- 1The University of Ottawa Heart Institute, Ottawa, ON, Canada
- 2Department of Biochemistry, Microbiology and Immunology, The University of Ottawa, Faculty of Medicine, Ottawa, ON, Canada
Excess adiposity can contribute to metabolic complications, such as type 2 diabetes mellitus (T2DM), which poses a significant global health burden. Traditionally viewed as a chronic and irreversible condition, T2DM management has evolved and new approaches emphasizing reversal and remission are emerging. Bariatric surgery demonstrates significant improvements in body weight and glucose homeostasis. However, its complexity limits widespread implementation as a population-wide intervention. The identification of glucagon-like peptide 1 (GLP-1) and the development of GLP-1 receptor agonists (GLP-1RAs) have improved T2DM management and offer promising outcomes in terms of weight loss. Innovative treatment approaches combining GLP-1RA with other gut and pancreatic-derived hormone receptor agonists, such as glucose-dependant insulinotropic peptide (GIP) and glucagon (GCG) receptor agonists, or coadministered with amylin analogues, are demonstrating enhanced efficacy in both weight loss and glycemic control. This review aims to explore the benefits of bariatric surgery and emerging pharmacological therapies such as GLP-1RAs, and dual and triple agonists in managing obesity and T2DM while highlighting the caveats and evolving landscape of treatment options.
Introduction
Obesity represents a multifaceted, chronic condition characterized by an accumulation of excessive body fat, known as adiposity, which can impair health and decrease lifespan [1]. Epidemiologic studies define obesity using the body mass index (BMI), which can stratify obesity-related health risks at the population level. Obesity is clinically defined as a BMI exceeding 30 kg/m2 and is subdivided further into class 1 (30–34.9 kg/m2), class 2 (35–39.9 kg/m2) and class 3 (≥40 kg/m2). At the population level, complications from obesity rise as BMI increases [2]. At the individual level, the relationship between health complications and BMI is influenced by diverse factors such as the extent of adiposity, its distribution throughout the body, and an array of environmental, genetic, biological, and socioeconomic influences [3]. Excessive adiposity can predispose to metabolic complications, such as type 2 diabetes mellitus (T2DM) [4]. T2DM is defined by hyperglycemia resulting from tissue insulin resistance and relative insulin deficiency [4]. Estimates indicate that approximately 537 million individuals worldwide had T2DM in 2021, a figure that is expected to increase by 46%–783 million by 2045 [5]. Individuals with T2DM are at high risk for microvascular complications, including retinopathy, nephropathy and neuropathy, and macrovascular complications such as cardiovascular comorbidities [6].
For years, T2DM has been viewed as a chronic, progressive condition necessitating continual adjustment of pharmacotherapy, with estimates that 50% of patients will require insulin dependence within 9–10 years [7]. However, a growing body of research challenges this timeline by introducing surgical and pharmacotherapy approaches to managing the disease, emphasizing reversal and remission [8]. Indeed, sustained weight loss of at least 15% of body weight has a positive effect on the progression of T2DM, inducing remission in a large proportion of patients and markedly improving metabolic status in many others [9, 10]. The World Health Organization now openly acknowledges that a window of time exists in which T2DM is metabolically reversible - which is defined as a normal HbA1c without glucose-lowering medications for at least 3 months [11]. Pioneering work by Pories et al. [12] laid the foundation for the notion that bariatric surgery could effectively address T2DM owing to its substantial impact on weight reduction and significant improvements in blood glucose levels, fasting insulin, and HbA1c. Subsequent studies have consistently reaffirmed the efficacy of bariatric surgery in enhancing glucose homeostasis, diminishing the requirement for glucose-lowering medications, and mitigating both microvascular and macrovascular complications associated with T2DM [13]. Notably, some patients have experienced complete remission of T2DM following surgery [13]. Furthermore, evidence suggests that individuals undergoing bariatric surgery are significantly less likely to receive a diagnosis of T2DM even 15 years post-surgery compared to those who do not undergo the procedure [14]. Despite its considerable benefits, a complex surgical procedure is not feasible or scalable as the mainstay for a population-wide intervention.
The discovery that glucagon-like peptide-1 (GLP-1) enhances insulin secretion in a glucose-dependent manner and suppresses glucagon release while minimizing the risk of hypoglycemia has led to the development of various structurally distinct GLP-1 receptor (GLP-1R) agonists (GLP-1RAs) with longer circulation times for the management of T2DM [15–17]. Beyond their now well-defined role in managing glucose levels, GLP-1RAs have emerged as important tools in weight management strategies for individuals living with obesity and T2DM. This effect on body weight primarily stems from their ability to reduce food intake and slow gastric emptying [18]. Innovative treatment approaches combining GLP-1RAs with other gut hormone-derived agonists, such as glucose-dependent insulinotropic polypeptide (GIP), and pancreatic hormone-derived agonists, such as glucagon (GCG) and amylin, are demonstrating promising outcomes, further enhancing both weight loss and glycemic control [19, 20]. This new line of pharmaceuticals to reduce body weight and decrease glucose levels could therefore be a more accessible treatment alternative for individuals living with obesity and T2DM (Figure 1).
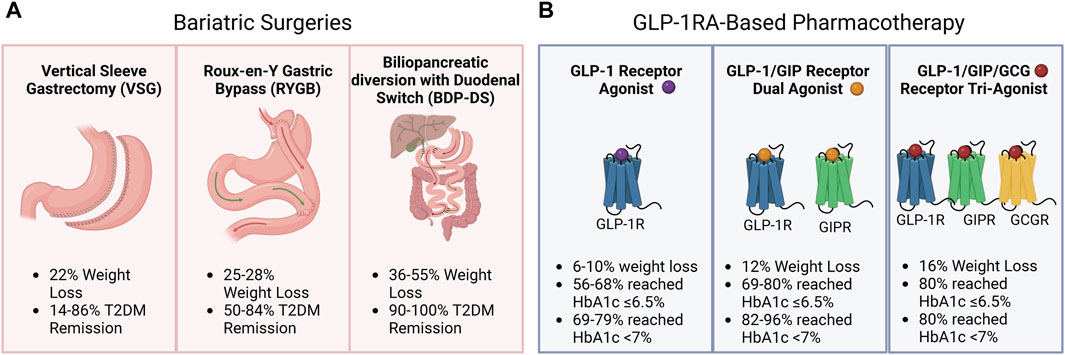
Figure 1. Weight loss and type 2 diabetes mellitus (T2DM) management with bariatric surgery and Glucagon-like peptide 1 receptor agonist (GLP-1RA)-based pharmacotherapy. (A) Weight loss (%) and type 2 diabetes mellitus (T2DM) remission (%) were observed following vertical sleeve gastrectomy, Roux-en-Y Gastric Bypass, or biliopancreatic diversion with duodenal switch. (B) Weight loss (%) and HbA1c targets of 6.5% and 7% reached by patients following GLP-1RA-based pharmacotherapy.
This review aims to explore bariatric surgery, currently considered the most effective intervention for addressing obesity and T2DM, and the potential pharmacological emerging therapies such as GLP-1 receptor agonists (GLP-1RAs), dual agonists, and tri-agonists in body weight and T2DM management. Additionally, we will discuss the caveats and potential future directions in treating patients living with obesity and T2DM.
Bariatric surgery
Bariatric surgery, also known as metabolic surgery, is an effective therapy that helps people with severe obesity achieve significant weight loss while decreasing related cardiometabolic comorbidities [21, 22]. The term metabolic surgery acknowledges the physiological changes caused by the procedure, which leads to a more favourable metabolic profile beyond the traditional belief that it is only provided through weight-dependant mechanisms [23–25]. The most widely performed bariatric surgeries are vertical sleeve gastrectomy (VSG), which consists of removing ∼80% of the stomach along the greater curvature, and Roux-en-Y gastric bypass (RYGB), which involves gastric size restriction with the creation of a small gastric pouch and re-routing of the intestinal tract, such that ingested nutrients empty directly into the jejunum and bypass 95% of the stomach, duodenum and proximal jejunum [26]. Biliopancreatic diversion with duodenal switch (BPD-DS) is a less-common procedure consisting of a sleeve gastrectomy followed by re-routing of the small intestine so that the ileum now connects to the pylorus of the stomach, bypassing both the jejunum and the duodenum [27].
Studies have shown that patients living with T2DM undergoing BDP-DS tend to lose between 36% and 55% of their initial body weight after 10 and 3 years, respectively [28, 29], compared to 28% with RYBG [30] and 22% with sleeve gastrectomy after 10 years [30]. Similarly, BPD-DS is the procedure conferring the highest rate of long-term (2–5 years) diabetes remission, ranging from 90 to 100% [27, 31] compared to 50–84% [29, 32] for RYGB and 14–86% for sleeve gastrectomy [33–35]. The longer duration of diabetes and the type of antidiabetic therapy used before surgery could influence postsurgical glycemic outcomes, thus explaining the heterogeneity in diabetes remission following bariatric surgery [36–38]. Despite being recognized for its durability in terms of weight loss and diabetes remission [29, 39, 40], DPB-DS constitutes only 2.2% of bariatric surgeries performed worldwide [41]. The technical complexity and demanding post-operative monitoring needed to avoid malnutrition due to the malabsorptive nature of this surgery may explain the reduced surgeries employing BPD-DS. As it is a more straightforward procedure that requires a shorter operative time, VSG is now the most widely performed bariatric surgery worldwide [21].
The precise mechanisms resulting in improved glucose control following bariatric surgery remain unclear. The degree of weight loss achieved is generally associated with the degree of resolution of T2DM [9, 42, 43], suggesting that those with greater weight loss after surgery have a greater propensity for improved management of T2DM and remission than those with less weight loss [44]. Indeed, weight loss yields a reduction in total, visceral and pancreatic adipose tissue, reductions in intrahepatic levels of lipids, and improved insulin sensitivity, all of which are expected to improve systemic glucose homeostasis [45]. One study demonstrated that in patients living with obesity and T2DM, 18% weight loss achieved either by RYGB or caloric restriction resulted in similar improvements in insulin sensitivity and β-cell function, suggesting that metabolic improvements are weight-related [44]. Metabolic surgery has also been found to have well-documented effects on improving blood glucose levels [13] and even achieving T2DM remission on a faster timeline that is disassociated from weight loss [21]. These weight-loss-independent improvements are thought to be in part related to changes in bile acid dynamics [46] and microbiota composition [47], a shift in gut physiology, including nutrient intake, gastric emptying, gastric acid production [48], and increases in postprandial gut hormone secretion [49]. Other factors to consider in T2DM remission following bariatric surgery include disease duration, age, and the level of glycemic control [9, 50]. These factors, linked to β-cell functional capacity, suggest that T2DM remission might be more achievable in patients with shorter disease duration, younger age, and better glycemic control. Nevertheless, it was reported in patients with T2DM using insulin before BPD-DS, 97% of patients had cessed insulin therapy after 10 years postoperatively [51].
Overall, the magnitude of change in body weight and glycemic control depends on the type of bariatric surgery performed and the improvements in T2DM management are related to both weight-loss-dependent and independent mechanisms.
GLP-1RA-based therapies
GLP-1 and GIP are incretin hormones released from gut enteroendocrine cells following a meal and potentiate glucose-dependent insulin secretion from the pancreas [52]. They exert their incretin actions through two distinct yet structurally related class B G protein-coupled receptors, the GIPR and the GLP-1R. These receptors are expressed in several organs tightly controlling energy homeostasis and metabolism, including the pancreas, cardiovascular system, and central and peripheral nervous system [52]. The essential role of incretin receptors in glucose homeostasis was demonstrated in single and double incretin receptor knockout mice. Glp1r−/− mice, and, to a greater extent, Glp1r−/− and Gipr−/− mice, exhibit impaired glucose tolerance and defective insulin secretion when fed a high-fat diet [53]. GLP-1 also exerts anorectic effects by activating GLP-1R + neurons in the hypothalamus and brainstem, which reduces food intake and promotes weight loss [54]. The action of GLP-1 to reduce glycemia by stimulating insulin secretion in a glucose-dependent manner provided the rationale for exploring incretin-based therapies and led to the approval of the first GLP-1R agonist in 2005 for treating T2DM [15]. The use of two GLP-1RAs, liraglutide and semaglutide, for weight loss was later approved in 2014 [55, 56].
The observed reduction in body weight with the use of the GLP-1RA liraglutide (1.2 and 1.8 mg once daily) in individuals living with T2DM prompted the exploration of higher doses of liraglutide in the treatment of overweight and obesity in the Satiety and Clinical Adiposity—Liraglutide Evidence (SCALE) program [55, 57–60]. In the Scale Diabetes trial, an average of 6% weight loss was achieved over 52 weeks in 623 individuals living with T2DM treated with 3 mg liraglutide once daily, with 25,2% of the participants experiencing >10% weight loss. Furthermore, 56.5% of participants receiving 3 mg liraglutide daily achieved a HbA1c ≤ 6.5%, which is considered prediabetic, compared to 15% in the placebo group, and 69.2% reached the target HbA1c <7% set by the American Diabetes Association (ADA) compared to 27.2% in the placebo group [58, 61].
The GLP-1RA semaglutide was also evaluated for the treatment of obesity in the Semaglutide Treatment Effect in People with Obesity (STEP) program at a dose of 2.4 mg once weekly [56, 62–64]. STEP 2 evaluated weight loss in 1,210 individuals living with T2DM and overweight/obesity not treated with insulin (HbA1c 7–10%). Participants were randomized to placebo, semaglutide 1 mg or semaglutide 2.4 mg weekly, together with lifestyle interventions over 68 weeks. Those receiving the highest dose lost an average of 9.6% of their body weight, compared to 3.4% with the placebo. At the highest dose, more than a quarter of the participants lost over 15% of their weight, almost half lost 10%, while two-thirds lost a minimum of 5%. After 68 weeks, participants receiving 2.4 mg had an average HbA1c of 6.4%, in the prediabetic range, and therefore below the threshold to diagnose T2DM, compared to 7.8% in the placebo group. After 68 weeks, 78.5% and 67.5% of those receiving 2.4 mg semaglutide weekly reached the <7% HbA1c target and ≤6.5% prediabetic range, respectively, compared to 26.5% in the placebo group [62].
The efficacy of GLP-1RA in managing body weight and T2DM has spurred significant efforts toward developing next-generation therapies that surpass the effectiveness of GLP-1RA alone. Tirzepatide, a novel dual GLP-1 and GIP analogue, was investigated at weekly subcutaneous doses of 5mg, 10mg and 15 mg compared to 1 mg semaglutide for 40 weeks in patients living with T2DM in the SURPASS phase 3 clinical trial program. The highest tirzepatide dose led to an 11.2 kg (11.9%) weight loss and decreased HbA1c by 2.3%. A total of 82–96% of the patients who received tirzepatide and 79% of those who received semaglutide reached the HbA1c target of <7.0%. Furthermore, HbA1c ≤ 6.5%%, which is considered prediabetic, was met in 69–80% of patients receiving tirzepatide compared to 64% of patients receiving semaglutide [65]. These findings are encouraging, highlighting the promising potential of tirzepatide in the management of T2DM.
Recently, tri-agonists (GLP-1/GIP/GCG) were shown to provide even greater improvements in glycemic control and robust reduction in body weight in individuals living with T2DM. In a phase 2 clinical trial including 281 participants with T2DM and a mean HbA1c of 8.3%, weekly administration of 12 mg retatrutide (starting dose 2 mg) for 36 weeks decreased HbA1c by 2.16% and participants lost ≥15% of body weight compared to baseline. Approximately 80% of those receiving the highest dose of retatrutide reached the <7.0% HbA1c target established by the ADA and roughly the same percentage attained the ≤6.5% HbA1c prediabetic level [66]. These outcomes align with the potential reversal of T2DM [10, 67]. Another study investigating the combination of semaglutide with the long-acting amylin analogue cagrilintide in patients living with T2DM also resulted in significant improvements in body weight and HbA1c in a phase 2 trial. Compared to baseline, once-weekly 2.4 mg of CagriSema for 32 weeks resulted in a 2.2% decrease in HbA1c (mean HbA1c of 6.3%) and a 15.6% body weight loss. Eighty-nine percent of patients achieved the <7% HbA1c target, and 75% had a HbA1c ≤ 6.5% considered in the prediabetic range [68].
While additional studies are required to validate the safety and effectiveness of these newer medications in larger cohorts, GLP-1RA-based pharmacotherapy represents a very promising avenue for managing body weight and T2DM.
Discussion
Bariatric surgery induces significant weight loss and T2DM remission (Figure 1A). However, there are several contraindications to bariatric surgeries, and not all patients may be eligible. As with any other medical intervention, bariatric surgery poses a health risk, such as postoperative surgical complications, and dumping syndrome, and patients need to be closely monitored for micronutrient deficiencies after the intervention [69]. Furthermore, surgical interventions are difficult to scale to reach everyone who could potentially benefit. It is therefore worth investigating if GLP-1RA-based pharmacotherapy could be a more accessible alternative to bariatric surgery for managing body weight and T2DM (Figure 1B).
Despite their safety and efficacy, individuals may experience adverse side effects using GLP-1R agonists, dual and tri-agonists, such as nausea, vomiting, constipation and diarrhea [70]. Furthermore, GLP-1RAs generally require once-weekly subcutaneous injections [55–60, 62–64]. Orally administered GLP-1RA, such as semaglutide and orforglipron represent another effective therapeutic strategy for managing body weight, blood glucose and other cardiometabolic risk factors [71, 72]. Additionally, an oral formulation for a GLP-1R/GIPR agonist is currently being tested in a phase 2 trial (ClinicalTrials.gov ID NCT06068946). One major limitation to the use of GLP-1RA-based therapies remains its high cost. A recent study even suggested that sleeve gastrectomy was cost-saving compared to semaglutide in the treatment of class II obesity and estimated that a 3-fold decrease in the price of semaglutide was needed to achieve nondominance [73]. Furthermore, long-term obesity and T2DM pharmacotherapy may also be required, as cessation of pharmacological treatment is frequently followed by weight regain, even with continued lifestyle intervention [64, 74]. Nevertheless, GLP-1RA-based pharmacotherapy remains a more accessible alternative than bariatric surgery for managing body weight and T2DM.
While both bariatric surgery and GLP-1RA-based pharmacotherapy represent promising options, surprisingly, few studies have directly compared surgery to pharmacotherapy for glycemic control and glycemic control in patients living with obesity and T2DM. Three studies have reported that RYGB and VSG surpass medical therapy in terms of weight loss, glycemic control and reduction in medical use among patients with T2DM [13, 38, 75]. However, it is important to mention that medical therapy, including various oral anti-hyperglycemic agents, insulin, GLP-1RAs and SGLT2 inhibitors, was heterogeneous across participants. Recent studies using GLP-1/GIP/GCG receptor agonists have demonstrated very promising results. It would therefore be interesting to explore whether these findings could be compared to the outcomes of surgery in regards to both weight loss and glycemic control.
In conclusion, bariatric surgery stands as a highly effective option for managing body weight and T2DM, yielding significant benefits. Yet, its widespread implementation faces scalability challenges, limiting access for many who could potentially benefit. In contrast, GLP-1RAs, and more particularly dual and triple agonists, offer a promising alternative, potentially extending to a larger patient population. Future research is imperative to ensure safety, and efficacy, and optimize treatment options, including decreasing side effects commonly reported by patients. Nevertheless, this novel pharmacotherapy could play a pivotal role in managing body weight, and T2DM, and preventing related micro- and macro-vascular complications.
Author contributions
AM and EM drafted and edited the manuscript. All authors contributed to the article and approved the submitted version.
Funding
The author(s) declare that financial support was received for the research, authorship, and/or publication of this article. Diabetes Canada grant and a Heart and Stroke New from a Investigator Award to EM.
Acknowledgments
The figure was produced using Biorender (Publication license: DB26MXS3PJ).
Conflict of interest
The authors declare that the research was conducted in the absence of any commercial or financial relationships that could be construed as a potential conflict of interest.
References
1. Whitlock, G, Lewington, S, Sherliker, P, Clarke, R, Emberson, J, Halsey, J, et al. Body-mass index and cause-specific mortality in 900 000 adults: collaborative analyses of 57 prospective studies. Lancet (2009) 373(9669):1083–96. doi:10.1016/s0140-6736(09)60318-4
2. Di Angelantonio, E, Bhupathiraju, SN, Wormser, D, Gao, P, Kaptoge, S, de Gonzalez, AB, et al. Body-mass index and all-cause mortality: individual-participant-data meta-analysis of 239 prospective studies in four continents. Lancet (2016) 388(10046):776–86. doi:10.1016/s0140-6736(16)30175-1
3. Sharma, AM. M, M, M and M: a mnemonic for assessing obesity. Obes Rev (2010) 11(11):808–9. doi:10.1111/j.1467-789x.2010.00766.x
4. James, DE, Stockli, J, and Birnbaum, MJ. The aetiology and molecular landscape of insulin resistance. Nat Rev Mol Cell Biol (2021) 22(11):751–71. doi:10.1038/s41580-021-00390-6
5. International Diabetes Federation. IDF diabetes atlas. 10th ed. (2021). Available from: https://diabetesatlas.org/data/en/world/ (Accessed May 16, 2024).
6. DeFronzo, RA, Ferrannini, E, Groop, L, Henry, RR, Herman, WH, Holst, JJ, et al. Type 2 diabetes mellitus. Nat Rev Dis Primers (2015) 1:15019. doi:10.1038/nrdp.2015.19
7. U.K. Prospective Diabetes Study Group. U.K. Prospective diabetes study 16: overview of 6 Years' therapy of type II diabetes: a progressive disease. Diabetes (1995) 44(11):1249–58. doi:10.2337/diab.44.11.1249
8. Hallberg, SJ, Gershuni, VM, Hazbun, TL, and Athinarayanan, SJ. Reversing type 2 diabetes: a narrative review of the evidence. Nutrients (2019) 11(4):766. doi:10.3390/nu11040766
9. Sjoholm, K, Sjöström, E, Carlsson, LM, and Peltonen, M. Weight change-adjusted effects of gastric bypass surgery on glucose metabolism: 2- and 10-year results from the Swedish obese subjects (SOS) study. Diabetes Care (2016) 39(4):625–31. doi:10.2337/dc15-1407
10. Lean, ME, Leslie, WS, Barnes, AC, Brosnahan, N, Thom, G, McCombie, L, et al. Primary care-led weight management for remission of type 2 diabetes (DiRECT): an open-label, cluster-randomised trial. The Lancet (2018) 391(10120):541–51. doi:10.1016/s0140-6736(17)33102-1
11. Riddle, MC, Cefalu, WT, Evans, PH, Gerstein, HC, Nauck, MA, Oh, WK, et al. Consensus report: definition and interpretation of remission in type 2 diabetes. Diabetic Med (2021) 39(10):2438–44. doi:10.1111/dme.14669
12. Pories, WJ, Macdonald, KG, Flickinger, EG, Dohm, GL, Sinha, MK, Barakat, HA, et al. Is type II diabetes mellitus (NIDDM) a surgical disease? Ann Surg (1992) 215(6):633–43. doi:10.1097/00000658-199206000-00010
13. Mingrone, G, Panunzi, S, De Gaetano, A, Guidone, C, Iaconelli, A, Capristo, E, et al. Metabolic surgery versus conventional medical therapy in patients with type 2 diabetes: 10-year follow-up of an open-label, single-centre, randomised controlled trial. The Lancet (2021) 397(10271):293–304. doi:10.1016/s0140-6736(20)32649-0
14. Carlsson, LM, Peltonen, M, Ahlin, S, Anveden, Å, Bouchard, C, Carlsson, B, et al. Bariatric surgery and prevention of type 2 diabetes in Swedish obese subjects. N Engl J Med (2012) 367(8):695–704. doi:10.1056/nejmoa1112082
15. Drucker, DJ, Habener, JF, and Holst, JJ. Discovery, characterization, and clinical development of the glucagon-like peptides. J Clin Invest (2017) 127(12):4217–27. doi:10.1172/jci97233
16. Drucker, DJ, and Nauck, MA. The incretin system: glucagon-like peptide-1 receptor agonists and dipeptidyl peptidase-4 inhibitors in type 2 diabetes. The Lancet (2006) 368(9548):1696–705. doi:10.1016/s0140-6736(06)69705-5
17. Ussher, JR, and Drucker, DJ. Cardiovascular actions of incretin-based therapies. Circ Res (2014) 114(11):1788–803. doi:10.1161/circresaha.114.301958
18. Drucker, DJ. GLP-1 physiology informs the pharmacotherapy of obesity. Mol Metab (2022) 57:101351. doi:10.1016/j.molmet.2021.101351
19. Lyons, SA, and Beaudry, JL. Synergistic combinations of gut- and pancreas-hormone-based therapies: advancements in treatments for metabolic diseases. Endocrinology (2023) 164(11):bqad153. doi:10.1210/endocr/bqad153
20. Baggio, LL, and Drucker, DJ. Glucagon-like peptide-1 receptor co-agonists for treating metabolic disease. Mol Metab (2021) 46:101090. doi:10.1016/j.molmet.2020.101090
21. Sandoval, DA, and Patti, ME. Glucose metabolism after bariatric surgery: implications for T2DM remission and hypoglycaemia. Nat Rev Endocrinol (2023) 19(3):164–76. doi:10.1038/s41574-022-00757-5
22. Aminian, A, Zajichek, A, Tu, C, Wolski, KE, Brethauer, SA, Schauer, PR, et al. How much weight loss is required for cardiovascular benefits? Insights from a metabolic surgery matched-cohort study. Ann Surg (2020) 272(4):639–45. doi:10.1097/sla.0000000000004369
23. Vidal, J, Corcelles, R, Jiménez, A, Flores, L, and Lacy, AM. Metabolic and bariatric surgery for obesity. Gastroenterology (2017) 152(7):1780–90. doi:10.1053/j.gastro.2017.01.051
24. Buchwald, H, and Buchwald, JN. Metabolic (bariatric and nonbariatric) surgery for type 2 diabetes: a personal perspective review. Diabetes Care (2019) 42(2):331–40. doi:10.2337/dc17-2654
25. Brethauer, SA, Kim, J, el Chaar, M, Papasavas, P, Eisenberg, D, Rogers, A, et al. Standardized outcomes reporting in metabolic and bariatric surgery. Surg Obes Relat Dis (2015) 11(3):489–506. doi:10.1016/j.soard.2015.02.003
26. Nguyen, NT, and Varela, JE. Bariatric surgery for obesity and metabolic disorders: state of the art. Nat Rev Gastroenterol Hepatol (2017) 14(3):160–9. doi:10.1038/nrgastro.2016.170
27. Marceau, P, Biron, S, Marceau, S, Hould, FS, Lebel, S, Lescelleur, O, et al. Biliopancreatic diversion-duodenal switch: independent contributions of sleeve resection and duodenal exclusion. Obes Surg (2014) 24(11):1843–9. doi:10.1007/s11695-014-1284-0
28. Scopinaro, N, Marinari, GM, Camerini, GB, Papadia, FS, and Adami, GF. Specific effects of biliopancreatic diversion on the major components of metabolic syndrome: a long-term follow-up study. Diabetes Care (2005) 28(10):2406–11. doi:10.2337/diacare.28.10.2406
29. Prachand, VN, Ward, M, and Alverdy, JC. Duodenal switch provides superior resolution of metabolic comorbidities independent of weight loss in the super-obese (BMI ≥ 50 kg/m2) compared with gastric bypass. J Gastrointest Surg (2010) 14(2):211–20. doi:10.1007/s11605-009-1101-6
30. Brethauer, SA, Aminian, A, Romero-Talamás, H, Batayyah, E, Mackey, J, Kennedy, L, et al. Can diabetes be surgically cured? Long-term metabolic effects of bariatric surgery in obese patients with type 2 diabetes mellitus. Ann Surg (2013) 258(4):628–37. doi:10.1097/sla.0b013e3182a5034b
31. Biertho, L, Lebel, S, Marceau, S, Hould, F, Lescelleur, O, Marceau, P, et al. Laparoscopic sleeve gastrectomy: with or without duodenal switch? A consecutive series of 800 cases. Dig Surg (2014) 31(1):48–54. doi:10.1159/000354313
32. Buchwald, H, Consensus Conference Panel. Bariatric surgery for morbid obesity: health implications for patients, health professionals, and third-party payers. J Am Coll Surgeons (2005) 200(4):593–604. doi:10.1016/j.jamcollsurg.2004.10.039
33. Brethauer, SA, Hammel, JP, and Schauer, PR. Systematic review of sleeve gastrectomy as staging and primary bariatric procedure. Surg Obes Relat Dis (2009) 5(4):469–75. doi:10.1016/j.soard.2009.05.011
34. Gan, SS, Talbot, ML, and Jorgensen, JO. Efficacy of surgery in the management of obesity-related type 2 diabetes mellitus. ANZ J Surg (2007) 77(11):958–62. doi:10.1111/j.1445-2197.2007.04290.x
35. Chang, SH, Stoll, CRT, Song, J, Varela, JE, Eagon, CJ, and Colditz, GA. The effectiveness and risks of bariatric surgery: an updated systematic review and meta-analysis, 2003-2012. JAMA Surg (2014) 149(3):275–87. doi:10.1001/jamasurg.2013.3654
36. Aminian, A, Brethauer, SA, Andalib, A, Punchai, S, Mackey, J, Rodriguez, J, et al. Can sleeve gastrectomy "cure" diabetes? Long-Term metabolic effects of sleeve gastrectomy in patients with type 2 diabetes. Ann Surg (2016) 264(4):674–81. doi:10.1097/sla.0000000000001857
37. Dixon, JB, and O'Brien, PE. Health outcomes of severely obese type 2 diabetic subjects 1 year after laparoscopic adjustable gastric banding. Diabetes Care (2002) 25(2):358–63. doi:10.2337/diacare.25.2.358
38. Schauer, PR, Bhatt, DL, Kirwan, JP, Wolski, K, Aminian, A, Brethauer, SA, et al. Bariatric surgery versus intensive medical therapy for diabetes - 5-year outcomes. N Engl J Med (2017) 376(7):641–51. doi:10.1056/nejmoa1600869
39. Kapeluto, JE, Tchernof, A, Masckauchan, D, Biron, S, Marceau, S, Hould, FS, et al. Ten-year remission rates in insulin-treated type 2 diabetes after biliopancreatic diversion with duodenal switch. Surg Obes Relat Dis (2020) 16(11):1701–12. doi:10.1016/j.soard.2020.06.052
40. Buchwald, H, Estok, R, Fahrbach, K, Banel, D, and Sledge, I. Trends in mortality in bariatric surgery: a systematic review and meta-analysis. Surgery (2007) 142(4):621–35. doi:10.1016/j.surg.2007.07.018
41. Marceau, P, Biron, S, Hould, FS, Lebel, S, Marceau, S, Lescelleur, O, et al. Duodenal switch: long-term results. Obes Surg (2007) 17(11):1421–30. doi:10.1007/s11695-008-9435-9
42. Blackstone, R, Bunt, JC, Cortés, MC, and Sugerman, HJ. Type 2 diabetes after gastric bypass: remission in five models using HbA1c, fasting blood glucose, and medication status. Surg Obes Relat Dis (2012) 8(5):548–55. doi:10.1016/j.soard.2012.05.005
43. Zechner, JF, Mirshahi, UL, Satapati, S, Berglund, ED, Rossi, J, Scott, MM, et al. Weight-independent effects of roux-en-Y gastric bypass on glucose homeostasis via melanocortin-4 receptors in mice and humans. Gastroenterology (2013) 144(3):580–90 e7. doi:10.1053/j.gastro.2012.11.022
44. Yoshino, M, Kayser, BD, Yoshino, J, Stein, RI, Reeds, D, Eagon, JC, et al. Effects of diet versus gastric bypass on metabolic function in diabetes. N Engl J Med (2020) 383(8):721–32. doi:10.1056/nejmoa2003697
45. Petrov, MS, and Taylor, R. Intra-pancreatic fat deposition: bringing hidden fat to the fore. Nat Rev Gastroenterol Hepatol (2022) 19(3):153–68. doi:10.1038/s41575-021-00551-0
46. Patti, ME, Houten, SM, Bianco, AC, Bernier, R, Larsen, PR, Holst, JJ, et al. Serum bile acids are higher in humans with prior gastric bypass: potential contribution to improved glucose and lipid metabolism. Obesity (Silver Spring) (2009) 17(9):1671–7. doi:10.1038/oby.2009.102
47. Anhe, FF, Varin, TV, Schertzer, JD, and Marette, A. The gut microbiota as a mediator of metabolic benefits after bariatric surgery. Can J Diabetes (2017) 41(4):439–47. doi:10.1016/j.jcjd.2017.02.002
48. Gala, K, Ghusn, W, and Abu Dayyeh, BK. Gut motility and hormone changes after bariatric procedures. Curr Opin Endocrinol Diabetes Obes (2024) 31(3):131–7. doi:10.1097/med.0000000000000860
49. Evers, SS, Sandoval, DA, and Seeley, RJ. The physiology and molecular underpinnings of the effects of bariatric surgery on obesity and diabetes. Annu Rev Physiol (2017) 79:313–34. doi:10.1146/annurev-physiol-022516-034423
50. Dang, JT, Sheppard, C, Kim, D, Switzer, N, Shi, X, Tian, C, et al. Predictive factors for diabetes remission after bariatric surgery. Can J Surg (2019) 62(5):315–9. doi:10.1503/cjs.014516
51. McGlone, ER, Carey, I, Veličković, V, Chana, P, Mahawar, K, Batterham, RL, et al. Bariatric surgery for patients with type 2 diabetes mellitus requiring insulin: clinical outcome and cost-effectiveness analyses. Plos Med (2020) 17(12):e1003228. doi:10.1371/journal.pmed.1003228
52. Hammoud, R, and Drucker, DJ. Beyond the pancreas: contrasting cardiometabolic actions of GIP and GLP1. Nat Rev Endocrinol (2023) 19(4):201–16. doi:10.1038/s41574-022-00783-3
53. Hansotia, T, Maida, A, Flock, G, Yamada, Y, Tsukiyama, K, Seino, Y, et al. Extrapancreatic incretin receptors modulate glucose homeostasis, body weight, and energy expenditure. J Clin Invest (2007) 117(1):143–52. doi:10.1172/jci25483
54. Sisley, S, Gutierrez-Aguilar, R, Scott, M, D’Alessio, DA, Sandoval, DA, and Seeley, RJ. Neuronal GLP1R mediates liraglutide's anorectic but not glucose-lowering effect. J Clin Invest (2014) 124(6):2456–63. doi:10.1172/jci72434
55. Pi-Sunyer, X, Astrup, A, Fujioka, K, Greenway, F, Halpern, A, Krempf, M, et al. A randomized, controlled trial of 3.0 mg of liraglutide in weight management. N Engl J Med (2015) 373(1):11–22. doi:10.1056/nejmoa1411892
56. Wilding, JPH, Batterham, RL, Calanna, S, Davies, M, Van Gaal, LF, Lingvay, I, et al. Once-weekly semaglutide in adults with overweight or obesity. N Engl J Med (2021) 384(11):989–1002. doi:10.1056/nejmoa2032183
57. le Roux, CW, Astrup, A, Fujioka, K, Greenway, F, Lau, DCW, Van Gaal, L, et al. 3 years of liraglutide versus placebo for type 2 diabetes risk reduction and weight management in individuals with prediabetes: a randomised, double-blind trial. The Lancet (2017) 389(10077):1399–409. doi:10.1016/s0140-6736(17)30069-7
58. Davies, MJ, Bergenstal, R, Bode, B, Kushner, RF, Lewin, A, Skjøth, TV, et al. Efficacy of liraglutide for weight loss among patients with type 2 diabetes: the SCALE diabetes randomized clinical trial. JAMA (2015) 314(7):687–99. doi:10.1001/jama.2015.9676
59. Blackman, A, Foster, GD, Zammit, G, Rosenberg, R, Aronne, L, Wadden, T, et al. Effect of liraglutide 3.0 mg in individuals with obesity and moderate or severe obstructive sleep apnea: the SCALE Sleep Apnea randomized clinical trial. Int J Obes (Lond) (2016) 40(8):1310–9. doi:10.1038/ijo.2016.52
60. Wadden, TA, Hollander, P, Klein, S, Niswender, K, Woo, V, Hale, PM, et al. Weight maintenance and additional weight loss with liraglutide after low-calorie-diet-induced weight loss: the SCALE Maintenance randomized study. Int J Obes (Lond) (2013) 37(11):1443–51. doi:10.1038/ijo.2013.120
61. American Diabetes Association. 6. Glycemic targets: standards of medical care in diabetes-2021. Diabetes Care (2021) 44(Suppl. 1):S73–S84. doi:10.2337/dc21-s006
62. Davies, M, Færch, L, Jeppesen, OK, Pakseresht, A, Pedersen, SD, Perreault, L, et al. Semaglutide 2·4 mg once a week in adults with overweight or obesity, and type 2 diabetes (STEP 2): a randomised, double-blind, double-dummy, placebo-controlled, phase 3 trial. The Lancet (2021) 397(10278):971–84. doi:10.1016/s0140-6736(21)00213-0
63. Wadden, TA, Bailey, TS, Billings, LK, Davies, M, Frias, JP, Koroleva, A, et al. Effect of subcutaneous semaglutide vs placebo as an adjunct to intensive behavioral therapy on body weight in adults with overweight or obesity: the STEP 3 randomized clinical trial. JAMA (2021) 325(14):1403–13. doi:10.1001/jama.2021.1831
64. Rubino, D, Abrahamsson, N, Davies, M, Hesse, D, Greenway, FL, Jensen, C, et al. Effect of continued weekly subcutaneous semaglutide vs placebo on weight loss maintenance in adults with overweight or obesity: the STEP 4 randomized clinical trial. JAMA (2021) 325(14):1414–25. doi:10.1001/jama.2021.3224
65. Frias, JP, Davies, MJ, Rosenstock, J, Pérez Manghi, FC, Fernández Landó, L, Bergman, BK, et al. Tirzepatide versus semaglutide once weekly in patients with type 2 diabetes. N Engl J Med (2021) 385(6):503–15. doi:10.1056/nejmoa2107519
66. Rosenstock, J, Frias, J, Jastreboff, AM, Du, Y, Lou, J, Gurbuz, S, et al. Retatrutide, a GIP, GLP-1 and glucagon receptor agonist, for people with type 2 diabetes: a randomised, double-blind, placebo and active-controlled, parallel-group, phase 2 trial conducted in the USA. The Lancet (2023) 402(10401):529–544. doi:10.1016/s0140-6736(23)01053-x
67. Meerasa, A, and Dash, S. Weighing in on type 2 diabetes remission. Diabetes Care (2022) 45(1):28–30. doi:10.2337/dci21-0041
68. Frias, JP, Deenadayalan, S, Erichsen, L, Knop, FK, Lingvay, I, Macura, S, et al. Efficacy and safety of co-administered once-weekly cagrilintide 2·4 mg with once-weekly semaglutide 2·4 mg in type 2 diabetes: a multicentre, randomised, double-blind, active-controlled, phase 2 trial. The Lancet (2023) 402(10403):720–730. doi:10.1016/s0140-6736(23)01163-7
69. Courcoulas, AP, Gallagher, JW, Neiberg, RH, Eagleton, EB, DeLany, JP, Lang, W, et al. Bariatric surgery vs lifestyle intervention for diabetes treatment: 5-year outcomes from a randomized trial. J Clin Endocrinol Metab (2020) 105(3):866–76. doi:10.1210/clinem/dgaa006
70. Drucker, DJ, and Holst, JJ. The expanding incretin universe: from basic biology to clinical translation. Diabetologia (2023) 66(10):1765–79. doi:10.1007/s00125-023-05906-7
71. Furusawa, S, Nomoto, H, Oba-Yamamoto, C, Takeuchi, J, Ito, M, Kurihara, H, et al. Real-world clinical evidence of oral semaglutide on metabolic abnormalities in subjects with type 2 diabetes: a multicenter retrospective observational study (the Sapporo-Oral SEMA study). Endocr J (2024). doi:10.1507/endocrj.ej23-0648
72. Wharton, S, Blevins, T, Connery, L, Rosenstock, J, Raha, S, Liu, R, et al. Daily oral GLP-1 receptor agonist orforglipron for adults with obesity. N Engl J Med (2023) 389(10):877–888. doi:10.1056/nejmoa2302392
73. Haseeb, M, Chhatwal, J, Xiao, J, Jirapinyo, P, and Thompson, CC. Semaglutide vs endoscopic sleeve gastroplasty for weight loss. JAMA Netw Open (2024) 7(4):e246221. doi:10.1001/jamanetworkopen.2024.6221
74. Wilding, JPH, Batterham, RL, Davies, M, Van Gaal, LF, Kandler, K, Konakli, K, et al. Weight regain and cardiometabolic effects after withdrawal of semaglutide: the STEP 1 trial extension. Diabetes Obes Metab (2022) 24(8):1553–64. doi:10.1111/dom.14725
Keywords: type 2 diabetes mellitus, gut hormones, glucagon like peptide 1, metabolic surgery, obesity
Citation: Morissette A and Mulvihill EE (2024) Obesity management for the treatment of type 2 diabetes: emerging evidence and therapeutic approaches. J. Pharm. Pharm. Sci 27:13065. doi: 10.3389/jpps.2024.13065
Received: 30 March 2024; Accepted: 22 May 2024;
Published: 06 June 2024.
Edited by:
John Reyes Ussher, University of Alberta, CanadaCopyright © 2024 Morissette and Mulvihill. This is an open-access article distributed under the terms of the Creative Commons Attribution License (CC BY). The use, distribution or reproduction in other forums is permitted, provided the original author(s) and the copyright owner(s) are credited and that the original publication in this journal is cited, in accordance with accepted academic practice. No use, distribution or reproduction is permitted which does not comply with these terms.
*Correspondence: Erin E. Mulvihill, ZW11bHZpaGlAdW90dGF3YS5jYQ==