- 1University Hospitals Cleveland Medical Center, Cleveland, OH, United States
- 2Department of Neurology, Case Western Reserve University, Cleveland, OH, United States
- 3Movement disorders and Neuromodulation Center, Department of Neurology, University of California, San Francisco, San Francisco, CA, United States
The primary target for deep brain stimulation (DBS) for medication refractory dystonia has traditionally been the globus pallidus internus (GPi), however alternate targets have also been explored with the hope they might offer similar or superior outcomes with less side effects and reduced battery demands. Recent studies have shown comparable outcomes with both pallidal and subthalamic (STN) DBS, although the level of evidence is still superior for the GPi. There may not be an “optimal target” for all dystonia patients, with both targets offering the potential for excellent control of dystonia but more comparison studies are needed. In this review, we will discuss the history, efficacy, as well as target specific benefits and possible side effects of STN DBS for dystonia.
Introduction
Over the last 10 years, STN DBS has emerged as a promising stimulation target for dystonia and has now been performed for various types, including isolated dystonia (1-4), dopa responsive dystonia (5), dystonic tremor (6), tremor associated with dystonia (7), tardive dystonia (8), neurodegeneration with brain Iron accumulation (9) and others. In this article, we will review the history of STN DBS for dystonia, the outcomes and discuss site-specific benefits and side effects in conditions such as isolated dystonia as well as acquired dystonia (10) Almost all studies report non-blinded outcomes and assess only STN as a target in a single cohort of patients. The debate if one target is superior (GPi or STN) for improving dystonia continues for any given individual but both targets offer the potential to provide excellent dystonia control. Other emerging targets (Thalamic/cerebellar) are also being explored. With newer imaging software and sensing systems now available, we are continuing to learn about the pathophysiology of dystonia and the mechanism of DBS across multiple “circuit nodes.”
History of STN DBS for Dystonia and Outcomes
GPi DBS for dystonia was first reported in 1999, when neurosurgeon Philip Coubes reported improvement of an 8 year old girl with refractory status dystonicus (11). He later reported successful treatment of seven patients with generalized dystonia with bilateral pallidal DBS. Simultaneously Krauss et al reported successful treatment of three patients with refractory cervical dystonia (12). Pallidal DBS is now a recognized and validated therapy for medication refractory isolated generalized or cervical dystonia (13-20), with Level 1 evidence (21) and long term benefits documented a decade and more post implantation (22-24).
The earliest studies of STN DBS in dystonia were published in 2007 (25). Sun et al reported a single center retrospective case series of 14 patients with generalized dystonia (two patients w tardive dystonia and 12 with primary generalized dystonia) with robust response to STN DBS. The mean improvement on the Burke Fahn Marsden Dystonia Rating scale (26) (BFMDRS) was reported to be 88% with a mean follow up of 28 months. That same year, Kleiner-Fisman (2) et al reported outcomes in four patients with predominantly cervical dystonia treated with STN DBS. Blinded rating with BFMDRS and Toronto Western Torticollis Rating Scale (TWSTRS) (27) at 12 months showed significant improvement only in the TWSTRS. The majority of patients in this study had significant comorbidities including fixed skeletal abnormalities, likely limiting benefit.
These early studies, the observation that Parkinson’s Disease associated dystonia could be improved with STN DBS (28, 29), and the limited benefit in some patients with GPi DBS (20), all prompted further investigation into the STN as an alternate dystonia target. Also, as the field gained more experience treating dystonia with GPi DBS, over time in some patients, new stimulation induced side effects began to be appreciated. This included development of bradykinesia most notably in previous non dystonic body regions, micrographia and other handwriting difficulties, trouble getting out of a chair, moving in bed, as well as gait changes (sometimes freezing of gait) (30-32). Often side effects could be managed with stimulation adjustments such as reducing frequency of stimulation (33), but not in all cases. This prompted wider interest in exploring alternative dystonia DBS targets including the STN.
STN DBS in Isolated Cervical and Generalized Dystonia
Table 1 includes all published cases of STN DBS in isolated dystonia that describe four or more patients (25, 34-45). The first blinded prospective study of STN DBS in dystonia was published in 2011, describing one-year outcomes in nine patients with predominantly cervical dystonia (4). The total TWSTRS score improved a mean of 62.9%, with most of the improvements achieved by 3 months. STN DBS was well tolerated and there were no serious adverse events. Three patients experienced marked weight gain and all patients experienced transient stimulation induced dyskinesia. Later the same group published a continuation of this study, which then included 20 patients who were followed prospectively for 3 years (3). The cohort included a majority of cervical and craniocevical dystonia patients, but also included generalized, bibrachial, segmental dystonia subtypes, and some with a DYT1 mutation. At 36 months, BFMDRS motor score improved by a mean of 70.4% and the TWSTRS by 66%, similar to previously reported outcomes with bilateral pallidal DBS (13, 17-20). No significant device related complications occurred, but one patient experienced persistent dysphoria when turning on the left STN lead. Non-responder rate was at 10%, which is lower than what has previously been described with pallidal DBS at 27% (12). Benefits noted by authors included more rapid improvement in dystonic symptoms with initiation of therapy as well as lower IPG drainage due to lower required stimulation settings. No reports of bradykinesia were reported, however, stimulation induced dyskinesia (SID) occurred as can be seen in other conditions treated with this target (46). A sub-study of the same cohort showed dystonia control was greater with high frequency subthalamic DBS compared to low frequency stimulation (47).
The longer-term (10 years) benefit of STN-DBS for generalized dystonia were retrospectively studied in a series of nine pediatric patients with generalized dystonia (42). The mean age at surgery was 15.9 years and the mean improvement in BFMDRS motor score was 77.1% at 1 year and 90.4% at 10-year. BFMDRS disability scores were also reduced by 87% at 10-year. Notably only two of the nine patients carried the TOR1A mutation for DYT1. As with several pallidal studies (48, 49), a negative correlation was found between the duration of disease to age of surgery ratio and the degree of BFMDRS motor and total score improvement. Stimulation related adverse events reported at 10 years included dysarthria, dysphagia (n = 4) and gait changes (n = 4). There were no significant surgery related side effects and, eight of the patients needed IPG replacement during follow up. One patient reported weight gain, and one patient experienced a lead breakage. Interestingly clinical efficacy was maintained after IPG depletion in one patient, and while the DBS was temporarily turned off in another, highlighting the possibility of STN DBS inducing functional brain changes even after cessation of neuromodulation (42), though this may not be target specific effect, as it has also been reported with pallidal DBS (50).
Meige Syndrome
Evidence for both STN and GPi DBS in Meige syndrome (an adult-onset segmental dystonia manifesting predominantly with oromandibular dystonia and blepharospasm) remains primarily retrospective or based on case reports (38, 39, 43, 44, 51). The largest report has been from China describing 32 Meige syndrome patients treated with bilateral STN DBS with a mean improvement in the BFMDRS of 79%. Ten of the patients experienced stimulation induced dyskinesia. The mean voltage for development of dyskinesia was 1.7 V and stimulation of ventral STN was more likely to induce dyskinesia (41).
Acquired and Neurodegenerative Dystonia (Formerly Secondary Dystonia)
Tardive dystonia (TD) is a disabling and irreversible iatrogenic movement disorder typically caused by exposure to dopamine blocking agents. Often axial musculature is predominantly affected, such as neck, jaw and trunk (45). Robust, rapid and sustained improvement of TD has been reported with pallidal DBS, with a mean percentage improvement in BMFDRS-motor sub score of 83% at a mean follow up of 41 months (46, 47). Similar outcomes appear to be possible with STN DBS with over 17 cases of TD treated with STN now published in the literature (26, 48-50). Deng et al reported long-term outcomes of 10 patients with tardive dystonia treated with bilateral STN DBS, with the mean follow up being 65 months (range 12–105 months). Mean improvement in BFMDRS motor sub score at last follow up was 88%. Patients showed a statistically significant improvement in Quality of Life as measured by the SF-36, and notably both Hamilton Anxiety Scale and Hamilton Depression scale displayed significant reductions (51). Several TD patient outcomes are described in mixed patient reports that also include patients with isolated dystonia describing, all with robust response to STN DBS(26)(48).
The STN has sometimes been targeted and implanted when the patient has existing damage to the pallidum noted on MRI(52) (53). Liu et al reported outcomes of three patients with PKAN implanted with STN DBS, with a mean improvement in BFMDRS motor scores of 58%, a more robust response than in the prior multicenter retrospective reports of GPi DBS in PKAN (54, 55) with authors suggesting the STN might be a good target especially for patients with prominent appendicular rather than axial dystonia (56). STN DBS has also been reported to be beneficial in two patients with Neuroacanthocytosis (57) including lingual dystonia (58).
Several case reports documenting outcomes of STN DBS for other cases of acquired or neurodegenerative dystonia have been published, with variable duration of follow up and efficacy (5, 9, 52, 53, 59-63). The improvement in dystonia rating scales is generally less robust than what is experienced in isolated dystonia (64), although meaningful improvements in quality of life is often seen- similar to what has been reported in pallidal DBS (Table 2).
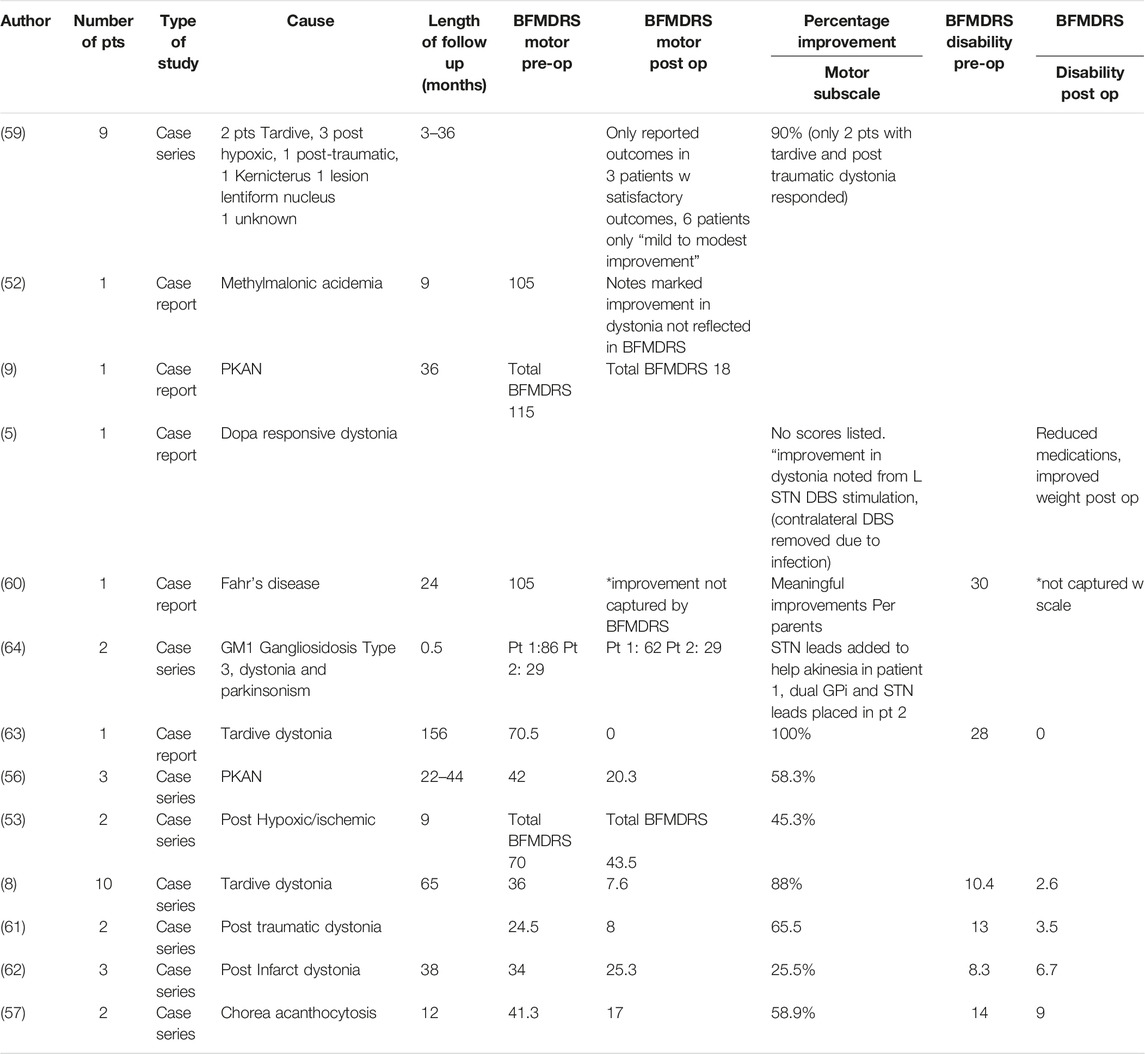
TABLE 2. Subthalamic nucleus DBS for acquired and neurodegenerative dystonia. BFMDRS: Burke-Fahn Marsden Dystonia Rating scale. BMDRS-M + motor subscore, BFMDRS-D: disability subscore.
Studies Directly Comparing Pallidal and Subthalamic DBS in Dystonia
To date, two prospective crossover studies of STN and GPi DBS in isolated dystonia have been conducted. The first reported by Schjerling et al performed a prospective double blind crossover study of 12 patients with focal, multifocal or generalized dystonia (37). Electrodes were implanted bilaterally in both the GPi and STN and each patient then randomly assigned to receive either 6 months of pallidal or subthalamic stimulation, followed by stimulation of the other target for the subsequent 6 months. No statistically significant difference in dystonia motor outcomes was found. Both targets showed improvement in dystonia motor severity and quality of life measures. All 12 patients accepted six-month subthalamic stimulation, compared to only seven with pallidal DBS. Among those who rejected pallidal stimulation, due to worsening dystonia, three accepted dual GPi and STN stimulation (unblinded). In 2022, a long-term open label follow-up results of the same cohort including some additional subjects was published (45). Twenty-one patients enrolled in the protocol, of which nine had generalized dystonia and 12 had cervical dystonia. Eighteen patients completed the one-year randomization study. Eleven patients chose chronic subthalamic stimulation; six chose pallidal stimulation and one patient opted for dual target stimulation. Fourteen out of 18 patients participated in the long-term follow up study, with a mean follow up of over 10 years. Efficacy between targets was reported to be similar with a mean improvement of 36% in BFMDRS motor sub score and a similar safety profile. Mean duration of dystonia prior to surgery was 19.3 years and age at time of surgery 50.1. The group of patients with cervical dystonia were significantly older than the group with generalized dystonia. Age at surgery predicted a smaller improvement in movement score. Duration of dystonia prior to surgery negatively correlated with improvement in motor score, although not significantly. The authors commented, the more rapid response in dystonia with STN DBS might have favored its use compared to GPi DBS (45).
The second prospective study by Liu et al investigated the response of short-term (several days) pallidal or subthalamic stimulation in eight patients with isolated dystonia (65). The group implanted electrodes into all four nuclei, performed test stimulation using externalized leads, with determination of optimal target and removal of less efficacious electrodes prior to IPG implantation, around 10–14 days after the initial surgery. Stimulation was performed in three phases; patients were randomly assigned to receive either pallidal or subthalamic DBS, followed by a sham phase, followed by the other target. Stimulation parameters were increased until maximal benefit or side effects occurred at which parameters were left static for 24 h prior to rating scale assessments. There was no statistical difference in BFMDRS motor sub score outcome with a mean improvement of 50.6% with pallidal and 43.7% with subthalamic DBS; however, a higher incidence of adverse events including dyskinesia and dysphoria were noted in the STN group. These side effects were generally mild and responsive to adjustments. The main side effects associated with STN DBS were dyskinesia in five patients and dysphoria in two patients. Permanent target selection was then made based on benefit and side effect profile. Of the eight patients, only one patient received subthalamic stimulation long-term (1 year follow-up) (65). Given the smaller size of the subthalamic nucleus and the more rapid response rate, it is not surprising that more side effects occurred with STN stimulation and thus the study design may have favored pallidal stimulation.
Several retrospective cohort studies have also compared target outcomes. Lin et al performed a single center matched retrospective cohort study of 14 GPi and 16 STN DBS generalized dystonia patients implanted over a three-year period. Five patients were lost to follow up. Both targets achieved significant motor symptom improvement at 12 months, with a mean improvement in BFMDRS motor subscore of 84% with STN and 72% with GPi DBS. STN DBS was noted to have a more rapid onset of improvement within the first month from programming initiation as well as significantly lower amount of total energy delivered but showed that pallidal stimulation was more effective in treatment of axial symptoms. Both targets improved quality of life (40). Tian et al performed a retrospective study of 17 consecutive patients treated with DBS for Meige syndrome that included 11 patients treated with STN DBS. Outcomes for both targets were similar, with a mean improvement in BFMDRS-motor score of 69.9% for STN and 71.7% for GPi DBS. BFMDRS disability subscore improvement was 71.6% with STN and 68.4% with GPi DBS. The stimulation voltage was significantly higher as expected in the GPi group (43).
There have been several meta-analyses published comparing outcomes. Tsuboi et al in 2020 summarized the evidence of STN (N = 24) versus GPi DBS (N = 184) for cervical dystonia. Only articles reporting TWSTRS outcomes were included. The authors concluded approximately 80–90% of patients with cervical dystonia are expected to experience a clinically meaningful improvement (defined as more than 25% improvement in TWSTRS) in symptoms following DBS of either target and that both STN and GPi DBS improve motor symptoms, disability, and pain, with similar efficacy. A meta-regression of long-term outcomes of isolated dystonia treated with either pallidal or subthalamic DBS was published in 2019. Authors included papers reporting baseline and follow-up BFMDRS scores up to three or more years and that included 10 or more patients. Three studies of STN DBS and 19 studies with GPi DBS were included. Treatment of both STN and GPI DBS, dystonic symptoms were improved and efficacy was maintained at 3 years. The results showed a positive correlation between a greater treatment effect and shorter disease duration. Authors observed greater improvement in the STN subgroup on univariate regression analysis, but due to the high correlation/association between disease duration and DBS target, no difference in relative efficacy in BFMDRS outcomes between sites was found (66).
Some groups have explored using a dual targeting strategy with bilateral GPi and STN in neurodegenerative dystonia. Two patients with secondary parkinsonism and dystonia due to GM1 Gangliosidosis showed greater improvement with STN versus the GPi stimulation (64). Ozturk et al reported outcomes of pallidal versus subthalamic DBS in acquired and neurodegenerative dystonia in studies published between 2005 and 2020. The authors found 72 studies reporting outcomes of 264 cases treated with pallidal DBS (average follow up 19 months) and reported a mean BFMDRS improvement of 52%. Ten studies of STN DBS in acquired or neurodegenerative dystonia were identified, reporting outcomes in 146 secondary dystonia cases. The mean BFMDRS improvement was 66% (minimum 28%, maximum 98%) with an average follow up of 20 months (67).
Specific Considerations for Neuromodulation in Dystonia
Relative to other movement disorders, patient selection and DBS programming can be more difficult in dystonia. The etiology of dystonia is sometimes unclear and the dystonia clinical features can vary widely (i.e., dystonia phenotype, distribution, age of onset, genetic status), Prognostic factors associated with better outcomes from DBS in dystonia include DYT1 genetic status (68), shorter disease duration (48) and absence of fixed skeletal abnormalities(69). Given the rapid evolution of dystonia genetics and with more available genetic testing, other prognostic markers are likely to emerge in the future.
Considerations in Target Selection
Specific limitations with pallidal DBS include higher energy stimulation and thus higher IPG drainage, although IPG lifetime and availability of rechargeable systems make this a less important consideration in target selection currently. The rate of hemorrhage during DBS lead implantation maybe slightly higher with pallidal DBS implantation, although thankfully the hemorrhage rate is low across both targets (70). The sometimes slow and insidious onset of benefit with pallidal stimulation can also offer challenges for DBS programmers, given the lack of immediate feedback while programming in some cases. STN DBS may offer a more rapid response and be helpful in optimizing stimulation parameters in a more timely fashion (4).
Stimulation Induced Dyskinesia
While GPi DBS has been reported to cause bradykinesia (30, 32), STN DBS can cause stimulation induced dyskinesia (SID) as previously reported with STN DBS in PD (71), Obsessive Compulsive Disorder (72) and Huntington’s disease (73). Similar SID can also occur with STN DBS in dystonia as we have mentioned (46, 41). The phenomenology of these hyperkinetic movements can be both varied and complex and can complicate optimizing DBS programming. Bledsoe et al reported athetotic movements of fingers, chorea, rapid jerks as well as more sustained dystonic posturing (46). These movements often occurred in previously non-dystonic or hyperkinetic areas. Importantly all patients in this case series did experience SID with initial programming; however, some experienced delayed onset of SID several months after last programming session. The latter is an important finding as it should prompt programmers to evaluate the patient in the OFF stimulation state, to establish if the new hyperkinetic movements are due to SID versus spread or worsening of dystonia. Programming strategies proposed to combat SID included slow increase in amplitude of stimulation, use of bipolar configuration as well as activating the dorsal contacts (4, 46). Programming the dorsal STN using interleaving has previously reported to be anti-dyskinetic in PD, likely due to activation of pallidofugal fibers (74) can also be applied. The dorsolateral STN has been reported to offer optimal dystonia control (40). Newer segmented directional leads may also help minimize SID with more exact stimulation delivery.
Weight Changes
Weight gain has frequently been reported with STN DBS for PD (75) and similar changes have been reported with some patients treated with STN DBS for dystonia and appears to be target specific (76). Three of nine patients in Ostrem et al initial prospective trial experienced 10% increase in body weight (4).
Neuropsychiatric Considerations
Neuropsychological outcomes after STN DBS are scarce. In the prospective study of STN DBS for dystonia, no consistent neuropsychological deficits occurred. Although transient worsening of depression was observed in several patients, the Beck depression Inventory (77) did not worsen at 12 months (4). Contrarily, the Hamilton Depression Scale and the Hamilton Anxiety Scale showed significant reduction at last follow up (>5 years) in 10 patients treated with STN DBS for TD(79). Although there have been many published reports of cognitive concerns in vulnerable patients after STN DBS in PD (79, 80), a study of working memory and attention using a dual task paradigm in eight cervical dystonia patients both ON and OFF STN DBS showed no significant worsening of cognitive function (81). Multiple studies have reported sustained improvement in quality of life post STN DBS in dystonia, usually reported by the SF-36 questionnaire (36, 78).
Role of Rescue Leads
A multi-center cohort study of 132 patients with DYT1 dystonia treated with pallidal DBS found a suboptimal (less than 30% improvement in BFMDRS) long term response in 8% (11 patients). All 11 of these patients experienced an initial response of more than 30% postoperatively, with subsequent worsening. Younger age of onset, faster disease progression and cranial involvement were felt to represent a more aggressive phenotype of DYT1. Off stimulation evaluation showed worsening of dystonia, despite missing the 30% improvement threshold, thus indicating some continued benefit. All 11 patients underwent further DBS surgeries, either targeting the STN or the GPi, Additional leads did not offer meaningful improvement, if the initial leads were optimally placed (82).
Discussion
Both pallidal and subthalamic bilateral and unilateral DBS are FDA approved under the Human Device Exemption (HDE) for treatment of drug refractory isolated dystonia, including generalized, cervical and hemidystonia in patient’s age seven and above. (https://www.accessdata.fda.gov/scripts/cdrh/cfdocs/cfhde/hde.cfm?id=H020007).
Pallidal DBS has Level I evidence for both generalized and cervical dystonia(18, 83) whereas STN DBS has level IV for generalized dystonia(3) and level III for cervical dystonia(4). Over 80 patients with generalized, 24 with cervical, 87 with Meige syndrome and 17 with tardive dystonia treated with STN DBS have been reported in the literature (Table 1, 2). Most studies have concluded outcomes after STN DBS in dystonia to be similar to GPi DBS. Challenges now exist for clinicians to choose from multiple effective targets for dystonia—much like we have for other disorders (PD, and off label Tourette syndrome and obsessive-compulsive disorder) (84-86), See Table 3. Not discussed in this review is also the application of thalamic DBS for dystonia (87) and dystonic tremor (88), cerebellar DBS (89, 90) as well as cortical stimulation (91) which may increase clinical options in the future. Although dystonia subtypes are often grouped together on the basis of their motor manifestations, pathogenesis may differ among the subtypes. Current understanding of the neuroanatomical basis of dystonia have evolved from a focus on dysfunction of the basal ganglia to include a broader motor network model involving the basal ganglia, cerebellum, cerebral cortex, and other brain regions (92). Important concepts in the pathophysiology include the impairment of sensorimotor integration, a loss of inhibitory control on several levels of the central nervous system including the basal ganglia, cortex and brain stem as well as changes in synaptic plasticity (93, 94). Reduced function of the indirect pathway with decreased pallidal inhibition of the thalamo-cortical circuitry as well as hyper functional activity of the direct basal ganglia pathway has been proposed to contribute to abnormal motor cortical excitability in dystonia (95). In addition, primate research has shown the STN receives substantial direct cortical projections, via the “hyperdirect” pathway(96) and cortical areas projecting to the STN have been implicated in dystonia pathophysiology(97).
Recent research has also highlighted the importance of the cerebellum in the genesis of dystonia (98). Previously it was thought the basal ganglia and cerebellum only communicated through higher order cortical relay and functioned somewhat independently by relaying projections to cortical areas via separate thalamic nuclei, however recently a is a disynaptic connection from the motor STN, via pontine nuclei, to the cerebellar cortex has been described (99, 100). Additionally, the cerebellar dentate nucleus has a disynaptic connection, via the intralaminar nucleus of the thalamus, directly to the striatum (100).
It is interesting that such diverse therapeutic effects are possible from multiple DBS targets and can improve both hypokinetic and hyperkinetic movement disorders. The exact mechanism of how DBS exerts its therapeutic effect remains unknown but evidence is mounting that DBS is suppressing pathological oscillatory activity within these brain networks (101). Current DBS systems have the ability to record local field potentials (LFP) from target nuclei and low frequency theta activity (3–8 Hz) has been shown to be associated with dystonia and suppressed by therapeutic DBS (102). Theta spectral activity has been recorded from both STN (103) and GPi (104) targets and correlated with clinical severity (105, 106). A recent proof of principle study showing adaptive DBS of the STN in cervical dystonia controlled by cortical theta oscillation showed improved clinical rating compared to continuous DBS as well as increased peak amplitudes without inducing SID (107).
Conclusion
Deep Brain stimulation represents a powerful treatment option for dystonia, regardless of target. The optimal target for neuromodulation remains unknown and future head-to-head studies are needed. Thus far, pallidal DBS still has significantly more robust evidence over STN DBS. Other emerging targets outside of the basal ganglia known to be important n dystonia, such as the cerebellum has also gained recent attention. Modulating brain circuits by targeting one of several different nodes within the network can offer powerful symptomatic benefit. Given that targeting multiple areas within the circuit have been shown to both disrupt the abnormal pathological oscillatory activity and offer robust and similar clinical efficacy, clinicians are now faced with determining a final target based on multiple factors including the best side effect profile, ease of surgical targeting, and programming for an individual patient. Emerging tools including improved imaging approaches such as connectomics as well as more sophisticated neurophysiology may help guide ideal nodes to target with DBS on an individual basis in the future.
Author Contributions
Study conception and design: CK and JO. Data collection: CK. Interpretation of results: CK and JO. First draft manuscript preparation: CK. All authors reviewed the results and approved the final version of the manuscript.
Conflict of Interest
The authors declare that the research was conducted in the absence of any commercial or financial relationships that could be construed as a potential conflict of interest.
References
1. Pahapill, PA, and O'Connell, B. Long-term Follow-Up Study of Chronic Deep Brain Stimulation of the Subthalamic Nucleus for Cervical Dystonia. Neuromodulation (2010) 13(1):26–30. doi:10.1111/j.1525-1403.2009.00231.x
2. Kleiner-Fisman, G, Liang, GS, Moberg, PJ, Ruocco, AC, Hurtig, HI, Baltuch, GH, et al. Subthalamic Nucleus Deep Brain Stimulation for Severe Idiopathic Dystonia: Impact on Severity, Neuropsychological Status, and Quality of Life. J Neurosurg (2007) 107(1):29–36. doi:10.3171/JNS-07/07/0029
3. Ostrem, JL, San Luciano, M, Dodenhoff, KA, Ziman, N, Markun, LC, Racine, CA, et al. Subthalamic Nucleus Deep Brain Stimulation in Isolated Dystonia: A 3-year Follow-Up Study. Neurology (2017) 88(1):25–35. doi:10.1212/WNL.0000000000003451
4. Ostrem, JL, Racine, CA, Glass, GA, Grace, JK, Volz, MM, Heath, SL, et al. Subthalamic Nucleus Deep Brain Stimulation in Primary Cervical Dystonia. Neurology (2011) 76(10):870–8. doi:10.1212/WNL.0b013e31820f2e4f
5. Tormenti, MJ, Tomycz, ND, Coffman, KA, Kondziolka, D, Crammond, DJ, and Tyler-Kabara, EC. Bilateral Subthalamic Nucleus Deep Brain Stimulation for Dopa-Responsive Dystonia in a 6-Year-Old Child. J Neurosurg Pediatr (2011) 7(6):650–3. doi:10.3171/2011.3.PEDS10402
6. Pauls, KA, Hammesfahr, S, Moro, E, Moore, AP, Binder, E, El Majdoub, F, et al. Deep Brain Stimulation in the Ventrolateral Thalamus/subthalamic Area in Dystonia with Head Tremor. Mov Disord (2014) 29(7):953–9. doi:10.1002/mds.25884
7. Chou, KL, Hurtig, HI, Jaggi, JL, and Baltuch, GH. Bilateral Subthalamic Nucleus Deep Brain Stimulation in a Patient with Cervical Dystonia and Essential Tremor. Mov Disord (2005) 20(3):377–80. doi:10.1002/mds.20341
8. Deng, ZD, Li, DY, Zhang, CC, Pan, YX, Zhang, J, Jin, H, et al. Long-term Follow-Up of Bilateral Subthalamic Deep Brain Stimulation for Refractory Tardive Dystonia. Parkinsonism Relat Disord (2017) 41:58–65. doi:10.1016/j.parkreldis.2017.05.010
9. Ge, M, Zhang, K, Ma, Y, Meng, FG, Hu, WH, Yang, AC, et al. Bilateral Subthalamic Nucleus Stimulation in the Treatment of Neurodegeneration with Brain Iron Accumulation Type 1. Stereotact Funct Neurosurg (2011) 89(3):162–6. doi:10.1159/000323374
10. Albanese, A, Bhatia, K, Bressman, SB, Delong, MR, Fahn, S, Fung, VS, et al. Phenomenology and Classification of Dystonia: a Consensus Update. Mov Disord (2013) 28(7):863–73. doi:10.1002/mds.25475
11. Coubes, P, Echenne, B, Roubertie, A, Vayssiere, N, Tuffery, S, Humbertclaude, V, et al. Treatment of Early-Onset Generalized Dystonia by Chronic Bilateral Stimulation of the Internal Globus Pallidus. Apropos of a Case. Neurochirurgie (1999) 45(2):139–44.
12. Krauss, JK, Pohle, T, Weber, S, Ozdoba, C, and Burgunder, JM. Bilateral Stimulation of Globus Pallidus Internus for Treatment of Cervical Dystonia. Lancet (1999) 354(9181):837–8. doi:10.1016/S0140-6736(99)80022-1
13. Vidailhet, M, Vercueil, L, Houeto, J-L, Krystkowiak, P, Benabid, A-L, Cornu, P, et al. Bilateral Deep-Brain Stimulation of the Globus Pallidus in Primary Generalized Dystonia. N Engl J Med (2005) 352(5):459–67. doi:10.1056/NEJMoa042187
14. Coubes, P, Cif, L, El Fertit, H, Hemm, S, Vayssiere, N, Serrat, S, et al. Electrical Stimulation of the Globus Pallidus Internus in Patients with Primary Generalized Dystonia: Long-Term Results. J Neurosurg (2004) 101(2):189–94. doi:10.3171/jns.2004.101.2.0189
15. Hung, SW, Hamani, C, Lozano, AM, Poon, YY, Piboolnurak, P, Miyasaki, JM, et al. Long-term Outcome of Bilateral Pallidal Deep Brain Stimulation for Primary Cervical Dystonia. Neurology (2007) 68(6):457–9. doi:10.1212/01.wnl.0000252932.71306.89
16. Kiss, ZH, Doig-Beyaert, K, Eliasziw, M, Tsui, J, Haffenden, A, Suchowersky, O, et al. The Canadian Multicentre Study of Deep Brain Stimulation for Cervical Dystonia. Brain (2007) 130(11):2879–86. doi:10.1093/brain/awm229
17. Kupsch, A, Benecke, R, Muller, J, Trottenberg, T, Schneider, GH, Poewe, W, et al. Pallidal Deep-Brain Stimulation in Primary Generalized or Segmental Dystonia. N Engl J Med (2006) 355(19):1978–90. doi:10.1056/NEJMoa063618
18. Vidailhet, M, Vercueil, L, Houeto, J-L, Krystkowiak, P, Lagrange, C, Yelnik, J, et al. Bilateral, Pallidal, Deep-Brain Stimulation in Primary Generalised Dystonia: a Prospective 3 Year Follow-Up Study. Lancet Neurol (2007) 6(3):223–9. doi:10.1016/S1474-4422(07)70035-2
19. Volkmann, J, Mueller, J, Deuschl, G, Kuhn, AA, Krauss, JK, Poewe, W, et al. Pallidal Neurostimulation in Patients with Medication-Refractory Cervical Dystonia: a Randomised, Sham-Controlled Trial. Lancet Neurol (2014) 13(9):875–84. doi:10.1016/S1474-4422(14)70143-7
20. Volkmann, J, Wolters, A, Kupsch, A, Muller, J, Kuhn, AA, Schneider, GH, et al. Pallidal Deep Brain Stimulation in Patients with Primary Generalised or Segmental Dystonia: 5-year Follow-Up of a Randomised Trial. Lancet Neurol (2012) 11(12):1029–38. doi:10.1016/S1474-4422(12)70257-0
21. Sackett, DL. Rules of Evidence and Clinical Recommendations on the Use of Antithrombotic Agents. Chest (1989) 95(2):2S–3S. doi:10.1378/chest.89.2_supplement.2s
22. Krause, P, Volzmann, S, Ewert, S, Kupsch, A, Schneider, GH, and Kuhn, AA. Long-term Effects of Bilateral Pallidal Deep Brain Stimulation in Dystonia: a Follow-Up between 8 and 16 Years. J Neurol (2020) 267(6):1622–31. doi:10.1007/s00415-020-09745-z
23. Meoni, S, Fraix, V, Castrioto, A, Benabid, AL, Seigneuret, E, Vercueil, L, et al. Pallidal Deep Brain Stimulation for Dystonia: a Long Term Study. J Neurol Neurosurg Psychiatry (2017) 88(11):960–7. doi:10.1136/jnnp-2016-315504
24. Kaelin-Lang, A, You, H, Burgunder, JM, Lonnfors-Weitze, T, Loher, TJ, Taub, E, et al. Bilateral Pallidal Stimulation Improves Cervical Dystonia for More Than a Decade. Parkinsonism Relat Disord (2020) 81:78–81. doi:10.1016/j.parkreldis.2020.10.028
25. Sun, B, Chen, S, Zhan, S, Le, W, and Krahl, SE. Subthalamic Nucleus Stimulation for Primary Dystonia and Tardive Dystonia. Acta Neurochir Suppl (2007) 97(2):207–14. doi:10.1007/978-3-211-33081-4_23
26. Burke, RE, Fahn, S, Marsden, CD, Bressman, SB, Moskowitz, C, and Friedman, J. Validity and Reliability of a Rating Scale for the Primary Torsion Dystonias. Neurology (1985) 35(1):73–7. doi:10.1212/wnl.35.1.73
27. Comella, CL, Stebbins, GT, Goetz, CG, Chmura, TA, Bressman, SB, and Lang, AE. Teaching Tape for the Motor Section of the Toronto Western Spasmodic Torticollis Scale. Mov Disord (1997) 12(4):570–5. doi:10.1002/mds.870120414
28. Benabid, AL, Koudsie, A, Benazzouz, A, Vercueil, L, Fraix, V, Chabardes, S, et al. Deep Brain Stimulation of the Corpus Luysi (Subthalamic Nucleus) and Other Targets in Parkinson's Disease. Extension to New Indications Such as Dystonia and Epilepsy. J Neurol (2001) 248(3):III37–47. doi:10.1007/pl00007825
29. Krack, P, Pollak, P, Limousin, P, Benazzouz, A, Deuschl, G, and Benabid, AL. From Off-Period Dystonia to Peak-Dose Chorea. The Clinical Spectrum of Varying Subthalamic Nucleus Activity. Brain (1999) 122(6):1133–46. doi:10.1093/brain/122.6.1133
30. Berman, BD, Starr, PA, Marks, WJ, and Ostrem, JL. Induction of Bradykinesia with Pallidal Deep Brain Stimulation in Patients with Cranial-Cervical Dystonia. Stereotact Funct Neurosurg (2009) 87(1):37–44. doi:10.1159/000195718
31. Zauber, SE, Watson, N, Comella, CL, Bakay, RA, and Metman, LV. Stimulation-induced Parkinsonism after Posteroventral Deep Brain Stimulation of the Globus Pallidus Internus for Craniocervical Dystonia. J Neurosurg (2009) 110(2):229–33. doi:10.3171/2008.6.17621
32. Mahlknecht, P, Georgiev, D, Akram, H, Brugger, F, Vinke, S, Zrinzo, L, et al. Parkinsonian Signs in Patients with Cervical Dystonia Treated with Pallidal Deep Brain Stimulation. Brain (2018) 141(10):3023–34. doi:10.1093/brain/awy217
33. Huebl, J, Brucke, C, Schneider, GH, Blahak, C, Krauss, JK, and Kuhn, AA. Bradykinesia Induced by Frequency-specific Pallidal Stimulation in Patients with Cervical and Segmental Dystonia. Parkinsonism Relat Disord (2015) 21(7):800–3. doi:10.1016/j.parkreldis.2015.04.023
34. Cao, C, Pan, Y, Li, D, Zhan, S, Zhang, J, and Sun, B. Subthalamus Deep Brain Stimulation for Primary Dystonia Patients: a Long-Term Follow-Up Study. Mov Disord (2013) 28(13):1877–82. doi:10.1002/mds.25586
35. Fonoff, ET, Campos, WK, Mandel, M, Alho, EJ, and Teixeira, MJ. Bilateral Subthalamic Nucleus Stimulation for Generalized Dystonia after Bilateral Pallidotomy. Mov Disord (2012) 27(12):1559–63. doi:10.1002/mds.25127
36. Deng, Z, Pan, Y, Zhang, C, Zhang, J, Qiu, X, Zhan, S, et al. Subthalamic Deep Brain Stimulation in Patients with Primary Dystonia: a Ten-Year Follow-Up Study. Parkinsonism Relat Disord (2018) 55:103–10. doi:10.1016/j.parkreldis.2018.05.024
37. Schjerling, L, Hjermind, LE, Jespersen, B, Madsen, FF, Brennum, J, Jensen, SR, et al. A Randomized Double-Blind Crossover Trial Comparing Subthalamic and Pallidal Deep Brain Stimulation for Dystonia. J Neurosurg (2013) 119(6):1537–45. doi:10.3171/2013.8.JNS13844
38. Zhan, S, Sun, F, Pan, Y, Liu, W, Huang, P, Cao, C, et al. Bilateral Deep Brain Stimulation of the Subthalamic Nucleus in Primary Meige Syndrome. J Neurosurg (2018) 128(3):897–902. doi:10.3171/2016.12.JNS16383
39. Yao, C, Horn, A, Li, N, Lu, Y, Fu, Z, Wang, N, et al. Post-Operative Electrode Location and Clinical Efficacy of Subthalamic Nucleus Deep Brain Stimulation in Meige Syndrome. Parkinsonism Relat Disord (2019) 58:40–5. doi:10.1016/j.parkreldis.2018.05.014
40. Lin, S, Wu, Y, Li, H, Zhang, C, Wang, T, Pan, Y, et al. Deep Brain Stimulation of the Globus Pallidus Internus versus the Subthalamic Nucleus in Isolated Dystonia. J Neurosurg (2019) 132(3):721–32. doi:10.3171/2018.12.JNS181927
41. Wang, N, Wang, K, Wang, Q, Fan, S, Fu, Z, Zhang, F, et al. Stimulation-Induced Dyskinesia after Subthalamic Nucleus Deep Brain Stimulation in Patients with Meige Syndrome. Neuromodulation (2021) 24(2):286–92. doi:10.1111/ner.13284
42. Xu, W, Li, H, Zhang, C, Sun, B, Wu, Y, and Li, D. Subthalamic Nucleus Stimulation in Pediatric Isolated Dystonia: A 10-Year Follow-Up. Can J Neurol Sci (2020) 47(3):328–35. doi:10.1017/cjn.2020.32
43. Tian, H, Xiong, NX, Xiong, N, Liu, XM, Rao, J, Xiang, W, et al. Similar Long-Term Clinical Outcomes of Deep Brain Stimulation with Different Electrode Targets for Primary Meige Syndrome: One Institution's Experience of 17 Cases. Neuromodulation (2021) 24(2):300–6. doi:10.1111/ner.13304
44. Ouyang, J, Hao, Q, Zhu, R, Wu, G, Ding, H, Wang, D, et al. Subthalamic Nucleus Deep Brain Stimulation in Primary Meige Syndrome: A 1-Year Follow-Up Study. Neuromodulation (2021) 24(2):293–9. doi:10.1111/ner.13174
45. Hock, AN, Jensen, SR, Svaerke, KW, Brennum, J, Jespersen, B, Bergdal, O, et al. A Randomised Double-Blind Controlled Study of Deep Brain Stimulation for Dystonia in STN or GPi - A Long Term Follow-Up after up to 15 Years. Parkinsonism Relat Disord (2022) 96:74–9. doi:10.1016/j.parkreldis.2022.02.001
46. Bledsoe, IO, Dodenhoff, KA, San Luciano, M, Volz, MM, Starr, PA, Markun, LC, et al. Phenomenology and Management of Subthalamic Stimulation-Induced Dyskinesia in Patients with Isolated Dystonia. Mov Disord Clin Pract (2020) 7(5):548–51. doi:10.1002/mdc3.12946
47. Ostrem, JL, Markun, LC, Glass, GA, Racine, CA, Volz, MM, Heath, SL, et al. Effect of Frequency on Subthalamic Nucleus Deep Brain Stimulation in Primary Dystonia. Parkinsonism Relat Disord (2014) 20(4):432–8. doi:10.1016/j.parkreldis.2013.12.012
48. Markun, LC, Starr, PA, Air, EL, Marks, WJ, Volz, MM, and Ostrem, JL. Shorter Disease Duration Correlates with Improved Long-Term Deep Brain Stimulation Outcomes in Young-Onset DYT1 Dystonia. Neurosurgery (2012) 71(2):325–30. doi:10.1227/NEU.0b013e318258e21b
49. Isaias, IU, Alterman, RL, and Tagliati, M. Outcome Predictors of Pallidal Stimulation in Patients with Primary Dystonia: the Role of Disease Duration. Brain (2008) 131(7):1895–902. doi:10.1093/brain/awn120
50. Ruge, D, Cif, L, Limousin, P, Gonzalez, V, Vasques, X, Hariz, MI, et al. Shaping Reversibility? Long-Term Deep Brain Stimulation in Dystonia: the Relationship between Effects on Electrophysiology and Clinical Symptoms. Brain (2011) 134(7):2106–15. doi:10.1093/brain/awr122
51. Wang, X, Zhang, C, Wang, Y, Liu, C, Zhao, B, Zhang, JG, et al. Deep Brain Stimulation for Craniocervical Dystonia (Meige Syndrome): A Report of Four Patients and a Literature-Based Analysis of its Treatment Effects. Neuromodulation (2016) 19(8):818–23. doi:10.1111/ner.12345
52. Chakraborti, S, Hasegawa, H, Lumsden, DE, Ali, W, Kaminska, M, Lin, JP, et al. Bilateral Subthalamic Nucleus Deep Brain Stimulation for Refractory Total Body Dystonia Secondary to Metabolic Autopallidotomy in a 4-Year-Old Boy with Infantile Methylmalonic Acidemia: Case Report. J Neurosurg Pediatr (2013) 12(4):374–9. doi:10.3171/2013.7.PEDS1350
53. Margolesky, J, Schoen, N, Jermakowicz, W, Sur, S, Cajigas, I, Singer, C, et al. Subthalamic Nucleus Deep Brain Stimulation for the Treatment of Secondary Dystonia: A Case Series and Review of Literature. Brain Stimul (2017) 10(4):870–2. doi:10.1016/j.brs.2017.04.123
54. Timmermann, L, Pauls, KAM, Wieland, K, Jech, R, Kurlemann, G, Sharma, N, et al. Dystonia in Neurodegeneration with Brain Iron Accumulation: Outcome of Bilateral Pallidal Stimulation. Brain (2010) 133(3):701–12. doi:10.1093/brain/awq022
55. Kruer, MC, Hiken, M, Gregory, A, Malandrini, A, Clark, D, Hogarth, P, et al. Novel Histopathologic Findings in Molecularly-Confirmed Pantothenate Kinase-Associated Neurodegeneration. Brain (2011) 134(4):947–58. doi:10.1093/brain/awr042
56. Liu, Z, Liu, Y, Yang, Y, Wang, L, Dou, W, Guo, J, et al. Subthalamic Nuclei Stimulation in Patients with Pantothenate Kinase-associated Neurodegeneration (PKAN). Neuromodulation (2017) 20(5):484–91. doi:10.1111/ner.12549
57. Wu, Y, Li, H, Zhang, C, Sun, B, Li, D, and Wu, Y. Subthalamic Nucleus Deep Brain Stimulation in Two Siblings with Chorea-Acanthocytosis. Neurol Sci (2020) 41(6):1623–5. doi:10.1007/s10072-020-04246-3
58. Wu, Y, Pan, Y, Huang, P, Wang, T, Zhang, C, Sun, B, et al. Subthalamic Deep Brain Stimulation in Lingual Dystonia: A Case Series Study. Parkinsonism Relat Disord (2021) 88:114–5. doi:10.1016/j.parkreldis.2021.06.012
59. Zhang, JG, Zhang, K, Wang, ZC, Ge, M, and Ma, Y. Deep Brain Stimulation in the Treatment of Secondary Dystonia. Chin Med J (2006) 119(24):2069–74. doi:10.1097/00029330-200612020-00008
60. Ma, Y, Ge, M, Meng, F, Zhang, K, and Zhang, J. Bilateral Deep Brain Stimulation of the Subthalamic Nucleus Effectively Relieves Dystonia Secondary to Fahr's Disease: a Case Report. Int J Neurosci (2013) 123(8):582–6. doi:10.3109/00207454.2013.772611
61. Li, HX, He, L, Zhang, CC, Eisinger, R, Pan, YX, Wang, T, et al. Deep Brain Stimulation in post-traumatic Dystonia: A Case Series Study. CNS Neurosci Ther (2019) 25(11):1262–9. doi:10.1111/cns.13145
62. Tambirajoo, K, Furlanetti, L, Samuel, M, and Ashkan, K. Subthalamic Nucleus Deep Brain Stimulation in Post-Infarct Dystonia. Stereotact Funct Neurosurg (2020) 98(6):386–98. doi:10.1159/000509317
63. Meng, D-W, Liu, H-G, Yang, A-C, Zhang, K, and Zhang, J-G. Long-term Effects of Subthalamic Nucleus Deep Brain Stimulation in Tardive Dystonia. Chin Med J (2016) 129(10):1257–8. doi:10.4103/0366-6999.181977
64. Baizabal-Carvallo, J-F, Roze, E, Aya-Kombo, M, Romito, L, Navarro, S, Flamand-Rouvière, C, et al. Combined Pallidal and Subthalamic Nucleus Deep Brain Stimulation in Secondary Dystonia-Parkinsonism. Parkinsonism Relat Disord (2013) 19(5):566–8. doi:10.1016/j.parkreldis.2013.01.010
65. Liu, Y, Zhu, G, Jiang, Y, Wang, X, Chen, Y, Meng, F, et al. Comparison of Short-Term Stimulation of the Globus Pallidus Interna and Subthalamic Nucleus for Treatment of Primary Dystonia. World Neurosurg (2019) 123:e211–7. doi:10.1016/j.wneu.2018.11.137
66. Wu, YS, Ni, LH, Fan, RM, and Yao, MY. Meta-Regression Analysis of the Long-Term Effects of Pallidal and Subthalamic Deep Brain Stimulation for the Treatment of Isolated Dystonia. World Neurosurg (2019) 129:e409–e16. doi:10.1016/j.wneu.2019.05.165
67. Ozturk, S, Temel, Y, Aygun, D, and Kocabicak, E. Deep Brain Stimulation of the Globus Pallidus Internus for Secondary Dystonia: Clinical Cases and Systematic Review of the Literature Regarding the Effectiveness of Globus Pallidus Internus versus Subthalamic Nucleus. World Neurosurg (2021) 154:e495–e508. doi:10.1016/j.wneu.2021.07.070
68. Cif, L, Vasques, X, Gonzalez, V, Ravel, P, Biolsi, B, Collod-Beroud, G, et al. Long-term Follow-up of DYT1 Dystonia Patients Treated by Deep Brain Stimulation: an Open-label Study. Mov Disord (2010) 25(3):289–99. doi:10.1002/mds.22802
69. Coubes, P, Roubertie, A, Vayssiere, N, Hemm, S, and Echenne, B. Treatment of DYT1-Generalised Dystonia by Stimulation of the Internal Globus Pallidus. Lancet (2000) 355(9222):2220–1. doi:10.1016/S0140-6736(00)02410-7
70. Binder, DK, Rau, GM, and Starr, PA. Risk Factors for Hemorrhage during Microelectrode-Guided Deep Brain Stimulator Implantation for Movement Disorders. Neurosurgery (2005) 56(4):722–32. doi:10.1227/01.Neu.0000156473.57196.7e
71. Benabid, AL, Benazzouz, A, Limousin, P, Koudsie, A, Krack, P, Piallat, B, et al. Dyskinesias and the Subthalamic Nucleus. Ann Neurol (2000) 47(1):S189–92.
72. Mulders, AEP, Leentjens, AFG, Schruers, K, Duits, A, Ackermans, L, and Temel, Y. Choreatic Side Effects of Deep Brain Stimulation of the Anteromedial Subthalamic Nucleus for Treatment-Resistant Obsessive-Compulsive Disorder. World Neurosurg (2017) 104:e9–1048. doi:10.1016/j.wneu.2017.05.067
73. Gruber, D, Kuhn, AA, Schoenecker, T, Kopp, UA, Kivi, A, Huebl, J, et al. Quadruple Deep Brain Stimulation in Huntington's Disease, Targeting Pallidum and Subthalamic Nucleus: Case Report and Review of the Literature. J Neural Transm (Vienna) (2014) 121(10):1303–12. doi:10.1007/s00702-014-1201-7
74. Aquino, CC, Duffley, G, Hedges, DM, Vorwerk, J, House, PA, Ferraz, HB, et al. Interleaved Deep Brain Stimulation for Dyskinesia Management in Parkinson's Disease. Mov Disord (2019) 34(11):1722–7. doi:10.1002/mds.27839
75. Macia, F, Perlemoine, C, Coman, I, Guehl, D, Burbaud, P, Cuny, E, et al. Parkinson's Disease Patients with Bilateral Subthalamic Deep Brain Stimulation Gain Weight. Mov Disord (2004) 19(2):206–12. doi:10.1002/mds.10630
76. Mills, KA, Scherzer, R, Starr, PA, and Ostrem, JL. Weight Change after Globus Pallidus Internus or Subthalamic Nucleus Deep Brain Stimulation in Parkinson's Disease and Dystonia. Stereotact Funct Neurosurg (2012) 90(6):386–93. doi:10.1159/000340071
77. Beck, AT, Steer, RA, and Brown, GK. Beck Depression Inventory (BDI-II). San Antonio, TX: Pearson (1996).
78. Deng, Z-D, Li, D-Y, Zhang, C-C, Pan, Y-X, Zhang, J, Jin, H, et al. Long-term Follow-Up of Bilateral Subthalamic Deep Brain Stimulation for Refractory Tardive Dystonia. Parkinsonism Relat Disord (2017) 41:58–65. doi:10.1016/j.parkreldis.2017.05.010
79. Parsons, TD, Rogers, SA, Braaten, AJ, Woods, SP, and Troster, AI. Cognitive Sequelae of Subthalamic Nucleus Deep Brain Stimulation in Parkinson's Disease: a Meta-Analysis. Lancet Neurol (2006) 5(7):578–88. doi:10.1016/S1474-4422(06)70475-6
80. Witt, K, Daniels, C, Reiff, J, Krack, P, Volkmann, J, Pinsker, MO, et al. Neuropsychological and Psychiatric Changes after Deep Brain Stimulation for Parkinson's Disease: a Randomised, Multicentre Study. Lancet Neurol (2008) 7(7):605–14. doi:10.1016/S1474-4422(08)70114-5
81. Mills, KA, Markun, LC, San Luciano, M, Rizk, R, Allen, IE, Racine, CA, et al. Effect of Subthalamic Nucleus Deep Brain Stimulation on Dual-Task Cognitive and Motor Performance in Isolated Dystonia. J Neurol Neurosurg Psychiatry (2015) 86(4):404–9. doi:10.1136/jnnp-2014-307942
82. Tsuboi, T, Cif, L, Coubes, P, Ostrem, JL, Romero, DA, Miyagi, Y, et al. Secondary Worsening Following DYT1 Dystonia Deep Brain Stimulation: A Multi-Country Cohort. Front Hum Neurosci (2020) 14:242. doi:10.3389/fnhum.2020.00242
83. Moro, E, LeReun, C, Krauss, JK, Albanese, A, Lin, JP, Walleser Autiero, S, et al. Efficacy of Pallidal Stimulation in Isolated Dystonia: a Systematic Review and Meta-Analysis. Eur J Neurol (2017) 24(4):552–60. doi:10.1111/ene.13255
84. Weaver, FM, Follett, KA, Stern, M, Luo, P, Harris, CL, Hur, K, et al. Randomized Trial of Deep Brain Stimulation for Parkinson Disease: Thirty-Six-Month Outcomes. Neurology (2012) 79(1):55–65. doi:10.1212/WNL.0b013e31825dcdc1
85. Wong, JK, Cauraugh, JH, Ho, KWD, Broderick, M, Ramirez-Zamora, A, Almeida, L, et al. STN vs. GPi Deep Brain Stimulation for Tremor Suppression in Parkinson Disease: A Systematic Review and Meta-Analysis. Parkinsonism Relat Disord (2019) 58:56–62. doi:10.1016/j.parkreldis.2018.08.017
86. Schrock, LE, Mink, JW, Woods, DW, Porta, M, Servello, D, Visser-Vandewalle, V, et al. Tourette Syndrome Deep Brain Stimulation: a Review and Updated Recommendations. Mov Disord (2015) 30(4):448–71. doi:10.1002/mds.26094
87. San Luciano, M, Robichaux-Viehoever, A, Dodenhoff, KA, Gittings, ML, Viser, AC, Racine, CA, et al. Thalamic Deep Brain Stimulation for Acquired Dystonia in Children and Young Adults: a Phase 1 Clinical Trial. J Neurosurg Pediatr (2020) 27(2):203–12. doi:10.3171/2020.7.PEDS20348
88. Tsuboi, T, Jabarkheel, Z, Zeilman, PR, Barabas, MJ, Foote, KD, Okun, MS, et al. Longitudinal Follow-Up with VIM Thalamic Deep Brain Stimulation for Dystonic or Essential Tremor. Neurology (2020) 94(10):e1073–84. doi:10.1212/WNL.0000000000008875
89. Brown, EG, Bledsoe, IO, Luthra, NS, Miocinovic, S, Starr, PA, and Ostrem, JL. Cerebellar Deep Brain Stimulation for Acquired Hemidystonia. Mov Disord Clin Pract (2020) 7(2):188–93. doi:10.1002/mdc3.12876
90. Miterko, LN, Baker, KB, Beckinghausen, J, Bradnam, LV, Cheng, MY, Cooperrider, J, et al. Consensus Paper: Experimental Neurostimulation of the Cerebellum. Cerebellum (2019) 18(6):1064–97. doi:10.1007/s12311-019-01041-5
91. Romito, LM, Franzini, A, Perani, D, Carella, F, Marras, C, Capus, L, et al. Fixed Dystonia Unresponsive to Pallidal Stimulation Improved by Motor Cortex Stimulation. Neurology (2007) 68(11):875–6. doi:10.1212/01.wnl.0000256816.83036.c9
92. Jinnah, HA, and Hess, EJ. Evolving Concepts in the Pathogenesis of Dystonia. Parkinsonism Relat Disord (2018) 46(1):S62–S65. doi:10.1016/j.parkreldis.2017.08.001
93. Hallett, M. Neurophysiology of Dystonia: The Role of Inhibition. Neurobiol Dis (2011) 42(2):177–84. doi:10.1016/j.nbd.2010.08.025
94. Berardelli, A, Rothwell, JC, Hallett, M, Thompson, PD, Manfredi, M, and Marsden, CD. The Pathophysiology of Primary Dystonia. Brain (1998) 121(7):1195–212. doi:10.1093/brain/121.7.1195
95. Simonyan, K, Cho, H, Hamzehei Sichani, A, Rubien-Thomas, E, and Hallett, M. The Direct Basal Ganglia Pathway Is Hyperfunctional in Focal Dystonia. Brain (2017) 140(12):3179–90. doi:10.1093/brain/awx263
96. Nambu, A, Tokuno, H, and Takada, M. Functional Significance of the Cortico-Subthalamo-Pallidal 'hyperdirect' Pathway. Neurosci Res (2002) 43(2):111–7. doi:10.1016/s0168-0102(02)00027-5
97. Carbon, M, and Eidelberg, D. Abnormal Structure-Function Relationships in Hereditary Dystonia. Neuroscience (2009) 164(1):220–9. doi:10.1016/j.neuroscience.2008.12.041
98. Jinnah, HA, Neychev, V, and Hess, EJ. The Anatomical Basis for Dystonia: The Motor Network Model. Tremor Other Hyperkinet Mov (N Y) (2017) 7:506. doi:10.7916/D8V69X3S
99. Bostan, AC, Dum, RP, and Strick, PL. The Basal Ganglia Communicate with the Cerebellum. Proc Natl Acad Sci U S A (2010) 107(18):8452–6. doi:10.1073/pnas.1000496107
100. Chen, CH, Fremont, R, Arteaga-Bracho, EE, and Khodakhah, K. Short Latency Cerebellar Modulation of the Basal Ganglia. Nat Neurosci (2014) 17(12):1767–75. doi:10.1038/nn.3868
101. Eusebio, A, and Brown, P. Synchronisation in the Beta Frequency-Band-Tthe Bad Boy of Parkinsonism or an Innocent Bystander? Exp Neurol (2009) 217(1):1–3. doi:10.1016/j.expneurol.2009.02.003
102. Pina-Fuentes, D, Beudel, M, Van Zijl, JC, Van Egmond, ME, Oterdoom, DLM, Van Dijk, JMC, et al. Low-frequency Oscillation Suppression in Dystonia: Implications for Adaptive Deep Brain Stimulation. Parkinsonism Relat Disord (2020) 79:105–9. doi:10.1016/j.parkreldis.2020.08.030
103. Neumann, WJ, Huebl, J, Brucke, C, Ruiz, MH, Kupsch, A, Schneider, GH, et al. Enhanced Low-Frequency Oscillatory Activity of the Subthalamic Nucleus in a Patient with Dystonia. Mov Disord (2012) 27(8):1063–6. doi:10.1002/mds.25078
104. Silberstein, P, Kuhn, AA, Kupsch, A, Trottenberg, T, Krauss, JK, Wohrle, JC, et al. Patterning of Globus Pallidus Local Field Potentials Differs between Parkinson's Disease and Dystonia. Brain (2003) 126(12):2597–608. doi:10.1093/brain/awg267
105. Barow, E, Neumann, WJ, Brucke, C, Huebl, J, Horn, A, Brown, P, et al. Deep Brain Stimulation Suppresses Pallidal Low Frequency Activity in Patients with Phasic Dystonic Movements. Brain (2014) 137(11):3012–24. doi:10.1093/brain/awu258
106. Piña-Fuentes, D, Beudel, M, Little, S, van Zijl, J, Elting, JW, Oterdoom, DM, et al. Toward Adaptive Deep Brain Stimulation for Dystonia. Neurosurg Focus (2018) 45(2):E3. doi:10.3171/2018.5.FOCUS18155
Keywords: dystonia, cervical dystonia, deep brain stimulation, subthalamic nucleus, secondary dystonia
Citation: Kilbane C and Ostrem JL (2022) Subthalamic Nucleus Deep Brain Stimulation for Dystonia: Evidence, Pros and Cons. Dystonia 1:10609. doi: 10.3389/dyst.2022.10609
Received: 29 April 2022; Accepted: 31 October 2022;
Published: 28 November 2022.
Edited by:
Aasef Shaikh, Case Western Reserve University, United StatesCopyright © 2022 Kilbane and Ostrem. This is an open-access article distributed under the terms of the Creative Commons Attribution License (CC BY). The use, distribution or reproduction in other forums is permitted, provided the original author(s) and the copyright owner(s) are credited and that the original publication in this journal is cited, in accordance with accepted academic practice. No use, distribution or reproduction is permitted which does not comply with these terms.
*Correspondence: Camilla Kilbane, Q2FtaWxsYS5LaWxiYW5lQHVoaG9zcGl0YWxzLm9yZw==