- 1Division of Neurology, The Hospital for Sick Children, Toronto, ON, Canada
- 2Edmond J. Safra Program in Parkinson’s Disease, Morton and Gloria Shulman Movement Disorders Clinic, Toronto Western Hospital, University Health Network, Toronto, ON, Canada
- 3Division of Neurology, University of Toronto, Toronto, ON, Canada
- 4Krembil Brain Institute, Toronto, ON, Canada
- 5Center for Advancing Neurotechnological Innovation to Application (CRANIA), Toronto, ON, Canada
Dystonia is the most common movement disorder in the pediatric population. It can affect normal motor development and cause significant motor disability. The treatment of pediatric dystonia can be very challenging as many children tend to be refractory to standard pharmacological interventions. Pharmacological treatment remains the first-line approach in pediatric dystonia. However, despite the widespread use of different ani-dystonia medications, the literature is limited to small clinical studies, case reports, and experts’ opinions. Botulinum neurotoxin (BoNT) is a well-established treatment in adults with focal and segmental dystonia. Despite the widespread use of BoNT in adult dystonia the data to support its use in children is limited with the majority extrapolated from the spasticity literature. For the last 2 decades, deep brain stimulation (DBS) has been used for a wide variety of dystonic conditions in adults and children. DBS gained increased popularity in the pediatric population because of the dramatic positive outcomes reported in some forms of genetic dystonia and the subsequent consensus that DBS is generally safe and effective. This review summarizes the available evidence supporting the efficacy and safety of pharmacological treatment, BoNT, and DBS in pediatric dystonia and provides practical frameworks for the adoption of these modalities.
Introduction
Dystonia is characterized by abnormal, often repetitive movements and/or postures that are caused by sustained or intermittent muscle contractions causing abnormal often repetitive movements and/or postures (1). Dystonia is classified along two axes. The first axis addresses the clinical characteristics, such as age at onset, body distribution, temporal pattern, and associated features (additional movement disorders and/or neurological features). The second axis concentrates on etiology which includes nervous system structural changes, effects of toxins/drugs, genetic mutation, etc. (2)
Dystonia is a clinical diagnosis and there are no specific diagnostic tests (3). There is great phenomenological heterogeneity in the presentation of pediatric dystonia, hence the importance of correct diagnosis to avoid diagnostic and therapeutic delays. Diagnosis of dystonia involves the identification of dystonic movements and postures that are typically repeated in individualized patients. Other physical signs are sensory tricks, activation with volitional movements, task specificity, and overflow activation (4). Accurate diagnosis of dystonia in the pediatric population poses additional challenges as many developmentally normal children have overflow movements due to an immature nervous system. Furthermore, children can present with very severe dystonia, often combined with spasticity, that manifests more as hypertonia than as abnormal movements (5).
Dystonia is among the most commonly observed movement disorders in pediatric clinical practice and usually involves the whole body (generalized) (3). Thus, dystonia frequently affects motor function and can be a source of significant motor disability. The etiology of childhood dystonia is very heterogeneous and requires a structured approach to reach the correct diagnosis promptly (6). Due to the rapid advances in the next generation sequences, more than 200 genes have been recognized as a cause of generalized child-onset dystonia. (7–11)
Dyskinetic CP is a common cause of dystonia in children and comprises 4%–17% of all cases of CP (12). Dystonia frequently co-exists with spasticity where it is referred to as “mixed tone.” (13, 14) In children with CP dystonia manifests with fluctuating hypertonia, involuntary postures, and abnormal movements triggered by arousal, cognitive tasks, and emotional state (15, 16). In these children dystonia can be generalized (trunk and at least two other sites are involved), focal (a single body part is affected), or segmental (two or more contiguous body regions are affected) (2). The presence of dystonia can impact motor function, pain, and ease of care in these patients (17). Despite the advances in the understanding of the pathophysiology of dystonia, treatment remains largely symptomatic. Thus, our review will not discuss specific therapies once the axis II reveals treatable dystonia (e.g., copper chelator in case of Wilson’s disease). Table 1 summarizes the inherited dystonias with specific treatments.
The goal of symptomatic treatment includes reducing pain, decreasing involuntary movements, preventing contractures, and improving motor function and quality of life (18). Therapeutic options must be individualized for each child and the majority of the children will require a combination of several drugs and treatments (19). Treatment options for childhood dystonia include physical and supportive treatment, oral medications, chemo denervation with botulinum toxin (BoNT), and neurosurgical procedures such as deep brain stimulation (DBS) (3). Although clearly beneficial the evidence of physical and other rehabilitative treatments is poor as very few studies have been performed in pediatric dystonia as well as in dystonia in general. Medications are first-line agents, and we’ll briefly discuss the principal ones, although even here the evidence is poor and mainly coming from old uncontrolled studies. In this article, we will focus on dystonia treatments with the highest level of evidence, namely BoNT and DBS, providing a narrative literature review and practical clinical recommendations.
Methods
In the following narrative review, we searched for systematic reviews on the topic of “pharmacological management of pediatric dystonia,” “BoNT treatment in dystonia/pediatric population,” and “DBS for treatment of pediatric dystonia.” The systematic reviews were supplemented with a review of the original articles.
Pharmacological Treatment for Pediatric Dystonia
Introduction
The first-line treatment of pediatric dystonia is pharmacological, although its scientific evidence is limited. The majority of therapeutic trials in childhood dystonia are not randomized controlled studies and the current treatment guidelines are based on the literature on adult movement disorders and expert opinion(3).
In 1984, Marsden et al. (20) described the so-called “Marsden cocktail” for the treatment of severe dystonia in children and adults. The cocktail is a combination of tetrabenazine, pimozide, and benzhexol. In recent years neuroleptics are rarely used for dystonia management due to inferior side effect profile and possible development of tardive dystonia, hence we will not discuss their use in the following review.
In the following section, we will review the most used medication to treat pediatric dystonia. Table 2 summarizes the recommended doses and side effects of the most used pharmacological agents, according to the rule A-B-C-D (anticholinergics, baclofen, clonazepam, and other benzodiazepines, and dopamine, i.e., levodopa, tetrabenazine, neuroleptics).
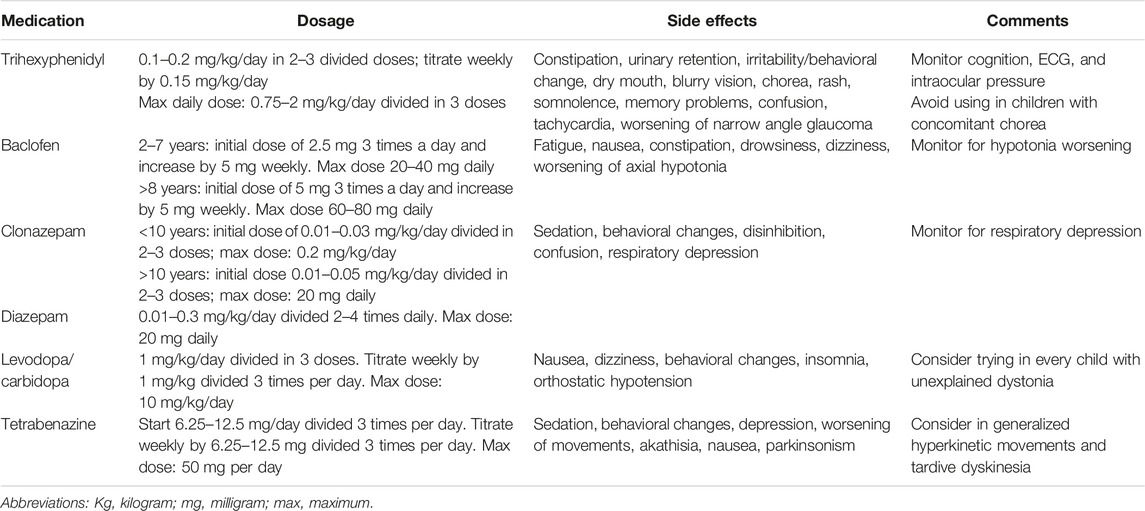
TABLE 2. Summary of common medications used to treat pediatric dystonia (from Lexicomp pediatric, AHFS clinical drug information pediatric.
Anticholinergics
Anticholinergic medications such as trihexyphenidyl are one of the most effective agents for the treatment of generalized dystonia in the pediatric population. Trihexyphenidyl blocks the action of acetylcholine on the central muscarinic receptors in the striatum. Animal studies demonstrated abnormal coupling between dopaminergic and cholinergic signaling and pathological elevation of striatal cholinergic tone in dystonia (21).
Trihexyphenidyl has the most robust evidence for dystonia management. It is usually considered a first- or second-line treatment for generalized dystonia in children. In a prospective, double-blind, crossover study (22) demonstrated significant clinical response with 30 mg trihexyphenidyl versus placebo in children and young adults with segmental and generalized dystonia (22 of 31 children and young adults age 9–32; the response was judged clinically significant if there had been improvement in dystonia scores if there had been an impact on daily function and if the benefit of the response outweighs side effects). Fahn et al. (23) conducted an open-label trial demonstrating a moderate to drastic improvement with trihexyphenidyl in 61%–71% (average daily dosage of 41 mg) of children versus 19%–38% in adults (average daily dosage of 24 mg).
A recent systematic review (24) evaluated dystonia management in children with cerebral palsy (CP). The authors identified 7 studies utilizing trihexyphenidyl in this population (23, 25–30). Overall the studies suggest that trihexyphenidyl results in little to no difference in dystonia, motor function, achievement of individualized goals, and ease of caregiving.
Trihexyphenidyl is better tolerated in children than in adults, however, adverse effects are still common in the pediatric population. The most common side effects are constipation (43%), decreased urinary frequency (19%), irritability/behavioral change (13%), and dry mouth (28). Other less common adverse effects include blurry vision, chorea, rash, and somnolence (26, 27). Children can tolerate higher doses than adults, but the cognitive effect remains a concern and requires close monitoring. Trihexyphenidyl usually requires prolonged treatment (weeks to months) before seeing a clinical response (26). Other anticholinergic medications include benztropine and procyclidine among others. The data on their use in the pediatric population is limited to the treatment of acute dystonic reactions (31).
Baclofen
Baclofen functions as gamma-aminobutyric acid (GABA) agonist on the GABA-B receptors. It reduces the motor neuron excitability mainly at the spinal cord level. Oral baclofen has a very limited blood-brain-barrier (BBB) penetration, hence high doses of oral baclofen are required to achieve clinical benefit (32).
Oral baclofen is frequently used in clinical practice in childhood dystonia, although the therapeutic evidence is extremely limited and most of the data is based on expert opinion (33, 34). While trihexyphenidyl is considered a first-line treatment, baclofen is considered a second or third-line treatment (34). Greene et al. (35) found moderate improvement with baclofen in 7 out of 16 children with idiopathic dystonia (based on patients’ reports). A subsequent study (36) evaluated baclofen treatment in idiopathic childhood dystonia: based on a retrospective chart review, 10% responded to oral baclofen versus 51% who responded to anticholinergic agents. No studies evaluating oral baclofen in the CP population were identified.
Baclofen is generally well-tolerated in children. The most common adverse effects include fatigue, nausea, constipation, drowsiness, and dizziness. High doses of baclofen can exacerbate axial hypotonia (36, 37). Given its poor ability to cross the BBB when given orally, baclofen administered intrathecally by means of a pump has a greater effect on spasticity and dystonia and will discuss later.
Benzodiazepines
Benzodiazepines are frequently used to treat pediatric dystonia. Benzodiazepines bind to central GABA-A receptors and increase the inhibitory hyperpolarization of the neurons expressing these receptors (37).
Benzodiazepines are generally considered a second or third-line treatment for dystonia. No studies have compared the different benzodiazepines in the pediatric dystonia population, but generally, longer-acting benzodiazepines are used. Greene et al. (36) found that 16% of individuals (children and adults) responded to clonazepam. Children had a better response rate than adults. Chuang et al. (38) evaluated 33 patients (children and adults) with hemidystonia and found a 50% response rate to clonazepam and diazepam (defined as any improvement reported by the patient). Clonazepam was also reported to be beneficial in the treatment of myoclonus-dystonia in the pediatric population (39, 40). The majority of the data regarding the treatment of myoclonus-dystonia comes from the adult literature and includes drugs such as zonisamide, tetrabenazine, and levodopa (41).
No studies evaluating benzodiazepines in the CP population were found.
Benzodiazepines are very well tolerated in the pediatric population and have fewer adverse effects compared to trihexyphenidyl, baclofen, and levodopa (34). The most common adverse effect is sedation. Less frequent side effects include behavioral changes, disinhibition, confusion, and respiratory depression (38). Sudden cessation of benzodiazepines can cause worsening of dystonia and withdrawal symptoms.
Dopaminergic Medications (Levodopa and Tetrabenazine)
Levodopa
Levodopa crosses the BBB and converts into dopamine. Its mechanism of action in dystonia is not fully understood.
Levodopa is the principal treatment in levodopa-responsive dystonia (DRD) and produces a significant clinical benefit in these conditions (42, 43). A trial of levodopa is warranted (for at least 3 months up to the dose of 600 mg/day (10 mg/kg/day) if needed) in every child with an unknown cause of dystonia (44).
A single randomized crossover trial evaluated the levodopa treatment in CP and dystonia (45). This trial failed to demonstrate upper extremity motor function improvement. The authors did not evaluate dystonia severity, pain/comfort, or quality of life measurements.
The side effect profile is generally favorable in the pediatric population. The most common side effects include nausea, dizziness, behavioral changes, insomnia, and orthostatic hypotension.
Not all DRDs respond greatly to levodopa with up to 36% of children with tyrosine hydroxylase deficiency showing no response to this treatment (46). Furthermore, depending on the genetic cause of DRD, additional treatments with mono-oxidase B inhibitors (e.g., selegiline), antidepressants, tryptophane, and carbidopa might be needed. This is especially the case for the autosomal recessive forms of DRD (47, 48).
Tetrabenazine
Tetrabenazine causes a selective and reversible depletion of monoamines from the synaptic terminals by blocking the VMAT2, a vesicular monoamine transporter expressed in the central nervous system. It preferentially affects dopamine, but norepinephrine, serotonin, and histamine are also depleted (49, 50). Tetrabenazine is mainly used for the treatment of chorea and other hyperkinetic movement disorders in the adult and pediatric population (51–53). The evidence of its efficacy in pediatric dystonia is very limited. Jankovic et al. (54) conducted a retrospective review of 124 patients (adults and children) with dystonia who were treated with tetrabenazine: 76% of them experienced moderate improvement. Individuals with generalized and tardive dystonia had greater improvement than patients with focal and cranial involvement. This study did not provide separate information on the pediatric subgroup and it is very difficult to conclude efficacy in children in mixed cohorts.
A recent multicenter retrospective longitudinal study evaluated the usage of tetrabenazine in children with CP. A significant clinical improvement was detected between baseline and after 6 and 12 months of treatment (55).
Adverse effects are common with tetrabenazine treatment. Most of the adverse effects are dose-related. The most common side effects include sedation, behavioral changes, depression, worsening of movements, nausea, and parkinsonism (50). Notably, tetrabenazine has never been associated with tardive dystonia, thus making it a good option for these patients.
Newer VMAT2 inhibitors (deutetrabenazine and valbenazine) were recently approved for tardive dystonia treatment in the adult population (56, 57), but no data on their use in pediatric dystonia is available at the moment.
Botulinum Toxin Treatment for Pediatric Dystonia
Introduction
BoNT is produced by an anaerobic spore-forming bacteria Clostridium botulinum. Seven immunologically distinct serotypes (A-G) have been identified (58). BoNT temporarily inhibits the release of acetylcholine at the neuromuscular junction levels by creating focal chemo-denervation at the injection site, eventually resulting in muscle relaxation (59). In a physiological state, when an action potential reaches the cholinergic presynaptic nerve terminal an influx of calcium facilitates acetylcholine vesicle fusion with the presynaptic membrane. This fusion is facilitated by a group of proteins called SNARE (soluble N-ethylmaleimide sensitive factor attachment receptor). BoNT acts on the presynaptic nerve terminal by inactivating serotypes-specific SNARE proteins (60). Neurotransmission recovers when the axon terminal sprouts new nerve endings and forms new synaptic contacts with an adjacent muscle fiber.
Neurotoxin type A (OnabotulunumtoxinA – Ona-A, AbobotulinumtoxinA – Abo-A, IncobotulinumtoxinA – Inco-A) and B (RimabotulinumtoxinB – Rim-B) are approved and used in clinical practice with type A being the most widely used in the pediatric population (58).
While BoNT is the first-line treatment for adult patients with focal dystonias (blepharospasm and cervical dystonia) and it is also effective in individuals with laryngeal and limb dystonia (61–65), the data to support the treatment of dystonia in the pediatric population is limited and the majority of data are extrapolated from the spasticity literature.
Botulinum Neurotoxin Treatment for Inherited Pediatric Dystonia
We failed to identify studies systematically evaluating the BoNT effect in this population, but in clinical practice, BoNT can be used as an adjunctive to pharmacological/surgical therapy to control pain and improve individualized goals, exactly as done in all dystonia patients (50).
Botulinum Neurotoxin Treatment for Acquired Pediatric Dystonia—Cerebral Palsy
A recent systematic review and meta-analysis evaluated BoNT treatment in children with CP and dystonia (24). This review identified a total of 5 studies (one randomized crossover trial and four non-randomized studies) (66–70). The randomized study failed to demonstrate improvement in the dystonia while non-randomized studies suggested possible improvement in the dystonia as well as upper extremity motor function (using the Quality of Upper Extremity Skills Test) (71). The studies also supported pain reduction, improvement in ease of care as well as improvements in the achievement of individualized goals within the CP population. These findings are limited due to the subjective nature of these observations. There was no change in the quality of life in CP children treated with BoNT. Four studies reported adverse events. There was an increased risk of dysphagia among participants with cervical dystonia and up to 40% of participants reported transitory focal weakness in the treated limb. The authors concluded that there is some evidence (although limited) to support the use of BoNT in children with CP and dystonia, mainly to improve ease of caregiving, control pain, and help with the achievement of individualized goals.
Botulinum Neurotoxin Formulations
All four commercially available BoNT’s formulations (Ona-A, Abo-A, Inco-A, and Rim-B) are FDA-approved for the treatment of adults with cervical dystonia while Ona-A and Inco-A are approved in individuals 12 years and older for blepharospasm. The use of BoNT for other dystonias is considered off-label (72). Ona-A and Abo-A are FDA-approved for upper and lower extremity spasticity in children (>2 years) and Inco-A is approved for pediatric sialorrhea and pediatric upper extremity spasticity (>2 years).
The most commonly used formulations in the pediatric population are Ona-A and Abo-A.
Conversion Between Different Formulations
All BoNT products are distinct in their molecular structure, manufacturing process, and methods to determine biological activity. There is no agreed conversion ratio between Ona-A and Abo-A, but some studies reported a ratio of 1:2.5–1:3. The doses of Inco-A are probably parallel those of Ona-A with a conversion ratio of 1:1 (73, 74).
Dilution
The most common dilutions used in the pediatric studies are 100 U/1 ml or 100 U/2 ml (range 1–4 ml saline/vial) for Ona-A and Inca-A and 500 U/2.5 ml (range 1–5 ml saline/vial) for Abo-A. The precise impact of the dilution on the rate of the spread is not known and most of the studies were done on children with spasticity. Studies in children demonstrated conflicting results. Some showed no difference between groups with different dilution ratios (100 U/5 ml −100 U/2.5 ml) while others showed improvement in the muscle tone and electrophysiology (dilution 100 U/2 ml) (75–77). In 2012 Fehlings et al. (78) evaluated the Canadian practice patterns of BoNT injections in pediatric hypertonia (spasticity and/or dystonia): 79% of physicians reported using 100 U/2 ml dilution of Ona-A for lower limb injections while only 36% were using similar dilution in upper extremities.
Botulinum Neurotoxin Doses
There are currently no standard guidelines for BoNT dosing for pediatric dystonia. Injection doses are often extrapolated from adult literature as well pediatric spasticity treatment (Table 3).
Dose calculation for each preparation is based on a few key factors: (1) total units per treatment session (i.e., the total number of units given during a single treatment session), (2) total units per kg body weight per session (i.e., total units per kg of body weight per single treatment session), (3) units per muscle, (4) units per injection site, and (5) units per kg of body weight per muscle. Additional dose modifiers to consider include the severity of the dystonia, accompanying diagnosis (dysphagia, aspiration, breathing problems), presence of other tone abnormalities (spasticity), goals of treatment, the activity of the injected muscle, muscle size, dynamic versus fibrotic muscle and experience with previous BoNT (58, 79).
The European consensus 2009 on the Use of Botulinum Toxin for Children with Cerebral Palsy recommended 400 U or 20 U/Kg of Ona-A as a maximum total amount per session and 1000 U or 20 (30) U/kg for Abo-A. Adult dosing is recommended for children heavier than 60 kg (79). Inco-A can be used at a maximal dose of 5 U/kg per session (80).
In the study of Canadian practice patterns of BoNT injections in children with hypertonia, the majority of the practitioners used 16 U/kg of Ona-A as a maximal injected dose per session (78).
Lower Extremities Injections
In the clinical studies lower limb doses ranged from 1 to 12 U/kg of Ona-A and 15–30 U/kg of Abo-A for the gastrocnemius/soleus muscles. For the hamstrings and adductor muscles, 1–5 U/kg of Ona-A or 20–30 U/kg of Abo-A were found to be safe and beneficial (81).
Upper Extremities Injections
The recommended doses of Ona-A for upper extremity injections are 0.5–2 U/Kg for forearm muscles, 2–3 U/kg for the arm muscles, and a total of 5–7.5 U for adductor or opponens pollicis (62, 82). Kawamura et al (83) performed a double-blind randomized control trial to evaluate the Ona-A doses for treatment of upper extremity spasticity in children with CP. The higher dose group received double the doses of the lower dose group. The study showed no differences between the treatment groups and concluded the following recommended doses: biceps brachii 1 U/kg, wrist/finger flexors 1.5 U/kg, brachioradialis, and pronator teres 0.75 U/kg, adductor/opponens pollicis 0.3 U/kg.
Pain Management and Localization Techniques
Procedural pain management is extremely important in children. BoNT treatment often requires repetitive, multiple painful injections. The optimal regimen will vary between individuals and is influenced by the child’s age, cognitive level, underlying condition, number of muscles to be treated, and institutional settings and resources. Procedural pain management includes pharmacological and non-pharmacological techniques. Possible techniques include distraction, application of local EMLA (lidocaine and prilocaine topical anesthetic cream), sedation, and general anesthesia (79).
In the past, palpation and the use of anatomical landmarks was the most widely used localization technique in the pediatric population. With the advances in technology, ultrasound has become a very helpful and widely used tool. The advantages of this technique include less pain and the possibility to control the injection placement even with anatomic variations, differentiation of neighboring anatomic structures, and not requiring cooperation, i.e. not being affected by sedation. Electromyography (EMG) is widely used in the adult population with dystonia. This method has a few significant disadvantages in children: painful injections and discomfort during muscle stimulation, limited cooperation for muscle activation, sedation may lower the EMG signal and it does not always ascertain that the needle is in the right muscle. (58, 79).
Safety and Adverse Events
BoNT is safe and well-tolerated in the pediatric population (3, 79). Several long-term longitudinal studies demonstrated its safety and effectiveness with prolonged treatment. Systemic adverse effects like generalized weakness, bulbar weakness, and respiratory involvement are rare (67, 84). Adverse reactions correlate to the location of the injection, dose, and frequency as well as the underlying condition and involvement of other systems. Children with CP experience a greater number of BoNT related adverse effects than other users (84).
Intrathecal Baclofen Treatment in Pediatric Dystonia
In 1985 the first pediatric treatment with intrathecal baclofen (ITB) in a 4-year-old girl with spasticity and opisthotonos following a near-drowning was reported, (85) followed a few years later by children with CP. (86) Oral baclofen absorbs rapidly, but less than 10% crosses the BBB. Because its main effect is within the central nervous system, intrathecal doses up to 100 times lower than oral ones can be used achieving a great therapeutic effect (87). In children, the typical dose range is between 200 and 350 µg/24 h (88). The therapy is delivered via an implantable infusion system directly to the intrathecal space.
The most common indication for ITB in children is spastic and dystonic CP. Other indications include metabolic and neurodegenerative conditions such as Pantothenate kinase-associated degeneration (PKAN), Lesch Nyhan syndrome (LNS), glutaric aciduria type 1, and mitochondrial disorders (89–92). ITB is particularly beneficial in reducing the tone in the lower limbs (with catheter placement at T10-11 level), although upper extremity tone reduction can be seen with a higher catheter placement (C1-C4) (93). A recent systematic review found a clinically significant improvement in dystonia severity, motor function, pain/improvement, and a trend toward improvement of individualized goals and ease of caregiving in children with dystonia due to CP treated with ITB (24). There is currently insufficient evidence to support the use of ITB in primary dystonia due to the absence of spasticity (now called “isolated dystonia”), for which ITB is more effective (94).
ITB pumps have relatively high complications rates (25%–30%) (95, 96). Complications can be divided into procedure-related, pump-related, catheter-related, and drug-related. Infection is the most common procedure-related complication (1%–5% of implantations) and usually occurs within the first 6 weeks. Other complications include CNS pressure-related problems, seromas, bleeding, spinal cord damage, and corticospinal fluid leaks. Catheter-related complications are reported in 5%–15% of cases and usually happen within the first 3 months. It includes catheter migration, disconnection, kinking, and occlusion. Dose-dependent tiredness is the most common drug-related side effect, followed by urinary retention, constipation, and hypotonia that can affect daily functions. These side effects are dose-related and reversible (97).
Sudden cessation of ITB can cause a “baclofen withdrawal syndrome,” which is a life-threatening medical emergency. Baclofen withdrawal can occur in children taking both oral baclofen and ITB. Patients typically experience withdrawal symptoms within hours to days following drug interruption often due to malfunction of their ITB system (98). In general, withdrawal from oral baclofen is associated with mild symptoms including the reappearance of baseline level of spasticity/dystonia, pruritus, sweating as well as neurogenic pulmonary edema, anxiety, and disorientation. More severe symptoms (seen more frequently with withdrawal from ITB) include hyperthermia, myoclonus, seizures, rhabdomyolysis, disseminated intravascular coagulation, multisystem organ failure, cardiac arrest, and death. (99–101) Baclofen withdrawal syndrome is a clinical diagnosis and should be considered and promptly treated in every child with ITB who presents with increased hypertonicity or irritability.
Deep Brain Stimulation in Pediatric Dystonia
Introduction to Pediatric Deep Brain Stimulation
Pediatric dystonia can be refractory and pose a significant challenge to healthcare providers and families. While pharmacological management is typically the mainstay of treatment, poor efficacy and high rates of adverse drug reactions contribute to the increased interest in neurosurgical approaches and particularly DBS (34). DBS for dystonia should be considered as a treatment option once it has become clear that medical therapy is insufficient to control symptoms. In 1999, Coubes et al were among the first to report chronic stimulation of globus pallidus pars interna (GPI) in an 8-year-old child with generalized dystonia (102). Since then, DBS gained increased popularity in the pediatric population because of the dramatic positive outcomes reported in the genetic dystonia and a consensus that DBS is generally safe and effective in many adult dystonias (103, 104). Furthermore, recent advances in DBS technology made it more suitable for the pediatric population (105). Smaller batteries, longer battery life, and the development of rechargeable systems made this technology applicable to children. Some of the current DBS systems allow simultaneous local field potential (LFP) recordings. The DBS programming process can be very challenging in non-verbal children and LFP recordings can ease and expedite the process (106).
The data pertaining to DBS in children is limited to small case series. During the last few years, there were several systematic reviews published summarizing the existent literature related to DBS in pediatric dystonia (107–109). In the following section, we will review some of these studies and make practical recommendations for patient selection, DBS- targets, and use of DBS in status dystonicus.
Deep Brain Stimulation Targets
Bilateral GPI is the most common target in pediatric dystonia. In a systematic review focusing on DBS in pediatric dystonia, Hale et al showed that 91% of children were treated with bilateral GPI-DBS. The remainder of the published cases received unilateral GPI-DBS with or without contralateral GPI lesioning (109). A few case reports described good dystonia outcomes in children post subthalamic nucleus (STN) DBS (110–112). STN has been chosen often in children with GPI lesions (metabolic strokes) or post-failed GPI-DBS. Limited data is available on the use of DBS of the thalamic nuclei ventralis oralis posterior (Vop) and ventralis intermedius (Vim) for the treatment of acquired dystonia in the pediatric population. Small studies in adults (113, 114) showed that combined Vop/Vim stimulation can affect the inputs from the cerebellum as well as from the globus pallidus leading to more effective treatment of secondary dystonia. Luciano et al. (115) reported variable dystonia outcomes in four individuals (three children and one young adult) who underwent Vop/Vim DBS for secondary dystonia. All the participants experienced an improvement in their disability and quality of life scores.
Overall, bilateral GPI-DBS is considered the most appropriate target for pediatric dystonia, and certainly more studies are needed to characterize the role of other targets.
Assessment of Deep Brain Stimulation Outcomes
Pre-treatment evaluation aims at characterizing the severity and topography of motor symptoms and their impact on activities of daily living and provides a baseline reference for post-treatment evaluations. The quality and accuracy of the pre-treatment assessment and the choice of assessment tools are crucial, as they will affect all subsequent post-treatment comparisons (116). The Burke-Fahn-Marsden Dystonia Rating Scale (BFMDRS) is a well-accepted rating scale for generalized dystonia in adults (117). It is composed of two clinician-rated subscales: a movement subscale (BFMDRS-M), based on patient examination, and a disability subscale (BFMDRS-D), based on the patient’s report of disability in activities of daily living. Although, there are no validity and reliability studies to assess this scale in children many pediatric DBS trials use the BFMDRS scores to measure the outcomes. This scale has significant limitations specifically in the pediatric population. It does not account for normal development dystonic-like movements patterns that are frequently encountered in normally developing children and the disability sub-score is hard to apply to the younger age groups (5, 118). Furthermore, it does not account for the important quality of life-related elements including preoperative functional goals and the Canadian Occupational Performance Measure functional goal area (outcome measure designed to assess performance and satisfaction with occupation (119)). These elements can improve even in an absence of significant BFMDRS benefits (120, 121). Pinter et al. (122) established the minimal clinically important difference for the BFMDRS in the adult population with generalized or segmental dystonia at 16.6%. Similar studies were not conducted in children.
Other frequently used dystonia scales in the pediatric population include Barry-Albright Dystonia Scale (BADS) (123) and the Movement disorder -Childhood Rating Scale (MD-CRS) (124,125). Despite the widespread use of these scales, none was rigorously tested across a broad range of development.
Patient Selection and Prediction Factors
Careful patient selection is extremely important. Expectations should be set based on patient-related factors including the type of dystonia, genetic cause, target symptoms, age at the time of surgery, disease duration, and presence of fixed skeletal deformities (126). Assessment of the sources of social and mental family support is also warranted (Table 4).
Age and Timing of the Surgery
The effect of patient age and timing of intervention on children’s outcomes were assessed in a few studies (127, 128). Lumsden et al. (127) followed 63 children with generalized dystonia for 1 year after GPI-DBS. No significant correlation was seen between motor outcomes (change in BFMDRS) and age at surgery or age at dystonia onset. Within the primary dystonia group, a negative correlation between dystonia duration and outcomes at 6 and 12 months was observed (Spearman’s correlation coefficient −0.425 and −0.472). A stronger negative correlation was found between disease duration to the age of surgery ratio (DD/AS) with Spearman’s correlation coefficient −0.614 (6 months) and −0.559 (12 months). Within the secondary dystonia group, no correlation was found between motor outcomes and dystonia duration, however, a negative correlation was found between DD/AS and improvement in BFMDRS at 6 and 12 months (Spearman’s correlation −0.251 and −0.416). A recent systematic review demonstrated that age at dystonia onset, and not age at surgery, is associated with treatment response. The duration of life with dystonia (i.e., shorter life between diagnosis and DBS) was a significant outcome predictor (107).
DBS was reported in children as young as 4 years of age, (104) and there are few unpublished cases even younger. Nevertheless, the majority of the reported cases are done in teenagers. In a recent systematic review, the mean age at DBS surgery was 13.8 ± 3.9 (109).
Etiology of the Dystonia
Children with genetic dystonias without central nervous system pathology have overall favorable outcomes after DBS. This notion is specifically true for TOR1A-, KMT2B-, and SCGE-related dystonia in particular (107, 129, 130). Children with inherited dystonia with clear structural brain damage, such as PKAN and LNS can experience clinically significant improvement post-DBS, although the response can be very variable and with limited impact on patients’ functionality (131–135). Children with dyskinetic CP have an inferior response to DBS with only 27% experiencing a clinically significant improvement (24, 107, 109). This is usually due to the occurrence of additional DBS-resistant neurological signs, such as weakness, ataxia, and spasticity. Thus, isolated dystonia responds better than acquired combined dystonias.
Mobile Versus Tonic Dystonia
Hyperkinetic movements respond more rapidly and better than tonic or fixed postures. Patients who had little, or no improvement tended to have severe tonic posturing (136). EMG studies in adults suggested that repeated bursts could indicate an earlier and better response to DBS (137).
Body Distribution
Dystonia distribution can also help with predicting the clinical outcomes. Significant improvement can be seen in the neck, arm, and leg regions; the trunk can also improve in absence of spine deformities and with time, while speech and other bulbar symptoms tend to be less responsive (136).
Neurophysiological Markers
McCelland et al. (138) evaluated the somatosensory evoked potentials (SEP) and central motor conduction times (CMCT) in children with dystonia who underwent GPI-DBS. Better DBS outcomes were seen in children with normal SEP and CMCT. These findings were independent of dystonia etiology and MRI findings. Further studies are needed to validate these findings and incorporate these assessments into clinical practice.
Deep Brain Stimulation for Monogenic Dystonia
The genetic understanding of dystonia has expanded with advances in next-generation sequencing. An increasing number of dystonia genes have been identified during the last decade (9, 10). The identification of causative genes has reduced the number of so-called “idiopathic” cases. The responsiveness to DBS appears to vary between different monogenic forms of dystonia, with some improving more than others. We will briefly review the most common monogenic dystonias in the pediatric population and their response to GPI-DBS.
DYT-TOR1A- related dystonia is one of the most common causes of young-onset familial dystonia and typically begins between 9 and 12 years of age. Lower extremities are commonly the first affected area followed by generalization with relative sparing of cervical and bulbar areas (139). Children with DYT-TOR1A dystonia respond very well to GPI-DBS with 93% demonstrating some improvement and 88.2% showing significant clinical improvement (>20% in the BFMDRS scores) (107). The median change in dystonia scores was 76% (movement) and 70% (disability). These findings are consistent with the results seen in adults with DYT-TOR1A (140).
DYT-SCGE-related dystonia is another autosomal dominant disorder with paternal expression and reduced penetrance with the maternal transmission. It presents in childhood with upper extremities myoclonus and dystonia causing writer’s cramps and cervical dystonia (Myoclonus- Dystonia’). Children commonly experience psychiatric comorbidities including anxiety and obsessive-compulsive disorder (141, 142). DBS significantly improves both dystonia and myoclonus. All the reported children in the literature showed significant improvement after DBS with a median improvement of 68% in their BFMDRS-M scores (107).
DYT-KMT2B-related dystonia is emerging as one of the most common causes of early-onset genetic dystonia. Key features of the disease include focal motor features at the disease presentation, evolving in a caudocranial pattern into generalized dystonia with prominent oromandibular, laryngeal, and cervical involvement (130). Additional features include dysmorphic features, microcephaly, developmental delay, and intellectual disability (143, 144). Children with KMT2B- related dystonia have a very good response to DBS. Cif et al. (130) reported 18 individuals (15 children) who underwent GPI-DBS. More than 50% fulfill criteria for optimal response (BFMDRS-M > 30% improvement). This effect was maintained in 62% of the long-term follow-up subgroup (follow-up> 5 years). The authors also concluded that the dystonia improvement was maintained for the trunk (53.2%), neck (50.5%), and oromandibular regions (35.7%). Dystonia improvement was inferior in the lower extremities with only 16.3% maintaining motor improvement.
GNAO1-related dystonia is caused by heterozygous mutations in GNAO1, a gene involved in a spectrum of disorders including early-onset epileptic encephalopathy, neurodevelopmental delay, and movement disorders (145, 146). DBS was found to be effective in children with GNAO1-related dystonia and hyperkinesia. Data is limited to case reports and small case series. Koy et al. (147) reviewed 12 children who underwent DBS. All had a good to excellent clinical response with the greatest effect on the hyperkinetic movements and prevention of status dystonicus. In the majority of the children, the beneficial response sustains for a long period of time (reported follow-up of up to 10 years).
THAP1- related dystonia is an autosomal dominant condition caused by mutations in the THAP1 gene (148). Onset typically occurs during childhood or adolescence with frequent involvement of the craniocervical, laryngeal and oromandibular areas. Generalization was reported in about 45% of the mutation carriers (149, 150). Initial case series reported only moderate response to DBS in this condition (151, 152). Recent retrospective, multicenter case series (153) of 14 individuals (12 with disease onset <18 years; 7 underwent GPI-DBS before the age of 18 years) demonstrated a median of 58% BFMDRS-M improvement. Overall, the effect was greater in the trunk and limbs as compared to the craniocervical and oropharyngeal areas. The authors did not find a correlation between disease duration, age at surgery, and preoperative disease burden. More research is needed to understand the role of DBS in children with this condition.
Deep Brain Stimulation for Neurodegenerative Disorders
Lesch-Nyhan syndrome (LNS) is a rare X-linked disorder characterized by a deficiency of the hypoxanthine-guanine phosphoribosyltransferase enzyme. Boys with this condition manifest with compulsive self-mutilation, generalized dystonia, and dyskinesia (132). Overall children with LNS respond well to DBS with clinically significant improvement in dystonia (107). Some children might also experience a decrease in self-mutilation. Several authors suggested a combination of anteromedial and posteroventral GPI stimulation to control dystonia as well as behavioral problems (138). A recent study by Visser et al. (154) followed 14 children with LNS who underwent GPI-DBS. Patient-centered outcome measures demonstrated substantial variability among individual patients suggesting that response might be less positive than previously reported. Children with LNS experience higher rates of DBS-related complications, such as infections and hardware-related complications. Thus, high vigilance for possible complications is warranted in this population.
Pantothenate kinase-associated neurodegeneration (PKAN) is a rare recessively inherited disorder. Typical PKAN presents in children younger than 6 years with a progressive course. Dystonia is typically the early manifestation with subsequent generalization and involvement of cranial, limb, and trunk muscles. Up to 90% of children present with gait difficulties followed by pyramidal features, movement disorders, neuropsychiatric involvement, and visual abnormalities (155, 156). Elkaim et al. (107) identified in the literature 36 children with PKAN who underwent GPI DBS. In general, these children experienced a significant clinical improvement in dystonia (median improvement of 27% in BFMDRS-M). Timmermann et al. (157) reported 14 individuals (children and young adults) who experience significant improvement in the severity of dystonia and quality of life up to 15 months post-DBS implantation. A recent meta-analysis confirmed these data but also showed limited benefits in terms of functional gains (119).
Deep Brain Stimulation for Acquired Dystonias
Recent systematic review and meta-analyses evaluated the effect of DBS on children with CP and dystonia (24). Overall the outcomes in these individuals are inferior to monogenic dystonias and neurodegenerative etiologies. The systematic review included 19 non-randomized studies. The meta-analysis (total number of included patients: 173) suggested an overall improvement in dystonia. Studies reporting on BFMDRS-M outcomes demonstrated 16.8% improvement, a figure just above the minimal clinically important difference established among individuals with primary dystonia (122). The follow-up ranged between 6 months and 4 years and 5 months. Eleven studies (number of included patients: 109) reported motor function outcomes. These studies demonstrated an improvement in motor function while no change in the BFMDRS-D was observed.
Five studies (number of included patients: 78) reported validated measures of pain and discomfort demonstrating improvement in these outcomes. There is a limited amount of evidence supporting the improvements in the achievement of individualized goals and quality of life.
In conclusion, although modest, recent evidence suggests that DBS may improve dystonia, motor function as well as pain and discomfort in children with CP. There might be a possible positive effect on the quality of life and the achievement of individualized goals.
Deep Brain Stimulation for Status Dystonicus
Status dystonicus (SD) is a movement disorder emergency characterized by severe episodes of generalized or focal hyperkinetic movements that have necessitated urgent hospital admission because of direct life-threatening complications of these movements, regardless of the patient’s neurological condition at baseline (158, 159). Sixty to 80 percent of SD occurs in children and adolescents and the majority are male, with dystonia duration of an average of 6 years (160, 161). Garone et al. (162) described 34 pediatric cases from a cohort of 336 dystonic children who experienced 63 acute exacerbations, suggesting that SD may affect up to 10% of children with dystonia. The most common underlying conditions in children with SD include CP followed by monogenic dystonias and degenerative conditions such as PKAN (163, 164). The mortality rate in SD ranges from 10.3% to 11.4% hence early recognition and aggressive intervention are warranted (161, 165). Pharmacologic treatment is the first-line treatment of SD and its complications; however, many refractory patients will still require further treatment. Whenever SD is not controlled with oral medications escalation to more aggressive sedation may be required. This is typically done with intravenous (IV) midazolam. If severe dystonia persists, anesthetic agents (e.g., IV propofol) should be considered (166).
Multiple case reports have shown that DBS can be an effective treatment for SD with rapid improvement of symptoms following the surgery (165, 167, 168). Nerrant et al. 173described 40 patients who underwent GPI DBS for SD (60% children). DBS was efficient in resolving 90% of episodes. 56.6% of children experienced a long-term improvement in their dystonia following DBS for SD, while 36.7% returned to baseline. Currently, there are no standard algorithms to treat children with SD, but we believe that surgical interventions should be considered early in the course of pediatric refractory SD. Figure 1 summarizes an updated proposed algorithm.
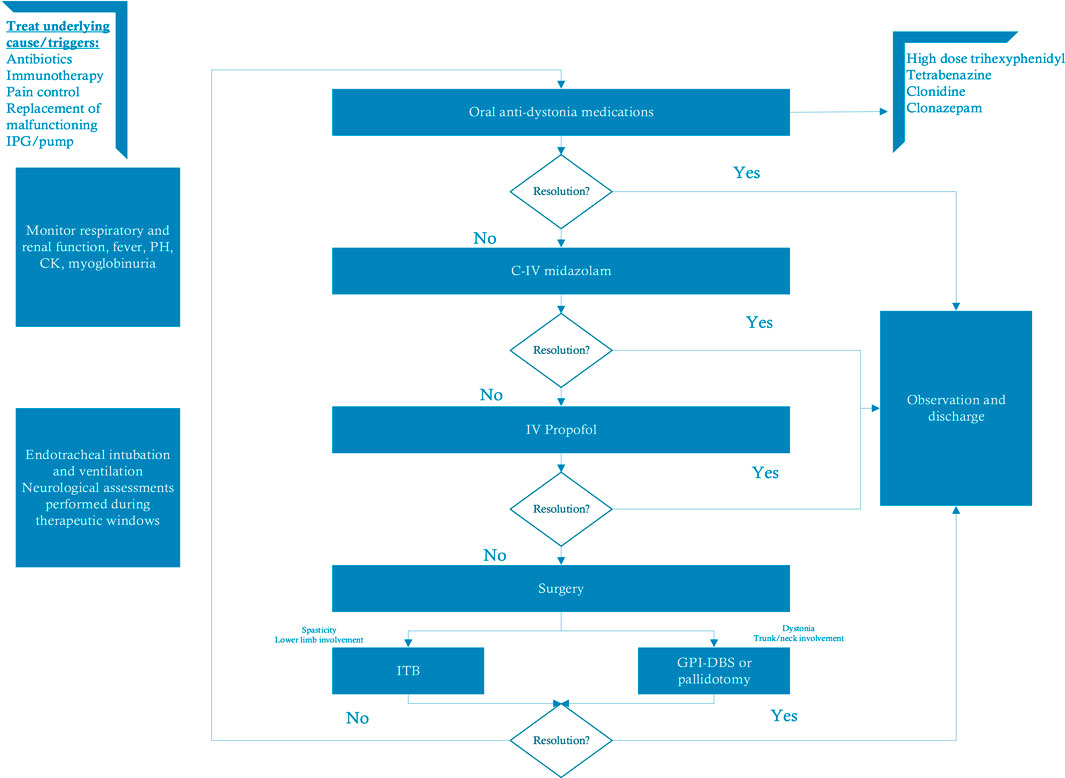
FIGURE 1. Proposed algorithm for treatment of status dystonicus (modified from Meijer I.A. & Fasano A). (173) In children with status dystonicus it is essential to identify and treat the underlying triggers. The first treatment step includes a trial of anti-dystonia medications followed by intravenous midazolam and propofol. In refractory cases, surgical intervention with GPi-DBS or ITB might be warranted. Abbreviations: C-IV, continuous intravenous; DBS, deep brain stimulation; GPi, globus pallidus internus; ITB, intrathecal baclofen; IV, intravenous; IPG, implantable pulse generator.
Side Effects in Pediatric Deep Brain Stimulation
Complication rates of pediatric DBS are higher than in the adult population (107). This notion is also seen in children with other implant procedures such as ventriculoperitoneal shunts (169). The most commonly reported complications include infections and mechanical failure. Kaminska et al. (169) analyzed complications in a prospective study and included 129 pediatric cases. The overall risk of surgical site infection was 10.3% for new implants with 86% of these requiring complete removal of hardware. Another 69 revisions were gathered, mostly due to battery changes and technical problems.
DBS may result in an increased risk of adverse events in children with CP. Overall reported rates of adverse events are ranging from 0% to 40%. The most common events included infections requiring hardware removal (7%–40%) and stimulation-induced dysarthria (17%–30%). (24)
Conclusion
Childhood-onset dystonia is a clinically and etiologically heterogeneous disorder. Despite the wide use of anti-dystonia medications in pediatric dystonia, the evidence is very limited. Levodopa should be tried in every child with an unknown cause of dystonia as its effect in DRD is remarkable. Trihexyphenidyl is considered a first-line treatment while benzodiazepines and tetrabenazine can be used in selected cases. Neuroleptics—once part of the so-called Marsden’s cocktail—are rarely used nowadays due to the recognized risk of inducing tardive dystonia.
Yet, many children tend to be refractory to standard pharmacological treatments. BoNT is safe and widely used in children to treat upper and lower extremity spasticity, but studies to assess its use in dystonia are limited. Recent evidence supports the notion that BoNT can improve dystonia and motor function, decrease pain as well as help with caregiving and achievement of individualized goals in a selected group of children. It is suggested to follow the established pediatric doses to avoid side effects and complications. The use of sedation or other pain-reducing techniques is recommended to increase patients’ comfort and long-term compliance.
DBS has revolutionized the field of pediatric dystonia. Children with genetic mobile dystonia involving the axial and appendicular muscles should be referred early for DBS consideration to avoid fixed contractures and assist with motor development. SD is a common underrecognized life-threatening condition with high mortality rates in the pediatric population. Early consideration of DBS for the super refractory cases is warranted. Complication rates are higher in children with infections and hardware-related problems being the most prominent ones.
Figure 2 summarizes the therapeutic approach to dystonia in the pediatric population.
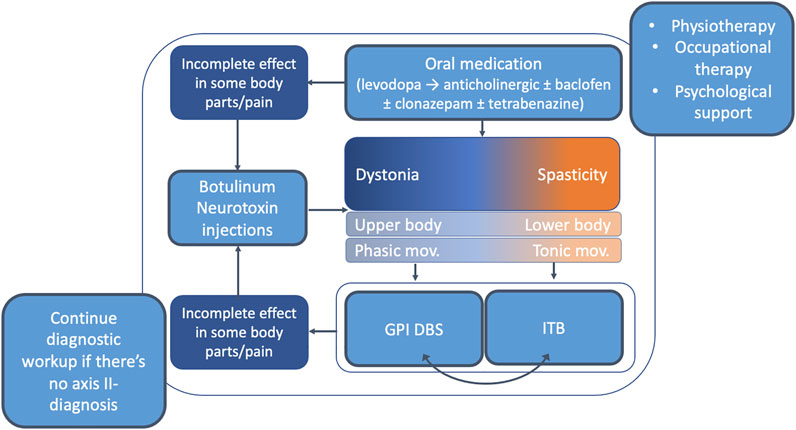
FIGURE 2. Overview of the therapeutic approach to dystonia in the pediatric population. It is recommended to enroll children with dystonia in formal physiotherapy and psychological support programs. The first-line treatment in generalized pediatric dystonia is oral medications. Levodopa should be tried in every child with idiopathic dystonia <18 years. If no significant improvement is seen on levodopa other anti-dystonia medications should be used (most robust evidence for anticholinergic treatment). Individuals who are refractory for pharmacological treatment should be considered for neurosurgical procedures including DBS and ITB. At any point during the implementation of this algorithm BoNT can be considered in cases of incomplete effect in some body parts or to improve specific issues such as pain. Abbreviations: DBS, deep brain stimulation; GPI, globus pallidus internus; ITB, intrathecal baclofen; mov, movements.
Despite the recent advances in the treatment of pediatric dystonia, there is a need for larger standardized multi-center trials as currently the treatment guidelines are largely based upon small clinical trials and expert opinion.
Author Contributions
CG: Writing—original draft, project administration AF: Conceptualization, writing—review and editing, supervision.
Funding
This work was supported by University of Toronto and University Health Network Chair in Neuromodulation to AF.
Conflict of Interest
AF: Received honoraria and/or research support from Abbott, Boston Scientific, Ceregate, Inbrain, Ipsen, Medtronic, Merz.
The remaining author declares that the research was conducted in the absence of any commercial or financial relationships that could be construed as a potential conflict of interest.
References
1. Albanese, A, Di Giovanni, M, and Lalli, S. Dystonia: Diagnosis and Management. Eur J Neurol (2019) 26(1):5–17. doi:10.1111/ENE.13762
2. Albanese, A, Bhatia, K, Bressman, SB, Delong, MR, Fahn, S, Fung, VSC, et al. Phenomenology and Classification of Dystonia: A Consensus Update. Mov Disord (2013) 28(7):863–73. doi:10.1002/MDS.25475
3. Luc, QN, and Querubin, J. Clinical Management of Dystonia in Childhood. Pediatr Drugs (2017) 19:447–61. doi:10.1007/s40272-017-0243-3
5. Mink, JW. Special Concerns in Defining, Studying, and Treating Dystonia in Children. Mov Disord (2013) 28:921–5. doi:10.1002/mds.25548
6. Van Egmond, ME, Kuiper, A, Eggink, H, Sinke, RJ, Brouwer, OF, Verschuuren-Bemelmans, CC, et al. Dystonia in Children and Adolescents: A Systematic Review and a New Diagnostic Algorithm. J Neurol Neurosurg Psychiatry (2015) 86(7):774–81. doi:10.1136/jnnp-2014-309106
7. Zech, M, Jech, R, Wagner, M, Mantel, T, Boesch, S, Nocker, M, et al. Molecular Diversity of Combined and Complex Dystonia: Insights from Diagnostic Exome Sequencing. Neurogenetics (2017) 18(4):195–205. doi:10.1007/s10048-017-0521-9
8. Saunders-Pullman, R, Fuchs, T, San Luciano, M, Raymond, D, Brashear, A, Ortega, R, et al. Heterogeneity in Primary Dystonia: Lessons from THAP1 , GNAL , and TOR1A in Amish-Mennonites. Mov Disord (2014) 29(6):812–8. doi:10.1002/mds.25818
9. Lange, LM, Junker, J, Loens, S, Baumann, H, Olschewski, L, Schaake, S, et al. Genotype-Phenotype Relations for Isolated Dystonia Genes: MDSGene Systematic Review. Mov Disord (2021) 36:1086–103. doi:10.1002/mds.28485
10. Zech, M, Boesch, S, Jochim, A, Weber, S, Meindl, T, Schormair, B, et al. Clinical Exome Sequencing in Early-Onset Generalized Dystonia and Large-Scale Resequencing Follow-Up. Mov Disord (2017) 32(4):549–59. doi:10.1002/mds.26808
11. Lohmann, K, and Klein, C. Update on the Genetics of Dystonia. Curr Neurol Neurosci Rep (2017) 17. doi:10.1007/s11910-017-0735-0
12. Fehlings, D, Brown, L, Harvey, A, Himmelmann, K, Lin, J-P, Macintosh, A, et al. Pharmacological and Neurosurgical Interventions for Managing Dystonia in Cerebral Palsy: A Systematic Review. Dev Med Child Neurol (2018) 60(4):356–66. doi:10.1111/dmcn.13652
13. Reid, SM, Carlin, JB, and Reddihough, DS. Distribution of Motor Types in Cerebral Palsy: How Do Registry Data Compare? Dev Med Child Neurol (2011) 53(3):233–8. doi:10.1111/j.1469-8749.2010.03844.x
14. Himmelmann, K, Hagberg, G, and Uvebrant, P. The Changing Panorama of Cerebral Palsy in Sweden. X. Prevalence and Origin in the Birth-Year Period 1999-2002. Acta Paediatr Int J Paediatr (2010) 99(9):1337–43. doi:10.1111/j.1651-2227.2010.01819.x
15. Stewart, K, Harvey, A, and Johnston, LM. A Systematic Review of Scales to Measure Dystonia and Choreoathetosis in Children with Dyskinetic Cerebral Palsy. Dev Med Child Neurol (2017) 59:786–95. doi:10.1111/dmcn.13452
16. Jethwa, A, Mink, J, Macarthur, C, Knights, S, Fehlings, T, and Fehlings, D. Development of the Hypertonia Assessment Tool (HAT): A Discriminative Tool for Hypertonia in Children. Dev Med Child Neurol (2010) 52(5):e83–e87. doi:10.1111/j.1469-8749.2009.03483.x
17. Rice, J, Skuza, P, Baker, F, Russo, R, and Fehlings, D. Identification and Measurement of Dystonia in Cerebral Palsy. Dev Med Child Neurol (2017) 59(12):1249–55. doi:10.1111/dmcn.13502
18. Jankovic, J. Treatment of Dystonia. Lancet Neurol (2006) 5:864–72. doi:10.1016/S1474-4422(06)70574-9
20. Marsden, CD, Marion, MH, and Quinn, N. The Treatment of Severe Dystonia in Children and Adults. J Neurol Neurosurg Psychiatry (1984) 47(11):1166–73. doi:10.1136/jnnp.47.11.1166
21. Deffains, M, and Bergman, H. Striatal Cholinergic Interneurons and Cortico-Striatal Synaptic Plasticity in Health and Disease. Mov Disord (2015) 30:1014–25. doi:10.1002/mds.26300
22. Burke, RE, Fahn, S, and Marsden, CD. Torsion Dystonia: A Double-Blind, Prospective Trial of High-Dosage Trihexyphenidyl. Neurology (1986) 36(2):160. doi:10.1212/wnl.36.2.160
23. Fahn, S. High Dosage Anticholinergic Therapy in Dystonia. Neurology (1983) 33(10):1255. doi:10.1212/wnl.33.10.1255
24. Bohn, E, Goren, K, Switzer, L, Falck-Ytter, Y, and Fehlings, D. Pharmacological and Neurosurgical Interventions for Individuals with Cerebral Palsy and Dystonia: a Systematic Review Update and Meta-analysis. Dev Med Child Neurol (2021) 63:1038–50. doi:10.1111/dmcn.14874
25. Rice, J, and Waugh, M-C. Pilot Study on Trihexyphenidyl in the Treatment of Dystonia in Children with Cerebral Palsy. J Child Neurol (2009) 24(2):176–82. doi:10.1177/0883073808322668
26. Sanger, TD, Bastian, A, Brunstrom, J, Damiano, D, Delgado, M, Dure, L, et al. Prospective Open-Label Clinical Trial of Trihexyphenidyl in Children with Secondary Dystonia Due to Cerebral Palsy. J Child Neurol (2007) 22(5):530–7. doi:10.1177/0883073807302601
27. Ben-Pazi, H. Trihexyphenidyl Improves Motor Function in Children with Dystonic Cerebral Palsy: A Retrospective Analysis. J Child Neurol (2011) 26(7):810–6. doi:10.1177/0883073810392582
28. Carranza-Del Rio, J, Clegg, NJ, Moore, A, and Delgado, MR. Use of Trihexyphenidyl in Children with Cerebral Palsy. Pediatr Neurol (2011) 44(3):202–6. doi:10.1016/j.pediatrneurol.2010.09.008
29. Battini, R, Casarano, M, Sgandurra, G, Olivieri, I, Di Pietro, R, Romeo, DM, et al. Responsiveness of the MD-Childhood Rating Scale in Dyskinetic Cerebral Palsy Patients Undergoing Anticholinergic Treatment. Eur J Paediatric Neurol (2014) 18(6):698–703. doi:10.1016/j.ejpn.2014.06.004
30. Hoon, AH, Freese, PO, Reinhardt, EM, Wilson, MA, Lawrie, WT, Harryman, SE, et al. Age-Dependent Effects of Trihexyphenidyl in Extrapyramidal Cerebral Palsy. Pediatr Neurol (2001) 25(1):55–8. doi:10.1016/S0887-8994(01)00287-9
31. Pringsheim, T, Doja, A, Belanger, S, Patten, S, Casselman, L, Davidson, J, et al. Treatment Recommendations for Extrapyramidal Side Effects Associated with Second-Generation Antipsychotic Use in Children and Youth. Paediatrics Child Health (2011) 16:590–8. doi:10.1093/pch/16.9.590
32. Davidoff, RA. Antispasticity Drugs: Mechanisms of Action. Ann Neurol (1985) 17(2):107–16. doi:10.1002/ana.410170202
33. Balash, Y, and Giladi, N. Efficacy of Pharmacological Treatment of Dystonia: Evidence-Based Review Including Meta-Analysis of the Effect of Botulinum Toxin and Other Cure Options. Eur J Neurol (2004) 11:361–70. doi:10.1111/j.1468-1331.2004.00845.x
34. Lumsden, DE, Kaminska, M, Tomlin, S, and Lin, J-P. Medication Use in Childhood Dystonia. Eur J Paediatric Neurol (2016) 20(4):625–9. doi:10.1016/j.ejpn.2016.02.003
35. Greene, PE, and Fahn, S. Baclofen in the Treatment of Idiopathic Dystonia in Children. Mov Disord (1992) 7(1):48–52. doi:10.1002/mds.870070109
36. Greene, P, Shale, H, and Fahn, S. Analysis of Open-Label Trials in Torsion Dystonia Using High Dosages of Anticholinergics and Other Drugs. Mov Disord (1988) 3(1):46–60. doi:10.1002/mds.870030107
37. Wick, JY. The History of Benzodiazepines. The Consultant Pharmacist (2013) 28:538–48. doi:10.4140/TCP.n.2013.538
38. Chuang, C, Fahn, S, and Frucht, SJ. The Natural History and Treatment of Acquired Hemidystonia: Report of 33 Cases and Review of the Literature. J Neurol Neurosurg Psychiatry (2002) 72(1):59–67. doi:10.1136/jnnp.72.1.59
39. Kinugawa, K, Vidailhet, M, Clot, F, Apartis, E, Grabli, D, and RozeMyoclonus-Dystonia, E. Myoclonus-dystonia: An Update. Mov Disord (2009) 24:479–89. doi:10.1002/mds.22425
40. Ghosh, D, and Indulkar, S. Primary Myoclonus-Dystonia. J Child Neurol (2013) 28(11):1418–22. doi:10.1177/0883073813488677
41. Fearon, C, Peall, KJ, Vidailhet, M, and Fasano, A. Medical Management of Myoclonus-Dystonia and Implications for Underlying Pathophysiology. Parkinsonism Relat Disord (2020) 77:48–56. doi:10.1016/j.parkreldis.2020.06.016
42. Pons, R. The Phenotypic Spectrum of Paediatric Neurotransmitter Diseases and Infantile Parkinsonism. J Inherit Metab Dis (2009) 32:321–32. doi:10.1007/s10545-008-1007-6
43. Segawa, M. Dopa-Responsive Dystonia. Handb Clin Neurol (2011) 100:539–57. doi:10.1016/B978-0-444-52014-2.00039-2
44. Malek, N, Fletcher, N, and Newman, E. Diagnosing Dopamine-Responsive Dystonias. Pract Neurol (2015) 15:340–5. doi:10.1136/practneurol-2015-001101
45. Pozin, I, Bdolah-Abram, T, and Ben-Pazi, H. Levodopa Does Not Improve Function in Individuals with Dystonic Cerebral Palsy. J Child Neurol (2014) 29(4):534–7. doi:10.1177/0883073812473645
46. Willemsen, MA, Verbeek, MM, Kamsteeg, E-J, De Rijk-Van Andel, JF, Aeby, A, Blau, N, et al. Tyrosine Hydroxylase Deficiency: A Treatable Disorder of Brain Catecholamine Biosynthesis. Brain (2010) 133(6):1810–22. doi:10.1093/brain/awq087
47. Wijemanne, S, and Jankovic, J. Dopa-responsive Dystonia-Clinical and Genetic Heterogeneity. Nat Rev Neurol (2015) 11:414–24. doi:10.1038/nrneurol.2015.86
48. Salles, PA, Terán-Jimenez, M, Vidal-Santoro, A, Chaná-Cuevas, P, Kauffman, M, and Espay, AJ. Recognizing Atypical Dopa-Responsive Dystonia and its Mimics. Neurol Clin Pract (2021) 11(6):10.1212/CPJ.0000000000001125. doi:10.1212/cpj.0000000000001125
49. Jankovic, J, and Clarence-Smith, K. Tetrabenazine for the Treatment of Chorea and Other Hyperkinetic Movement Disorders. Expert Rev Neurotherapeutics (2011) 11(11):1509–23. doi:10.1586/ern.11.149
50. Fasano, A, and BentivoglioTetrabenazine, AR. Tetrabenazine. Expert Opin Pharmacother (2009) 10:2883–96. doi:10.1517/14656560903386292
51. Paleacu, D, Giladi, N, Moore, O, Stern, A, Honigman, S, and Badarny, S. Tetrabenazine Treatment in Movement Disorders. Clin Neuropharmacology (2004) 27(5):230–3. doi:10.1097/01.wnf.0000136892.24629.96
52. Jankovic, J, and Beach, J. Long-Term Effects of Tetrabenazine in Hyperkinetic Movement Disorders. Neurology (1997) 48(2):358–62. doi:10.1212/WNL.48.2.358
53. Jain, S, Greene, PE, and Frucht, SJ. Tetrabenazine Therapy of Pediatric Hyperkinetic Movement Disorders. Mov Disord (2006) 21:1966–72. doi:10.1002/mds.21063
54. Jankovic, J, and Orman, J. Tetrabenazine Therapy of Dystonia, Chorea, Tics, and Other Dyskinesias. Neurology (1988) 38(3):391. doi:10.1212/wnl.38.3.391
55. Scalise, R, Sgandurra, G, Menici, V, Capodagli, N, Di Pietro, R, Romeo, DM, et al. A Retrospective Longitudinal Study in a Cohort of Children with Dyskinetic Cerebral Palsy Treated with Tetrabenazine. Front Neurol (2021) 12:12. doi:10.3389/fneur.2021.612429
56. Khorassani, F, Luther, K, and Talreja, O. Valbenazine and Deutetrabenazine: Vesicular Monoamine Transporter 2 Inhibitors for Tardive Dyskinesia. Am J Heal Pharm (2020) 77(3):167–74. doi:10.1093/ajhp/zxz299
57. Patel, RS, Mansuri, Z, Motiwala, F, Saeed, H, Jannareddy, N, Patel, H, et al. A Systematic Review on Treatment of Tardive Dyskinesia with Valbenazine and Deutetrabenazine. Ther Adv Psychopharmacol (2019) 9:204512531984788. doi:10.1177/2045125319847882
58. Sätilä, H. Over 25 Years of Pediatric Botulinum Toxin Treatments: What Have We Learned from Injection Techniques, Doses, Dilutions, and Recovery of Repeated Injections? Toxins (2020) 12:440. doi:10.3390/toxins12070440
59. Pirazzini, M, Rossetto, O, Eleopra, R, and Montecucco, C. Botulinum Neurotoxins: Biology, Pharmacology, and Toxicology. Pharmacol Rev (2017) 69:200–35. doi:10.1124/pr.116.012658
60. Anandan, C, and Jankovic, J. Botulinum Toxin in Movement Disorders: An Update. Toxins (2021) 13:42. doi:10.3390/toxins13010042
61. Hallett, M, Albanese, A, Dressler, D, Segal, KR, Simpson, DM, Truong, D, et al. Evidence-based Review and Assessment of Botulinum Neurotoxin for the Treatment of Movement Disorders. Toxicon (2013) 67:94–114. doi:10.1016/j.toxicon.2012.12.004
62. Simpson, DM, Hallett, M, Ashman, EJ, Comella, CL, Green, MW, Gronseth, GS, et al. Practice Guideline Update Summary: Botulinum Neurotoxin for the Treatment of Blepharospasm, Cervical Dystonia, Adult Spasticity, and Headache. Neurology (2016) 86(19):1818–26. doi:10.1212/WNL.0000000000002560
63. Zakin, E, and Simpson, D. Evidence on Botulinum Toxin in Selected Disorders. Toxicon (2018) 147:134–40. doi:10.1016/j.toxicon.2018.01.019
64. Albanese, A, Asmus, F, Bhatia, KP, Elia, AE, Elibol, B, Filippini, G, et al. EFNS Guidelines on Diagnosis and Treatment of Primary Dystonias. Eur J Neurol (2011) 18(1):5–18. doi:10.1111/j.1468-1331.2010.03042.x
65. Jinnah, HA. Medical and Surgical Treatments for Dystonia. Neurol Clin (2020) 38:325–48. doi:10.1016/j.ncl.2020.01.003
66. Yi, Y, Kim, K, Yi, Y, Choi, Y-A, Leigh, J-H, and Bang, M. Botulinum Toxin Type a Injection for Cervical Dystonia in Adults with Dyskinetic Cerebral Palsy. Toxins (2018) 10(5):203. doi:10.3390/toxins10050203
67. Arens, LJ. Experience with Botulinum Toxin in the Treatment of Cerebral Palsy. South Afr Med. J. (1997) 87(8):1001–3.
68. Sanger, TD, Kukke, SN, and Sherman-Levine, S. Botulinum Toxin Type B Improves the Speed of Reaching in Children with Cerebral Palsy and Arm Dystonia: An Open-Label, Dose-Escalation Pilot Study. J Child Neurol (2007) 22(1):116–22. doi:10.1177/0883073807299975
69. Lundy, CT, Doherty, GM, and Fairhurst, CB. Botulinum Toxin Type A Injections Can Be an Effective Treatment for Pain in Children with Hip Spasms and Cerebral Palsy. Dev Med Child Neurol (2009) 51(9):705–10. doi:10.1111/j.1469-8749.2009.03315.x
70. Kitai, Y, Hirai, S, Okuyama, N, Hirotsune, M, Nishimoto, S, Mizutani, S, et al. A Questionnaire Survey on the Efficacy of Various Treatments for Dyskinetic Cerebral Palsy Due to Preterm Bilirubin Encephalopathy. Brain Development (2020) 42(4):322–8. doi:10.1016/j.braindev.2020.01.006
71. Thorley, M, Lannin, N, Cusick, A, Novak, I, and Boyd, R. Construct Validity of the Quality of Upper Extremity Skills Test for Children with Cerebral Palsy. Dev Med Child Neurol (2012) 54(11):1037–43. doi:10.1111/j.1469-8749.2012.04368.x
72. Spiegel, LL, Ostrem, JL, and Bledsoe, IO. FDA Approvals and Consensus Guidelines for Botulinum Toxins in the Treatment of Dystonia. Toxins (2020) 12:332. doi:10.3390/toxins12050332
73. Scaglione, F. Conversion Ratio between Botox, Dysport, and Xeomin in Clinical Practice. Toxins (2016) 8:65. doi:10.3390/toxins8030065
74. Ramirez-Castaneda, J, Jankovic, J, Comella, C, Dashtipour, K, Fernandez, HH, and Mari, Z. Diffusion, Spread, and Migration of Botulinum Toxin. Mov Disord (2013) 28:1775–83. doi:10.1002/mds.25582
75. Lee, JH, Sung, IY, Yoo, JY, Park, EH, and Park, SR. Effects of Different Dilutions of Botulinum Toxin Type A Treatment for Children with Cerebral Palsy with Spastic Ankle Plantarflexor: A Randomized Controlled Trial. J Rehabil Med (2009) 41:740–5. doi:10.2340/16501977-0418
76. Hu, G-C, Chuang, Y-C, Liu, J-P, Chien, K-L, Chen, Y-M, and Chen, Y-F. Botulinum Toxin (Dysport) Treatment of the Spastic Gastrocnemius Muscle in Children with Cerebral Palsy: A Randomized Trial Comparing Two Injection Volumes. Clin Rehabil (2009) 23(1):64–71. doi:10.1177/0269215508097861
77. Lee, L-R, Chuang, Y-C, Yang, B-J, Hsu, M-J, and Liu, Y-H. Botulinum Toxin for Lower Limb Spasticity in Children with Cerebral Palsy. Am J Phys Med Rehabil (2004) 83(10):766–73. doi:10.1097/01.PHM.0000137314.38806.95
78. Fehlings, D, Narayanan, U, Andersen, J, Beauchamp, R, Gorter, JW, Kawamura, A, et al. Botulinum Toxin-A Use in Paediatric Hypertonia: Canadian Practice Patterns. Can J Neurol Sci (2012) 39:508–15. doi:10.1017/S0317167100014049
79. Heinen, F, Desloovere, K, Schroeder, AS, Berweck, S, Borggraefe, I, van Campenhout, A, et al. The Updated European Consensus 2009 on the Use of Botulinum Toxin for Children with Cerebral Palsy. Eur J Paediatric Neurol (2010) 14:45–66. doi:10.1016/j.ejpn.2009.09.005
80. Carraro, E, Trevisi, E, and Martinuzzi, A. Safety Profile of Incobotulinum Toxin A [Xeomin] in Gastrocnemious Muscles Injections in Children with Cerebral Palsy: Randomized Double-Blind Clinical Trial. Eur J Paediatric Neurol (2016) 20(4):532–7. doi:10.1016/j.ejpn.2016.04.008
81. Russman, BS, Tilton, A, and Gormley, ME. Cerebral Palsy: a Rational Approach to a Treatment Protocol, and the Role of Botulinum Toxin in Treatment. Muscle Nerve Suppl (1997) 6:S181–S193.
82. Fehlings, D, Novak, I, Berweck, S, Hoare, B, Stott, NS, and Russo, RN. Botulinum Toxin Assessment, Intervention and Follow-Up for Paediatric Upper Limb Hypertonicity: International Consensus Statement. Eur J Neurol (2010) 17(Suppl. 2):38–56. doi:10.1111/J.1468-1331.2010.03127.X
83. Kawamura, A, Campbell, K, Lam-Damji, S, and Fehlings, D. A Randomized Controlled Trial Comparing Botulinum Toxin A Dosage in the Upper Extremity of Children with Spasticity. Dev Med Child Neurol (2007) 49(5):331–7. doi:10.1111/j.1469-8749.2007.00331.x
84. Albavera-Hernández, C, Rodríguez, JM, and Idrovo, AJ. Safety of Botulinum Toxin Type A Among Children with Spasticity Secondary to Cerebral Palsy: A Systematic Review of Randomized Clinical Trials. Clin Rehabil (2009) 23:394–407. doi:10.1177/0269215508099860
85. Dralle, D, Müller, H, Zierski, J, and Klug, N. Intrathecal Baclofen for Spasticity. The Lancet (1985) 326:1003. doi:10.1016/S0140-6736(85)90541-0
86. Albright, AL, Cervi, A, and Singletary, J. Intrathecal Baclofen for Spasticity in Cerebral Palsy. JAMA J Am Med Assoc (1991) 265(11):1418–22. doi:10.1001/jama.1991.0346011008402910.1001/jama.265.11.1418
87. Winter, G, Beni-Adani, L, and Ben-Pazi, H. Intrathecal Baclofen Therapy-Practical Approach: Clinical Benefits and Complication Management. J Child Neurol (2018) 33:734–41. doi:10.1177/0883073818785074
88. Albright, AL, Thompson, K, Carlos, S, and Minnigh, MB. Cerebrospinal Fluid Baclofen Concentrations in Patients Undergoing Continuous Intrathecal Baclofen Therapy. Dev Med Child Neurol (2007) 49(6):423–5. doi:10.1111/j.1469-8749.2007.00423.x
89. Kyriagis, M, Grattan-Smith, P, Scheinberg, A, Teo, C, Nakaji, N, and Waugh, M. Status Dystonicus and Hallervorden-Spatz Disease: Treatment with Intrathecal Baclofen and Pallidotomy. J Paediatr Child Health (2004) 40(5–6):322–5. doi:10.1111/j.1440-1754.2004.00374.x
90. Mariotti, P, Fasano, A, Contarino, MF, Della Marca, G, Piastra, M, Genovese, O, et al. Management of Status Dystonicus: Our Experience and Review of the Literature. Mov Disord (2007) 22(7):963–8. doi:10.1002/mds.21471
91. Jinnah, HA, Visser, JE, Harris, JC, Verdu, A, Larovere, L, Ceballos-Picot, I, et al. Delineation of the Motor Disorder of Lesch-Nyhan Disease. Brain (2006) 129(5):1201–17. doi:10.1093/brain/awl056
92. Albright, AL, Barry, MJ, Shafron, DH, and Ferson, SS. Intrathecal Baclofen for Generalized Dystonia. Dev Med Child Neurol (2001) 43(10):652. doi:10.1017/s0012162201001190
93. Kirshblum, S. Treatment Alternatives for Spinal Cord Injury Related Spasticity. J Spinal Cord Med (1999) 22(3):199–217. doi:10.1080/10790268.1999.11719570
94. Albanese, A, Barnes, MP, Bhatia, KP, Fernandez-Alvarez, E, Filippini, G, Gasser, T, et al. A Systematic Review on the Diagnosis and Treatment of Primary (Idiopathic) Dystonia and Dystonia Plus Syndromes: Report of an EFNS/MDS-ES Task Force. Eur J Neurol (2006) 13:433–44. doi:10.1111/j.1468-1331.2006.01537.x
95. Borowski, A, Littleton, AG, Borkhuu, B, Presedo, A, Shah, S, Dabney, KW, et al. Complications of Intrathecal Baclofen Pump Therapy in Pediatric Patients. J Pediatr Orthop January (2010) 30:76–81. doi:10.1097/BPO.0b013e3181c6b257
96. Motta, F, and Antonello, CE. Analysis of Complications in 430 Consecutive Pediatric Patients Treated with Intrathecal Baclofen Therapy: 14-Year Experience. Ped (2014) 13(3):301–6. doi:10.3171/2013.11.PEDS13253
97. Dario, A, and Tomei, G. A Benefit-Risk Assessment of Baclofen in Severe Spinal Spasticity. Drug Saf (2004) 27:799–818. doi:10.2165/00002018-200427110-00004
98. Romito, JW, Turner, ER, Rosener, JA, Coldiron, L, Udipi, A, Nohrn, L, et al. Baclofen Therapeutics, Toxicity, and Withdrawal: A Narrative Review. SAGE Open Med (2021) 9:205031212110221. doi:10.1177/20503121211022197
99. Zuckerbraun, NS, Ferson, SS, Albright, AL, and Vogeley, E. Intrathecal Baclofen Withdrawal. Pediatr Emerg Care (2004) 2(11):759–64. doi:10.1097/01.pec.0000144919.08619.95
100. Shirley, KW, Kothare, S, Piatt, JH, and Adirim, TA. Intrathecal Baclofen Overdose and Withdrawal. Pediatr Emerg Care (2006) 22(4):258–61. doi:10.1097/01.pec.0000210175.40763.c5
101. Coffey, RJ, Edgar, TS, Francisco, GE, Graziani, V, Meythaler, JM, Ridgely, PM, et al. Abrupt Withdrawal from Intrathecal Baclofen: Recognition and Management of a Potentially Life-Threatening Syndrome. Arch Phys Med Rehabil (2002) 83(6):735–41. doi:10.1053/apmr.2002.32820
102. Coubes, , Echenne, , RoubertieCoubes, P, Echenne, B, Roubertie, A, Vayssiere, N, et al. Treatment of Early-Onset Generalized Dystonia by Chronic Bilateral Stimulation of the Internal Globus Pallidus. About a Case. Neurosurgery (1999) 45:139–44.
103. Vidailhet, M, Jutras, M-F, Grabli, D, and Roze, E. Deep Brain Stimulation for Dystonia. J Neurol Neurosurg Psychiatry (2013) 84:1029–42. doi:10.1136/jnnp-2011-301714
104. Air, EL, Ostrem, JL, Sanger, TD, and Starr, PA. Deep Brain Stimulation in Children: Experience and Technical Pearls. Ped (2011) 8(6):566–74. doi:10.3171/2011.8.PEDS11153
105. Paff, M, Loh, A, Sarica, C, Lozano, AM, and Fasano, A. Update on Current Technologies for Deep Brain Stimulation in Parkinson's Disease. Jmd (2020) 13:185–98. doi:10.14802/jmd.20052
106. Fasano, A, Gorodetsky, C, Paul, D, Germann, J, Loh, A, Yan, H, et al. Local Field Potential-Based Programming: A Proof-Of-Concept Pilot Study. Neuromodulation: Technology Neural Interf (2022) 25:271–5. doi:10.1111/ner.13520
107. Elkaim, L, Alotaibi, N, Sigal, A, Alotaibi, HM, Lipsman, N, Kalia, SK, et al. Deep Brain Stimulation for Pediatric Dystonia: a Meta-analysis with Individual Participant Data. Dev Med Child Neurol (2019) 61:49–56. doi:10.1111/dmcn.14063
108. Magown, P, Andrade, RA, Soroceanu, A, and Kiss, ZHT. Deep Brain Stimulation Parameters for Dystonia: A Systematic Review. Parkinsonism Relat Disord (2018) 54:9–16. doi:10.1016/j.parkreldis.2018.04.017
109. Hale, AT, Monsour, MA, Rolston, JD, Naftel, RP, and Englot, DJ. Deep Brain Stimulation in Pediatric Dystonia: a Systematic Review. Neurosurg Rev (2020) 43:873–80. doi:10.1007/s10143-018-1047-9
110. Benato, A, Carecchio, M, Burlina, A, Paoloni, F, Sartori, S, Nosadini, M, et al. Long-Term Effect of Subthalamic and Pallidal Deep Brain Stimulation for Status Dystonicus in Children with Methylmalonic Acidemia and GNAO1 Mutation. J Neural Transm (2019) 126(6):739–57. doi:10.1007/s00702-019-02010-2
111. Barbosa, BJAP, Carra, RB, Duarte, KP, Godinho, F, de Andrade, DC, Teixeira, MJ, et al. Bilateral Subthalamic Nucleus Stimulation in Refractory Status Dystonicus. J Neurol Sci (2018) 388:159–61. doi:10.1016/j.jns.2018.03.007
112. Xu, W, Li, H, Zhang, C, Sun, B, Wu, Y, and Li, D. Subthalamic Nucleus Stimulation in Pediatric Isolated Dystonia: A 10-Year Follow-Up. Can J Neurol Sci (2020) 47(3):328–35. doi:10.1017/cjn.2020.32
113. Sanger, TD, Liker, M, Arguelles, E, Deshpande, R, Maskooki, A, Ferman, D, et al. Pediatric Deep Brain Stimulation Using Awake Recording and Stimulation for Target Selection in an Inpatient Neuromodulation Monitoring Unit. Brain Sci (2018) 8(7):135. doi:10.3390/brainsci8070135
114. Wolf, ME, Blahak, C, Saryyeva, A, Schrader, C, and Krauss, JK. Deep Brain Stimulation for Dystonia-Choreoathetosis in Cerebral Palsy: Pallidal versus Thalamic Stimulation. Parkinsonism Relat Disord (2019) 63:209–12. doi:10.1016/j.parkreldis.2019.01.029
115. San Luciano, M, Robichaux-Viehoever, A, Dodenhoff, KA, Gittings, ML, Viser, AC, Racine, CA, et al. Thalamic Deep Brain Stimulation for Acquired Dystonia in Children and Young Adults: A Phase 1 Clinical Trial. J Neurosurg Pediatr (2021) 27(2):203–12. doi:10.3171/2020.7.PEDS20348
116. Albanese, A, Sorbo, FD, Comella, C, Jinnah, HA, Mink, JW, Post, B, et al. Dystonia Rating Scales: Critique and Recommendations. Mov Disord (2013) 28:874–83. doi:10.1002/mds.25579
117. Burke, RE, Fahn, S, Marsden, CD, Bressman, SB, Moskowitz, C, and Friedman, J. Validity and Reliability of a Rating Scale for the Primary Torsion Dystonias. Neurology (1985) 35(1):73. doi:10.1212/wnl.35.1.73
118. Kuiper, MJ, Vrijenhoek, L, Brandsma, R, Lunsing, RJ, Burger, H, Eggink, H, et al. The Burke-Fahn-Marsden Dystonia Rating Scale Is Age-dependent in Healthy Children. Mov Disord Clin Pract (2016) 3(6):580–6. doi:10.1002/mdc3.12339
119. McColl, MA, Law, M, Baptiste, S, Pollock, N, Carswell, A, and Polatajko, HJ. Targeted Applications of the Canadian Occupational Performance Measure. Can J Occup Ther (2005) 72(5):298–300. doi:10.1177/000841740507200506
120. Gimeno, H, Tustin, K, Lumsden, D, Ashkan, K, Selway, R, and Lin, J-P. Evaluation of Functional Goal Outcomes Using the Canadian Occupational Performance Measure (COPM) Following Deep Brain Stimulation (DBS) in Childhood Dystonia. Eur J Paediatric Neurol (2014) 18(3):308–16. doi:10.1016/j.ejpn.2013.12.010
121. Gimeno, H, Gordon, A, Tustin, K, and Lin, J-P. Functional Priorities in Daily Life for Children and Young People with Dystonic Movement Disorders and Their Families. Eur J Paediatric Neurol (2013) 17(2):161–8. doi:10.1016/j.ejpn.2012.07.007
122. Pintér, D, Janszky, J, and Kovács, N. Minimal Clinically Important Differences for Burke-Fahn-Marsden Dystonia Rating Scale and 36-Item Short-Form Health Survey. Mov Disord (2020) 35(7):1218–23. doi:10.1002/mds.28057
123. Barry, MJ, VanSwearingen, JM, and Albright, AL. Reliability and Responsiveness of the Barry-Albright Dystonia Scale. Dev Med Child Neurol (1999) 41(6):404–11. doi:10.1017/S0012162299000870
124. Battini, R, Sgandurra, G, Petacchi, E, Guzzetta, A, Di Pietro, R, Giannini, MT, et al. Movement Disorder-Childhood Rating Scale: Reliability and Validity. Pediatr Neurol (2008) 39(4):259–65. doi:10.1016/j.pediatrneurol.2008.07.002
125. Battini, R, Guzzetta, A, Sgandurra, G, Di Pietro, R, Petacchi, E, Mercuri, E, et al. Scale for Evaluation of Movement Disorders in the First Three Years of Life. Pediatr Neurol (2009) 40(4):258–64. doi:10.1016/j.pediatrneurol.2008.11.003
126. Mills, KA, Starr, PA, and Ostrem, JL. Neuromodulation for Dystonia. Neurosurg Clin North America (2014) 25:59–75. doi:10.1016/j.nec.2013.08.014
127. Lumsden, DE, Kaminska, M, Gimeno, H, Tustin, K, Baker, L, Perides, S, et al. Proportion of Life Lived with Dystonia Inversely Correlates with Response to Pallidal Deep Brain Stimulation in Both Primary and Secondary Childhood Dystonia. Dev Med Child Neurol (2013) 55(6):567–74. doi:10.1111/dmcn.12117
128. Vasques, X, Cif, L, Gonzalez, V, Nicholson, C, and Coubes, P. Factors Predicting Improvement in Primary Generalized Dystonia Treated by Pallidal Deep Brain Stimulation. Mov Disord (2009) 24(6):846–53. doi:10.1002/mds.22433
129. Jinnah, HA, Alterman, R, Klein, C, Krauss, JK, Moro, E, Vidailhet, M, et al. Deep Brain Stimulation for Dystonia: a Novel Perspective on the Value of Genetic Testing. J Neural Transm (2017) 124:417–30. doi:10.1007/s00702-016-1656-9
130. Cif, L, Demailly, D, Lin, J-P, Barwick, KE, Sa, M, Abela, L, et al. KMT2B-Related Disorders: Expansion of the Phenotypic Spectrum and Long-Term Efficacy of Deep Brain Stimulation. Brain (2020) 143(11):3242–61. doi:10.1093/brain/awaa304
131. Piedimonte, F, Andreani, JC, Piedimonte, L, Micheli, F, Graff, P, and Bacaro, V. Remarkable Clinical Improvement with Bilateral Globus Pallidus Internus Deep Brain Stimulation in a Case of Lesch-Nyhan Disease: Five-Year Follow-Up. Neuromodulation: Technology Neural Interf (2015) 18(2):118–22. doi:10.1111/ner.12261
132. Tambirajoo, K, Furlanetti, L, Hasegawa, H, Raslan, A, Gimeno, H, Lin, J-P, et al. Deep Brain Stimulation of the Internal Pallidum in Lesch-Nyhan Syndrome: Clinical Outcomes and Connectivity Analysis. Neuromodulation: Technology Neural Interf (2021) 24(2):380–91. doi:10.1111/ner.13217
133. Svetel, M, Tomić, A, Dragašević, N, Petrović, I, Kresojević, N, Jech, R, et al. Clinical Course of Patients with Pantothenate Kinase-Associated Neurodegeneration (PKAN) before and after DBS Surgery. J Neurol (2019) 266(12):2962–9. doi:10.1007/s00415-019-09499-3
134. Garcia-Ruiz, PJ, Ayerbe, J, Vela Desojo, L, Feliz, CE, and del Val Fernandez, J. Deep Brain Stimulation for Pantothenate Kinase-Associated Neurodegeneration. Case Rep Neurol Med (2015) 2015:1–3. doi:10.1155/2015/245735
135. Liu, Z, Liu, Y, Yang, Y, Wang, L, Dou, W, Guo, J, et al. Subthalamic Nuclei Stimulation in Patients with Pantothenate Kinase-Associated Neurodegeneration (PKAN). Neuromodulation: Technology Neural Interf (2017) 20(5):484–91. doi:10.1111/ner.12549
136. Bronte-Stewart, H, Taira, T, Valldeoriola, F, Merello, M, Marks, WJ, Albanese, A, et al. Inclusion and Exclusion Criteria for DBS in Dystonia. Mov Disord (2011) 26(Suppl. 1):S5–S16. doi:10.1002/mds.23482
137. Yianni, J, Wang, SY, Liu, X, Bain, PG, Nandi, D, Gregory, R, et al. A Dominant Bursting Electromyograph Pattern in Dystonic Conditions Predicts an Early Response to Pallidal Stimulation. J Clin Neurosci (2006) 13(7):738–46. doi:10.1016/j.jocn.2005.07.022
138. McClelland, VM, Fialho, D, Flexney-Briscoe, D, Holder, GE, Elze, MC, Gimeno, H, et al. Somatosensory Evoked Potentials and Central Motor Conduction Times in Children with Dystonia and Their Correlation with Outcomes from Deep Brain Stimulation of the Globus Pallidus Internus. Clin Neurophysiol (2018) 129(2):473–86. doi:10.1016/j.clinph.2017.11.017
139. Nomura, Y, and Segawa, M. Genetics and Pathophysiology of Primary Dystonia with Special Emphasis on Dyt1 and Dyt5. Semin Neurol (2014) 34(3):306–11. doi:10.1055/s-0034-1386768
140. Andrews, C, Aviles-Olmos, I, Hariz, M, and Foltynie, T. Which Patients with Dystonia Benefit from Deep Brain Stimulation? A Metaregression of Individual Patient Outcomes. J Neurol Neurosurg Psychiatry (2010) 81:1383–9. doi:10.1136/jnnp.2010.207993
141. Peall, KJ, Kurian, MA, Wardle, M, Waite, AJ, Hedderly, T, Lin, J-P, et al. SGCE and Myoclonus Dystonia: Motor Characteristics, Diagnostic Criteria and Clinical Predictors of Genotype. J Neurol (2014) 261(12):2296–304. doi:10.1007/s00415-014-7488-3
142. Roze, E, Lang, AE, and Vidailhet, M. Myoclonus-dystonia: Classification, Phenomenology, Pathogenesis, and Treatment. Curr Opin Neurol August (2018) 31:484–90. doi:10.1097/WCO.0000000000000577
143. Zech, M, Boesch, S, Maier, EM, Borggraefe, I, Vill, K, Laccone, F, et al. Haploinsufficiency of KMT2B , Encoding the Lysine-specific Histone Methyltransferase 2B, Results in Early-Onset Generalized Dystonia. Am J Hum Genet (2016) 99(6):1377–87. doi:10.1016/j.ajhg.2016.10.010
144. Meyer, E, Carss, KJ, Carss, KJ, Rankin, J, Nichols, JME, Grozeva, D, et al. Mutations in the Histone Methyltransferase Gene KMT2B Cause Complex Early-Onset Dystonia. Nat Genet (2017) 49(2):223–37. doi:10.1038/ng.3740
145. Dietel, T, Evers, C, Hinderhofer, K, Korinthenberg, R, Ezzo, D, Bönnemann, C, et al. Expanding Phenotype of De Novo Mutations in GNAO1: Four New Cases and Review of Literature. Neuropediatrics (2017) 48(5):371–7. doi:10.1055/s-0037-1603977
146. Schirinzi, T, Garone, G, Travaglini, L, Vasco, G, Galosi, S, Rios, L, et al. Phenomenology and Clinical Course of Movement Disorder in GNAO1 Variants: Results from an Analytical Review. Parkinsonism Relat Disord (2019) 61:19–25. doi:10.1016/j.parkreldis.2018.11.019
147. Koy, A, Cirak, S, Gonzalez, V, Becker, K, Roujeau, T, Milesi, C, et al. Deep Brain Stimulation Is Effective in Pediatric Patients with GNAO1 Associated Severe Hyperkinesia. J Neurol Sci (2018) 391(February):31–9. doi:10.1016/j.jns.2018.05.018
148. Fuchs, T, Gavarini, S, Saunders-Pullman, R, Raymond, D, Ehrlich, ME, Bressman, SB, et al. Mutations in the THAP1 Gene Are Responsible for DYT6 Primary Torsion Dystonia. Nat Genet (2009) 41(3):286–8. doi:10.1038/ng.304
149. LeDoux, MS, Xiao, J, Rudzińska, M, Bastian, RW, Wszolek, ZK, Van Gerpen, JA, et al. Genotype-phenotype Correlations in THAP1 Dystonia: Molecular Foundations and Description of New Cases. Parkinsonism Relat Disord (2012) 18:414–25. doi:10.1016/j.parkreldis.2012.02.001
150. Bressman, SB, Raymond, D, Fuchs, T, Heiman, GA, Ozelius, LJ, and Saunders-Pullman, R. Mutations in THAP1 (DYT6) in Early-Onset Dystonia: A Genetic Screening Study. Lancet Neurol (2009) 8(5):441–6. doi:10.1016/S1474-4422(09)70081-X
151. Groen, JL, Ritz, K, Contarino, MF, Van De Warrenburg, BP, Aramideh, M, Foncke, EM, et al. DYT6 Dystonia: Mutation Screening, Phenotype, and Response to Deep Brain Stimulation. Mov Disord (2010) 25(14):2420–7. doi:10.1002/mds.23285
152. Zittel, S, Moll, CKE, Brüggemann, N, Tadic, V, Hamel, W, Kasten, M, et al. Clinical Neuroimaging and Electrophysiological Assessment of Three DYT6 Dystonia Families. Mov Disord (2010) 25(14):2405–12. doi:10.1002/mds.23279
153. Danielsson, A, Carecchio, M, Cif, L, Koy, A, Lin, J-P, Solders, G, et al. Pallidal Deep Brain Stimulation in DYT6 Dystonia: Clinical Outcome and Predictive Factors for Motor Improvement. Jcm (2019) 8(12):2163. doi:10.3390/jcm8122163
154. Visser, JE, Cotton, AC, Schretlen, DJ, Bloch, J, Tedroff, K, Schechtmann, G, et al. Deep Brain Stimulation in Lesch-Nyhan Disease: Outcomes from the Patient's Perspective. Dev Med Child Neurol (2021) 63(8):963–8. doi:10.1111/dmcn.14852
155. Hayflick, SJ. Neurodegeneration with Brain Iron Accumulation: From Genes to Pathogenesis. Semin Pediatr Neurol (2006) 13:182–5. doi:10.1016/j.spen.2006.08.007
156. Hogarth, P, Kurian, MA, Gregory, A, Csányi, B, Zagustin, T, Kmiec, T, et al. Consensus Clinical Management Guideline for Pantothenate Kinase-Associated Neurodegeneration (PKAN). Mol Genet Metab (2017) 120(3):278–87. doi:10.1016/j.ymgme.2016.11.004
157. Timmermann, L, Pauls, KAM, Wieland, K, Jech, R, Kurlemann, G, Sharma, N, et al. Dystonia in Neurodegeneration with Brain Iron Accumulation: Outcome of Bilateral Pallidal Stimulation. Brain (2010) 133(3):701–12. doi:10.1093/brain/awq022
158. Ruiz-Lopez, M, and Fasano, A. Rethinking Status Dystonicus. Mov Disord (2017) 32:1667–76. doi:10.1002/mds.27207
159. Ruiz-Lopez, M, and Fasano, A. Letter in Response to "Rethinking Status Dystonicus - A welcome Start to a Challenging Problem". Movement Disord (2018) 33:345. doi:10.1002/mds.27290
160. Allen, NM, Lin, J-P, Lynch, T, and King, MD. Status Dystonicus: A Practice Guide. Dev Med Child Neurol (2014) 56(2):105–12. doi:10.1111/dmcn.12339
161. Fasano, A, Ricciardi, L, Bentivoglio, AR, Canavese, C, Zorzi, G, Petrovic, I, et al. Status Dystonicus: Predictors of Outcome and Progression Patterns of Underlying Disease. Mov Disord (2012) 27(6):783–8. doi:10.1002/mds.24981
162. Garone, G, Graziola, F, Nicita, F, Frascarelli, F, Randi, F, Zazza, M, et al. Prestatus and Status Dystonicus in Children and Adolescents. Dev Med Child Neurol (2020) 62(6):742–9. doi:10.1111/dmcn.14425
163. Lumsden, DE. The Spectrum of Dystonia Severity before Status Dystonicus. Dev Med Child Neurol (2020) 62:668. doi:10.1111/dmcn.14435
164. Iodice, A, and Pisani, F. Status Dystonicus: Management and Prevention in Children at High Risk. Acta Biomed (2019) 90:207–12. doi:10.23750/abm.v90i3.7207
165. Lumsden, DE, Kaminska, M, Ashkan, K, Selway, R, and Lin, J-P. Deep Brain Stimulation for Childhood Dystonia: Is 'where' as Important as in 'whom'? Eur J Paediatric Neurol (2017) 21:176–84. doi:10.1016/j.ejpn.2016.10.002
166. Lumsden, DE, King, MD, and Allen, NM. Status Dystonicus in Childhood. Curr Opin Pediatr (2017) 29:674–82. doi:10.1097/MOP.0000000000000556
167. Nerrant, E, Gonzalez, V, Milesi, C, Vasques, X, Ruge, D, Roujeau, T, et al. Deep Brain Stimulation Treated Dystonia-Trajectory via Status Dystonicus. Mov Disord (2018) 33(7):1168–73. doi:10.1002/mds.27357
168. Lobato-Polo, J, Ospina-Delgado, D, Orrego-González, E, Gómez-Castro, JF, Orozco, JL, and Enriquez-Marulanda, A. Deep Brain Stimulation Surgery for Status Dystonicus: A Single-Center Experience and Literature Review. World Neurosurg (2018) 114:e992–e1001. doi:10.1016/j.wneu.2018.03.129
169. Kousa, YA, Murnick, J, Burton, J, DiSabella, M, and Pergami, P. Acute Pontine Ischemic Stroke in a Healthy Child with Intracranial Vasculopathy. J Child Neurol (2019) 34(13):820–3. doi:10.1177/0883073819861851
170. Ebrahimi-Fakhari, D, Van Karnebeek, C, and Münchau, A. Movement Disorders in Treatable Inborn Errors of Metabolism. Mov Disord (2019) 34:598–613. doi:10.1002/mds.27568
171. Jinnah, HA. The Dystonias. CONTINUUM: Lifelong Learn Neurol (2019) 25:976–1000. doi:10.1212/CON.0000000000000747
172. Kinnett, D. Botulinum Toxin A Injections in Children. Am J Phys Med Rehabil (2004) 83:S59–S64. doi:10.1097/01.PHM.0000141131.66648.E9
Keywords: dystonia, pediatric dystonia, deep brain stimulation, botulinum toxins, cerebral palsy, pharmacological treatment for dystonia
Citation: Gorodetsky C and Fasano A (2022) Approach to the Treatment of Pediatric Dystonia. Dystonia 1:10287. doi: 10.3389/dyst.2022.10287
Received: 06 December 2021; Accepted: 13 June 2022;
Published: 02 August 2022.
Edited by:
Aasef Shaikh, Case Western Reserve University, United StatesReviewed by:
Aparna Wagle Shukla, University of Florida, United StatesAasef Shaikh, Case Western Reserve University, United States
Copyright © 2022 Gorodetsky and Fasano. This is an open-access article distributed under the terms of the Creative Commons Attribution License (CC BY). The use, distribution or reproduction in other forums is permitted, provided the original author(s) and the copyright owner(s) are credited and that the original publication in this journal is cited, in accordance with accepted academic practice. No use, distribution or reproduction is permitted which does not comply with these terms.
*Correspondence: Alfonso Fasano, alfonso.fasano@uhn.ca