- Department of Dermatology, Gunma University Graduate School of Medicine, Maebashi, Gunma, Japan
Over the past decade, significant advancements in stem cell research led by mesenchymal stem cells (MSCs) have facilitated their practical application in clinical settings, including inflammatory skin diseases. Urine-derived stem cells (USCs) are obtained from healthy human urine in a noninvasive approach with properties similar to mesenchymal stem cells (MSCs). However, the therapeutic potential of USCs for inflammatory skin diseases has not yet been fully explored. Herein, we report the therapeutic effects of USCs-derived culture supernatants on mice with psoriasis-like dermatitis using our originally established human USCs model. We examined the isolation of USCs from human urine using a simple centrifugation process. Cell markers related to MSCs-like cell were positive for CD29, CD44, CD73, CD90, and negative for HLA-DR, CD34, and CD45 by FACS analysis. Differentiation assays revealed that the cells possessed the capability to differentiate into adipocytes, chondrocytes, and osteocytes. USCs-conditioned medium (CM) treatment significantly suppressed the severity of dermatitis in imiquimod (IMQ)-treated psoriasis mice model. Histopathological examination revealed that USCs-CM treatment attenuated epidermal thickness and the numbers of infiltrating inflammatory cells, including neutrophils, T-cells, and macrophages in dermatitis-affected areas in IMQ-treated psoriasis mice. Furthermore, USCs-CM treatment decreased mRNA levels of IL-17A, IL-17F, and IL-23p19 was reduced in dermatitis area. In summary, our findings revealed new potential strategies for utilizing USCs and USCs-CM as therapeutic agents for inflammatory skin diseases, including psoriasis.
Introduction
Stem cells are self-renewing and multipotent cells, which are vital for the development and homeostasis of living organisms. In the past 10 years, significant advancements in stem cell research have facilitated their practical application in clinical settings, utilizing induced pluripotent cells [1, 2], mesenchymal stem cells (MSCs) [3], and stem cells from adipose tissue for therapeutic purposes [4]. Various cell types, such as bone marrow, adipocytes, and skin fibroblasts, have been utilized to generate these stem cells. However, adopting collection methods that employ minimally invasive procedures is essential for their development. Urine-derived stem cells (USCs) are obtained from human urine through a noninvasive process [5], and cells can be acquired from donors without regard to their age, gender, or medical history [6]. Additionally, they share biological characteristics with MSCs [7]. The usefulness of USCs has been proven through animal studies to investigate their tissue-protective effects, such as hepatic injury, ischemic neurological dysfunction, ischemia-perfusion injury in kidneys, and cutaneous wound healing [8–11]. Besides direct differentiation by stem cells, various functions related to cytokines and microRNAs within exosomes derived from stem cells have also been documented [12, 13]. Several studies have examined the possible therapeutic benefits of MSCs and extracellular vesicles for inflammatory skin disorders, including AD and psoriasis [14]. However, the therapeutic potential of USCs for inflammatory skin diseases has not yet been fully explored.
Herein, we report the therapeutic effects of USCs-derived culture supernatants on mice with psoriasis-like dermatitis using our originally established human USCs model.
Materials and methods
Isolation and purification of USCs
Isolation and purification of USCs was performed as previously described [5, 6]. Human urine specimens were collected from a 38-year old female subject. To isolate USCs, the fresh urine samples (160 mL) was centrifuged for 5 min at 500 × g at room temperature. The supernatant was aspirated, and the pellet including cells was rinsed twice with phosphate buffered saline (PBS). Cells were grown in 24-well plates with a mixture of Keratinocyte Serum Free Medium (Gibco, United States), and Essential 6™ Medium (Gibco, United States) in a 1:1 ratio, along with 100 U/mL penicillin and 1 mg/mL streptomycin (Gibco), and 5% fatal bovine serum (Gibco). The cell suspension was seeded info 24-well plates or 6 cm dish or 10 cm dish and incubated at 37°C with 5% CO2. The cells were subcultured using 0.25% trypsin at a confluence level of approximately 80%, and the medium was replated every 2 days. Cells stocks were stored in liquid nitrogen for further examination.
Flow cytometry analysis
To examine the manifestation of surface markers of USCs, cells were subjected to consecutive incubation with Alexa Fluor® 488-conjugated anti-human CD29, CD34, CD44, CD45, HLA-DR, and FITC-conjugated CD73, CD90 antibodies (Abs) or no staining, followed by flow cytometric analysis using an Attune Flow Cytometer (Life, United States). The data was analyzed utilizing FlowJo™ software program (Version 10.9.0, BD, Biosciences).
Differentiation assay
In order to evaluate the differentiation potential of MSC, we employed the Human Mesenchymal Stem Cell Functional Identification Kit (R&D systems) in accordance with the manufacturer’s guidelines. USCs were cultured in an adipogenic differentiation medium, which comprised a mixed culture medium supplemented with 10% FBS, hydrocortisone, isobutyl methylxanthine, and indomethacin (R&D systems). The medium was replaced every 3 days. After 21 days of induction, the cells were fixed in a solution of 4% paraformaldehyde (PFA) for 10 min and subsequently stained with oil-red-O for 15 min at room temperature. For immunostaining, cells were stained with anti-mouse FABP4 Ab (R&D systems). For osteogenic differentiation, USCs were cultured in a specialized osteogenic differentiation medium, which consisted of a mixture of culture medium supplemented with β-glycerolphosphate, ascorbate-phosphate, recombinant human BMP-2, and 10% FBS. The medium was replaced every 3 days for 2 weeks. Cells were fixed with cold 70% ethanol and then stained with 40 mM alizarin red S (pH 4.2) for 15 min, followed by fixation with 4% PFA. Cells were immunostained with anti-human Aggrecan antibodies (R&D Systems). USCs were cultured in a pellet culture technique to promote chondrogenic differentiation, utilizing a chondrogenic differentiation medium that comprised of mixed culture medium complemented with ascorbate-phosphate, pyruvate, recombinant human TGF-β3, proline, and dexamethasone. The medium was replaced every 3 days for 3 weeks. Following the fixation of pallets with 4% PFA, they were embedded in an optimum cutting temperature compound and 10 µm frozen sections were stained with anti-human Osteocalcin Ab (R&D systems).
Animals
Eight-to 12 weeks-old female BALB/C mice were obtained from the SLC (Shizuoka, Japan). Mice were maintained in the Institute of Experimental Animal Research at Gunma University under specific pathogen-free conditions, and they were handled in accordance with the animal care guidelines established by Gunma University.
Psoriasis-like dermatitis mice model
Psoriasis mouse model experiments were performed using IMQ cream (5% Beselna Cream, Mochida Pharmaceuticals, Tokyo, Japan) described previously [15]. Topical application of IMQ cream (62.5 mg/day) was performed to on the back skin for a period of 6 days in a row. The evaluation of dermatitis severity was conducted using the Psoriasis Severity (PSI) Score, which ranges from 0 to 4, as previously detailed [16]. To examine the influence of USCs-conditioned medium (CM) on the development of dermatitis in mice, 200 µL of USCs-CM or the same volume of cell culture medium as control was administered subcutaneously at four sites, each comprising 50 μL, in the back subcutaneous tissue just after IMQ treatment.
Antibodies
The following antibodies (Abs) were used: myeloperoxidase (MPO) Abs (Thermo Scientific, RB-373-A0), CD3 (Abcam, ab5690), CD68 Abs (Bio-Rad Laboratories, MCA1957GA), CD29 Abs (Biolegend, #303015), CD44 Abs (Biolegend, #338829), CD73 Abs (Biolegend, #344015), CD90 Abs (Biolegend, #328107), CD34 Abs (Biolegend, #343517), CD45 Abs (Biolegend, #304019), HLA-DR Abs (Biolegend, #307619), FABP4 Abs (R&D systems, #967799), Osteocalcin Abs (R&D systems, #967801), Aggrecan Abs (R&D systems, #967800). Alexa Fluor® 488 or 568 secondary Abs (Invitrogen, Carlsbad, California).
Histological and immunohistochemical examination
Histological and immunohistochemial examinations were performed as previously described [16–18]. Skin tissues were excised, treated with formalin, and then embedded in paraffin blocks. Paraffined embedded sections were stained using hematoxylin and eosin (H&E). Six random microscopic images per slide were obtained to measure the epidermal thickness. Calculation was performed using ImageJ software (Version 1.54) in H&E-stained sections of control group (n = 7) and USCs-CM group (n = 6). For immunohistochemical staining, sections were subjected to deparaffinization and heating for 10 min with citrate buffer or Tris-EDTA (pH 8.0) buffer. Blocking step was performed using endogenous peroxidase blocking solution and protein block solution (Dako). The specified antibodies (Abs) were incorporated into the appropriate sections and allowed to incubate overnight at a temperature of 4°C. Subsequently, these sections were exposed to horseradish peroxidase (HRP)-labeled secondary antibodies. The quantification of inflammatory cells (CD3+ T-cells, MPO+ neutrophils and CD68+ macrophages) in the dermis was carried out by counting the numbers of positive cells. Six microscopic images were obtained in one slide. n = 3 for CD3, CD68 and n = 6 mice for MPO per group. Immunofluorescence staining protocol of cryostat sections and subsequent analyses were carried out in accordance with previously described [17]. Sections were stained with Alexa Fluor 568- conjugated secondary antibodies, followed by staining with 4,6-diamidino 2-phenylindole (DAPI) to visualize nuclei. Finally, sections were mounted in a Pro-Long Gold antifade reagent (Life Technologies). Immunofluorescence images were acquired and visualized using an FV10i-DOC confocal laser scanning microscope (Olympus).
Real-time reverse transcriptase PCR
RNA collection and RT-qPCR experiments were performed as previously described [16, 18]. Total RNA from skin tissue was obtained using the RNeasy Mini Kit (Qiagen, Valencia, California), and then reverse transcription using a GoScript Reverse Transcription System for RT-PCR (Promega) as previously described. We examined RT-qPCR experiment with the SYBR system (Applied Biosystems, Foster City, California) using Applied Biosystems 7300 Real-Time PCR System (Life Technologies). SYBR probes and primers for IL-17A, IL-17F, IL-23p19, and 18S were purchased from Takara Bio (Otsu, Japan) Inc and Sigma (St. Louis, Missouri). The levels of 18S mRNA were quantified in parallel with the target genes as an internal control. The normalization and fold-changes were calculated using the comparative Ct method.
Statistics
All statistical analyses were performed using GraphPad Prism software (Version 10) with one-way analysis of variance followed by Bonferroni test, non-parametric Mann–Whitney U test, or Student's t-test (two-sided). Error bars indicate standard errors of the mean. The numbers of experiments (n) are as described below. indicated. Figure 1: (B) n = 3. Figure 2: (A) n = 3, (B-D) n = 3. Figure 3: (A) n = 7 for control, n = 6 for USCs-CM. Figure 4: (A) n = 7 for control, n = 6 for USCs, (B) n = 3 per group, (C) n = 6 per group, (D) n = 3 per group. Figure 5: n = 6 for no treatment, n = 8 for ctl, and n = 10 for USCs-CM.
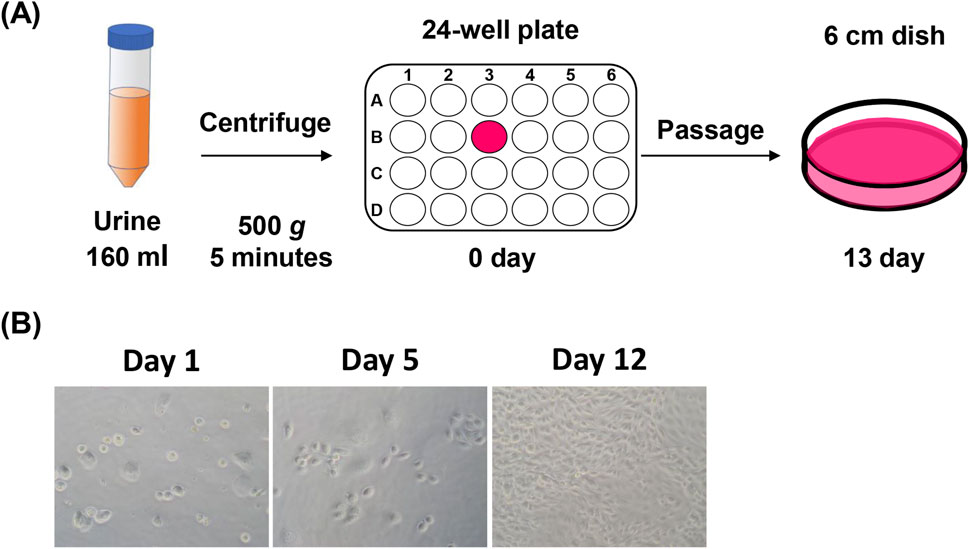
Figure 1. Collection and establishment of USCs from human urine samples. (A) Schematic representation of isolation and incubation process of urine-derived stem cells (USCs). (B) Photographs illustrating cell morphology in a time series on days 1, 5, and 12 after seeding; n = 3.
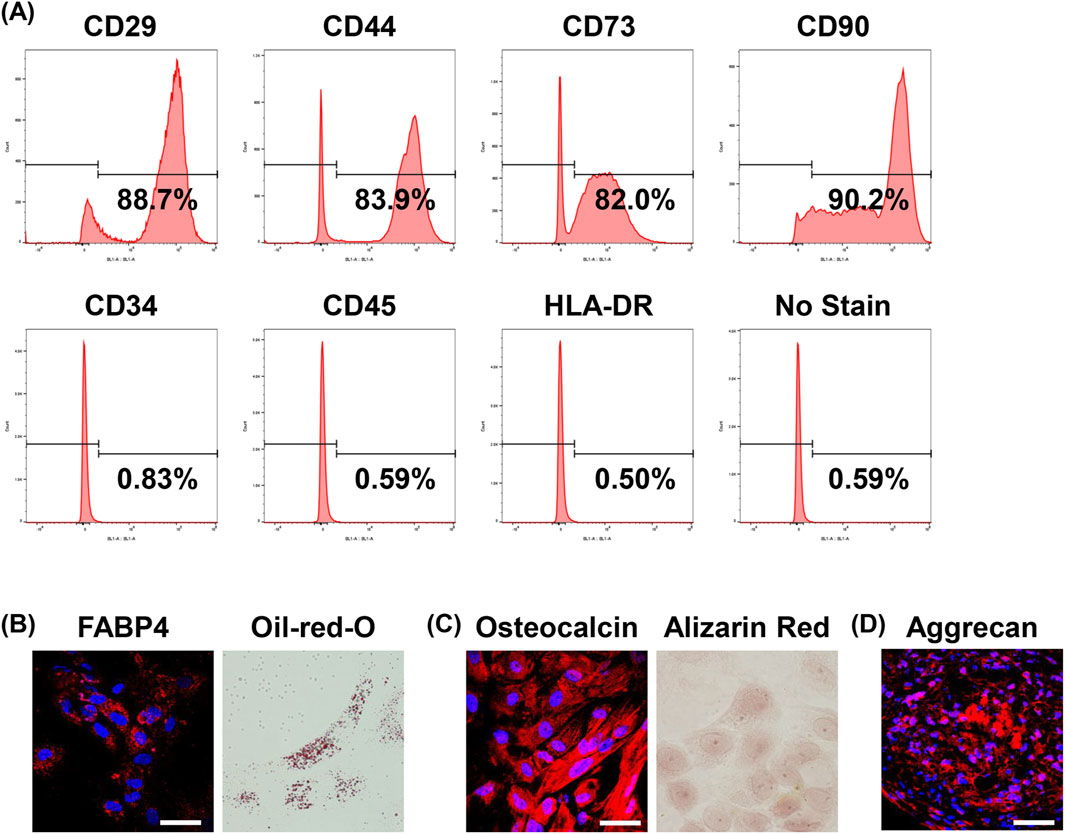
Figure 2. Investigation of the characteristics of human USC. (A) Representative flow cytometry analyses of the cell surface expression of CD29, CD34, CD44, CD45, CD73, CD90, HLA-DR, and no staining as a negative control in USCs; n = 3. (B) Adipogenic differentiation was assessed by immunostaining for FABP4 (left) and Oil-red-O staining (right). Bar = 50 μm; n = 3. (C) Osteogenic differentiation was assessed by immunostaining for osteocalcin (left) and Alizarin Red staining (right). Bar = 50 μm; n = 3. (D) Chondrogenic differentiation was assessed by immunostaining for Aggrecan. Bar = 50 μm; n = 3.
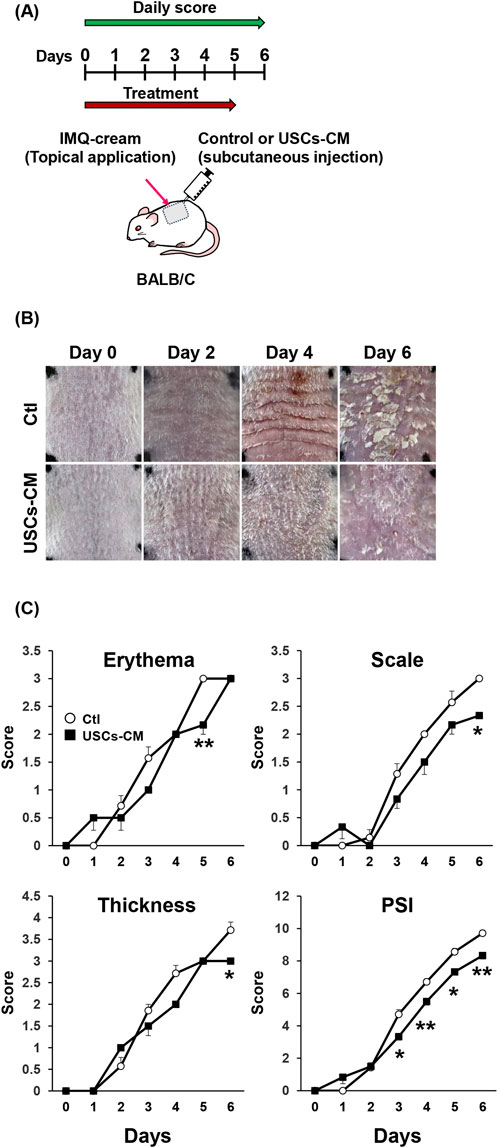
Figure 3. Therapeutic potential of USCs-conditioned medium (CM) on IMQ-treated psoriasis-like dermatitis. (A) Time course in in vivo experiments. USCs-CM or control medium was administered by subcutaneous injection and topical application of IMQ cream on the back skin for six consecutive days. (B) Representative images of skin manifestation on days 0, 2, 4, and 6 in control or USCs-CM-treated mice. (C) Skin erythema, scaling, thickness, and cumulative PSI score are depicted; n = 7 for the control and n = 6 for the USCs-CM group. All data are expressed as mean ± standard error of the mean. *P < 0.05 and **P < 0.01.
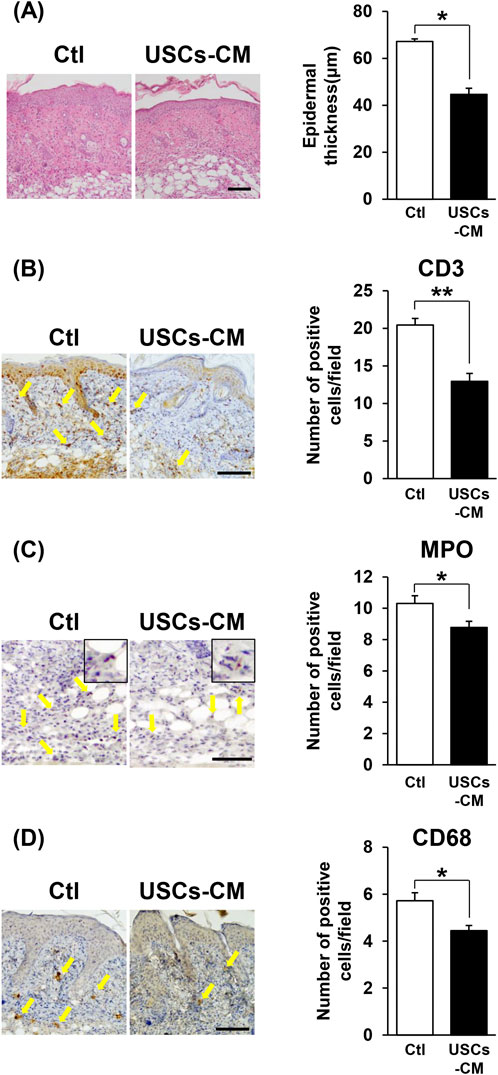
Figure 4. USCs-conditioned medium treatment attenuated epidermal thickness and inflammatory cell infiltration in the dermatitis-affected area in IMQ-treated mice with psoriasis. (A) Representative H&E images of skin sections from mice treated with control or USCs-CM on day 6. The right panel depicts the quantification of epidermal thickness in each group, with n = 7 for the control group and n = 6 for the USCs-CM group. Bar = 100 μm. (B–D) Immunohistochemical images showing infiltrating inflammatory cells stained for (B) CD3+ T-cells, (C) MPO+ neutrophils, and (D) CD68+ macrophages on days 6. Allows indicate positive cells for each staining (yellow). The right panels show quantitative evaluation by counting the number of positive cells in six random fields of view; n = 3 for (B) and (D). n = 6 for (C). Scale bar = 100 μm for (B) and (D), and 50 μm for (C). All data are expressed as mean ± standard error of the mean. *P < 0.05 and **P < 0.01.
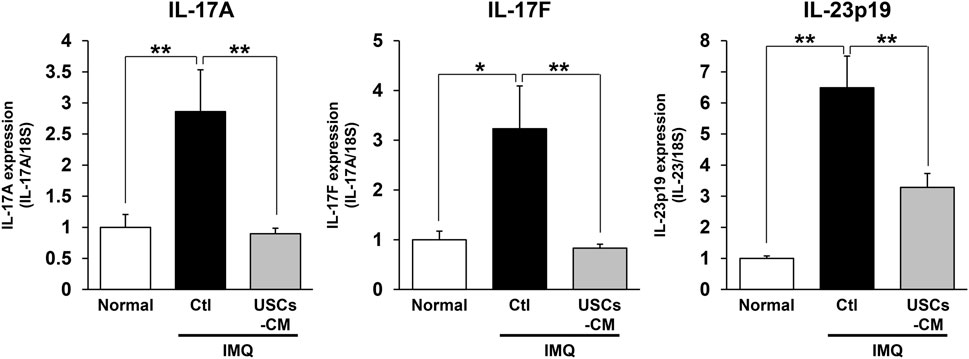
Figure 5. USCs-conditioned medium treatment attenuated IL-17 and IL-23 expression in lesional skin site in IMQ-treated psoriasis mice. Quantification of mRNA expression levels in the dermatitis area on day 4 using skin tissue from ctl or USCs-CM mice by qPCR. n = 6 for normal, n = 8 for ctl, and n = 10 for USCs-CM. All data are expressed as mean ± standard error of the mean. *P < 0.05 and **P < 0.01.
Results
Collection and establishment of USCs from human urine samples
First, we examined the isolation of USCs from 160 mL of human urine using a simple centrifugation process (Figure 1A). After washing with PBS, cells were cultured in 24-well plastic plates for 12 days. At 12 days after seeding, cells reaching 90%–95% confluency were passaged to a 6 cm dish with minimal processing. During the incubation, round-shaped cells accumulated and formed clusters (Figure 1B).
Investigation of the characteristics of human USC
Next, we investigated the cell surface markers and performed differentiation analysis. MSCs-like cell surface markers, including CD29, CD44, CD73, and CD90, were positive in about 80–90% of cells. However, the markers of hematopoietic stem cell, including CD34, CD45, and HLA-DR, were not expressed by FACS analysis (Figure 2A).
The differentiation potential was investigated in vitro. Adipogenic differentiation was evaluated by immunostaining for FABP4, a known marker of adipose cells, and Oil-Red-O staining (Figure 2B). Osteogenic differentiation was confirmed by immunostaining for osteocalcin, a marker of osteocytes, and Alizarin Red S staining (Figure 2C). Chondrogenic differentiation was assessed by Aggrecan (Figure 2D).
USCs-conditioned medium treatment suppressed the severity of dermatitis in IMQ-treated psoriasis mice model
Several studies have shown the therapeutic potential of conditioned medium of MSCs and USCs in multiple inflammatory disease animal models, such as wound healing, and radiation injury [19, 20]. Then, we assessed the therapeutic effects of the conditioned medium of USCs in the IMQ-treated mice model. USCs-conditioned medium (USCs-CM) and cell-free medium, used as a control (Ctl), were subcutaneously injected into the dorsal skin every day (Figure 3A). The results indicated that treatment with USCs-CM attenuated the severity of IMQ-induced psoriasis-like dermatitis compared to that observed in control mice (Figure 3B). In mice treated with USCs-CM, skin erythema was significantly inhibited on days 3 and 6. Additionally, skin thickness and scale were significantly suppressed on day 6 compared to control mice. The total PSI score demonstrated a significant inhibition in the progression of skin dermatitis on USCs-CM-treated animals from day 5 to day 6 (Figure 3C).
USCs-conditioned medium treatment attenuated acanthosis and inflammatory cells infiltration in dermatitis-affected areas in IMQ-treated psoriasis mice
To investigate the influence of USCs-CM treatment on the pathological changes in the dermatitis-affected area, skin samples were collected on day 6 and subjected to H&E staining. The histological analysis showed a significant reduction in epidermal thickness within the dermatitis-affected area of IMQ-treated mice after USCs-CM treatment, compared to control mice (Figure 4A). Moreover, immunohistochemical studies demonstrated a significant reduction in the number of infiltrated CD3+ T-cells, MPO+ neutrophils, and CD68+ macrophages in the skin following USCs-CM treatment (Figures 4B–D). These findings suggest that USCs-CM treatment modulates keratinocyte function and reduces skin inflammation, thereby improving IMQ-indued dermatitis.
USCs-conditioned medium treatment attenuated IL-17 and IL-23 expression in lesional skin site in IMQ-treated psoriasis mice
Finally, we assessed the effect of USCs-CM treatment on the mRNA levels of proinflammatory cytokines in lesional skin site in IMQ-treated psoriasis mice with RT-PCR assay. The result indicated that elevated mRNA expression of proinflammatory cytokines, including IL-17A, IL-17F, and IL-23p19 by imiquimod treatment in ctl mice was significantly decreased in USCs-CM-treated mice on day 4 (Figure 5).
Discussion
This study demonstrated the establishment of USCs in adult human and investigated the suppressive effect of originally generated USCs-CM in IMQ treatment-induced psoriasis-like dermatitis mouse model. Additionally, the potential therapeutic effects of USCs-CM were investigated by utilizing skin tissue samples obtained from the dermatitis-affected region.
The characteristics of MSCs are defined as follows: 1) they can attach to plastic culture bottles; 2) at least 95% of the cell population expresses specific surface antigens, including CD73, CD90, and CD105, while not expressing CD11b, CD14, CD19, CD34, CD45, CD79α, or HLA-II antigens; and 3) they have ability to differentiated into adipocytes, chondrocytes, and osteocytes [21]. Several studies reported the distinctive markers of USCs, such as positivity for CD29, CD44, CD54, CD73, CD90, CD105, CD 166, STRO-1, and HLA-DR, and negativity for CD11b, CD14, CD19, CD31, CD34, CD43, and CD 45 [5, 22, 23]. Our urine-derived cells meet the specified criteria for adhesion, cell surface markers, and differentiation potential, thereby demonstrating their identity as USCs.
The results demonstrated a significant attenuation in intension of skin dermatitis and inflammation in USCs-CM-treated animal with IMQ treatment induced psoriasis mouse model. Neutrophils infiltrating the skin lesions of psoriasis are mediators of local inflammation and are induced by chemokines, such as CXCL1. Neutrophils contribute to psoriasis pathogenesis by activating dendritic cells through antimicrobial peptides, such as LL-37, participating in IL-36 activation, and inducing tissue damage through the development of neutrophil extracellular traps [24–27]. T-cells activated by IL-12 and IL-23, especially Th17 cells producing IL-17 and Th22 cells producing IL-22, are pivotal in the pathogenesis of psoriasis, resulting in the hyperactivation of keratinocytes [28]. Macrophages migrate to inflammatory sites upon stimulation by chemokines, such as MCP-1. They produce cytokines including TNF-α, IL-23, and IL-6, and, which contribute to the formation of the local inflammatory environment in psoriasis [29, 30]. The decreased numbers of inflammatory cell in the psoriasis-affected skin could reduce the severity of dermatitis in the IQM-mice model. Results showed the decreased mRNA levels of IL-17A, IL-17F and IL-23 expression in USCs-CM treatment, indicating that USCs-CM might inhibit IL-23/Th17 axis in imiquimod-induced psoriasis mice.
An interesting case study demonstrated complete regression in patients with psoriasis vulgaris after the topical application, but not local injection, of concentrated adipose tissue-derived MSCs-CM [31]. Moreover, the phase I clinical study demonstrated that subcutaneous administration of allogenic adipose-derived MSCs into psoriasis skin site in humans decreased PASI score and the expression levels of psoriasis associated cytokines mRNA, such as IL-17, IL-23, and TNF-α. Additionally, it increased the numbers of FOXP3+ regulatory T-cells at the local skin site [32]. These results suggest that MSCs and/or MSCs-CM may hold therapeutic potential for inflammatory skin disorders by modulating the local immunological environment. To our knowledge, our study represents a novel exploration as there have been no reports investigating the therapeutic potentials of USCs-CM on psoriasis.
Within a decade, the treatment of psoriasis has evolved innovatively with the advent of systemic therapies, including antibody drugs targeting the IL23/Th17 axis and JAK inhibitors. Although biologics provide safety and high therapeutic efficacy, their long-term use, the occurrence of secondary ineffectiveness upon treatment discontinuation and resumption, and the high cost of medical care pose challenges. When treating patients with JAK inhibitors, healthcare providers should always consider adverse events, such as heart disease and blood clots, which are highlighted in the black box warning issued by the U.S. Food and Drug Administration [33]. Hence, the ongoing necessity for developing new treatments that are effective, long-lasting, and safe remains evident. Psoriasis, a chronic inflammatory skin condition, is commonly associated with metabolic abnormalities, including diabetes, dyslipidemia, and obesity. This relationship is widely acknowledged. [34]. Given the ongoing global research into the therapeutic potential of stem cells or its supernatants in diabetes mellitus, there also exists potential for new therapeutic applications in psoriasis [35–37].
In summary, our findings revealed new potential strategies for utilizing USCs and USCs-CM as therapeutic agents for inflammatory skin diseases, including psoriasis.
Limitations
First, we conducted experiments using USCs from an individual. It would be necessary to repeat experiments using newly generated USCs from different participants. Second, since we have not investigated the therapeutic potential of USCs-CM in humans, we should carefully consider its clinical application in future projects. Third, we did not examine the depth of the immunological aspect of psoriasis in a mouse model. Further investigation is necessary to examine the underlying mechanisms responsible for the suppressive effect of USCs-CM on psoriasis. In addition, a detailed examination of cytokines, mRNAs, and other components of cell supernatants is required.
Data availability statement
The data that support the findings of this study are available from the corresponding author AU, upon reasonable request.
Ethics statement
The studies involving humans were approved by the ethics committee of Gunma University (HS2022-058). The studies were conducted in accordance with the local legislation and institutional requirements. The participants provided their written informed consent to participate in this study. The animal study was approved by the Gunma University Animal Care and Experimentation Committee (22-050). The study was conducted in accordance with the local legislation and institutional requirements.
Author contributions
All authors contributed to the connection, interpretation of the studies and analysis of the data and review of the manuscript; MN, AU, and S-iM wrote the manuscript. All authors contributed to the article and approved the submitted version.
Funding
The author(s) declare that financial support was received for the research, authorship, and/or publication of this article. This research was funded by Management Expenses Grants for Gunma University to S-iM.
Acknowledgments
We would like to thank Enago (http://www.enago.jp) for the English language review.
Conflict of interest
The authors declare that the research was conducted in the absence of any commercial or financial relationships that could be construed as a potential conflict of interest.
References
1. Aguirre, M, Escobar, M, Forero Amézquita, S, Cubillos, D, Rincón, C, Vanegas, P, et al. Application of the yamanaka transcription factors Oct4, Sox2, Klf4, and c-Myc from the laboratory to the clinic. Genes (Basel) (2023) 14(9):1697. doi:10.3390/genes14091697
2. Takahashi, K, and Yamanaka, S. Induction of pluripotent stem cells from mouse embryonic and adult fibroblast cultures by defined factors. Cell (2006) 126(4):663–76. doi:10.1016/j.cell.2006.07.024
3. Phinney, DG, and Prockop, DJ. Concise review: mesenchymal stem/multipotent stromal cells: the state of transdifferentiation and modes of tissue repair--current views. Stem Cells (2007) 25(11):2896–902. doi:10.1634/stemcells.2007-0637
4. Mizuno, H, Tobita, M, and Uysal, AC. Concise review: adipose-derived stem cells as a novel tool for future regenerative medicine. Stem Cells (2012) 30(5):804–10. doi:10.1002/stem.1076
5. Zhang, Y, McNeill, E, Tian, H, Soker, S, Andersson, KE, Yoo, JJ, et al. Urine derived cells are a potential source for urological tissue reconstruction. J Urol (2008) 180(5):2226–33. doi:10.1016/j.juro.2008.07.023
6. Chun, SY, Kim, HT, Lee, JS, Kim, MJ, Kim, BS, Kim, BW, et al. Characterization of urine-derived cells from upper urinary tract in patients with bladder cancer. Urology (2012) 79(5):1186.e1–7. doi:10.1016/j.urology.2011.12.034
7. Chen, AJ, Pi, JK, Hu, JG, Huang, YZ, Gao, HW, Li, SF, et al. Identification and characterization of two morphologically distinct stem cell subpopulations from human urine samples. Sci China Life Sci (2020) 63(5):712–23. doi:10.1007/s11427-018-9543-1
8. Fu, Y, Guan, J, Guo, S, Guo, F, Niu, X, Liu, Q, et al. Human urine-derived stem cells in combination with polycaprolactone/gelatin nanofibrous membranes enhance wound healing by promoting angiogenesis. J Transl Med (2014) 12:274. doi:10.1186/s12967-014-0274-2
9. Hu, C, He, Y, Fang, S, Tian, N, Gong, M, Xu, X, et al. Urine-derived stem cells accelerate the recovery of injured mouse hepatic tissue. Am J Transl Res (2020) 12(9):5131–50.
10. Li, X, Liao, J, Su, X, Li, W, Bi, Z, Wang, J, et al. Human urine-derived stem cells protect against renal ischemia/reperfusion injury in a rat model via exosomal miR-146a-5p which targets IRAK1. Theranostics (2020) 10(21):9561–78. doi:10.7150/thno.42153
11. Wu, R, Luo, S, Yang, H, Hu, X, Lin, A, Pan, G, et al. Transplantation of neural progenitor cells generated from human urine epithelial cell-derived induced pluripotent stem cells improves neurological functions in rats with stroke. Discov Med (2020) 29(156):53–64.
12. Suto, N, Mieda, T, Iizuka, A, Nakamura, K, and Hirai, H. Morphological and functional attenuation of degeneration of peripheral neurons by mesenchymal stem cell-conditioned medium in spinocerebellar ataxia type 1-Knock-in mice. CNS Neurosci Ther (2016) 22(8):670–6. doi:10.1111/cns.12560
13. Baral, H, Uchiyama, A, Yokoyama, Y, Sekiguchi, A, Yamazaki, S, Amalia, SN, et al. Antifibrotic effects and mechanisms of mesenchymal stem cell-derived exosomes in a systemic sclerosis mouse model: possible contribution of miR-196b-5p. J Dermatol Sci (2021) 104(1):39–47. doi:10.1016/j.jdermsci.2021.08.006
14. Yang, J, Xiao, M, Ma, K, Li, H, Ran, M, Yang, S, et al. Therapeutic effects of mesenchymal stem cells and their derivatives in common skin inflammatory diseases: atopic dermatitis and psoriasis. Front Immunol (2023) 14:1092668. doi:10.3389/fimmu.2023.1092668
15. van der Fits, L, Mourits, S, Voerman, JS, Kant, M, Boon, L, Laman, JD, et al. Imiquimod-induced psoriasis-like skin inflammation in mice is mediated via the IL-23/IL-17 axis. J Immunol (2009) 182(9):5836–45. doi:10.4049/jimmunol.0802999
16. Amalia, SN, Baral, H, Fujiwara, C, Uchiyama, A, Inoue, Y, Yamazaki, S, et al. TRPV4 regulates the development of psoriasis by controlling adenosine triphosphate expression in keratinocytes and the neuroimmune system. J Invest Dermatol (2023) 143(12):2356–65.e5. doi:10.1016/j.jid.2023.05.009
17. Uchiyama, A, Yamada, K, Perera, B, Ogino, S, Yokoyama, Y, Takeuchi, Y, et al. Protective effect of MFG-E8 after cutaneous ischemia-reperfusion injury. J Invest Dermatol (2015) 135(4):1157–65. doi:10.1038/jid.2014.515
18. Inoue, Y, Uchiyama, A, Amalia, SN, Ishikawa, M, Kosaka, K, Sekiguchi, A, et al. Keratinocyte-specific SOX2 overexpression suppressed pressure ulcer formation after cutaneous ischemia-reperfusion injury via enhancement of amphiregulin production. J Invest Dermatol (2024) 144(1):142–51.e5. doi:10.1016/j.jid.2023.06.202
19. Brunchukov, V, Astrelina, T, Usupzhanova, D, Rastorgueva, A, Kobzeva, I, Nikitina, V, et al. Evaluation of the effectiveness of mesenchymal stem cells of the placenta and their conditioned medium in local radiation injuries. Cells (2020) 9(12):2558. doi:10.3390/cells9122558
20. Zhang, Y, Wang, J, Yang, B, Qiao, R, Li, A, Guo, H, et al. Transfer of MicroRNA-216a-5p from exosomes secreted by human urine-derived stem cells reduces renal ischemia/reperfusion injury. Front Cell Dev Biol (2020) 8:610587. doi:10.3389/fcell.2020.610587
21. Dominici, M, Le Blanc, K, Mueller, I, Slaper-Cortenbach, I, Marini, F, Krause, D, et al. Minimal criteria for defining multipotent mesenchymal stromal cells. the international society for cellular therapy position statement. Cytotherapy (2006) 8(4):315–7. doi:10.1080/14653240600855905
22. Bharadwaj, S, Liu, G, Shi, Y, Wu, R, Yang, B, He, T, et al. Multipotential differentiation of human urine-derived stem cells: potential for therapeutic applications in urology. Stem Cells (2013) 31(9):1840–56. doi:10.1002/stem.1424
23. Chen, JL, Li, N, Xu, M, Wang, L, Sun, J, Li, L, et al. Implantation of human urine stem cells promotes neural repair in spinal cord injury rats associated cadeharin-1 and integrin subunit beta 1 expression. J Gene Med (2024) 26(1):e3615. doi:10.1002/jgm.3615
24. Knight, JS, Carmona-Rivera, C, and Kaplan, MJ. Proteins derived from neutrophil extracellular traps may serve as self-antigens and mediate organ damage in autoimmune diseases. Front Immunol (2012) 3:380. doi:10.3389/fimmu.2012.00380
25. Ogawa, E, Sato, Y, Minagawa, A, and Okuyama, R. Pathogenesis of psoriasis and development of treatment. J Dermatol (2018) 45(3):264–72. doi:10.1111/1346-8138.14139
26. Ogawa, Y, Muto, Y, Kinoshita, M, Shimada, S, and Kawamura, T. Neutrophil extracellular traps in skin diseases. Biomedicines (2021) 9(12):1888. doi:10.3390/biomedicines9121888
27. Sachen, KL, Arnold Greving, CN, and Towne, JE. Role of IL-36 cytokines in psoriasis and other inflammatory skin conditions. Cytokine (2022) 156:155897. doi:10.1016/j.cyto.2022.155897
28. Nestle, FO, Kaplan, DH, and Barker, J. Psoriasis. N Engl J Med (2009) 361(5):496–509. doi:10.1056/NEJMra0804595
29. Fuentes-Duculan, J, Suárez-Fariñas, M, Zaba, LC, Nograles, KE, Pierson, KC, Mitsui, H, et al. A subpopulation of CD163-positive macrophages is classically activated in psoriasis. J Invest Dermatol (2010) 130(10):2412–22. doi:10.1038/jid.2010.165
30. Schultze, JL, Schmieder, A, and Goerdt, S. Macrophage activation in human diseases. Semin Immunol (2015) 27(4):249–56. doi:10.1016/j.smim.2015.07.003
31. Seetharaman, R, Mahmood, A, Kshatriya, P, Patel, D, and Srivastava, A. Mesenchymal stem cell conditioned media ameliorate psoriasis vulgaris: a case study. Case Rep Dermatol Med (2019) 2019:8309103. doi:10.1155/2019/8309103
32. Bajouri, A, Dayani, D, Taj Sharghi, A, Karimi, S, Niknezhadi, M, Moeinabadi Bidgoli, K, et al. Subcutaneous injection of allogeneic adipose-derived mesenchymal stromal cells in psoriasis plaques: clinical trial phase I. Cell J (2023) 25(6):363–71. doi:10.22074/cellj.2023.1973793.1167
33. Kragstrup, TW, Glintborg, B, Svensson, AL, McMaster, C, Robinson, PC, Deleuran, B, et al. Waiting for JAK inhibitor safety data. RMD Open (2022) 8(1):e002236. doi:10.1136/rmdopen-2022-002236
34. Alajroush, WA, Alrshid, AI, Alajlan, AH, Alsalamah, YB, Alhumaidan, MI, Alhoumedan, AI, et al. Psoriasis and metabolic disorders: a comprehensive meta-analysis of million adults worldwide. Cureus (2024) 16(1):e52099. doi:10.7759/cureus.52099
35. Izadi, M, Sadr Hashemi Nejad, A, Moazenchi, M, Masoumi, S, Rabbani, A, Kompani, F, et al. Mesenchymal stem cell transplantation in newly diagnosed type-1 diabetes patients: a phase I/II randomized placebo-controlled clinical trial. Stem Cell Res Ther (2022) 13(1):264. doi:10.1186/s13287-022-02941-w
36. Lian, XF, Lu, DH, Liu, HL, Liu, YJ, Yang, Y, Lin, Y, et al. Safety evaluation of human umbilical cord-mesenchymal stem cells in type 2 diabetes mellitus treatment: a phase 2 clinical trial. World J Clin Cases (2023) 11(21):5083–96. doi:10.12998/wjcc.v11.i21.5083
Keywords: psoriasis, mesenchymal stem cells, conditioned medium, urine-derived stem cells, imiquimod
Citation: NasanOchir M, Uchiyama A, Amalia SN, Ishikawa M, Nasanbat B, Taivanbat B, Kosaka K, Nishio M, Yokoyama Y, Ogino S, Torii R and Motegi S-i (2024) Human urine-derived stem cells: potential therapy for psoriasis-like dermatitis in mice. J. Cutan. Immunol. Allergy 7:13069. doi: 10.3389/jcia.2024.13069
Received: 31 March 2024; Accepted: 16 August 2024;
Published: 02 September 2024.
Copyright © 2024 NasanOchir, Uchiyama, Amalia, Ishikawa, Nasanbat, Taivanbat, Kosaka, Nishio, Yokoyama, Ogino, Torii and Motegi. This is an open-access article distributed under the terms of the Creative Commons Attribution License (CC BY). The use, distribution or reproduction in other forums is permitted, provided the original author(s) and the copyright owner(s) are credited and that the original publication in this journal is cited, in accordance with accepted academic practice. No use, distribution or reproduction is permitted which does not comply with these terms.
*Correspondence: Akihiko Uchiyama, akihiko1016@gunma-u.ac.jp