during adulthood
In Experiment 2, female rats were exposed to CIE for 6 weeks throughout adulthood (beginning ∼9 weeks of age). Anxiety-like behavior was assessed using the elevated plus maze (EPM) during acute withdrawal from alcohol (6–8 h after vapor turned off); CIE-exposed females demonstrated a significantly lower time in the open arm of the EPM compared to alcohol-naïve controls (expressed as a percentage of total open+closed arm time; t14 = 2.29, p = 0.038, two-tailed t-test; Figure 3A). Total number of open arm entries was not significantly reduced in CIE rats compared to alcohol-naïve controls (t14 = 2.1, p = 0.056, two-tailed t-test; Figure 3B), and no difference between closed arm entries was observed between groups (t14 = 1.9, p = 0.075, two-tailed t-test; Figure 3C). VTA-containing sections were used to evaluate expression of Hcrtr1, Fos, and Th (Figure 3D), and NAcshell-, BNST-, and CeA-containing sections were used to evaluate expression of Hcrtr1, Fos, and Drd1. When comparing tissue from CIE females to naïve controls in each brain region, we observed a significantly greater number of Hcrtr1+ cells in the VTA of CIE rats compared to alcohol-naïve controls (expressed as a percentage of all DAPI+ neurons; t11 = 3.07, p = 0.011; Figure 3E), as well as a significantly greater number of Hcrtr1+ cells (expressed as a percentage of all Th+ neurons in the VTA; t11 = 2.4, p = 0.034, two-tailed t-test; Figure 3F). No significant difference in the number of Hcrtr1+ VTA neurons that expressed Th was observed between CIE and alcohol-naïve control rats (29.5% ± 6.3% in alcohol-naive controls; 40.7 ± 8.0% CIE; t11 = 1.1, p = 0.29, two-tailed t-test), and no significant difference in expression of any other markers was found in the VTA or other brain regions analyzed (Supplementary Table S2), indicating region-specific changes in Hcrtr1 expression in CIE rats. We next plotted the number of VTA Hcrtr1+ cells against open arm time in the EPM and found a significant negative correlation between the two (R2 = 0.35, p = 0.035, linear regression analysis; Figure 3G). These data indicate a difference in Hcrtr1 expression in CIE rats compared to alcohol-naïve controls that is limited to the VTA, as well as a relationship between VTA Hcrtr1 expression and increased anxiety-like behavior that manifests during alcohol withdrawal.
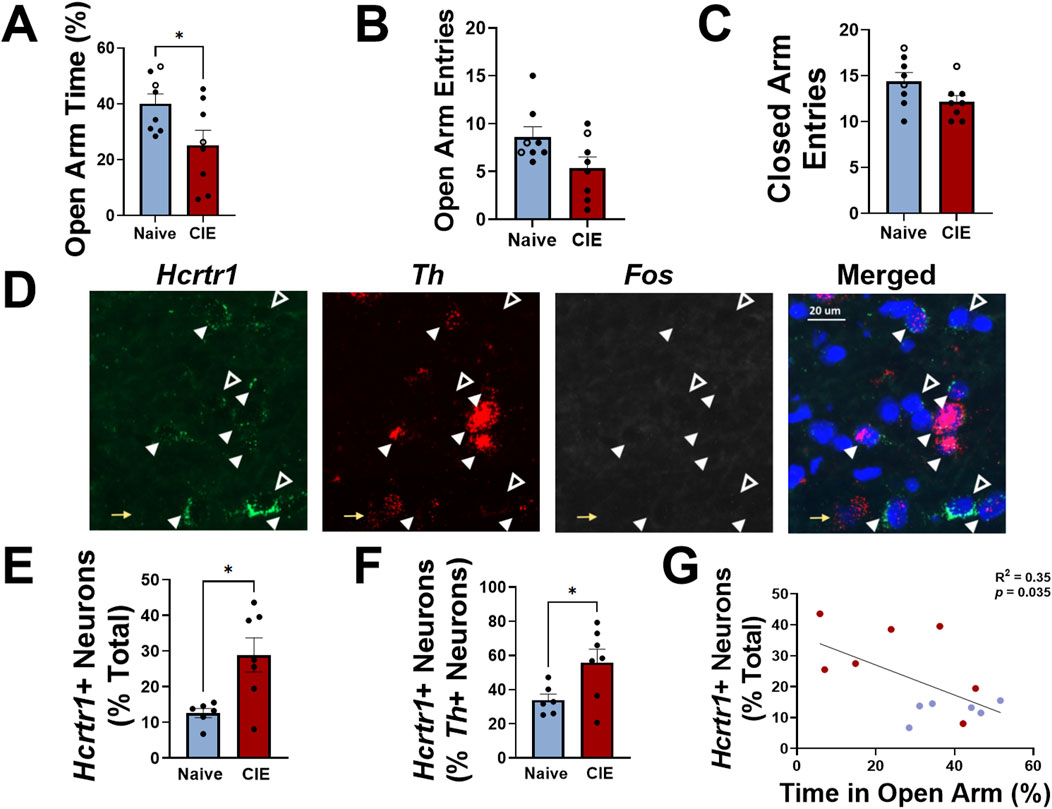
Figure 3. Adult females exhibit higher anxiety-like behavior than alcohol-naïve controls during alcohol withdrawal that is positively correlated with VTA Hcrtr1 expression. (A) Time spent in the open arm of an elevated plus maze (expressed as a percentage of total open and closed arm time) in alcohol-naïve (air-exposed, blue) or CIE (red) female rats tested during acute withdrawal. Total number of open (B) and closed (C) arm entries shown for both groups. Closed circles in (A–C) indicate rats used for tissue analysis; open circles indicate rats used for behavioral testing, but not tissue analysis. (D) Representative images of VTA Hcrtr1 (green), Fos (white), and Th (red) expression. Right panel shows merged image with DAPI (blue). Yellow arrows indicate Th+, Hcrtr1-, Fos- neurons; white closed arrowheads indicate Th+, Hcrtr1+, Fos- neurons; white open arrowheads indicate Th-, Hcrtr1+, Fos- neurons. Scale bar, 20 µm. (E) Number of VTA Hcrtr1+ neurons, expressed as a percentage of all neurons (DAPI+) in alcohol-naïve and CIE rats. (F) Number of VTA Hcrtr1+ neurons, expressed as a percentage of all Th+ neurons, in alcohol-naïve and CIE rats. (G) Number of VTA Hcrtr1 neurons plotted against EPM open arm time for individual rats. Data in (A–C); E, F shown as mean ± SEM; *p < 0.05; two-tailed t-test. Data in (G) analyzed using linear regression; R2 = 0.35; p = 0.035.
Intra-VTA administration of orexin A
In Experiment 3, we sought to determine whether intra-VTA administration of orexin A in otherwise experimentally naïve females could produce an anxiety-like phenotype observed in CIE females tested during withdrawal. In a separate cohort of adult female rats, guide cannulae were positioned above the VTA, and 500 nL injections of orexin A (50 nM in aCSF) or vehicle (aCSF) were delivered 5 min prior to behavioral testing in an EPM (Figures 4A, B). Intra-VTA orexin A administration significantly reduced time spent in the open arm of an EPM (t21 = 3.1, p = 0.0052, two-tailed t-test; Figure 4C), as well as the total number of open arm entries (t21 = 2.5, p = 0.023, two-tailed t-test; Figure 4D), suggesting that activation of VTA orexin receptors is sufficient to produce an anxiety-like phenotype similar to what is observed during withdrawal from chronic alcohol. Total number of closed arm entries did not differ between groups (t21 = 1.5, p = 0.14, two-tailed t-test; Figure 4E), indicating that intra-VTA orexin A administration did not reduce general locomotor activity in the EPM.
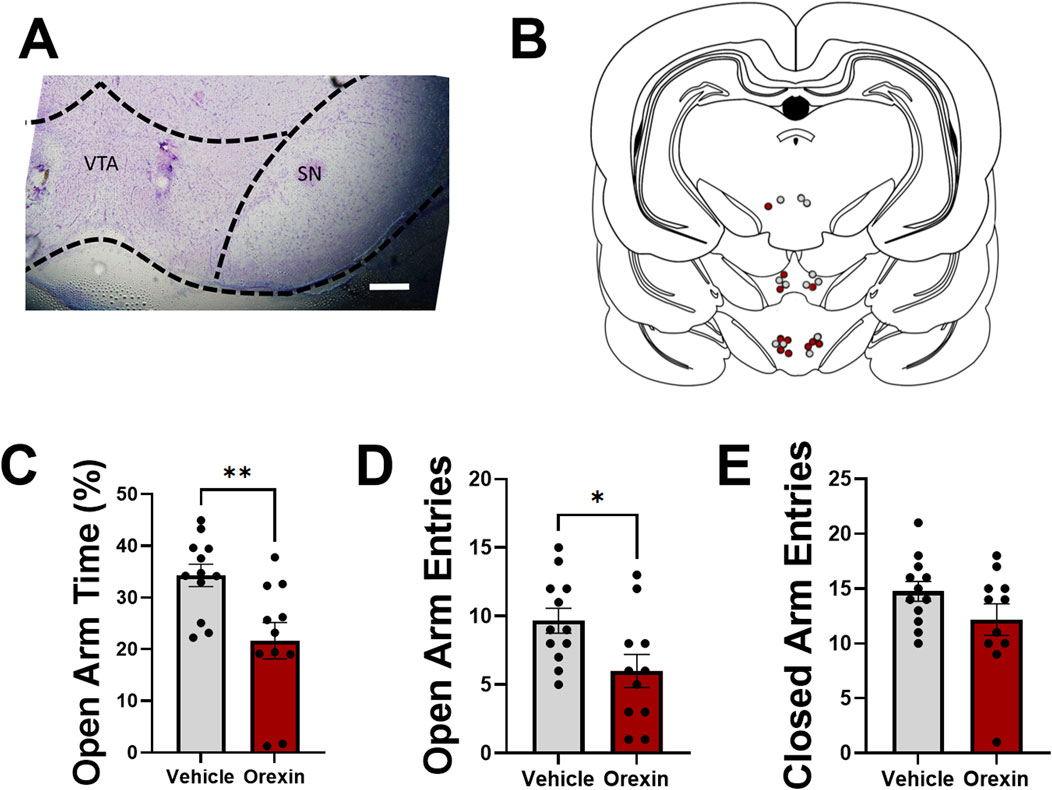
Figure 4. Intra-VTA administration of orexin-A increases anxiety-like behavior in alcohol-naïve adult female rats. (A) Representative injection site demonstrating cannula placement in VTA. Substantia nigra (SN) indicated for reference. Scale bar, 500 µm. (B) Map indicating representative injection sites of aCSF (grey) or orexin A (red) for all experimental rats. Bilateral injections were performed, although only one injection site is shown per rat for simplification. (C) Time spent in the open arm of an elevated plus maze (expressed as a percentage of total open and closed arm time) in rats following intra-VTA administration of vehicle (aCSF; grey) or orexin A (50 nM; red). Total number of open (D) and closed (E) arm entries shown for both groups. Data in (C–E) shown as mean ± SEM; *p < 0.05, **p < 0.01, two-tailed t-test.
Discussion
These results indicate a higher number of Hcrtr1-expressing neurons specifically in the VTA of female rats following chronic alcohol exposure, which is correlated with two separate alcohol withdrawal-induced behaviors, during adolescence and adulthood. These changes were not observed in regions within the extended amygdala, including the NAcshell, BNST, and CeA, indicating region-specific alterations in Hcrtr1 expression.
In humans with AUD, higher levels of circulating orexin are found during early withdrawal, which decrease over 4 weeks of abstinence [26, 27], and a positive correlation between orexin levels and negative affective symptoms including depression and anxiety has been reported in humans [28]. Administration of orexin in brain produces a high-anxiety phenotype in mice, rats, and hamsters [29–32], providing additional preclinical support for a role of orexin signaling in anxiogenic behavior. Here, we demonstrate an increase in VTA Hcrtr1 expression following chronic alcohol exposure that is significantly correlated with anxiety-like behavior, suggesting that higher levels of OX1R expression (and potentially greater VTA OX1R activation) may be linked to higher anxiety-like behavior. Additionally, we demonstrate that intra-VTA orexin A administration alone can produce an anxiety-like phenotype in otherwise experimentally naïve adult female rats. Because orexin A can bind to both OX1R and OX2Rs, and both OX1R and OX2R are expressed in the VTA [25], we cannot rule out the possibility that intra-VTA orexin A administration produces an anxiogenic effect via actions on OX2Rs. Acute i.c.v. administration of orexin A administration elicits a stress response in rats [46, 47] and an anxiogenic phenotype in mice and rats [31], although the specific brain regions involved in these behaviors are not defined. Our data demonstrate a role of the VTA in anxiogenic effects of orexin A specifically. Whether increased activation of VTA OX1Rs causes an anxiogenic phenotype during withdrawal from chronic alcohol remains to be determined, but our data do point to a relationship between the VTA orexin system and anxiety-like behavior.
The relationship between orexin and nociception is less straightforward, however. Preclinical studies have shown an analgesic effect of intravenous administration of orexin A in mice and rats via actions at the level of the spinal cord [48], and in a model of neuropathic pain, LH stimulation decreased thermal nociception in female rats via OX1R signaling [49], suggesting that orexin A can produce an antinociceptive effect by binding to OX1Rs in certain areas. Systemic administration of a dual OX1/OX2R antagonist reduced increased thermal and mechanical nociception in adult male C57BL/6J mice in a model of chronic predictable mild stress [50], indicating that blockade of OX1R signaling can also have an antinociceptive effect. These conflicting results may be due to the location of OX1Rs and potentially the preclinical model used to induce a pain-like phenotype. Here, we demonstrate an increase in Hcrt in the LH of female AIE rats compared to alcohol-naïve naïve controls, an increase in VTA Hcrtr1+, Th+ neurons, and a negative correlation between VTA Hcrtr1 expression and hindpaw withdrawal latency. While these data do not allow us to draw causal conclusions, they do raise a potential relationship between orexin signaling at the level of the VTA and heightened nociception during alcohol withdrawal. Future research can test this relationship directly by determining whether inhibition of VTA OX1Rs can rescue heightened thermal nociception in AIE rats compared to alcohol-naïve controls and/or by evaluating whether intra-VTA orexin administration can produce a hyperalgesia phenotype in otherwise experimentally naïve rats.
While our in situ hybridization analysis included multiple gene targets across several brain regions, the significant differences found were limited to VTA Hcrtr1 expression only, indicating regional specificity in expression of this gene. We did not observe changes in VTA Th expression or Drd1 expression in NAcshell, BNST, or CeA, and we did not observe changes in Fos expression in any region. We have previously demonstrated increased Fos protein expression in the VTA of CIE male rats during withdrawal [22], although it is likely that acute withdrawal (6–8 h after last exposure) is too diffuse a time point to observe changes in mRNA expression. Indeed, Fos mRNA expression typically peaks shortly after exposure to a stimulus and decreases within 1–2 h, while Fos protein expression persists for several hours after stimulus exposure [51]. Whether the changes in Hcrtr1 expression translate to changes in protein expression or function remains to be determined.
While VTA OX1R signaling has been implicated in behavioral response to other drugs of misuse, including cocaine, morphine, and methamphetamine [39–41], less is known about VTA OX1R and its relationship to behavioral dysregulation following chronic alcohol specifically. Collectively, our data provide evidence of altered Hcrtr1 expression that is region specific and correlated with two separate alcohol withdrawal-associated behaviors (thermal hyperalgesia and anxiety-like behavior) following chronic alcohol exposure at two separate developmental timepoints (adolescence and adulthood, respectively). Whether these relationships are maintained throughout development (i.e., whether VTA Hcrtr1 expression correlates with anxiety-like behavior during adolescence and thermal nociception in adulthood) remains to be determined. These data raise the possibility that VTA orexin signaling plays a role in mediating alcohol withdrawal-associated behaviors. Ongoing and future experiments will bridge these observations by determining whether orexin modulation of VTA neurons and/or LH-VTA circuit activity is necessary for these behaviors during WD from chronic alcohol, and whether these observations are found in male rats as well.
Data availability statement
The raw data supporting the conclusions of this article will be made available by the authors, without undue reservation.
Ethics statement
The animal study was approved by Institutional Animal Care and Use Committee of the Louisiana State University Health Sciences Center. The study was conducted in accordance with the local legislation and institutional requirements.
Author contributions
EA, TW, and NG designed the experiments; SC, MS, LK, NS, KR-G, and EA performed research; MS, KR-G, and EA quantified and analyzed data; SC and EA wrote the paper; All authors contributed to the article and approved the submitted version.
Funding
The author(s) declare financial support was received for the research and/or publication of this article. The work was Supported by National Institute of Health grants K01 AA028541 (EA), U01 AA028709 (NG/TW), and P60 AA009803 (KRG).
Conflict of interest
NG owns shares in Glauser Life Sciences, Inc., a start-up company with interest in development of therapeutics for treatment of mental illness.
The remaining authors declare that the research was conducted in the absence of any commercial or financial relationships that could be construed as a potential conflict of interest.
Generative AI statement
The author(s) declare that no Generative AI was used in the creation of this manuscript.
Supplementary material
The Supplementary Material for this article can be found online at: https://www.frontierspartnerships.org/articles/10.3389/adar.2025.14199/full#supplementary-material
References
1. Koob, GF. Alcohol use disorder treatment: problems and solutions. Annu Rev Pharmacol Toxicol (2024) 64:255–75. doi:10.1146/annurev-pharmtox-031323-115847
2. American Psychiatric Association. Diagnostic and statistical manual of mental disorders (5th ed.) (2013). Arlington, VA: American Psychiatric Association Publishing.
3. Flores-Bonilla, A, and Richardson, HN. Sex differences in the neurobiology of alcohol use disorder. Alcohol Res (2020) 40(2):04. doi:10.35946/arcr.v40.2.04
4. Guinle, MIB, and Sinha, R. The role of stress, trauma, and negative affect in alcohol misuse and alcohol use disorder in women. Alcohol Res (2020) 40(2):05. doi:10.35946/arcr.v40.2.05
5. White, A, Castle, IJ, Chen, CM, Shirley, M, Roach, D, and Hingson, R. Converging patterns of alcohol use and related outcomes among females and males in the United States, 2002 to 2012. Alcohol Clin Exp Res (2015) 39(9):1712–26. doi:10.1111/acer.12815
6. Grant, JD, Scherrer, JF, Lynskey, MT, Lyons, MJ, Eisen, SA, Tsuang, MT, et al. Adolescent alcohol use is a risk factor for adult alcohol and drug dependence: evidence from a twin design. Psychol Med (2006) 36(1):109–18. doi:10.1017/S0033291705006045
7. Cheng, HG, Cantave, MD, and Anthony, JC. Taking the first full drink: epidemiological evidence on male-female differences in the United States. Alcohol Clin Exp Res (2016) 40(4):816–25. doi:10.1111/acer.13028
8. Cheng, HG, and Anthony, JC. Male-female differences in the onset of heavy drinking episode soon after first full drink in contemporary United States: from early adolescence to young adulthood. Drug Alcohol Depend (2018) 190:159–65. doi:10.1016/j.drugalcdep.2017.12.035
9. Naeger, S. Emergency department visits involving underage alcohol misuse: 2010 to 2013. In: The CBHSQ report. Rockville (MD): substance Abuse and mental Health services administration. US (2013). p. 1–14.
10. Alheid, GF, and Heimer, L. New perspectives in basal forebrain organization of special relevance for neuropsychiatric disorders: the striatopallidal, amygdaloid, and corticopetal components of substantia innominata. Neuroscience (1988) 27(1):1–39. doi:10.1016/0306-4522(88)90217-5
11. Avegno, EM, and Gilpin, NW. Reciprocal midbrain-extended amygdala circuit activity in preclinical models of alcohol use and misuse. Neuropharmacology (2022) 202:108856. doi:10.1016/j.neuropharm.2021.108856
12. Koob, GF. Neurocircuitry of alcohol addiction: synthesis from animal models. Handb Clin Neurol (2014) 125:33–54. doi:10.1016/B978-0-444-62619-6.00003-3
13. Lee, KM, Coelho, MA, Class, MA, Sern, KR, Bocz, MD, and Szumlinski, KK. mGlu5 receptor blockade within the nucleus accumbens shell reduces behavioral indices of alcohol withdrawal-induced anxiety in mice. Front Pharmacol (2018) 9:1306. doi:10.3389/fphar.2018.01306
14. Rose, JH, Karkhanis, AN, Chen, R, Gioia, D, Lopez, MF, Becker, HC, et al. Supersensitive kappa opioid receptors promotes ethanol withdrawal-related behaviors and reduce dopamine signaling in the nucleus accumbens. Int J Neuropsychopharmacol (2016) 19(5):pyv127. doi:10.1093/ijnp/pyv127
15. Ferragud, A, Velazquez-Sanchez, C, Minnig, MA, Sabino, V, and Cottone, P. Pituitary adenylate cyclase-activating polypeptide (PACAP) modulates dependence-induced alcohol drinking and anxiety-like behavior in male rats. Neuropsychopharmacology (2021) 46(3):509–18. doi:10.1038/s41386-020-00904-4
16. Marcinkiewcz, CA, Dorrier, CE, Lopez, AJ, and Kash, TL. Ethanol induced adaptations in 5-HT2c receptor signaling in the bed nucleus of the stria terminalis: implications for anxiety during ethanol withdrawal. Neuropharmacology (2015) 89:157–67. doi:10.1016/j.neuropharm.2014.09.003
17. Harper, KM, Knapp, DJ, Butler, RK, Cook, CA, Criswell, HE, Stuber, GD, et al. Amygdala arginine vasopressin modulates chronic ethanol withdrawal anxiety-like behavior in the social interaction task. Alcohol Clin Exp Res (2019) 43(10):2134–43. doi:10.1111/acer.14163
18. Pandey, SC, Roy, A, and Zhang, H. The decreased phosphorylation of cyclic adenosine monophosphate (cAMP) response element binding (CREB) protein in the central amygdala acts as a molecular substrate for anxiety related to ethanol withdrawal in rats. Alcohol Clin Exp Res (2003) 27(3):396–409. doi:10.1097/01.ALC.0000056616.81971.49
19. Avegno, EM, Lobell, TD, Itoga, CA, Baynes, BB, Whitaker, AM, Weera, MM, et al. Central amygdala circuits mediate hyperalgesia in alcohol-dependent rats. J Neurosci (2018) 38(36):7761–73. doi:10.1523/JNEUROSCI.0483-18.2018
20. Bertagna, NB, Holmgren, EB, Engi, SA, Ha, L, Cruz, FC, Albrechet-Souza, L, et al. BNST CRF receptor type 1 modulates mechanical hypersensitivity induced by adolescent alcohol exposure in adult female mice. Psychopharmacology (Berl). (2024) 241:2513–23. doi:10.1007/s00213-024-06693-8
21. Kelley, AM, Del Valle, EJ, Zaman, S, and Karkhanis, AN. Adolescent ethanol exposure promotes mechanical allodynia and alters dopamine transmission in the nucleus accumbens shell. Pain (2024) 165(6):e55–e64. doi:10.1097/j.pain.0000000000003097
22. Avegno, EM, Kasten, CR, Snyder, WB, Kelley, LK, Lobell, TD, Templeton, TJ, et al. Alcohol dependence activates ventral tegmental area projections to central amygdala in male mice and rats. Addict Biol (2020) 26:e12990. doi:10.1111/adb.12990
23. Harris, GC, Wimmer, M, and Aston-Jones, G. A role for lateral hypothalamic orexin neurons in reward seeking. Nature (2005) 437(7058):556–9. doi:10.1038/nature04071
24. Tung, LW, Lu, GL, Lee, YH, Yu, L, Lee, HJ, Leishman, E, et al. Orexins contribute to restraint stress-induced cocaine relapse by endocannabinoid-mediated disinhibition of dopaminergic neurons. Nat Commun (2016) 7:12199. doi:10.1038/ncomms12199
25. Marcus, JN, Aschkenasi, CJ, Lee, CE, Chemelli, RM, Saper, CB, Yanagisawa, M, et al. Differential expression of orexin receptors 1 and 2 in the rat brain. J Comp Neurol (2001) 435(1):6–25. doi:10.1002/cne.1190
26. Ziolkowski, M, Czarnecki, D, Budzynski, J, Rosinska, Z, Zekanowska, E, and Goralczyk, B. Orexin in patients with alcohol dependence treated for relapse prevention: a pilot study. Alcohol Alcohol (2016) 51(4):416–21. doi:10.1093/alcalc/agv129
27. Bayerlein, K, Kraus, T, Leinonen, I, Pilniok, D, Rotter, A, Hofner, B, et al. Orexin A expression and promoter methylation in patients with alcohol dependence comparing acute and protracted withdrawal. Alcohol (2011) 45(6):541–7. doi:10.1016/j.alcohol.2011.02.306
28. von der Goltz, C, Koopmann, A, Dinter, C, Richter, A, Grosshans, M, Fink, T, et al. Involvement of orexin in the regulation of stress, depression and reward in alcohol dependence. Horm Behav (2011) 60(5):644–50. doi:10.1016/j.yhbeh.2011.08.017
29. Johnson, PL, Truitt, W, Fitz, SD, Minick, PE, Dietrich, A, Sanghani, S, et al. A key role for orexin in panic anxiety. Nat Med (2010) 16(1):111–5. doi:10.1038/nm.2075
30. Avolio, E, Alo, R, Carelli, A, and Canonaco, M. Amygdalar orexinergic-GABAergic interactions regulate anxiety behaviors of the Syrian golden hamster. Behav Brain Res (2011) 218(2):288–95. doi:10.1016/j.bbr.2010.11.014
31. Suzuki, M, Beuckmann, CT, Shikata, K, Ogura, H, and Sawai, T. Orexin-A (hypocretin-1) is possibly involved in generation of anxiety-like behavior. Brain Res (2005) 1044(1):116–21. doi:10.1016/j.brainres.2005.03.002
32. Li, Y, Li, S, Wei, C, Wang, H, Sui, N, and Kirouac, GJ. Orexins in the paraventricular nucleus of the thalamus mediate anxiety-like responses in rats. Psychopharmacology (Berl). (2010) 212(2):251–65. doi:10.1007/s00213-010-1948-y
33. Aston-Jones, G, Smith, RJ, Sartor, GC, Moorman, DE, Massi, L, Tahsili-Fahadan, P, et al. Lateral hypothalamic orexin/hypocretin neurons: a role in reward-seeking and addiction. Brain Res (2010) 1314:74–90. doi:10.1016/j.brainres.2009.09.106
34. Mahler, SV, Smith, RJ, Moorman, DE, Sartor, GC, and Aston-Jones, G. Multiple roles for orexin/hypocretin in addiction. Prog Brain Res (2012) 198:79–121. doi:10.1016/B978-0-444-59489-1.00007-0
35. Brown, RM, Khoo, SY, and Lawrence, AJ. Central orexin (hypocretin) 2 receptor antagonism reduces ethanol self-administration, but not cue-conditioned ethanol-seeking, in ethanol-preferring rats. Int J Neuropsychopharmacol (2013) 16(9):2067–79. doi:10.1017/S1461145713000333
36. Shoblock, JR, Welty, N, Aluisio, L, Fraser, I, Motley, ST, Morton, K, et al. Selective blockade of the orexin-2 receptor attenuates ethanol self-administration, place preference, and reinstatement. Psychopharmacology (Berl). (2011) 215(1):191–203. doi:10.1007/s00213-010-2127-x
37. Anderson, RI, Becker, HC, Adams, BL, Jesudason, CD, and Rorick-Kehn, LM. Orexin-1 and orexin-2 receptor antagonists reduce ethanol self-administration in high-drinking rodent models. Front Neurosci (2014) 8:33. doi:10.3389/fnins.2014.00033
38. Olney, JJ, Navarro, M, and Thiele, TE. The role of orexin signaling in the ventral tegmental area and central amygdala in modulating binge-like ethanol drinking behavior. Alcohol Clin Exp Res (2017) 41(3):551–61. doi:10.1111/acer.13336
39. Borgland, SL, Taha, SA, Sarti, F, Fields, HL, and Bonci, A. Orexin A in the VTA is critical for the induction of synaptic plasticity and behavioral sensitization to cocaine. Neuron (2006) 49(4):589–601. doi:10.1016/j.neuron.2006.01.016
40. Narita, M, Nagumo, Y, Hashimoto, S, Khotib, J, Miyatake, M, et al. Direct involvement of orexinergic systems in the activation of the mesolimbic dopamine pathway and related behaviors induced by morphine. J Neurosci (2006) 26(2):398–405. doi:10.1523/JNEUROSCI.2761-05.2006
41. Zamanirad, F, Fattahi, M, Amirteymori, H, Mousavi, Z, and Haghparast, A. The role of orexin-1 receptors within the ventral tegmental area in the extinction and reinstatement of methamphetamine place preference. Behav Brain Res (2023) 453:114608. doi:10.1016/j.bbr.2023.114608
42. Muschamp, JW, Hollander, JA, Thompson, JL, Voren, G, Hassinger, LC, Onvani, S, et al. Hypocretin (orexin) facilitates reward by attenuating the antireward effects of its cotransmitter dynorphin in ventral tegmental area. Proc Natl Acad Sci U S A. (2014) 111(16):E1648–1655. doi:10.1073/pnas.1315542111
43. Secci, ME, Kelley, LK, Avegno, EM, Holmgren, EB, Chen, L, Rein, SL, et al. Adolescent alcohol exposure produces sex-specific long-term hyperalgesia via changes in central amygdala circuit function. Biol Psychiatry (2024) 95(3):207–19. doi:10.1016/j.biopsych.2023.09.006
44. Secci, ME, Reed, T, Quinlan, V, Gilpin, NW, and Avegno, EM. Quantitative analysis of gene expression in RNAscope-processed brain tissue. Bio Protoc (2023) 13(1):e4580. doi:10.21769/BioProtoc.4580
45. Bankhead, P, Loughrey, MB, Fernández, JA, Dombrowski, Y, McArt, DG, Dunne, PD, et al. QuPath: open source software for digital pathology image analysis. Scientific Rep (2017) 7(1):16878. doi:10.1038/s41598-017-17204-5
46. España, RA, Plahn, S, and Berridge, CW. Circadian-dependent and circadian-independent behavioral actions of hypocretin/orexin. Brain Res (2002) 943(2):224–36. doi:10.1016/s0006-8993(02)02653-7
47. Ida, T, Nakahara, K, Katayama, T, Murakami, N, and Nakazato, M. Effect of lateral cerebroventricular injection of the appetite-stimulating neuropeptide, orexin and neuropeptide Y, on the various behavioral activities of rats. Brain Res (1999) 821(2):526–9. doi:10.1016/s0006-8993(99)01131-2
48. Bingham, S, Davey, PT, Babbs, AJ, Irving, EA, Sammons, MJ, Wyles, M, et al. Orexin-A, an hypothalamic peptide with analgesic properties. Pain (2001) 92(1-2):81–90. doi:10.1016/s0304-3959(00)00470-x
49. Wardach, J, Wagner, M, Jeong, Y, and Holden, JE. Lateral hypothalamic stimulation reduces hyperalgesia through spinally descending orexin-A neurons in neuropathic pain. West J Nurs Res (2016) 38(3):292–307. doi:10.1177/0193945915610083
50. Chavan, P, Chikahisa, S, Shiuchi, T, Shimizu, N, Dalanon, J, Okura, K, et al. Dual orexin receptor antagonist drug suvorexant can help in amelioration of predictable chronic mild stress-induced hyperalgesia. Brain Res Bull (2022) 188:39–46. doi:10.1016/j.brainresbull.2022.07.011
Keywords: alcohol, nociception, female, orexin, ventral tegmental area, anxiety-like behavior, withdrawal
Citation: Cruise S, Secci ME, Kelley LK, Sharfman NM, Rodriguez-Graciani K, Wills TA, Gilpin NW and Avegno EM (2025) Chronic alcohol withdrawal-associated increases in VTA Hcrtr1 expression are associated with heightened nociception and anxiety-like behavior in female rats. Adv. Drug Alcohol Res. 5:14199. doi: 10.3389/adar.2025.14199
Received: 11 December 2024; Accepted: 28 February 2025;
Published: 03 April 2025.
Edited by:
Lucia Hipolito, University of Valencia, SpainReviewed by:
Esi Domi, University of Camerino, ItalyHoward Becker, Medical University of South Carolina, United States
Yolanda Campos-Jurado, Washington University in St. Louis, United States
Copyright © 2025 Cruise, Secci, Kelley, Sharfman, Rodriguez-Graciani, Wills, Gilpin and Avegno. This is an open-access article distributed under the terms of the Creative Commons Attribution License (CC BY). The use, distribution or reproduction in other forums is permitted, provided the original author(s) and the copyright owner(s) are credited and that the original publication in this journal is cited, in accordance with accepted academic practice. No use, distribution or reproduction is permitted which does not comply with these terms.
*Correspondence: Elizabeth M. Avegno, ZWF2ZWduQGxzdWhzYy5lZHU=