- School of Electrical and Mechanical Engineering, University of Portsmouth, Portsmouth, United Kingdom
The UK’s aviation sector currently accounts for about 8% of the country’s total greenhouse gas emissions. In order to meet the country’s ambitious Net-Zero goals, significant change in the industry will be necessary over the coming decade. While a variety of technologies have been proposed with this goal in mind, due to the slow-moving nature of the industry, the most feasible of these is the increased use of sustainable aviation fuel (SAF) that requires little to no change to existing aircraft systems, procedures, as well as ground facilities. This fact is further supported by the passing of the UK’s 2030 SAF mandate. SAF is a biofuel produced from 100% renewable waste or residue materials, called feedstock. Therefore, due to the many options available as potential sources for SAF, it is important to look at the feasibility, both economic and environmental, of these various sources and their various blends. This paper analyses a number of these potential SAF sources by exploring the current state of the SAF industry as well as the current availability of feedstock. Next, a detailed analysis into the environmental and economic aspects of each fuel blend is done. These findings are fed into a novel weighting algorithm that is designed to assess a number of factors, including various technical characteristics and feasibility studies, as well as produce a detailed outlook of the future potential of each fuel blend. This work would support the industry in making decisions related to their SAF fuel blend options.
Introduction
The UK government’s Sustainable Aviation Fuel (SAF) mandate is set to be implemented in 2025, it will determine that at least 10% of the jet fuel used in the UK be made from sustainable feedstocks by 2030. The SAF program has three main goals: to create a secure and growing UK SAF demand, build up a domestic SAF industry, and work with industry and investors to build a long-term supply [1]. In comparison to the EU’s SAF targets for 2030 the UK’s 10% goal is more ambitious than the EU’s 2% by 2025 and 70% by 2050 goals, however the EU’s involvement of multiple countries would naturally mean that the targets need to be lower to be obtainable across multiple countries and governments [2].
SAF is a biofuel which is produced from up to 100% renewable waste and residue raw materials, a variety of different feedstocks can be used including: used cooking oil, recycled carbon fuels (RCF), agricultural and forestry residues, waste fat and grease, corn grain and oil seeds. All SAF is made from low carbon feedstocks and is required to lessen lifecycle emissions by at least 70% compared to fossil jet fuels whilst not displacing or competing with food crops. These biofuels can be easily blended with fossil jet fuel and used in existing aircraft, engines and infrastructure with the added benefit of improving aircraft performance as SAF contains fewer aromatic compounds and burns cleaner in jet engines [3]. Further studies into the affects of SAF on aircraft performance show increased thrust and range in aircraft using SAF over normal fossil jet fuels alongside just the environmental benefits [4].
The aim of this project is to investigate the different feedstocks used for SAF from both environmental and economic perspectives to determine which would be most effective at making the UK 10% 2030 SAF mandate feasible. This will involve a literature review covering the current state of the SAF industry and available feedstocks, an environmental analysis looking at the difference in energy density, emissions and conversion methods, an economic analysis into the levelized cost of energy (LCOE) for different fuel blends and the costs associated with building a long-term supply. A mathematical algorithm will then be designed to find which feedstock meets the given criteria the best, followed by an in-depth evaluation to analyze the data further. The algorithm will involve identifying key factors during the analysis which will be ranked in order of importance, the feedstocks will then be given a weighting for each factor with the lowest being the best. The final result will be found by putting the results for each factor together, the feedstock with the lowest overall result will be the most feasible according to this algorithm.
Literature Review
Legislation and Schemes
The International Civil Aviation Organization (ICAO) adopted a new scheme in 2016 known as the Carbon Offsetting and Reduction Scheme for International Aviation (CORSIA) which aims to stabilize the net CO2 emissions of international air traffic at levels from 2020 and will be a major long-term driver for international SAF demand [5]. Just by using SAF over standard fossil fuels a reduction of CO2 emissions of up to 80% is possible based on lifecycle emissions. This is possible despite SAF still producing emissions as the carbon they use is already a part of the carbon cycle and is not being directly taken from the ground and burned. For SAF the production process effectively reabsorbs the emissions produced during flight [6]. Investments and interest in SAF technology is growing steadily. The first biofuel test flight took place in 2008 and since then over 689,500 commercial flights have been completed using SAF worldwide since 2011 [7].
A variety of grants and incentives are likely to be implemented in order to decrease the minimum selling price, lowering risk for investors and encouraging further investment despite SAF costs being likely to remain above that of conventional jet fuel in the coming years [8]. For example, the UK Emission Trading Scheme (ETS) which aims to lower the cost difference between SAF and conventional jet fuel, allowing aircraft operators to claim a reduction in UK ETS obligations on ETS routes when reported [9].
From an environmental perspective, the UK mandate will require that SAF have overall lifecycle emission savings of at least 50%–70% when fully replacing fossil derived kerosene [10]. This lines up with the CORSIA scheme which requires all fuels to generate lower carbon emissions on a lifecycle basis than conventional fossil jet fuel and should not be made from biomass obtained from high carbon stock [11]. Other legislative bodies such as the Renewable Transport Fuel Obligation (RTFO) and the UK government state that alternative fuel blends must be able to be have a total renewable content of at least 25% by volume in the final blend and meet the necessary British standards (BS EN 228 or BS EN 590) [12]. Alongside this, SAF is currently limited internationally to being used as a drop-in fuel where it makes up no more than 50% per liter of fuel used in the aircraft [5].
UK SAF Goals
The UK’s SAF mandate “Jet Zero” is set to be implemented in 2025, it will require at least 10% of jet fuel used in the UK to be made from sustainable feedstocks by 2030. The three major goals for the future of SAF in the UK are: to create a secure and growing demand, build up a domestic industry and work with investors to build a stable long-term supply [1]. This is supported further by the UK’s 2050 Net Zero goals as the transportation industry as a whole takes up over a quarter of UK emissions making it the largest emitting sector. When included, 10% of these emissions came from international aviation alone as of 2021 [13].
Current UK SAF Usage and Industry
SAF is currently produced and used within the UK, however, the production capacity is currently limited due to high costs and limited access to feedstock and infrastructure [8]. Since six conversion processes were certified for safe production of SAF in December 2018, over 150,000 commercial flights have been completed with the resulting fuels, despite this only 26 million liters of SAF were supplied in the UK in 2022 [14]. If the UK goals of creating a secure and growing demand and industry within the UK succeed, there is potential for it to support at least 5,200 jobs by 2035 within the UK and a further 13,600 through global exports [15]. The first transatlantic flight by a large passenger plane using a 100% SAF mix took place on the 28th November 2023 flying from London to New York demonstrating an increasing interest and attention for SAF around the world [16].
Feedstocks and Sources
There is a wide variety of feedstocks that can be used in the production of SAF, some main examples of these are: used cooking oil, recycled carbon fuels (RCF), agricultural and forestry residues, waste fat and grease, corn grain and oil seeds. However, under the UK mandate, SAF must only be produced from wastes and residues or low carbon electricity, crop-based biofuels will not be permitted to ensure that the production of SAF does not put pressure on food crop production or farmland use [1]. In regards to the UK, fuels derived from waste are most likely to be the primary source of SAF for the time being though this will vary internationally [5]. The best conversion process from a technical perspective, would most likely be producing SAF from waste oils, however, this feedstock has constrained resources and is already largely used in road sectors [17]. The main fuels being discussed at the moment for SAF production in the UK are hydroprocessed esters and fatty acids (HEFA), recycled carbon fuels (RCF), power to liquid fuels (PtL) and municipal solid waste (MSW) derived fuels.
Hydroprocessed Esters and Fatty Acids (HEFA)
The most common feedstock used in SAF production currently is HEFA. This is down to its low costs and high greenhouse gas (GHG) savings (87.7% relative to fossil fuels) [1]. They are produced from the hydrogenation of waste and vegetable oils and can be blended with petroleum-based kerosene up to 50% per volume [17]. However, it is likely that a HEFA cap will be introduced in order to reduce the risk of the UK becoming overly reliant on, and relieve pressure from, a single feedstock whilst encouraging the continued development of other sources and technologies [18].
Recycled Carbon Fuels (RCF)
RCFs are another example of a waste derived fuel. They are produced from household and industrial waste gases, using gasification and pyrolysis technology to turn them into fuel. Materials used to make RCFs usually are not renewable due to being made from fossil-derived waste or non-renewable sources, however, they redirect waste from incineration or landfill which has a positive end result. Non-recyclable wastes are ideal, however even without using these specific feedstocks RCFs still result in higher GHG savings than the alternative waste energy plants. On top of this the Advanced Fuels Fund (AFF) has made an investment of £165 million for these facilities and have confirmed funding for four UK plants which will use RCF source waste material to produce SAF [19].
Municipal Solid Waste (MSW)
The use of MSW as a feedstock can be challenging due to the variation in its composition and properties caused by the locations of which MSW is collected and sorted. The sorting and collecting of MSW is typically carried out partially by local councils themselves which can help in ensuring unsuitable materials such as glass and metals are removed before going through the conversion process [20]. After screening and separating recyclable materials, using MSW as a source of energy and feedstock adds value and reduces impacts and costs for handling and treatment, diverting MSW into SAF production also prevents the landfill GHG emissions that would otherwise occur [21].
Power to Liquid (PtL)
A type of SAF which does not rely on the use of waste derived feedstocks are PtL fuels. These are synthetically produced liquid hydrocarbons coming from renewable energy sources, water or carbon dioxide. They are very versatile and can be used to produce hydrogen and SAF as well as encouraging the use of carbon capture but they require a lot of energy and are inherently one of the most expensive production methods meaning the production of these fuels are currently limited [22].
Analysis
Fossil Jet Fuel vs. SAF
Emissions from aviation are growing faster than any other mode of transport, with the non-CO2 effects such as nitrous oxide (NOx) emissions contributing twice as much to global warming as CO2 itself [23]. The aviation industry as whole is responsible for about 4% of human-induced global warming, which demonstrates the importance of finding more sustainable ways of flying. In contrast with conventional jet fuel, SAF production processes and combustion typically result in very lowlevel sulfur and aromatic content [particulate matter (PM) emissions]. PM emissions are reduced by 50%–97% even when using fuel blended with SAF over conventional jet fuel alone, with the highest reductions occurring at low engine power, when the aircraft is being taxied. This means there would also be significant improvement to local air quality around airports [24].
Land Use
Biofuels produced from energy crops would require farmland or other facilities for growing and harvesting, this is known as direct land use change (DLUC) as the land is being directly repurposed. In accordance with the UK SAF mandate, biofuels which take land away from food production would not be supported, so this is unlikely to have a significant impact on the UK SAF industry as a whole. However, indirect or induced land use change (ILUC) which can occur when an increased demand for the biofuels causes more land to be needed for infrastructure, negatively impacts natural areas causing deforestation and CO2 to be released from the trees and soil. The effects of ILUC and DLUC are commonly included in life cycle assessments, except where the emissions from ILUC would offset carbon savings from using more sustainable fuels, in these cases further analysis takes place [25].
Long Term Sustainability
A life cycle assessment (LCA) covers all parts of the lifecycle of aviation fuels through feedstock recovery, transportation, fuel production and combustion, giving a comprehensive look at the potential environmental benefits the use of SAF could have [26]. The LCA or CO2 equivalent emissions are shown in more detail in CO2 Emissions section where the estimated CO2 equivalent emissions for varying flight lengths and fuel blends were calculated, these results can be found in Figures 7–9.
It is also important that there is a good enough supply of the feedstock readily available to support a growing fuel demand and a domestic UK SAF industry. Out of the four feedstocks discussed in this report, PtL is the only one which is not derived from waste relying instead on access to renewable energy sources. Waste derived feedstocks have got both a large quantity of resources as well as the added benefit of reducing the amount of solid waste ending up in landfills and waste gases released into the atmosphere [17]. In Figure 1 the estimated amount of feedstock likely to be available for SAF use in the next few years is shown.
Conversion Processes
The most common conversion methods for the four main types of SAF feedstock discussed throughout this report are described below and shown comprehensively in Table 1. The viability of these also depends on the location and transport required to move the feedstock to the conversion facility, for example, waste derived fuels have an abundance of resources available but these tend to be spread over a wide geographical area [20].
Thermal conversion processes such as gasification, pyrolysis and liquefaction tend to be the most common. In gasification the combustible parts of solid waste feedstocks are completely converted into a mixture of advanced biofuels and RCF, the resulting syngas can be used for high efficiency fuels and chemicals as substitutes for the fossil fuel alternative. In the case of pyrolysis and liquefaction, biofuels can be produced by liquefying fossil waste material which has been previously rejected from recycling plants or would otherwise be sent to landfill [28]. Advanced gasification is a popular conversion method due to its adaptability and the fact it does not require any further pollution or emissions treatment due to its non-combustible process, this makes it both the cleanest and most economically viable waste to energy technology currently available [32]. Out of these three thermal conversion methods, gasification also has the highest efficiency at 70%–80% [33].
Fischer-Tropsch (F-T) technology involves a chemical reaction process converting syngas (a mixture of hydrogen and carbon monoxide) into liquid hydrocarbons which can be used as synthetic biofuel alternatives for kerosene or gasoline [34]. In the case of PtL fuels, carbon capture and electrolysis are used to obtain the required hydrogen and carbon dioxide from renewable energy sources before fuel synthesis occurs [35]. F-T pathways tend to offer the best GHG reductions when compared to conventional jet fuels showing potential for over 84% less GHG emissions [36]. In comparison to thermal conversion processes F-T has a thermal efficiency of around 50% making it more similar to liquefaction and pyrolysis than gasification [37].
For HEFA fuels, a different process known as hydrogenation which uses hydrodeoxygenation (the removal of oxygen in the presence of hydrogen) and cracking to refine the vegetable oils, waste oils or fats into jet fuel standard biofuels. Bio jet fuels like HEFA which are produced using this method tend to have a more beneficial thermal stability and high paraffin content [31]. Hydrogenation has the highest efficiency out of the conversion processes discussed in this report at around 86% which is likely another reason as to why there is so much competition around HEFA fuels [38].
In comparison AVGAS is made from crude oil which is then desalinated, refined, alkylated and distilled, a colored dye is then added for identification purposes before the fuel is delivered to the airport [39, 40].
Energy Density
Energy density is an important factor when comparing and analyzing fuel types as those with a lower energy density would need to be used in a greater quantity in order to provide the same amount of energy, this can impact both the environmental and economic aspects relating to the type of fuel being used. In Figure 2 the calorific values for conventional fossil jet fuel and the four main SAF biofuels are compared. The jet fuel used in this analysis is aviation gasoline (AVGAS).
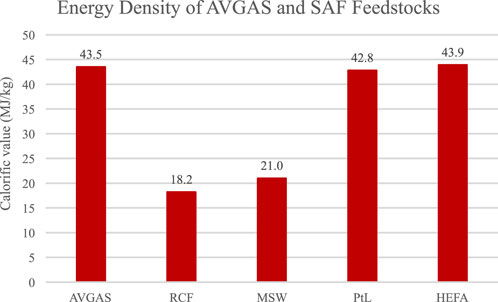
Figure 2. Energy density of AVGAS and feedstocks. Data: AVGAS [30], RCF [41], MSW [42], PtL [43], HEFA [44].
Combustion
The plane model used for short haul (SH) and medium haul (MH) flights was the Boeing 737 and the larger Boeing 747 was used for long haul (LH) flights, these were chosen as they are some of the most common plane models for these flight distances [27]. The aircraft data, seating capacity and fuel efficiency per seat can be found in Supplementary Table S2. To make the analysis more accurate example destinations were taken for SH, MH and LH with the departure airport as London Heathrow, the relative times and distances for these can be found in the Supplementary Material and were chosen based on the time and distance of the flight (SH < 3 h, MH 3–6 h, LH 6–12 h) [45]. The data for Equations 1–3 can be found in the Supplementary Material for this report this data includes the Boeing 737 seating capacity [27], fuel efficiency per seat SH and MH [46] and Boeing 747 seating capacity [27] and fuel efficiency per seat LH [47]. Figures 3–5 can also be found in the Supplementary Material and show the results from calculating the fuel required for each fuel blend using Equations 4–6 [48].
Equation 1 – Fuel consumption in liters, where ηs is the fuel efficiency per seat, d is the distance and SC is the seating capacity
Equation 2 – Fuel consumption in tons, where ρF is the relative fuel density (0.825 g/mL [49])
Equation 3 – Energy used during flight, where ρE is the fuel energy density (MJ/ton)
Equation 4 – Fuel required for flight in tons for 100% fuel blends, where ER is the required energy in MJ
Equation 5 – Fuel required for flight in tons for 50% fuel blends, where FRSAF is the amount of SAF fuel required and FRAV is the amount of AVGAS required
Equation 6 – Fuel required for flight in tons for 25% fuel blends
Emissions
CO2 Emissions
In the last 200 years alone, the CO2 content has risen a further 50% from human causes which has led to the more prominent global warming effects that can already be seen today such as more frequent extreme weather and rising sea levels [50]. The values for the AVGAS, HEFA [51], RCF [12], MSW [52] and PtL [43] emissions per gCO2e individually can be found in the Supplementary Material this data used to calculate the total CO2 emissions for the different flight lengths using Equations 7–9 with the results shown in Figures 7–9.
Equation 7 – Total emissions in gCO2e for 100% blends, where ED is the energy demand (MJ) and eF is the fuel emissions
Equation 8 – Total emissions in gCO2e for 50% blends, where eSAF is the emissions for SAF and eAV is the emissions for AVGAS
Equation 9 – Total emissions in gCO2e for 25% blends
Non-CO2 Emissions
Planes using conventional jet fuels emit other greenhouse gases aside from carbon dioxide, such as, nitrous oxides (NOx), sulfur oxides (SOx) and particulate matter (PM) which also contribute to global warming [53].
The effects of NOx gases [nitric oxide (NO) and nitrogen dioxide (NO2)] can be detrimental to both human/animal health and the environment. Exposure to NO2 can cause an increased risk of respiratory issues and vulnerability to allergens, as well as worsening symptoms for those who have existing health conditions. Excess nitrogen either as a gas or in precipitation can cause changes in soil chemistry and impact local biodiversity in more sensitive habitats [54]. Another aspect they can affect is the levels of ozone (O3) and methane (CH4) in the atmosphere which can impact the radiative balance of the Earth by altering the amount of the sun’s energy reaching and leaving the earth’s surface. However, aviation contributes to only about 2% of global NOx emissions with most NOx emissions coming from road traffic emissions. In this case higher efficiency engines can also help to reduce the amount of NOx emitted by aircraft during combustion [55].
Similarly, SOx emissions can affect the health of both humans/animals and the environment with potential to impact respiratory systems and worsen existing health conditions. The presence of high concentrations of sulfur dioxide (SO2) in the air can form other SOx compounds which cause significant PM pollution. With this considered, the environmental impact of these gases includes a decrease in visibility from hazes caused by particulates, damage and reduced growth in plants and trees, and acid rain which can be very harmful to certain ecosystems [56]. The level of SOx emissions produced during flight is directly dependent on how high the sulfur content of the fuel is, this is true for the majority of the different types of emissions produced by aircraft which ultimately result from the incomplete combustion of the fuel [57].
Some of the worst warming effects come from contrails caused by water vapor which make up approximately 30% of the exhaust [53]. Contrails themselves are not generally harmful and last only a short period of time, however, when conditions are cold enough and the resulting water droplets freeze causing contrail-induced cirrus clouds which reflect infrared rays resulting in at least twice the warming effect of CO2 alone [58, 59].
Economic Outlook
The aviation industry consumed roughly 500,000 tons of SAF in 2023 which doubled that of the previous year, this figure is predicted to triple again in 2024 to 1.5 million tons of SAF priced at $2,500 per ton, nearly three times more than the cost of conventional aviation fuel [60]. However, as the technology continues to develop and improve these prices are expected to fall to around $760$900 per ton by 2050 which would put it well within the price range for conventional jet fuel though it is likely to remain just above the average cost of fossil derived fuels.
From a worldwide perspective the SAF industry is likely to require at least 5,000 new refineries by 2050 to keep up with the expected increase in aviation fuel demand (both conventional and SAF), this could require nearly $1,500 billion total capital expenditure. This is still less than the historical capital expenditure used for oil and gas facilities, costs going forward are predicted to drop when the infrastructure is in place, the industry would also provide and sustain over 13 million jobs worldwide and improve local energy security for many countries due to the widespread nature of the feedstock and energy production [61].
Levelized Cost of Energy (LCOE)
The levelized cost of energy considers the cost in terms of the energy produced over the lifetime of the biofuel [62]. It also allows the viability of a project to be assessed on an even level, in this case a lower LCOE value is more advantageous. As SAF is currently being mostly used as a drop-in fuel within the UK different blends and flight lengths will be looked at, this is also helpful in seeing how the costs could change for the aviation industry itself. The costs per liter of each fuel is shown in the Supplementary Material, this data is used to calculate the total fuel costs using Equations 11–13 with the results shown in the Supplementary Material based on the fuel requirement calculations done using Equation 10 [62–65]. This information was then used to complete the LCOE calculations using Equation 14.
The total fuel required was calculated based on the projected energy demand found using the information in Combustion section with Equation 3, for short haul flights the value was 274963.5MJ, this value was used alongside the cost in the Supplementary Material to calculate the LCOE shown in Figure 6.
The same method used to determine the energy and therefore the fuel needed for short haul flights was also used for medium haul, this time with a value of 774561MJ being used for the corresponding LCOE calculations and data. Due to the same aircraft being used for the SH and MH calculations, the trends and results for the LCOE as shown in Figures 6, 7 are very similar.
For the long haul flights an energy value of 5510971.5MJ was used, the overall LCOE results can be seen in Figure 8.
Equation 10 – Fuel required during flight in liters
Equation 11 – Total fuel cost in £ for 100% blends, where CF is the fuel cost in £/l
Equation 12 – Total fuel cost in £ for 50% blends, where CSAF is the cost of the SAF fuel and CAV is the cost of the AVGAS
Equation 13 – Total fuel cost in £ for 25% blends
Equation 14 – Levelized cost of energy, where EP is the energy produced
Fuel Blending and Existing Facilities
Due to the strict standards associated with the aircraft fuel, SAF would most likely need to be blended and certified before reaching the airport through pipelines, if this involves SAF produced at biofuel facilities the fuels can be blended on site using existing infrastructure [66]. Should the fuel be delivered to the airport pre-blended no new infrastructure would be required at the airport itself but, depending on the type of SAF being used and the availability of its conversion facilities, new infrastructure may be required for procuring, transporting and mixing the fuels [67].
In terms of the facilities required to produce SAF the costs are estimated by ICAO to be around $48billion a year over the next 30 years, in comparison to the capital costs associated with oil and gas production in the last decade ($420billion a year) this is drastically lower but will still make up a significant proportion of the incremental costs associated with achieving net zero [68]. For meeting the 2030 goals many new conversion technologies and facilities need to be properly commercialized and funded in order to meet the projected demand, for this to work further external support is likely to be needed. The high capital costs, uncertainty and complexity related to this means this support is unlikely to be found before more information and policy support is in place [69].
It is currently estimated that a further 14 SAF production facilities will be developed by 2035 within the UK, these new facilities are projected to support over 3,500 jobs and add £2.7billion gross value (GVA) to the UK economy [70]. With the help of the Advanced Fuel Fund (AFF) supporting 15 new SAF company projects, it is estimated that should they all be completed and operational on schedule, approximately 702.4 kt/year of different aviation biofuels could be produced annually by 2030 [70].
Carbon Offsetting and Subsidies
One part of the push towards more sustainable flying is the use of Carbon Offsetting schemes which are already in place. These schemes entail selecting the “carbon offset” option when booking flights, this gives financial support to projects or companies dedicated to preventing further, or removing existing CO2 from the atmosphere [71].
Due to the high costs associated with using newer fuels a variety of different incentives are being introduced to encourage an increase in support and use of SAF. An example of this is the scheme currently in place at Heathrow Airport where the company pays half the difference between conventional aviation fuel and SAF for airlines using blended fuels; this has already shown success with an increased use of SAF in the airport. With an additional £71 million set aside for this initiative in 2024, the use of SAF is predicted to increase from 1.5% in 2023 to 2.5% in 2024 [72].
From a broader perspective, the UK government is planning to introduce an industry funded revenue certainty system in order to support the production of SAF within the UK, including the £165 million from the AFF already in place to lower the risk of, and encourage further investment in, the development of a local SAF industry [73]. Objectively, the AFF is aimed at supporting the UK advanced fuels sector and the advancement of related technologies which will assist in reducing aviation emissions in the near future, the timeline given for this funding project ranges from July 2022 to the end of March 2025, which would be a positive sign leading up to the implementation of the UK SAF mandate that year [74].
The UK Emissions trading scheme (ETS) came into effect in January 2021 in order to take the place of the EU ETS when the UK left the European Union. These schemes work on a cap and trade basis where the government limits the amount of emissions allowed and creates permits for each unit of emissions allowed. Companies involved can either reduce emissions or trade with other companies if they believe the number of permits they have are not enough, some permits can be given freely by the government or sold at auction [75]. This new version of the scheme is very similar to the original but considers the relevant CORSIA guidelines to allow the same methods to be used for both and simplify the system for those affected by it.
Methodology and Design
Having analyzed the different feedstocks and blends of SAF from numerous perspectives, a mathematical algorithm will be designed to quantify the results and provide an answer to the original question (which feedstock would be the most feasible). To do this several factors will be identified from the prior research which will cover both the technical and feasibility aspects of the SAF industry and be ranked in order of importance from one to five with one being the most important. The feedstocks will then be weighed against the best feedstock result for each factor forming an equation. Where the fuel blends, not just the feedstock are involved, an average result will be used.
Technical Factors
Emissions
This area will look at both the reduction in emissions from the feedstock in comparison to conventional aviation fuel and the CO2 emissions of the feedstock itself compared with other SAF blends (using the gCO2e calculations). This covers the LCA values for the fuels as well. Due to the environmental benefits being the main goal of the UK SAF mandate and reason behind the increased use and production of SAF it was ranked the highest out of the five selected factors [76, 77].
Ranking- 1
Calorific Value
The energy density of the fuels used is important as in order for any type of fuel to be a viable option it needs to be able to supply the required energy in both an economically and environmentally sustainable manner. Unfortunately, these things very rarely line up with the more environmentally advantageous option being more expensive or vice versa. In this context a fuel which is both economically and environmentally effective but has a low energy density would not be the best choice for aviation use. This factor was given the lowest ranking as it has a lesser impact when used as a drop in or blended fuel, and as the feedstocks used for SAF have to meet the standards all the feedstocks are viable even if those with a higher energy density or calorific value may be more efficient [78].
Ranking- 5
LCOE
Another important factor to consider is the LCOE as it gives a clear overview of the economic impacts for the different fuels. By using this method in particular the costs of the fuels can be assessed based on the energy they produce making for a fairer comparison overall. With incentives and carbon offsetting schemes some of the initial price increases that airports and airlines are likely to see when switching to SAF blended fuel can be lessened, and this will continue as the industry grows and gains a more solid footing [79]. This factor was ranked second as the cost of SAF will have a large impact on how willing companies are to invest and switch to using blended fuels. In order for SAF to work as a long-term solution and industry it cannot be reliant on grants and incentives forever and therefore the fuels and feedstocks need to be economically sustainable throughout their use [80].
Ranking- 2
Feasibility Factors
Production Capabilities
For the SAF industry to grow the necessary production facilities need to be available to support it and meet the increasing demand. This will require an influx of new facilities alongside the use of existing or repurposed facilities, due to the varying conversion and production methods used for different SAF types, it is likely that some fuels will be easier to produce in larger quantities sooner where there are more existing facilities rather than those that require newer infrastructure. This factor was ranked fourth, though it was on similar standing with the availability of resources due to both needing to be able to meet the growing demands, conversion and production facilities are currently available and plans are already in place for new and repurposed plants to be completed in the coming years [81].
Ranking- 4
Availability of Resources
In order for a feedstock to be used in the increased production of SAF the availability of the feedstock itself is a major factor in its sustainability and feasibility. Fuels derived from waste will generally have an abundance of resources available but may also have more competition from other sectors looking to use more sustainable fuels in transport, whereas fuels relying on newer less developed technology like PtL may struggle more initially to build a steady supply of available fuel [82]. For ranking, this factor was in the middle, as it is important that it is possible for the feedstock to meet the growing demand and keep up with it long term, if this is not the case then it would be unsuitable and unsustainable for this application.
Ranking- 3
Finalized Equation
The most viable feedstock will be the one with the lowest result from this algorithm. The first stage involves determining the feedstocks weighting for each factor, this is done by using the best result in the data sets (the lowest for emissions and LCOE and the highest for availability, production and calorific value) to calculate how many times bigger/smaller each other feedstock was and dividing this by the factors rank to give a weighted result. For the emissions and LCOE where the feedstocks were looked at in terms of fuel blends the weighted results were then averaged before being put through the final equation to get the feasibility results for each feedstock. The weightings will be done based on the calculations completed and data collected in Analysis section, Figures 1, 3, 6 and Table 1 and Figure 2 for emissions, LCOE, production capabilities, resources availability and calorific value respectively.
Equation 15 – Weighted algorithm results, where SAFB is the best feedstock value for each factor, SAF is the given feedstock and r is the rank of the given factor
Equation 16 – Averaged weighted results for algorithm
Equation 17 – Feedstock feasibility, where ARe is the average emissions result, ARLCOE is the average LCOE result, WRA is the weighted availability result, WRPR is the weighted production result and WRCV is the weighted calorific value result
Implementation of Methodology
The following figures show the results of the feasibility algorithm explained in Methodology and Design section, Equations 15, 16 were used for the emissions and LCOE results in Figures 9, 10 and Equation 15 was used for the availability, production and calorific value results shown in Figures 11–13. The overall feasibility results are shown in Figure 14 with the best feedstock (the lowest result) highlighted in bold, these results were calculated using Equation 17.
For the emissions MSW had the best results with PtL and HEFA having around double the amount in comparison. This is largely due to the effectiveness of redirecting waste to use as fuel and the positive impact this has on the LCA data.
Contrary to the previous figure, in terms of cost PtL has the best result and MSW the worst, this difference is largely because of the higher complexity in gathering and processing resources for MSW production.
In terms of availability PtL and HEFA score significantly worse mainly due to competition from other industries for either the renewable energy or waste oils used to produce the fuels, as RCF and MSW are both waste derived fuels, there is a higher abundance of feedstock available.
RCF and MSW came out better in terms of production due to the wider variety of production methods available for them making them more versatile in the types of facilities they would need to be produced.
The energy density of PtL and HEFA are generally better and closer to that of standard AVGAS than the waste derived feedstocks due to them being more uniform in their content.
The final results show MSW as the most feasible feedstock for the UK SAF industry, these results are discussed in detail in the following Implementation of Methodology section.
Project Evaluation
Discussion of Results
From the mathematical algorithm used in Methodology and Design section, the most feasible feedstock for meeting the UK’s 2030 SAF mandate is MSW as it is widely available and has the lowest combustion emissions and LCA value out of the four feedstocks considered in this report. The use of MSW sourced fuels has the additional benefit of lessening the quantity of waste ending up in landfills, effecting and lowering the environmental impact of the waste disposal industry as well as the aviation industry alone. The effects of this can be seen even before it reaches landfills or conversion facilities as the diversion of selected non-recyclable MSW into energy production reduces the pressure and costs on the waste disposal industry due to lowering the amount of waste going through the handling and treatment processes.
Using MSW does however pose a series of challenges: it has the highest LCOE and a low energy density, meaning it costs more to produce the same amount of energy than it does in comparison to AVGAS or other SAF blends, making it less appealing to potential investors. Other concerns would be the inconsistency of the waste being used for feedstock, the types of materials in the waste used will not be the same for every batch of fuel and the conversion methods used to produce this fuel tend to be more expensive. These risks can be reduced with the use of proper quality control which would be required for any aviation grade fuel, and concerns over the viability of F-T conversion methods due to its cost is generally balanced by the high level of reduced GHG emissions it provides.
A similar trend of benefits and challenges can be observed with the other feedstocks as well. For example, HEFA has the highest energy density and ranks second for both LCOE and production capabilities but faces a lot of competition from other industries leaving a relatively small amount available for use in the aviation industry and will likely be subject to limitations from the proposed HEFA cap to prevent the UK SAF industry becoming overly reliant on a singular feedstock. In the case of RCF, it has similar benefits to MSW in terms of repurposing waste and the biggest variation in conversion methods making its production much more flexible, but has a lower energy density and higher emissions in comparison. PtL fuels stand out being the only feedstock out of the four which is not waste derived, although being produced from renewable energy sources makes it a newer and more innovative option, the technology itself is likely to need more development to lower the costs of fuel production. As PtL fuels are not waste derived, the emissions and LCA values are higher but its LCOE is better due to a higher energy density and the benefit of not having to rely on a specific feedstock source for production.
Sensitivity Analysis
The lowest ranked factor in the algorithm was the calorific value or energy density of the fuels, largely because each of the feedstocks are capable of being used in aviation and using SAF as drop-in fuels minimizes the impact it has overall. However, with RCF and MSW having such significantly lower calorific values they may still be less suitable for longer haul flights. This is due to the extra fuel that would be required for the flights to still meet the energy demand of the aircraft and the likelihood that this would not be fully mitigated by blending the fuels.
Put into the context of this project, if the calorific was to be given a higher ranking within the algorithm itself the final results would likely favor PtL and HEFA over RCF and MSW as these feedstocks have energy densities only fractionally different from AVGAS and therefore lower risks and any additional fuel requirements would be minimal. With this taken into account, despite restrictions on availability and production capabilities for these feedstocks, they may be more suited to longer haul flights than RCF and MSW derived SAF. Should a variety of different feedstocks be used in the building of the UK’s SAF industry, prioritizing limited PtL or HEFA fuels for use in longer haul flights and RCF and MSW fuels for shorter haul flights would be beneficial.
Benefits and Risks
The main benefit of using SAF over other decarbonization methods is that these biofuels can be used in existing aircraft, infrastructure and blended with conventional jet fuel. This means that progress can be made without relying on new aircraft designs and construction which would be necessary for electric or hydrogen-based prototypes. However, the sources of SAF come with their own benefits with the use and repurposing of used oils, non-recyclable waste and other residues which would otherwise end up in landfills or going through expensive treatment processes. Using SAF under the UK mandate ensures that the lifecycle emissions of the fuels must be reduced by at least 70% in comparison to fossil jet fuel whilst avoiding the use of energy crops as a feedstock to limit the impact on food production and land use.
In regards to long term feasibility, producing SAF from waste derived fuels tends to be more reliable due to the quantity of waste both available and being produced at any given time. With improvements to pretreatment and sorting techniques surrounding waste collection and distribution, the viability of feedstocks like MSW would increase and the costs associated with them would likely decrease alongside them. Over time there is also the possibility that restrictions in place for HEFA and PtL use in the aviation industry change or decrease as more feedstock becomes available and production methods improve.
Despite this there are still risks associated with the use of SAF including the cost of the fuels, new facilities and the pressure to keep up with the growing demand going forwards. Though there have already been massive investments into the industry alongside incentives to encourage more investors to get involved but this alone would not be sustainable long term. To meet the demand as it continues to increase both for conventional jet fuel and SAF itself multiple reliable feedstock sources and production facilities will need to be in place, though there are multiple new facilities planned and under construction alongside several existing facilities being repurposed, whether they will be operational in time to meet the 10% SAF by 2030 goal remains to be seen.
Blends and Flight Lengths
Other aspects assessed within this report were how different blends of fuel and lengths of flight may affect the viability and support of SAF as an option for decarbonizing the aviation industry. By using SAF as a drop-in fuel, more time is given to both investors and producers to have a clearer understanding of the impact that using cleaner fuels can have whilst diminishing the immediate need for a high and potentially unreachable demand, the costs associated with SAF are also likely to decrease overtime making a gradual introduction of alternative fuels more beneficial. Though evidence suggests that biofuels like SAF are likely to improve the life of aircraft engines rather than cause any increase in corrosion or damage, it will also give more time for proper assessments to be made for the overall safety and secondary benefits that may be seen. However, to reach 10% SAF by 2030 in the UK, more may need to be done to ensure the mandate goals are met as well as the overall Net Zero aims.
Looking at different lengths of flights was an important aspect due to the difference in demands and results from both the aircraft and fuel when considering a domestic 1-hour flight in comparison to an international 12-hour flight. For example, a 12-hour flight with 600 passengers would have a better fuel efficiency per seat compared to a 3-hour flight with 200 passengers even though the flight is longer, in accordance with this, the emissions per passenger mile can therefore be less for a jumbo jet aircraft carrying 600 people than a car transporting only one person. Short local flights done by private jets with only a few passengers can be an exception to this where many of these journeys could have been completed using a more suitable mode of transport for the number of passengers. For these reasons and to give a more in-depth analysis considering different aircraft models multiple different flight lengths were looked into.
Another factor that works alongside SAF and other sustainability measures is the improved efficiency of modern aircraft. As the overall performance and efficiency of aircraft continue to improve over time the amount of fuel burned during flights is reduced and used more effectively. This not only assists in meeting the overall 2050 Net Zero goals but benefits the whole aviation industry and further encourages the growing uptake of SAF.
Conclusion and Future Work
Having completed extensive research on UK SAF feedstocks and conducting environmental and economic analysis to form a mathematical algorithm, the feedstock likely to make the UK’s SAF goals most feasible was municipal solid waste (MSW) which repurposes everyday household waste into fuel. This is due to it being a widely available resource and having the most significant reduction in CO2 emissions when compared to the other feedstock analyzed within this report. It is however, unlikely that one feedstock alone will be able to produce enough of the biofuel to meet the 10% SAF by 2030 goals meaning that a mixture of the feedstocks are still likely to be needed alongside MSW derived fuels in order to keep up with the growing aviation fuel demand.
Should this project be continued or undertaken again in the future it could be improved by using more visual elements and variables. For example, having a wider variety of factors taken into account alongside the fuel blends and flight lengths. A more in depth look at the economic analysis could include factoring in the capital and operational expenditure for the LCOE and more information on quantities of non-CO2 emissions would add to the environmental analysis. More in depth modelling and simulations could also add more value, especially if multiple different flight paths were considered instead of just one for each short, medium and long haul.
Data Availability Statement
The original contributions presented in the study are included in the article/Supplementary Material, further inquiries can be directed to the corresponding author.
Author Contributions
RC wrote the manuscript with AH as supervisor and editor. All authors contributed to the article and approved the submitted version.
Funding
The author(s) declare that no financial support was received for the research, authorship, and/or publication of this article.
Conflict of Interest
The authors declare that the research was conducted in the absence of any commercial or financial relationships that could be construed as a potential conflict of interest.
Generative AI Statement
The author(s) declare that no Generative AI was used in the creation of this manuscript.
Supplementary Material
The Supplementary Material for this article can be found online at: https://www.frontierspartnerships.org/articles/10.3389/arc.2024.14015/full#supplementary-material
Abbreviations
AFF, Advanced Fuel Fund; Avtur, Aviation Turbine Fuel; AVGAS, Aviation Gasoline; CORSIA, Carbon Offsetting and Reduction Scheme for International Aviation; DLUC, Direct Land Use Change; ETS, Emission Trading Scheme; F-T, Fischer-Tropsch; gCO2e, Grams CO2 equivalent; GHG, Greenhouse Gases; GVA, Gross Value Added; HEFA, Hydroprocessed Esters and Fatty Acids; ICAO, International Civil Aviation Organization; ILUC, Indirect/Induced Land Use Change; LCA, Life Cycle Assessment; LCOE, Levelized Cost of Energy; LH, Long Haul; MH, Medium Haul; MSW, Municipal Solid Waste; PM, Particulate Matter; PtL, Power to Liquid; RCF, Recycled Carbon Fuels; RTFO, Renewable Transport Fuel Obligation; SAF, Sustainable Aviation Fuel; SH, Short Haul.
References
1. Department for Transport. Pathway to Net Zero Aviation: Developing the UK Sustainable Aviation Fuel Mandate. GOV.UK (2024). Available from: https://www.gov.uk/government/consultations/pathway-to-net-zero-aviation-developing-theuk-sustainable-aviation-fuel-mandate (Accessed January, 2024).
2. ReFuelEU Aviation. Mobility and Transport. Available from: https://transport.ec.europa.eu/transport-modes/air/environment/refueleu-aviation_en (Accessed January, 2024).
3. International Civil Aviation Organization. Global Aviation and Our Sustainable Future (2012). Available from: https://www.icao.int/environmentalprotection/documents/rio+20_booklet.pdf (Accessed January, 2024).
4. Alrebei, OF. Aircraft Performance of a Novel SAF: Lower Costs, Lower Environmental Impact, and Higher Aircraft Performance. Energy Convers Manag X.24. Available from: https://www.sciencedirect.com/science/article/pii/S2590174524002174#:∼:text=Specifically%2C%20SAF%20exhibited%20a%2017,also%20exhibited%20superior%20aircraft%20performance (Accessed January, 2024).
5. Sustainable Aviation. Sustainable Aviation Fuels Road-Map. Available from: https://www.sustainableaviation.co.uk/wp-content/uploads/2020/02/SustainableAviation_FuelReport_20200231.pdf (Accessed January, 2024).
6. Airbus. Sustainable Aviation Fuels (2024). Available from: https://www.airbus.com/en/innovation/energy-transition/sustainable-aviation-fuels (Accessed January, 2024).
7. Aviation Benefits Without Borders. Aviation Industry Reducing Its Environmental Impact (2023). Available from: https://aviationbenefits.org/environmentalefficiency/climate-action/#:∼:text=Sustainable%20aviation%20fuels%20have%20great,systems%20or%20engines%20of%20aircraft (Accessed January, 2024).
8. ICAO. Guidance on Potential Policies and Coordinated Approaches for the Deployment of SAF (2022). Available from: https://www.icao.int/environmentalprotection/Pages/saf_guidance_potential_policies.aspx (Accessed January, 2024).
9. GOV.UK. UK Emissions Trading Scheme Markets (2024). Available from: https://www.gov.uk/government/publications/uk-emissions-trading-scheme-markets/ukemissions-trading-scheme-markets (Accessed January, 2024).
10. Flightworx, F. UK Government's £53 Million Injection Into SAF: Advancing Green Aviation. Flightworx (2023). Available from: https://www.flightworx.aero/blog/uk-governments-53-million-injection-into-saf-advancinggreen-aviation/ (Accessed January, 2024).
11. International Civil Aviation Organization. CORSIA Sustainability Criteria for CORSIA Eligible Fuels (2021). Available from: https://www.icao.int/environmental-protection/CORSIA/Documents/ICAO%20document%2005%20%20Sustainability%20Criteria%20-%20November%202021.pdf (Accessed January, 2024).
12. Department for Transport. Supporting Recycled Carbon Fuels Through the Renewable Transport Fuel Obligation. GOV.UK (2024). Available from: https://www.gov.uk/government/consultations/supporting-recycled-carbon-fuels-throughthe-renewable-transport-fuel-obligation (Accessed January, 2024).
13. GOV.UK. Transport and Environment Statistics (2023). Available from: https://www.gov.uk/government/statistics/transport-and-environment-statistics-2023/transport-and-environment-statistics-2023 (Accessed January, 2024).
14. International Civil Aviation Organization. Sustainable Aviation Fuels Guide (2018). Available from: https://www.icao.int/environmentalprotection/SAF/Documents/SAF%20User%20Guide%20Edition%20II.pdf (Accessed January, 2024).
15. House of Commons. Net Zero and the UK Aviation Sector (2023). Available from: https://committees.parliament.uk/publications/42703/documents/212154/default/ (Accessed January, 2024).
16. Austin, BK. Groundbreaking Transatlantic Flight Using Greener Fuel Lands in the US (2023). Available from: https://www.bbc.com/news/business-67548961 (Accessed January, 2024).
17. International Council on Clean Transportation. Estimating Sustainable Aviation Fuel Feedstock Availability to Meet Growing European Union Demand (2021). Available from: https://theicct.org/publication/estimating-sustainable-aviation-fuel-feedstock-availability-tomeet-growing-european-union-demand/ (Accessed January, 2024).
18. Carroll, SG, and Carroll, SG. UK Suggests Restricting Green Jet Fuel From Waste Cooking Oil (2024). Available from: https://www.euractiv.com/section/aviation/news/uk-suggests-restricting-green-jet-fuel-fromwaste-cooking-oil/ (Accessed January, 2024).
19. GOV.UK. Energy Security Bill Factsheet: Recycled Carbon Fuels and Nuclear Derived Fuels for Transport (2023). Available from: https://www.gov.uk/government/publications/energy-security-bill-factsheets/energysecurity-bill-factsheet-recycled-carbon-fuels-and-nuclear-derived-fuels-for-transport (Accessed January, 2024).
20. Sanchez, NM, Link, F, Chauhan, G, Halmenschlager, CM, El-Sayed, HEM, Sehdev, R, et al. Conversion of Waste to Sustainable Aviation Fuel via Fischer-Tropsch Synthesis: Front End Design Decisions. Energy Sci Eng (2022) 10(5):1763–89. doi:10.1002/ese3.1072
21. King County and Port of Seattle. Municipal Solid Waste-to-Fuels Study Summary (2023). Available from: https://your.kingcounty.gov/dnrp/library/solid-waste/Solid-waste-planningmonitoring/Solid-waste-monitoring/MSW-fuels-study-summary.pdf (Accessed February, 2024).
22. German Environment Agency. Power to Liquid (PTL) for Aviation Initiatives and Projects. Int Civil Aviation Organ (2016). Available from: https://www.icao.int/environmental-protection/GFAAF/Pages/Project.aspx?ProjectID=46 (Accessed February, 2024).
23. Environment and Transport. New Technologies. Brussels: Transport and Environment (2024). Available from: https://www.transportenvironment.org/challenges/planes/airplanepollution/#:∼:text=Emissions%20from%20aviation%20are%20a,altitude%20at%20which%20aircraft%20operate%20 (Accessed February, 2024).
24. Owen, B, Lee, DS, and Lim, L. Flying Into the Future: Aviation Emissions Scenarios to 2050. Environ Sci and Technol (2010) 44(7):2255–60. doi:10.1021/es902530z
25. Piris-Cabezas, P. Environmental Defense Fund, Microsoft Corporation. The HighIntegrity Sustainable Aviation Fuels Handbook. Available from: https://www.edf.org/sites/default/files/2022-08/EDF%20HIGH-INTEGRITY%20SAF%20HANDBOOK.pdf (Accessed February, 2024).
26. EASA Eco. How Sustainable Are SAF? Germany: EASA Eco. Available from: https://www.easa.europa.eu/eco/eaer/topics/sustainable-aviation-fuels/how-sustainable-aresaf (Accessed February, 2024).
27. Franklin, Z. The Most Common Airplane Types: An Exploration Across Various Aircraft Categories. United States: EntireFlight LLC (2023). Available from: https://www.entireflight.com/blogs/aviation-is-a-lifestyle/most-common-airplanetypes#:∼:text=The%20737%20is%20known%20for,in%20a%20variety%20of%20models (Accessed February, 2024).
28. ARTFuels. Statement on the Use of Recycled Carbon Fuels at Member State Level (2020). Available from: https://artfuelsforum.eu/outputs/26-05-2020statement-on-the-use-of-recycled-carbon-fuels-at-member-state-level/ (Accessed February, 2024).
29. Sipra, AT, Gao, N, and Sarwar, H. Municipal Solid Waste (MSW) Pyrolysis for Biofuel Production: A Review of Effects of MSW Components and Catalysts. Fuel Process Technol (2018) 175:131–47. doi:10.1016/j.fuproc.2018.02.012
30. Air Bp. How All Sustainable Aviation Fuel (SAF) Feedstocks and Production Technologies Can Play a Role in Decarbonising Aviation. News and Views (2024). Available from: https://www.bp.com/en/global/air-bp/news-and-views/views/how_all_sustainable_aviation_fuel_SAF_feedstocks_and_production_technologies_can_play_a_role_in_decarbonising_aviation.html (Accessed March, 2024).
31. Monteiro, RRC, Santos, Ia. D, Arcanjo, MRA, Cavalcante, CL, De Luna, FMT, Fernandez-Lafuente, R, et al. Production of Jet Biofuels by Catalytic Hydroprocessing of Esters and Fatty Acids: A Review. Catalysts (2022) 12(2):237. doi:10.3390/catal12020237
32. Brunner, PH, and Morf, LS. Waste to Energy, Indispensable Cornerstone for Circular Economy: A Mini-Review. Waste Manage and Res J a Sustain Circular Economy (2024) 43:26–38. doi:10.1177/0734242x241227376
33. Babu, S. Thermal Gasification of Biomass. Available from: https://www.ieabioenergy.com/wp-content/uploads/2013/10/18_AR1999Task20colour.pdf (Accessed March, 2024).
34. Höök, M, Fantazzini, D, Angelantoni, A, and Snowden, S. Hydrocarbon Liquefaction: Viability as a Peak Oil Mitigation Strategy (2013). Available from: https://papers.ssrn.com/sol3/papers.cfm?abstract_id=2264419 (Accessed March, 2024).
35. Marsh, J. Power-to-Liquid Fuel for Sustainable Aviation. Sustain Rev (2023). Available from: https://sustainablereview.com/power-to-liquid-fuel-for-sustainableaviation/#:∼:text=Power%2Dto%2Dliquid%20fuel%20is%20a%20type%20of%20Sustainable%20Aviation,it%20through%20a%20refinement%20process (Accessed March, 2024).
36. De Jong, S, Antonissen, K, Hoefnagels, R, Lonza, L, Wang, M, Faaij, A, et al. Life-Cycle Analysis of Greenhouse Gas Emissions From Renewable Jet Fuel Production. Biotechnol Biofuels (2017) 10(1):64. doi:10.1186/s13068-017-0739-7 (Accessed March, 2024).
37. Kamkeng, A, and Wang, M. Technical Analysis of the Modified Fischer-Tropsch Synthesis Process for Direct CO2 Conversion Into Gasoline Fuel: Performance Improvement via Ex-Situ Water Removal. United Kingdom: University of Sheffield. Available from: https://eprints.whiterose.ac.uk/197558/7/1-s2.0-S1385894723007799-main.pdf (Accessed March, 2024).
38. He, Y, Liu, S, Fu, W, Chen, J, Zhai, Y, Bi, X, et al. Assessing the Efficiency of CO2 Hydrogenation for Emission Reduction: Simulating Ethanol Synthesis Process as a Case Study. Chem Eng Res Des (2023) 195:106–15. doi:10.1016/j.cherd.2023.05.043
39. Avgas, Air bp. Aviation Fuel. Available from: https://www.bp.com/en/global/air-bp/aviation-fuel/avgas.html (Accessed April, 2024).
40. Tamalouzt, S, Benyahia, N, Tounzi, A, and Bousbaine, A. Renewable Energy - Resources, Challenges and Applications. IntechOpen eBooks (2020). Available from: https://www.intechopen.com/books/8871 (Accessed April, 2024).
41. Department for Transport, NNFCC. Annex B: Supporting Recycled Carbon Fuels Through the Renewable Transport Fuel Obligation. United Kingdom: Department for Transport (2022). Available from: https://assets.publishing.service.gov.uk/government/uploads/system/uploads/attachment_data/file/1097040/annex-b-supporting-recycled-carbon-fuels-through-rtfo-summary-ofanalysis.pdf (Accessed April, 2024).
42. Forest Research. Typical Calorific Values of Fuels - Forest Research (2022). Available from: https://www.forestresearch.gov.uk/tools-and-resources/fthr/biomass-energyresources/reference-biomass/facts-figures/typical-calorific-values-of-fuels/ (Accessed April, 2024).
43. Rojas-Michaga, MF, Michailos, S, Cardozo, E, Akram, M, Hughes, KJ, Ingham, D, et al. Sustainable Aviation Fuel (SAF) Production Through Power to Liquid (PTL): A Combined Techno-Economic and Life Cycle Assessment. Energy Convers Manage (2023) 292:117427. doi:10.1016/j.enconman.2023.117427
44. Boehm, R. Lower Heating Value of Jet Fuel From Hydrocarbon Class Concentration Data and Thermo-Chemical Reference Data: An Uncertainty Quantification. Energy Convers Manage 292. Available from: https://www.sciencedirect.com/science/article/pii/S001623612102411X (Accessed May, 2024).
45. Moffitt, M. What Is Considered a Short-Haul, Medium-Haul and Long-Haul Flight? Point Hacks (2022). Available from: https://www.pointhacks.com.au/differencesshort-medium-long-haul-flights/ (Accessed May, 2024).
46. Brady, C. Boeing 737 Performance Summary. Wayback Machine. Available from: https://archive.org/details/boeing737technic0000chri (Accessed May, 2024).
47. MediaRoom. Boeing Achieves Power on for New 747-8 Intercontinental (2010). Available from: https://boeing.mediaroom.com/2010-11-05-Boeing-Achieves-PowerOn-for-New-747-8-Intercontinental (Accessed May, 2024).
48. Distance to flights. Available from: https://www.distance.to/Heathrow,Hillingdon,Greater-London,England,GBR (Accessed May, 2024).
49. Stanhope-Seta. Jet Fuel Hydrometer (2024). Available from: https://www.stanhope-seta.co.uk/product/hydrometer-jet-fuel/ (Accessed May, 2024).
50. NASA Global Climate Change. Carbon Dioxide Concentration. United States: NASA Global Climate Change. Climate Change: Vital Signs of the Planet. Available from: https://climate.nasa.gov/vital-signs/carbon-dioxide/?intent=121#:∼:text=Key%20Takeaway%3A,in%20less%20than%20200%20years (Accessed May, 2024).
51. Ure, A, Martin, C, Campbell, M, Lokesh, K, McNaught, C, Twisse, F, et al. Low-Carbon Transport Fuels – An Evidence Review (2022). Available from: https://www.climatexchange.org.uk/wp-content/uploads/2023/10/cxc-low-carbon-transportfuels-an-evidence-review-oct-2023.pdf (Accessed May, 2024).
52. International Civil Aviation Organization. CORSIA Default Life Cycle Emissions Values for CORSIA Eligible Fuels (2021). Available from: https://www.icao.int/environmental-protection/CORSIA/Documents/ICAO%20document%2006%20-%20Default%20Life%20Cycle%20Emissions%20-%20March%202021.pdf (Accessed May, 2024).
53. Environmental and Energy Study Institute (EESI). Issue Brief | the Growth in Greenhouse Gas Emissions From Commercial Aviation (2019, Updated 2022) | White Papers | EESI (2022). Available from: https://www.eesi.org/papers/view/fact-sheet-the-growthin-greenhouse-gas-emissions-from-commercial-aviation (Accessed May, 2024).
54. GOV.UK. Missions of Air Pollutants in the UK – Nitrogen Oxides (NOx) (2024). Available from: https://www.gov.uk/government/statistics/emissions-of-airpollutants/emissions-of-air-pollutants-in-the-uk-nitrogen-oxides-nox (Accessed May, 2024).
55. Abel, S. Encyclopedia of Soils in the Environment (2023). Available from: https://www.sciencedirect.com/referencework/9780123485304/encyclopedia-of-soils-in-theenvironment (Accessed May, 2024).
56. US EPA. Sulfur Dioxide Basics (2024). Available from: https://www.epa.gov/so2-pollution/sulfur-dioxide-basics (Accessed May, 2024).
57. Eurocontrol Supporting European Aviation. Advanced Emission Model. Available from: https://www.eurocontrol.int/model/advanced-emission-model#:∼:text=The%20SOX%20emissions%20depend%20directly,unburnt%20hydrocarbons%20(HC)%20produced (Accessed May, 2024).
58. Transport and Environment. Airline Contrails Warm the Planet Twice as Much as CO2, EU Study Finds (2024). Available from: https://www.transportenvironment.org/articles/airline-contrails-warm-planet-twice-muchco2-eu-study-finds (Accessed May, 2024).
59. Kärcher, B. The Importance of Contrail Ice Formation for Mitigating the Climate Impact of Aviation. JGR Atmospheres (2016) 121(7):3497–505. doi:10.1002/2015jd024696
60. GreenAir News. IATA Expects SAF Production to Rise to 0.5% of Airlines’ Fuel Consumption in 2024, Adding $2.4bn to Costs (2024). Available from: https://www.greenairnews.com/?p=5103#:∼:text=IATA%20estimates%20the%20industry%20will,industry%20fuel%20bill%20this%20year (Accessed May, 2024).
61. ICF, ATAG. Fueling Net Zero. An ICF Report for ATAG Waypoint 2050 (2021). Available from: https://aviationbenefits.org/media/167495/fueling-netzero_september-2021.pdf (Accessed May, 2024).
62. Team C. Levelized Cost of Energy (LCOE). Canada: Corporate Finance Institute (2024). Available from: https://corporatefinanceinstitute.com/resources/valuation/levelizedcost-of-energy-lcoe/ (Accessed May, 2024).
63. Levelized Cost of Energy+. Available from: https://www.lazard.com/research-insights/levelized-cost-of-energyplus/ (Accessed May, 2024).
64. Alternative Fuels Data Center. Sustainable Aviation Fuel (2024). Available from: https://afdc.energy.gov/fuels/sustainable-aviation-fuel (Accessed May, 2024).
65. International Energy Agency (IEA). Energy and New Fuels Infrastructure Net Zero Roadmap. Aircraft Technology Roadmap. Available from: https://www.iata.org/contentassets/8d19e716636a47c184e7221c77563c93/energy-and-newfuels-infrastructure-net-zero-roadmap.pdf (Accessed May, 2024).
66. Sustainable Avaition ICF. Road Map for the Development of the UK SAF Industry (2023). Available from: https://www.sustainableaviation.co.uk/wp-content/uploads/2023/04/Sustainable-AviationSAF-Roadmap-Final.pdf (Accessed May, 2024).
67. Innovate UK Business Connect. Sustainable Aviation Fuel Innovation Programme. Available from: https://iuk-businessconnect.org.uk/programme/sustainable-aviation-fuel-innovation/ (Accessed May, 2024).
68. GOV.UK. Advanced Fuels Fund (AFF) Competition Winners (2024). Available from: https://www.gov.uk/government/publications/advanced-fuels-fundcompetition-winners/advanced-fuels-fund-aff-competition-winners (Accessed May, 2024).
69. Neste. Offsetting and Sustainable Aviation. Available from: https://www.neste.com/news-and-insights/aviation/offsetting-and-sustainable-aviation (Accessed May, 2024).
70. Edie. Heathrow Extends Incentive Scheme for Alternative Fuels With Lower Carbon Footprint (2023). Available from: https://www.edie.net/heathrow-extends-incentivescheme-for-alternative-fuels-with-lower-carbon-footprint/ (Accessed May, 2024).
71. Department for Transport. Government Support for a UK SAF Industry. GOVUK (2023). Available from: https://www.gov.uk/government/speeches/government-support-for-a-uk-saf-industry (Accessed May, 2024).
72. Advanced Fuels Fund. Advanced Fuels Fund Guidance Document for Applicants (2022). Available from: https://www.ricardo.com/media/ea3ldpe2/aff-guidancev11.pdf (Accessed May, 2024).
73. Grantham Research Institute on Climate Change and the Environment. How Do Emissions Trading Systems Work? (2024). Available from: https://www.lse.ac.uk/granthaminstitute/explainers/how-do-emissions-trading-systemswork/ (Accessed May, 2024).
74. Carbon Direct. How a UK SAF Mandate Could Change the Sustainable Fuel Industry. Available from: https://www.carbon-direct.com/insights/how-a-uk-saf-mandatecould-change-the-sustainable-fuel-industry (Accessed May, 2024).
75. Department for Transport. Lift Off for Projects Fuelling Jet Liners With Bin Liners. GOV. UK (2022). Available from: https://www.gov.uk/government/news/lift-off-for-projects-fuelling-jet-liners-with-bin-liners (Accessed May, 2024).
76. International Air Transport Association. IATA Guidance Material for Sustainable Aviation Fuel Management (2015). Available from: https://pdf4pro.com/file/eb00e/contentassets_d13875e9ed784f75bac90f000760e998_iata20guidance20material20for20saf.pdf.pdf (Accessed May, 2024).
77. Bp Global. Energy in Focus Magazine. News and Insights. Available from: https://www.bp.com/en/global/corporate/news-and-insights/energy-in-focus.html (Accessed May, 2024).
78. Solomon, R, Hayes, M, Brown, C, Parolini, G, Dangova, M, and Anderson, J. Sustainable Aviation Fuel Ready for Lift Off? (2022). Available from: https://www.semanticscholar.org/paper/Sustainable-Aviation-Fuel-Ready-for-lift-off-Solomon-Hayes/f6f377ae3d859e2f4c709e323f2fc6a516f60eec (Accessed May, 2024).
79. Singh, S. Why the UK Is Eager to Develop a SAF Production Industry. Canada: Simple Flying (2023). Available from: https://simpleflying.com/uk-develop-saf-productionindustry/ (Accessed May, 2024).
80. Department for Transport. UK SAF Industry Goes From Forest Waste to New Heights With £53 Million Boost. GOV. UK (2023). Available from: https://www.gov.uk/government/news/uk-saf-industry-goes-from-forest-waste-to-newheights-with-53-million-boost (Accessed May, 2024).
81. Innovate UK Business Connect. Feedstocks for a Sustainable Aviation Fuel Industry. Available from: https://iuk-businessconnect.org.uk/perspectives/feedstocks-for-a-sustainable-aviation-fuel-industry/ (Accessed May, 2024).
82. Sustainable Aviation. Urgent Government Action Needed to Meet 10% Sustainable Aviation Fuel by 2030 Target (2022). Available from: https://www.sustainableaviation.co.uk/news/urgent-government-actionneeded-to-meet-10-sustainable-aviation-fuel-by-2030-target/ (Accessed May, 2024).
Keywords: sustainability, feedstock, aviation, emissions, feasibility
Citation: Chapman RJ and Hettiarachchige Don A (2025) An Investigation of the Feasibility of Feedstock to Support the UK’s Sustainable Aviation Fuel Goals. Aerosp. Res. Commun. 2:14015. doi: 10.3389/arc.2024.14015
Received: 31 October 2024; Accepted: 24 December 2024;
Published: 24 January 2025.
Copyright © 2025 Chapman and Hettiarachchige Don. This is an open-access article distributed under the terms of the Creative Commons Attribution License (CC BY). The use, distribution or reproduction in other forums is permitted, provided the original author(s) and the copyright owner(s) are credited and that the original publication in this journal is cited, in accordance with accepted academic practice. No use, distribution or reproduction is permitted which does not comply with these terms.
*Correspondence: Rebecca J. Chapman, cmViZWNjYS5jaGFwbWFuMUBteXBvcnQuYWMudWs=