- 1Grupo de Edafología Ambiental, Department of Agrochemistry and Environment, University Miguel Hernández, Elche, Spain
- 2Center of Applied Ecology and Sustainability (CAPES), Pontificia Universidad Católica de Chile, Santiago, Chile
- 3Facultad de Agronomía e Ingeniería Forestal, Pontificia Universidad Católica de Chile, Santiago, Chile
- 4Universidad de la Ciénega del Estado de Michoacán de Ocampo, Sahuayo, Mexico
- 5Chair of Soil Science, TUM School of Life Sciences Weihenstephan, Technical University of Munich, Freising, Germany
- 6Departamento de Sistemas y Recursos Naturales, Universidad Politécnica de Madrid, Madrid, Spain
Wildfires are a common phenomenon globally, nonetheless some ecosystems are more adapted than others. In this work, we show results of a study conducted in Torres del Paine National Park (Chile) that suffered a big forest fire in 2011, affecting 17,666 ha. The Park is located in the transitional forest-steppe zone whose annual rainfall varies between 300 and 1,500 mm. Plant communities goes from Patagonian steppe, pre-Andean scrub to Magellanic forest. Based on vegetation community and trying to cover all representative sectors affected by the fire, 5 areas of the park were sampled in 2019 following the transects where a vegetation recovery study has been monitored since the fire occurred. Soil parameters used to evaluate were: texture, soil water repellency (WR), organic matter (OM), and aggregation, including total content of macroaggregates (TCA) and their stability (AS). The results showed high values of OM, however three of the 5 areas showed statistically lower values of OM in burned samples. WR was present (from slight to severe) in the 75% of the samples, and without differences between burned and unburned. The correlations analyses indicated that WR is more related with the OM type than with quantity, since better correlations were obtained when only samples from the same area were included in the analyses. The results of aggregation indicated that soils have a poor structural development. The TCA varies from 16 to 50%, and the AS is not very high (average of 66%), being the highest in the area with lowest TCA and more affected by the fire and erosion processes. This suggests that the higher values of AS are a consequence of the destruction and loss of the less resistant fraction after the fire. The combination of the high sand content (low specific surface area) and high OM make them very susceptible to develop WR. Since these soils have a scarce development with a poor structure, the combination of the WR and the poor soil structure make them very vulnerable to erosion processes after the fire. Measures to protect the soils or accelerate the recovery are recommended in these areas when new human caused wildfires could occur.
Introduction
Wildfires are a common and natural phenomenon globally and have played a vital role in the history and evolution of ecosystems (Pausas and Keeley, 2009). However, some ecosystems are more adapted to this perturbation than others due to the fire regime relationship with climate conditions. Chile is a country with large forest areas, where anthropogenic forest fires are widespread; they not only occur in the Mediterranean climate region in central Chile but more recently also in more humid and cold climatic zones like the southern Patagonian Region (Salas et al., 2016; Úbeda and Sarricolea, 2016; Mcwethy et al., 2018). It is known that there were fires during the Pleistocene-Holocene in Patagonia-Tierra de Fuego (Moreno et al., 2018). Huber et al. (2004) suggest that the causes of those wildfires were both natural and anthropogenic. There is evidence of human occupation in Tierra del Fuego-Patagonia dated to ca 11 14C ka BP (Borrero and McEwan, 1997). The most likely source of these early fires was the indigenous population (Holz and Veblen, 2011). These authors documented an increase in the number of fires after the colonization and permanent settlement of the region by Euro Chileans. However, there are evidences that the climate conditions of the area would have inhibited the spread and dimension of most of those fires (Mundo et al., 2017). Fire does not form part of the ecosystem dynamics in southern Patagonia, meaning a lack of resilience to wildfire, and patches of the Patagonian steppe are exposed and more susceptible to be colonized by invasive species (Vidal, 2012; Kitzberger et al., 2016). The ecotonal zones between eastern semi-arid steppes and Nothofagus spp. forests in western Patagonia results from broad landscape and ecosystem changes, intensified in the last 140 years, with anthropogenic forest fires, logging, exotic pasture establishment, and mining as the leading causes (Zegers et al., 2020).
Forest fires can affect several soil properties depending on fire intensity, severity, and soil type (Certini, 2005). Vegetation and soil recovery are directly affected by heat and/or indirectly as a consequence of the temporal elimination of soil biota, vegetation coverage, and the effects of ash incorporation on soil conditions (Bodí et al., 2014). Among the soil properties affected, some of the most relevant are soil organic matter (OM), water repellency (WR), and soil aggregation (Doerr et al., 2000; Mataix-Solera et al., 2011). It is known that some soils are much more vulnerable to fire and post-fire erosion processes than others due to their nature and properties, and therefore with a very different response to fire perturbations (García-Corona et al., 2004). The OM content can decrease or increase due to fire depending on other factors such as type of fire, its intensity, and severity (Almendros and González-Vila, 2012). Soil OM reduction can be produced in high intensity fires as a consequence of the combustion processes and the remaining OM suffers quality changes in its composition (González-Pérez et al., 2004). Low intensity fires and fires that spread through the crowns tend to increase the OM content by the incorporation of partially burned vegetation residuals (Mataix-Solera et al., 2002). Following fires, runoff and soil erosion can increase, reducing the OM content in the topsoil (Larsen et al., 2009; Bonilla et al., 2014). Soil WR is a property that reduces the affinity of soils to water, affecting the infiltration-runoff balance and therefore the erosion rates (Jordán et al., 2013). It can be naturally caused by some soil organic compounds or induced by the effect of fires (DeBano, 2000; Doerr et al., 2000). Previous studies conducted in Mediterranean soils demonstrated that their susceptibility to developing WR depends not only on soil temperatures produced by fires but also on soil texture, mineralogy of clay fraction and the quantity and quality of the soil organic matter (Mataix-Solera et al., 2014). The measurement of soil structure and aggregate stability described soil vulnerability to erosion processes after wildfires. Previous studies have found different patterns of aggregation in fire-affected soils (Mataix-Solera et al., 2011). The decreases of aggregate stability are related to high fire severity and loss of OM content (Badía and Martí, 2003), and the increases could be explained by changes in WR at certain temperatures (García-Corona et al., 2004; Terefe et al., 2008), or some specific mineral transformations with high fire severity (Giovannini et al., 1988; Jiménez-Pinilla et al., 2016). Consequently, there is no general pattern of soil behaviour to wildfire since many factors are involved.
Wildfires are relatively rare in cold temperate rainforest, but they can strongly change forest landscape (Zegers et al., 2020). In Chile, there are reported evidence about the mid- and short-term effect of fire mostly on vegetation in the Mediterranean region (Úbeda and Sarricolea, 2016). Under new climate change scenarios, the frequency of fires will increase in Patagonia, with anomalous dry conditions (Holz and Veblen, 2012). Understanding the mid-term effects of fire on soil and vegetation is necessary to implement effective restoring practices. Torres del Paine National Park, a world biosphere reserve, is one of the most emblematic parks in the Chilean Patagonia. It is located in the southwestern part of the region and preserves a sensitive ecosystem in a landscape dominated by evergreen shrubs, Nothofagus forests and grasses that resist harsh wind and weather. Between 1985 and 2011, 29 fires caused by humans were recorded in the Park, affecting more than 36,000 ha, three of them were the most significant events produced in recent years (1985, 2005, and 2011). Following the last fire, Bonilla et al. (2014) reported no difference in soil organic content when comparing burned with unburned sites. However, the burned sites showed a high soil erosion potential. In this work, we analysed the results of soil properties from samples collected in 2019 in the Torres del Paine National Park (Chilean Patagonia) from different areas that suffered a large forest fire during the end of 2011 (Medina, 2013).
The objectives of this research are: 1) to evaluate the medium-term impacts of forest fire in some soil properties in a cold temperate rainy climate, like Torres del Paine, and 2) to compare the vulnerability of these soils to this perturbation with other environments also affected by wildfires but much more studied and resilient such as the Mediterranean.
Materials and Methods
Study Area, Fieldwork and Soil Sampling
The Torres del Paine National Park has a total surface close to 181,000 ha and it is in a transitional forest-steppe zone in the Region of Magallanes and Chilean Antarctic (50°58′59″S, 72°57′59″W) (Figure 1). The climate is a temperate cold rainy zone without dry season, the temperatures range below 15°C, being this the maximum temperature for the warmest month (January), and a fairly rigorous winter with an average minimum temperature for the coldest month (July) never lower than −2.5°C. Precipitation from westerly winds shows a marked gradient from west to east, and annual rainfall varies between 300 mm in the east part of the park to 1,500 mm in the west side (Pisano, 1974). Within the park, it is possible to find four plant communities: Patagonian steppe, pre-Andean scrub (Xerophilous and Mesophilic), deciduous Magellanic Forest, and Andean desert. The Park was completely covered by ice during the last glacial maximum and therefore the soils are young. According to Marden and Clapperton (1995) the steppe area of Torres del Paine was not colonized by peat until after by ca 12 ka BP. The soils of the study areas, mostly developed over recent glaciofluvial sediments and with scarce development, are typical of cold regions. According to World Reference Base for Soil Resources system of classification the dominant soil types in the study areas are Phaeozems, Regosols and Leptosols (IUSS Working Group WRB, 2015).
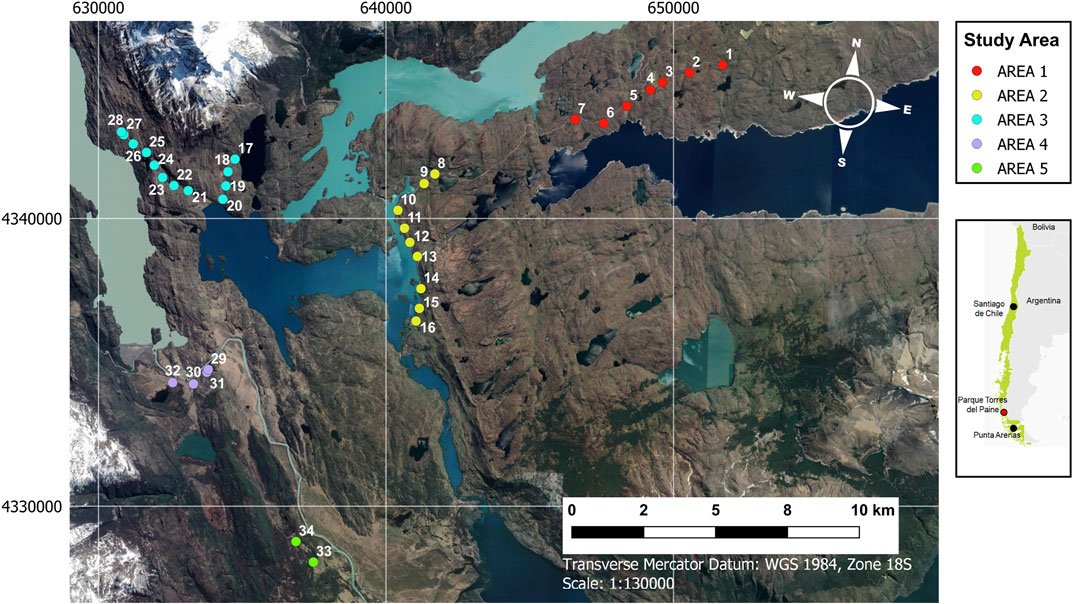
FIGURE 1. Geographical location of Torres del Paine National Park in Chile and Google earth image of the study areas and points of soil samplings.
In December 2011, a big forest fire occurred in the National Park, affecting 17,666 ha. Based on geographical location and vegetation coverage, in 2019, 5 areas of the Park were sampled following the transects where a vegetation recovery study had been previously monitored, to understand the status of the ecosystem and how fire and post-fire conditions affected the area (Figure 1) (Armesto et al., 2012). The record was concentrated on sites affected by the 2011 fire considering: Cover of native and exotic herbaceous plants and regeneration of seedlings, and regrowth of shrubs and trees. Landsat satellite images (FORMOSAT-2) from 2011 and maps made by the National Park administration from areas previously affected by wildfires were used to select the vegetation and soil monitoring sites. These areas were chosen to cover all the range of plant communities, considering the accessibility and the presence of unburned control areas on each of the selected locations. The main difference between the study areas was the vegetation, being a transition between Patagonian steppe and xerophilous pre-Andean scrub in study area 1, the driest sector, area being more dominated by scrub (xerophilous and mesophilic), study areas three and four were predominantly of mesophilic scrub and forest, corresponding with the western sector of the Park, as indicated more humid, and area 5 low-density of Magellanic forest (Figure 2). The main species present in each of the study areas are included in Table 1. After the fire in 2011, a field assessment of soil fire severity was done using the field guide for mapping post-fire developed by the USDA-Forest Service. The burned sites were classified into three levels; low severity, moderate severity and, high severity of above-ground vegetation and below-ground (Robichaud et al., 2000; Pearsons et al., 2010). The burned soil severity evaluation also considered three categories (Pearsons et al., 2010). 1) Low soil burn severity: Surface organic layers are not entirely consumed and are still recognizable. Structural aggregate stability is not changed from its unburned condition. Roots are generally unchanged because the heat pulse below the soil surface was not significant enough to consume or char any underlying organics, 2) Moderate soil burn severity: Up to 80% of the pre-fire ground cover (litter and ground fuels) may be consumed but generally not all of it. Fine roots (∼0.1 inches or 0.25 cm diameter) may be scorched but rarely consumed over most of the areas, and 3) High soil burn severity: All or nearly all of the pre-fire ground cover and surface organic matter (litter, duff, and fine roots) is generally consumed, and charring may be visible on more prominent roots. According to this and with the results of the assessments in all the sampling sites, for the five study areas, fire severity was classified as follows: low severity for area 1, low to moderate in area 2, moderate to high in area 3, and moderate severity in areas 4 and 5. Each study area includes a control (unburned) site as a reference (numbers 1, 13, 28, 32, and 34 of Figure 1). The others were affected by the fire of 2011, and only three of the points from area 2 (numbers 14, 15, and 16) were also affected by a previous fire in 1985. There is no overlapping with the fire of 2005 that affected an area of the park located in the Park’s northern section.
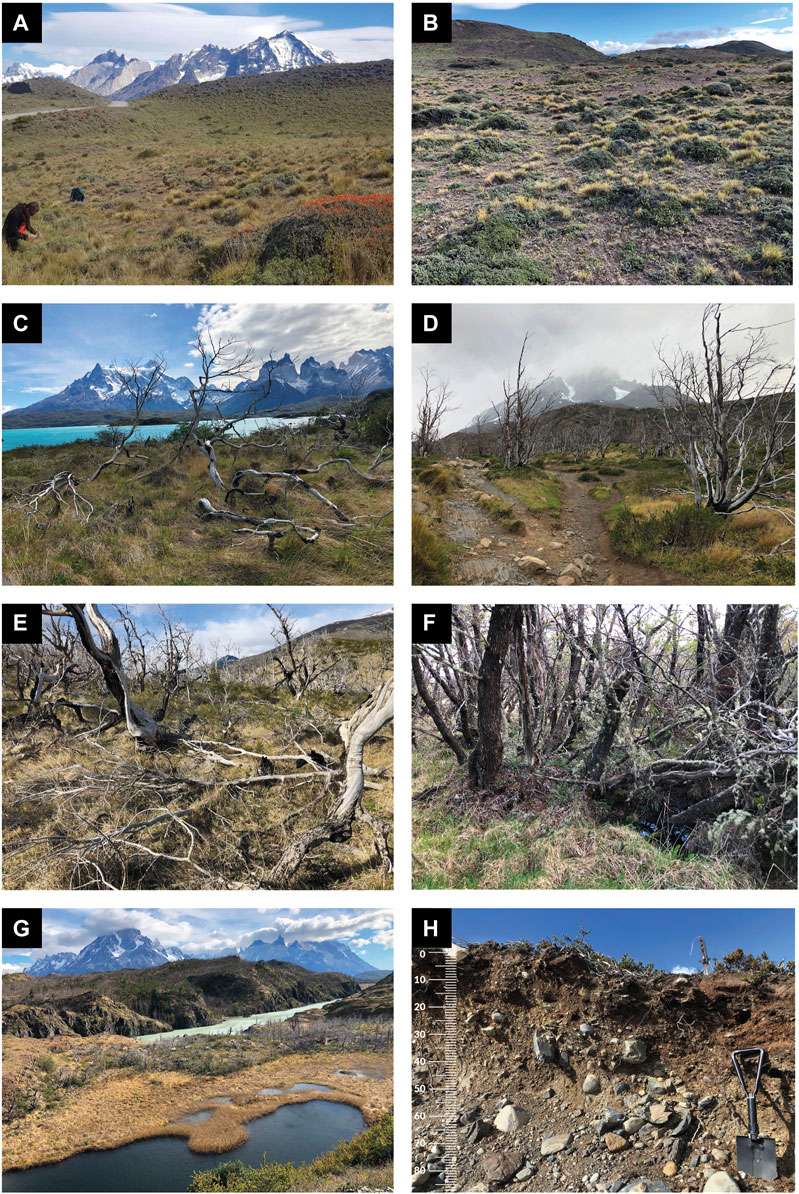
FIGURE 2. Some representative images of the study areas: (A,B) area 1 burned; (C) area 2 burned; (D) area 3 burned; (E) area 4 burned; (F) area 5 unburned; (G) Landscape between areas 4 and 5; (H) soil profile of area 2 burned. Regosol over glaciofluvial sediments. Numbers in soil profile line are in cm.
Vegetation cover was estimated in a 2 m radius (12.56 m2) circular plots randomly located in each point (n = 4 plots/sampling point × 34 soil sampling points = 136). We estimated the percentages of soil occupied by litter, standing trees, tree stumps, and/or burned shrubs, trunks, herbaceous cover and bare soil in each one of the plots. All individuals with phanerophyte habits were classified into five categories: burned with no leaves present, burned with burned leaves present, burned with green leaves, burned with sprouts, and not burned. This manuscript use the percentage of soil cover (or the percentage of bare soil) in 2019 to compare with the initial data collected in 2012, just after the fire occurred.
At each of the 34 sampling points (Figure 1), composite soil samples from the first 5 cm depth of mineral soil were taken in November 2019. Two or three composite samples per sampling point, by pooling subsamples from different sites, were collected for laboratory analyses, resulting in a total of 74 soil samples, including burned and unburned soils in the 2011 fire.
Analytical Methods
In the laboratory, the following soil properties were analysed: pH, electrical conductivity (EC), soil texture, soil water repellency (WR), organic matter content (OM), and aggregation, including total content of macroaggregates (TCA; % of sample that are forming macroaggregates) and their stability (AS; % of macroaggregates that resist the energy of a rainfall simulation of known energy). Soil pH was measured in deionised water (1:2.5 w/v). EC was also measured in deionised water (1:5 w/v). Texture determined by the Bouyoucos method (Gee and Bauder, 1986). OM content was determined by potassium dichromate oxidation (Nelson and Sommers, 1982). The water drop penetration time (WDPT) test (Wessel, 1988) was used to measure the persistence of WR. Prior to WR assessment, approximately 15 g of each soil sample was placed on separate 50-mm diameter plastic dishes and exposed to a controlled laboratory atmosphere (20°C, ∼50% relative humidity) for 1 week in order to eliminate potential effects of any variations in preceding atmospheric humidity on soil WR and in accordance with Doerr et al. (2005). This involved placing three drops of distilled water (∼0.05 ml) on the sample surface and recording the time required for their complete penetration. The average time for triplicate drops is taken as the WDPT value of a sample, and then classified according to Bisdom et al. (1993) and Doerr et al. (1998), as follows: wettable (<5 s), slightly water repellent (5–60 s), strongly water repellent (60–600 s), severely water repellent (600–3,600 s), and extremely water repellent (>3,600 s). Soil aggregate stability (AS) and total content of macroaggregates (TCA) were studied in the macroaggregate fraction of 4–0.25 mm and determined with the rainfall simulator method according to Roldán et al. (1994) and based on the method of Benito and Díaz-Fierros (1989). This method examines the proportion of macroaggregates that remained intact after a soil sample was subjected to artificial rainfall of a specific energy. Four grams of soil material (4–0.25 mm) were placed into a 0.25-mm sieve and exposed to an energy of 270 J m−2 applied by an artificial rainfall. After treatment, the material remaining within the sieve was dried and weighed. The material on the sieve, consisting of remaining macroaggregates, mineral particles and organic debris, was then washed until only mineral particles and organic debris remained. The dry weight of the remaining mineral particles and organic debris material allowed the calculation of the difference in aggregate weight (%) within a sample before and after artificial rainfall (AS). The method also allows one to calculate the proportions of sample that forms macroaggregates by differences of weights (TCA). Results are based on the mean value of three replicate experiments per soil sample.
Statistical Procedures
The fitting of the data to a normal distribution was checked with the Kolmogorov–Smirnov test. When necessary, analytical data was transformed using logarithms to assure normal distribution. The t-test for independent samples was used to compare the means of parameters between the burned and control soil groups per study area. Pearson correlation coefficients (r) were calculated to quantify the linear relationship between parameters. A Principal Component Analysis (PCA) was performed with results of all soil samples in order to study the relationship between the soil parameters measured regarding the study areas using the “FactoMineR” package implemented in R (Le et al., 2008). The vegetation cover percentage comparison (2012 vs 2019) per study area was evaluated with non-parametric Kruskal-Wallis test. All statistical analyses were performed using the RStudio v.3.6.2 (RStudio Team, 2020).
Results
The results of soils characterization (Table 2) showed that two of the areas have a loam soil texture and sandy loam in the other three, pH ranging from 5.4 to 6.7, and low values of EC. Area 1 was observed as the most vulnerable to erosion processes being the area where higher percentage of bare soil (43% in average) remained 8 years after the fire (Figure 3A). It is also the only area where under unburned conditions soil bare (18%) was found. The other study areas were recovered by vegetation and after 8 years of the fire, the percentage of bare soil is low, ranging from 4 to 14% (Figure 3A), however there is still significant differences also in areas 3 and 4. Table 3 show the comparison of vegetation cover in burned soils between 2012 and 2019 per study area. In all study areas except area 1 it was observed statistical differences with a significant increase in vegetation cover. The area 1 however, despite the low fire severity registered and starting in 2012 with a soil coverage close to 50%, the values did not reach 60% in 2019 without significant differences between years.
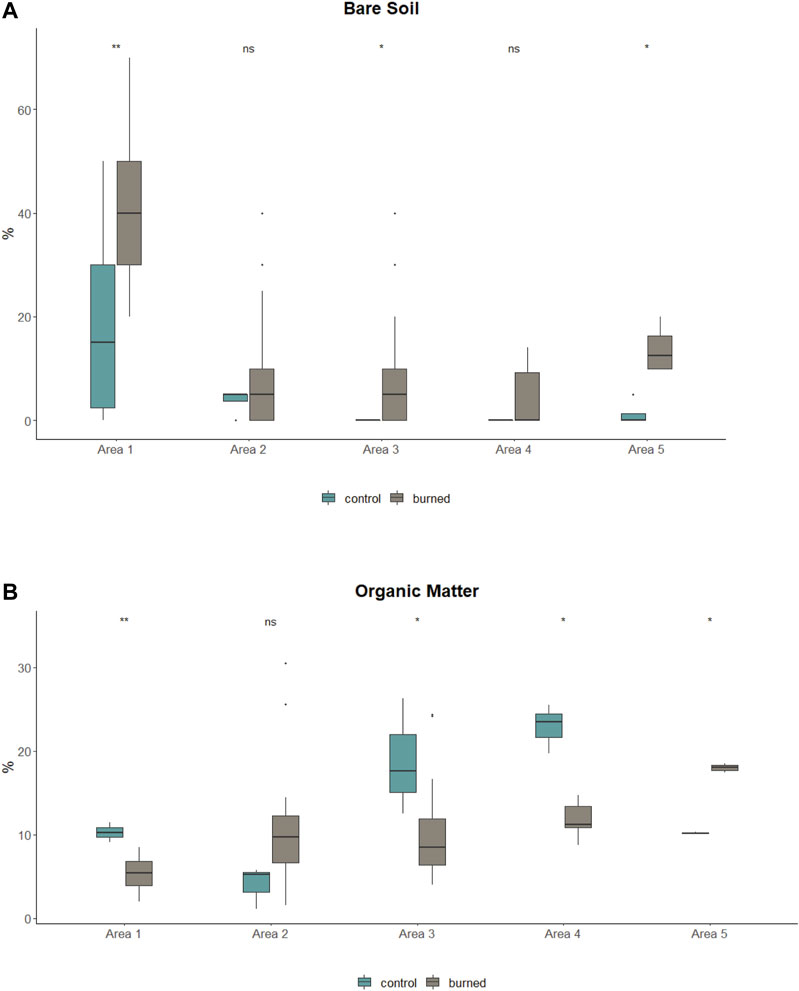
FIGURE 3. Box-plots of (A) bare soil (%) and (B) organic matter content (%) per study area comparing burned soils vs controls. **, *: significant level for p < 0.01 and p < 0.05, respectively; ns, not significant at p > 0.05.
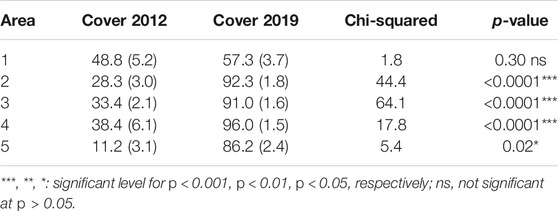
TABLE 3. Mean and standard error of vegetation cover (%) 2012–2019 for burned soils per study area, and Kruskal Wallis test.
The OM content of the soil samples is in general high, with an average of 10.5% and with some soil areas reaching more than 20% (Figure 3B). Three of the five areas showed statistically lower values of OM in burned samples (areas 1, 3 and 4), and in one of them, area 5, levels were higher after burning. No differences were found for area 2. Soil WR was present in the 75% of the samples with WDPT classes ranging from slight to severe water repellent, and without differences between burned (222 ± 392 s) and unburned samples (298 ± 318 s; p > 0.05). All study areas showed WR (79, 67, 76, 75, and 100% of water repellent samples for areas 1 to 5, respectively; Figure 4). The correlations analyses (Table 4) indicated that WR is positively correlated with OM content (Figure 5A), but this correlation is higher when only samples from areas where the vegetation structure and composition is more homogeneous are included. As an example, it is showed the correlation between both parameters in area 1 (r = 0.852; p < 0.01, Figure 5B). The results of aggregation indicated that these soils have a poor structural development. The TCA varies from 16 to 50% (Figure 6A), and the AS measured were not very high (average of 67%; Figure 6B), being the highest in the area 1 which is the area with lowest TCA. In the area 1 the TCA was statistically lower for burned soils and AS higher. The OM content was positively correlated with TCA but not with AS and a negative correlation was found between TCA and AS. Not correlations were found between AS and OM and between AS and WR (Table 4). The PCA performed explained a total of 76.8% of variability (Figure 7) showing that some areas like 5, and specially 1, have more homogeneous data and they clearly differentiate from the other areas. Area 1 is clearly separated for the soil aggregation parameters studied (TCA and AS). Area 2 seems to collect the most variability of all study areas. Area 5 is related with higher values of WR and AS, while areas 3 and 4 are related with high values of TCA and OM.
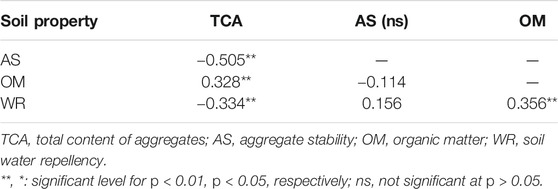
TABLE 4. Pearson correlation coefficients (r values) for relationships between the studied properties for all soil samples (n = 74).
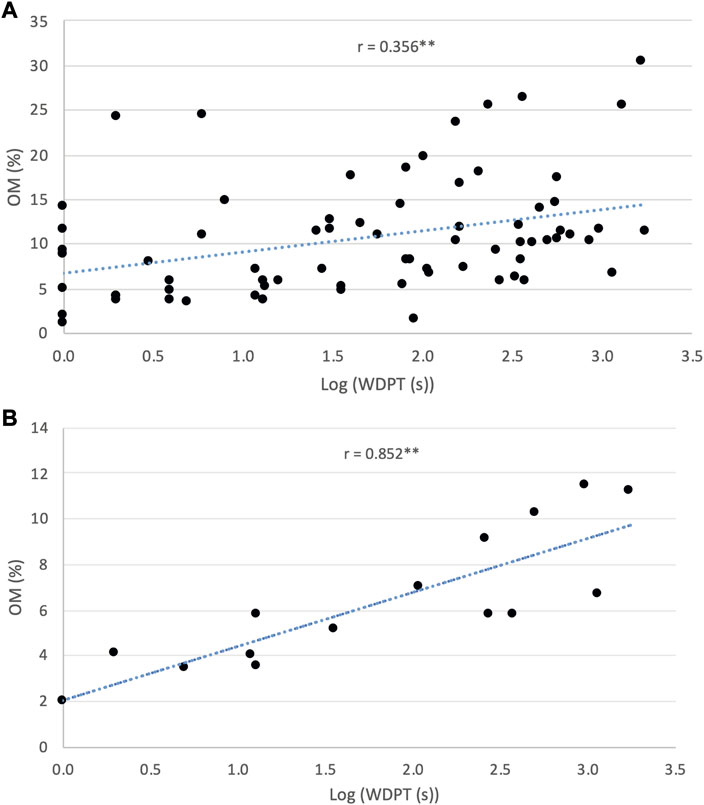
FIGURE 5. Relationship between organic matter content and water repellency [Log(WDPT)]. (A) By pooling all samples from different study areas and (B) Only samples from area 1.
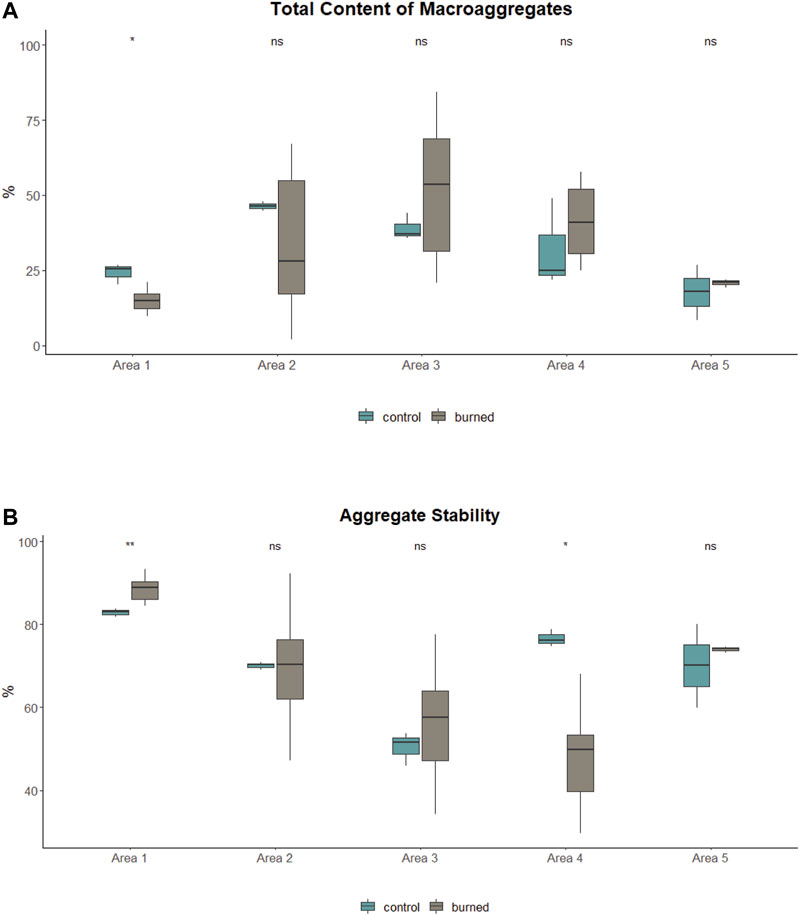
FIGURE 6. Box-plots of (A) total content of macroaggregates (TCA %) and (B) aggregate stability (AS, %) per study area comparing burned soils vs controls. **, *: significant level for p < 0.01 and p < 0.05, respectively; ns, not significant at p > 0.05.
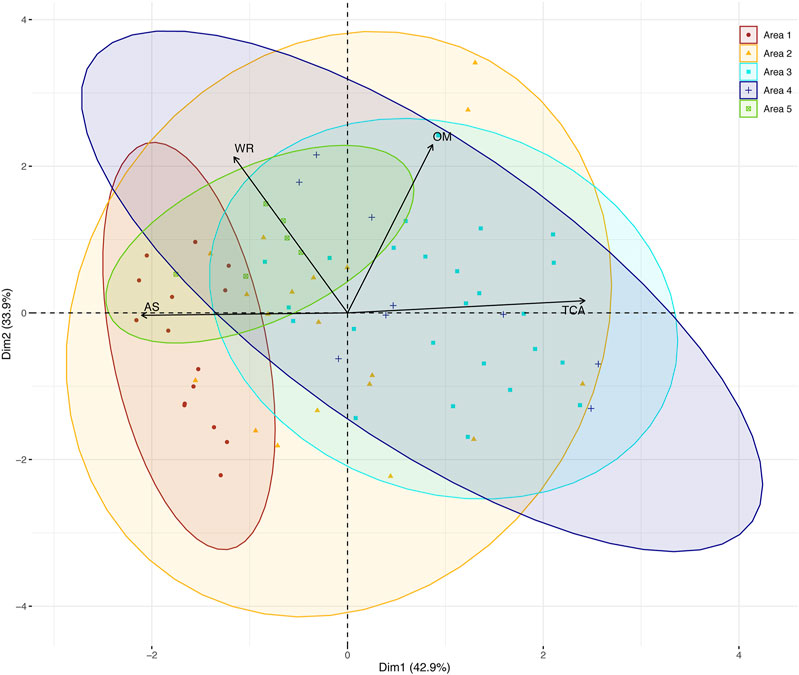
FIGURE 7. Scores and loadings for PCA performed with results of all soil samples per study area. AS, aggregate stability; OM, organic matter content; TCA, total content of macroaggregates; WR, water repellency.
Regarding the effect of fire recurrency on soil properties, for this study, it is only limited to a section of area 2. The compartive of the results for those sampling points (burned in 1985 and 2011), with those of the same area only affected by the fire in 2011 showed no statistical differences for the parameters selected for this study.
Discussion
Our soil sampling results in various vegetation areas burned and not burned in 2011 show that the effect of fires on soil properties depends on local site conditions and vegetation recovery.
Soil Organic Matter
The OM content of the studied soils is high but revealed decreases in three of the five study areas as consequence of the fire and/or post-fire erosion processes. Fire can affect directly to the OM content depending on fire intensity and severity, in fact previous studies have observed both increases or decreases depending on the fire behaviour (Mataix-Solera et al., 2002). The area 1 showed statistically lower OM content in burned soils despite this is the area where the fire had low severity, therefore this decrease should be more probable due as consequence of the post-fire erosion processes. In the cases of areas 3 and 4 where fire severity was higher, the decrease observed could be caused for both, higher combustion and post-fire erosion. Bonilla et al. (2014) studied burned areas in Torres del Paine, some of them affected in the same fire of this research but just 1 year after the fire. They reported no difference on soil organic content comparing burned with control (unburned) sites, one reason could be because the depth of the soil samples collected (0–20 and 20–40 cm). The fire effects on soils tend to affect only the first mm or cm of mineral soil and these effects are masked if soil samples that are taken with more depth (Neary et al., 2005). In this case the samples are from 0 to 5 cm, thus more sensitive to reflect the changes. In any case, taking into account the fire severity reached, it is considered that the detected losses of OM in the areas 1, 3, and 4, eight years after the fire are more probably due to post-fire erosion processes than from a direct effect of combustion during the fire. This is also supported from the results of the erosion rates estimated in the same study area by Bonilla et al. (2014) using data from fire affected sites in 2005 with the Water Erosion Prediction Project (WEPP Model). Their results showed that water erosion increased after the fire, mainly due to the effect of vegetation removal but also due to changes in soil properties and erodibility. These authors reported that the sediment yield after fire increased from 0.02 to 9.25 t ha−1 year−1 and produced an estimated increase in soil organic carbon losses from 0 to 0.54 t ha−1 year−1. Other studies have reported reduction in OM content by post-fire erosion when vulnerable soil conditions and low vegetation cover persist in time (García-Orenes et al., 2017). Area 1 was observed as the most vulnerable to erosion processes being the area where higher percentage of bare soil (43% in average) remained 8 years after the fire (Figures 2A,B, 3A). It is also the only area where under unburned conditions some of soil bare (18%) is found. This also supports the affirmation that especially in the area 1 the OM loss has been produced by topsoil erosion. The other study areas were recovered by vegetation and 8 years after fire the percentage of bare soil observed were low (Figure 2A). On the other hand, the increased OM content in area 5 and the results of area 2 without statistical differences are indicative of how variable the effect of fire can be in the organic fraction of the soil.
Soil Water Repellency
A high percentage of soil samples (75%) showed WR, without differences between burned and unburned soils. This reveals that WR is a natural property in these soils. Many studies have found natural soil WR under different vegetation types, mainly associated with evergreen tree types and particularly, trees with considerable amounts of resins, waxes or aromatic oils. However, soil WR has also been detected under deciduous trees, shrubs and grass species (Doerr et al., 2000). The combination of the high proportion of sand, high OM content and acid pH make the soils of Torres del Paine very susceptible to develop WR (Doerr et al., 2000), although the variability of WR persistence observed is elevated (Figure 4). Mataix-Solera et al. (2014), found that small variations in soil properties such as texture, mineralogy of clay fraction, and organic matter quantity and quality can control the development and persistence of WR, being the sand content, one of the most relevant factors. These soils have a high sand content with textures that varies from loam to sandy loam. Sandy soils are more prone to develop WR because of the lower specific surface area to be covered by hydrophobic substances (González-Peñaloza et al., 2013). The pH of the soils of this study varies from 5.7 to 6.7 (Table 2), and this can also contribute to the presence of WR. Many studies showed that acidic soils are more prone to develop WR than alkaline (e.g., Dekker and Jungerius, 1990; Doerr et al., 1998; Varela et al., 2005). There are different reasons that can contribute to this; alkaline conditions enhancing the solubility of the humic acid fraction (implied in WR development), the dependency of the pH of water contact angles of fulvic and humic acids and their negative relationship with pH (Lin et al., 2006), and the enhanced activity of fungi releasing hydrophobic compounds in soils with lower pH (Jex et al., 1985; Paul and Clark, 1996; Weyman-Kaczmarkowa and Pędziwilk, 2000).
Regarding the relationship between WR and OM, numerous researchers have also attempted to establish general relationships, but with some inconsistent results. Some authors found positive correlations (e.g., Wallis et al., 1990; McKissock et al., 1998). However, Teramura (1980) found a negative relationship and DeBano (1991) and Wallis et al. (1993) no relation between the two variables. This inconsistency has been explained because only some compounds of soil organic matter induce WR, and therefore not always is correlated with the amount, particularly when soils with different organic matter quality are compared. In this study case the correlations analyses (Table 4) support also the idea that WR is more related with the type of OM than with the quantity, since better correlations were obtained when only samples from areas with similar vegetation were included in the analyses, and a worse correlation was obtained for areas with more heterogeneity in forest (herbs, scrub, trees) or when all the samples from different sites were pooled together. An example is showed in Figure 5A, with all samples from the different areas pooled together, (r = 0.356; p < 0.01), and how the correlation improves when only samples from area 1 (steppe vegetation, Figure 5B) are included (r = 0.852; p < 0.01).
Fire effects on WR can be very variable since they can induce it in wettable soils, enhancing or destroying in original water repellent ones, depending on many factors, being the temperatures reached in the soil one of the most determinant (DeBano, 2000), but in this case, 8 years after the fire, no significant differences were found in WR between burned and unburned soils. The natural presence of WR in these soils and the time passed since the fire could be masking any fire effect on soil WR.
Different studies have suggested that the natural presence of soil WR caused by plants is thought to be a competitive strategy of species, improving water conservation by channelling water to greater depth (e.g., Scott, 1992; Moore and Blackwell, 1998), and reducing evaporation of water in the surface layer (Doerr et al., 2000). This property, however, becomes a problem during the temporal window from the fire event (bare soil) to the point when the recovery of vegetation occurs, since erosion processes are accelerated due to increased runoff rates (Shakesby and Doerr, 2006).
Soil Aggregation
These soils showed a low structural development. The proportion of soil that are forming macroaggregates (TCA) varies from very low (16% of average in area 1) to medium (50% in area 3), and the stability of macroaggregates (AS) showed results with high variability among study areas. There are different methods to measure AS and between them the data are not always comparable, since they are relative to the energy applied and the way to do it (drop impacts, water immersion, ultrasounds, etc.). In this case a laboratory rainfall simulator method was used, and to compare the results previous studies that adhere to this methodology are used. Chrenková et al. (2014) using this methodology, studied aggregation in different types of Mediterranean soils (including different range of soil textures) and with different land use (forest and agricultural). They found that the TCA in Mediterranean forest soils varied from 55 to 95%, much higher that the results obtained here for soils of Torres del Paine. Regarding AS, results from different studies in Mediterranean forest soils showed a range from 45 to 95% (Zornoza et al., 2007; Chrenková et al., 2014), or from 46 to 76% in A horizons of reforested soils affected bt gully erosion in Slovakia (Dlapa et al., 2012).
Fire can affect in a different way to the soil parameters related to aggregation (Mataix-Solera et al., 2011). Many authors have found that high severity fires can destroy directly macroaggregates or reduce their stability making them less resistant to erosion (Zavala et al., 2010; Jiménez-Pinilla et al., 2016). However, others didn’t found differences (Llovet et al., 2008; Zavala et al., 2010) and even in some cases AS increases have been reported (Josa et al., 1994; García-Corona et al., 2004; Mataix-Solera and Doerr, 2004). The different patterns of aggregation after the fire have been explained depending on the type of soil, the temperatures reached, and how this can affect to other soil properties related to aggregation such as fungi content, organic matter, water repellency or mineralogy (Mataix-Solera et al., 2011). In this study, 8 years after the fire, differences were found for both aggregation parameters only in area 1, with a decrease in TCA and an increase in AS, being the higher values precisely in this area with lower TCA. Taking into account the results of both parameters and the reduced observed in OM content in burned soils of this area, this suggests that the higher values of AS found are consequence of the destruction and loss of the less resistant fraction after the fire and erosion processes. This is also supported with the negative correlation obtained between TCA and AS (Table 4), the PCA (Figure 7), and with the results of percentage of bare soil (Figure 3A), higher also in area 1, thus more soil directly exposed to rainfall and wind events.
Despite many previous works has found positive correlations between AS and OM (Soto et al., 1991; Roldán et al., 1994; Badía and Martí, 1999; Dlapa et al., 2012), and between AS and WR (Chenu et al., 2000; Benito et al., 2003; García-Corona et al., 2004), this is not the case of this study (Table 4), and OM was only positively correlated with TCA and with WR. These relationships used to be strong in undisturbed and well aggregated soils (Martí et al., 2001), but becoming inconsistent and more complex in burned and degraded soils because all the factors involved, showing contrasted responses in some cases (Mataix-Solera et al., 2011). The lower proportion of soil forming macroaggregates, and the erosion processes, breaking some of them and increasing the relative proportion of the stronger ones, could be explaining the lack of correlation between OM and AS. For this reason, the evaluation of the aggregation only with AS data can lead us to erroneous interpretations. It is necessary to include measurements such as the TCA. This becomes of particular interest and necessary when studying soils with low degree of structure development and subjected to disturbances such as fires and subsequent erosive processes.
General Implications
The role that vegetation is playing in these soils, as a protector against erosive processes and through the dense network of roots, is fundamental given the low percentage of soil that is forming macroaggregates. There are many areas of the Park where vegetation was able to regrowth, however changes in soil properties might affect the regrowth of key species that depend on seed production. One of the main concerns in the Park is that some tree species like Nothofagus pumilio and Nothofagus betuloides do not regenerate naturally and could be replaced by shrubs that resist fire and resprout vigorously (Mermoz et al., 2005). In addition to that, this study indicates that the soil should also be an object of concern given the vulnerability of degradation shown by some indicators. Evaluations with analysis of NDVI series for the 2011 burned areas showed that for the year 2014, the herbaceous cover of Patagonian steeps had an important recovery of coverage, not so the pre-Andean scrubs areas (Peña and Ulloa, 2017). For these reasons, measures to protect the soils such as mulching or accelerating the recovery are recommended in these areas when new wildfires caused by humans occur (Díaz Vidal et al., 1959; Keeley, 2009; CONAF, 2015; Díaz-Raviña et al., 2018; Girona-García et al., 2021). This will be of particular interest in the areas of the national park where annual precipitation is lower and both the soil and the ash from the fire are highly exposed to erosive processes, not only water but also wind erosion, since blizzard episodes are frequent in the area.
This work promotes the achievement of Sustainable Development Goal 15, by addressing how sustainably manage forests and reverse land degradation.
Data Availability Statement
The raw data supporting the conclusion of this article will be made available by the authors, without undue reservation.
Author Contributions
JM-S funding acquisition to conduct the study, conceived and designed research, conducted the fieldwork, collected data, supervised analyses and wrote the manuscript. EA and PV also participated in the funding acquisition, design of the research and fieldwork. EA participated in the fieldwork and collected data. LO, JG, VA, MG-C, and NG-F did soil laboratory and statistical analysis. All authors contributed to discussions of the results, revised and approved the final version of the manuscript.
Funding
This work was supported by funding by the POSTFIRE_CARE project of the Spanish Research Agency (AIE) and the European Union through European Funding for Regional Development (FEDER) (Ref.: CGL2016-75178-C2-1-R), UMH grant for international mobility and ANID PIA/BASAL FB0002 (Chile).
Conflict of Interest
The authors declare that the research was conducted in the absence of any commercial or financial relationships that could be construed as a potential conflict of interest.
Acknowledgments
Authors want to thank to CONAF Chile, the administration of Parque Nacional Torres del Paine, Refugio Paine Grande and Hielos Patagónicos Sur for the logistic support. Special thanks to Patricio Salinas, David Vasquez, Ignacio Fernandez, Jaime (Vértice) and Mikel (CONAF). We also want to thank the two reviewers for the constructive comments and suggestions that helped to improve the manuscript.
References
Almendros, G., and González-Vila, F. J. (2012). Wildfires, Soil Carbon Balance and Resilient Organic Matter in Mediterranean Ecosystems. Span. J. Soil Sci. 2, 8–33. doi:10.3232/SJSS.2012.V2.N2.01
Armesto, J., Nuñez, M., and Gallardo, B. (2012). Plan de Restauración Ecológica del Parque Nacional Torres del Paine afectado por incendios 2011-2012. Santiago, Chile: Ministerio del Medio Ambiente.
Badía, D., and Martí, C. (1999). Suelos del Pirineo Central: Fragen. Huesca, Spain: I.N.I.A., U.Z., C.P.N.A., I.E.A., 190.
Badía, D., and Martí, C. (2003). Plant Ash and Heat Intensity Effects on Chemical and Physical Properties of Two Contrasting Soils. Arid Land Res. Manag. 17, 23–41. doi:10.1080/15324980301595
Benito, E., and Díaz-Fierros, F. (1989). Estudio de los principales factores que intervienen en la estabilidad estructural de los suelos de Galicia. Anal Edafol. Agrobiol. 48, 229–253.
Benito, E., Santiago, J. L., de Blas, E., and Varela, M. E. (2003). Deforestation of Water-Repellent Soils in Galicia (NW Spain): Effects on Surface Runoff and Erosion under Simulated Rainfall. Earth Surf. Process. Landf. 28, 145–155. doi:10.1002/esp.431
Bisdom, E. B. A., Dekker, L. W., and Schoute, J. F. T. (1993). Water Repellency of Sieve Fractions From Sandy Soils and Relationships With Organic Material and Soil Structure. Geoderma 56, 105–118. doi:10.1016/0016-7061(93)90103-R
Bodí, M. B., Martin, D. A., Balfour, V. N., Santín, C., Doerr, S. H., Pereira, P., et al. (2014). Wildland Fire Ash: Production, Composition and Eco-Hydro-Geomorphic Effects. Earth Sci. Rev. 130, 103–127. doi:10.1016/j.earscirev.2013.12.007
Bonilla, C. A., Pastén, P. A., Pizarro, G. E., González, V. I., Carkovic, A. B., and Céspedes, R. A. (2014). “Forest Fires and Soil Erosion Effects on Soil Organic Carbon in the Serrano River Basin (Chilean Patagonia),” in Soil Carbon. Progress in Soil Science. Editors A. Hartemink, and K. McSweeney (Cham: Springer), 229–237. doi:10.1007/978-3-319-04084-4_24
Borrero, L. A., and McEwan, C. (1997). “The Peopling of Patagonia, the First Human Occupation,” in Patagonia. Natural History, Prehistory and Ethnography at the Uttermost End of the Earth. Editors C. McEwan, L. A. Borrero, and A. Prieto (London, United Kingdom: British Museum Press), 22–45.
Certini, G. (2005). Effects of Fire on Properties of Forest Soils: A Review. Oecologia 143, 1–10. doi:10.1007/s00442-004-1788-8
Chenu, C., Le Bissonnais, Y., and Arrouays, D. (2000). Organic Matter Influence on Clay Wettability and Soil Aggregate Stability. Soil Sci. Soc. Am J. 64, 1479–1486. doi:10.2136/sssaj2000.6441479x
Chrenková, K., Mataix-Solera, J., Dlapa, P., and Arcenegui, V. (2014). Long-Term Changes in Soil Aggregation Comparing Forest and Agricultural Land Use in Different Mediterranean Soil Types. Geoderma 235–236, 290–299. doi:10.1016/j.geoderma.2014.07.025
CONAF (2015). Incendios forestales en Chile. Available at: http://www.conaf.cl/incendios-forestales/incendios-forestales-en-chile/ (Accessed May 25, 2020).
DeBano, L. F. (1991). “The Effects of Fire on Soil Properties,” in Paper Presented at the Symposium on Management and Productivity of Western-Montane Forest Soils, Boise, ID, April 10–12, 1990 (Ogden, UT: US Department of Agriculture Forest Service), 151–156. General Technical Report INT-280.
DeBano, L. F. (2000). The Role of Fire and Soil Heating on Water Repellency in Wildland Environments: A Review. J. Hydrol. 231–232, 195–206. doi:10.1016/S0022-1694(00)00194-3
Dekker, L. W., and Jungerius, P. D. (1990). “Water Repellency in the Dunes With Special Reference to the Netherlands,” in Dunes of the European Coasts: Geomorphology - Hydrology - Soils (Netherland: Catena, Supplement), 173–183.
Díaz Vidal, C., Avilés Sommers, C., and Roberts, R. C. (1959). Los grandes grupos de suelo de la Provincia de Magallanes. Agr. Tec. Chile. XIX–XX, 228–302.
Díaz-Raviña, M., Lombao Vázquez, A., Barreiro Buján, A. I., Martín Jiménez, A., Carballas, T., and Fernández, T. (2018). Medium-Term Impact of Post-Fire Emergency Rehabilitation Techniques on a Shrubland Ecosystem in Galicia (NW Spain). Span. J. Soil Sci. 8, 322–346. doi:10.3232/SJSS.2018.V8.N3.03
Dlapa, P., Chrenková, K., Mataix-Solera, J., and Šimkovic, I. (2012). Soil Profile Improvement as a By-Product of Gully Stabilization Measures. Catena 92, 155–161. doi:10.1016/j.catena.2011.12.002
Doerr, S. H., Douglas, P., Evans, R. C., Morley, C. P., Mullinger, N. J., Bryant, R., et al. (2005). Effects of Heating and Post-Heating Equilibration Times on Soil Water Repellency. Soil Res. 43, 261–267. doi:10.1071/SR04092
Doerr, S. H., Shakesby, R. A., and Walsh, R. P. D. (1998). Spatial Variability of Soil Hydrophobicity in Fire-Prone Eucalyptus and Pine Forests, Portugal. Soil Sci. 163, 313–324. doi:10.1097/00010694-199804000-00006
Doerr, S. H., Shakesby, R. A., and Walsh, R. P. D. (2000). Soil Water Repellency: Its Causes, Characteristics and Hydro-Geomorphological Significance. Earth Sci. Rev. 51, 33–65. doi:10.1016/S0012-8252(00)00011-8
García-Corona, R., Benito, E., de Blas, E., and Varela, M. E. (2004). Effects of Heating on Some Soil Physical Properties Related to its Hydrological Behaviour in Two North-Western Span. Soils. Int. J. Wildland Fire 13, 195–199. doi:10.1071/WF03068
García-Orenes, F., Arcenegui, V., Chrenková, K., Mataix-Solera, J., Moltó, J., Jara-Navarro, A. B., et al. (2017). Effects of Salvage Logging on Soil Properties and Vegetation Recovery in a Fire-Affected Mediterranean Forest: A Two Year Monitoring Research. Sci. Total Environ. 586, 1057–1065. doi:10.1016/j.scitotenv.2017.02.090
Gee, G. W., and Bauder, J. W. (1986). “Particle-Size Analysis,” in Methods of Soil Analysis 1: Physical and Mineralogical Methods. Editor A. Klute 2nd ed. (Madison, WI: Am. Soc. Agron. Madison), 383–411.
Giovannini, G., Lucchesi, S., and Giachetti, M. (1988). Effect of Heating on Some Physical and Chemical Parameters Related to Soil Aggregation and Erodibility. Soil Sci. 146, 255–261. doi:10.1097/00010694-198810000-00006
Girona-García, A., Vieira, D. C. S., Silva, J., Fernández, C., Robichaud, P. R., and Keizer, J. J. (2021). Effectiveness of Post-Fire Soil Erosion Mitigation Treatments: A Systematic Review and Meta-Analysis. Earth Sci. Rev. 217, 103611. doi:10.1016/j.earscirev.2021.103611
González-Peñaloza, F. A., Zavala, L. M., Jordán, A., Bellinfante, N., Bárcenas-Moreno, G., Mataix-Solera, J, et al. (2013). Water Repellency as Conditioned by Particle Size and Drying in Hydrophobized Sand. Geoderma 209–210, 31–40. doi:10.1016/j.geoderma.2013.05.022
González-Pérez, J. A., González-Vila, F. J., Almendros, G., and Knicker, H. (2004). The Effect of Fire on Soil Organic Matter: A Review. Environ. Int. 30 (6), 855–870. doi:10.1016/j.envint.2004.02.003
Holz, A., and Veblen, T. T. (2011). The Amplifying Effects of Humans on Fire Regimes in Temperate Rainforests in Western Patagonia. Palaeogeogr. Palaeoclimatol. Palaeoecol. 311, 82–92. doi:10.1016/j.palaeo.2011.08.008
Holz, A., and Veblen, T. T. (2012). Wildfire Activity in Rainforests in Western Patagonia Linked to the Southern Annular Mode. Int. J. Wildland Fire 21, 114–126. doi:10.1071/WF10121
Huber, U. M., Markgraf, V., and Schäbitz, F. (2004). Geographical and Temporal Trends in Late Quaternary Fire Histories of Fuego-Patagonia, South America. Quat. Sci. Rev. 23, 1079–1097. doi:10.1016/j.quascirev.2003.11.002
IUSS Working Group WRB. (2015). Base referencial mundial del recurso suelo 2014, Actualización 2015. Sistema internacional de clasificación de suelos para la nomenclatura de suelos y la creación de leyendas de mapas de suelos. Informes sobre recursos mundiales de suelos 106. Roma: FAO.
Jex, G. W., Bleakley, B. H., Hubbell, D. H., and Munro, L. L. (1985). High Humidity-Induced Increase in Water Repellency in Some Sandy Soils. Soil Sci. Soc. Am. J. 49, 1177–1182. doi:10.2136/sssaj1985.03615995004900050021x
Jiménez-Pinilla, P., Mataix-Solera, J., Arcenegui, V., Delgado, R., Martín-García, J. M., Lozano, E., et al. (2016). Advances in the Knowledge of How Heating Can Affect Aggregate Stability in Mediterranean Soils: A XDR and SEM-EDX Approach. Catena 147, 315–324. doi:10.1016/j.catena.2016.07.036
Jordán, A., Zavala, L. M., Mataix-Solera, J., and Doerr, S. H. (2013). Soil Water Repellency: Origin, Assessment and Geomorphological Consequences. Catena 108, 1–5. doi:10.1016/j.catena.2013.05.005
Josa, R., Arias, X., and Solé, A. (1994). “Effects of Slashburning on Some Soil Physical Properties in an Olm-Oak Coppice,” in Soil Erosion and Degradation as a Consequence of Forest Fires. Editors M. Sala, and J. L. Rubio (Logroño: Geoforma Ediciones), 29–42.
Keeley, J. E. (2009). Fire Intensity, Fire Severity and Burn Severity: A Brief Review and Suggested Usage. Int. J. Wildland Fire 18, 116–126. doi:10.1071/WF07049
Kitzberger, T., Perry, G., Paritsis, J., Gowda, J., Tepley, A., Holz, A., et al. (2016). Fire-Vegetation Feedbacks and Alternative States: Common Mechanisms of Temperate Forest Vulnerability to Fire in Southern South America and New Zealand. N. Z. J. Bot. 54, 247–272. doi:10.1080/0028825x.2016.1151903
Larsen, I. J., MacDonald, L. H., Brown, E., Rough, D., Welsh, M. J., Pietraszek, J. H., et al. (2009). Causes of Post-Fire Runoff and Erosion: Water Repellency, Cover, or Soil Sealing? Soil Sci. Soc. Am. J. 73 (4), 1393–1407. doi:10.2136/sssaj2007.0432
Le, S., Josse, J., and Husson, F. (2008). FactoMineR: An R Package for Multivariate Analysis. J. Stat. Soft. 25, 1–18. doi:10.18637/jss.v025.i01
Lin, C.-Y., Chou, W.-C., Tsai, J.-S., and Lin, W.-T. (2006). Water Repellency of Casuarina Windbreaks (Casuarina equisetifolia Forst.) Caused by Fungi in Central Taiwan. Ecol. Eng. 26, 283–292. doi:10.1016/j.ecoleng.2005.10.010
Llovet, J., Josa, R., and Vallejo, V. R. (2008). Thermal Shock and Rain Effects on Soil Surface Characteristics: A Laboratory Approach. Catena 74, 227–234. doi:10.1016/j.catena.2008.03.017
Marden, C. J., and Clapperton, C. M. (1995). Fluctuations of the South Patagonian Ice-Field During the Last Glaciation and the Holocene. J. Quat. Sci. 10 (3), 197–209. doi:10.1002/jqs.3390100302
Martí, C., Badía, D., and Buesa, M. A. (2001). Determinación de la estabilidad estructural de suelos del Alto Aragón por tamizado en húmedo y lluvia simulada. Edafología 8, 21–30.
Mataix-Solera, J., Arcenegui, V., Zavala, L. M., Pérez-Bejarano, A., Jordán, A., Morugán-Coronado, A., et al. (2014). Small Variations of Soil Properties Control Fire-Induced Water Repellency. Span. J. Soil Sci. 4, 51–60. doi:10.3232/SJSS.2014.V4.N1.03
Mataix-Solera, J., Cerdà, A., Arcenegui, V., Jordán, A., and Zavala, L. M. (2011). Fire Effects on Soil Aggregation: A Review. Earth Sci. Rev. 109, 44–60. doi:10.1016/j.earscirev.2011.08.002
Mataix-Solera, J., and Doerr, S.H. (2004). Hydrophobicity and Aggregate Stability in Calcareous Topsoils from Fire-Affected Pine Forests in Southeastern Spain. Geoderma 118, 77–88. doi:10.1016/S0016-7061(03)00185-X
Mataix-Solera, J., Gómez, I., Navarro-Pedreño, J., Guerrero, C., and Moral, R. (2002). Soil Organic Matter and Aggregates Affected by Wildfire in a Pinus halepensis Forest in a Mediterranean Environment. Int. J. Wildland Fire 11, 107–114. doi:10.1071/WF02020
McKissock, I., Gilkes, R. J., Harper, R. J., and Carter, D. J. (1998). Relationships of Water Repellency to Soil Properties for Different Spatial Scales of Study. Soil Res. 36, 495–507. doi:10.1071/S97071
Mcwethy, D. B., Pauchard, A., García, R. A., Holz, A., González, M. E., Veblen, T. T., et al. (2018). Correction: Landscape Drivers of Recent Fire Activity (2001–2017) in South-Central Chile. PLoS One 13, e0205287. doi:10.1371/journal.pone.0205287
Medina, R. C. (2013). “Mega Wildfire in the World Biosphere Reserve (UNESCO), Torres del Paine National Park, Patagonia – Chile 2012: Work Experience in Extreme Behavior Conditions in the Context of Global Warming,” in Proceedings of the Fourth International Symposium on Fire Economics, Planning, and Policy: Climate Change and Wildfires. Gen. Tech. Rep. PSW-GTR-245 (English). Editor A. González-Cabán (Albany, CA: Pacific Southwest Research Station: U.S. Department of Agriculture, Forest Service), 191–201. Available at: https://www.fs.usda.gov/treesearch/pubs/44244
Mermoz, M., Kitzberger, T., and Veblen, T. T. (2005). Landscape Influences on Occurrence and Spread of Wildfires in Patagonian Forests and Shrublands. Ecology 86, 2705–2715. doi:10.1890/04-1850
Moore, G., and Blackwell, P. (1998). “Water Repellence,” in Soil Guide: A Handbook for Understanding and Managing Agricultural Soils. Editor G. Moore (Perth: Department of Agriculture, Western Australia), 49–59.
Moreno, P. I., Vilanova, I., Villa-Martínez, R. P., and Francois, J. P. (2018). Modulation of Fire Regimes by Vegetation and Site Type in Southwestern Patagonia since 13 Ka. Front. Ecol. Evol. 6, 34. doi:10.3389/fevo.2018.00034
Mundo, I. A., Villalba, R., Veblen, T. T., Kitzberger, T., Holz, A., Paritsis, J., et al. (2017). Fire History in Southern Patagonia: Human and Climate Influences on Fire Activity in Nothofagus pumilio Forests. Ecosphere 8, e01932. doi:10.1002/ecs2.1932
Neary, D. G., Ryan, K. C., and DeBano, L. F. (2005). Wildland Fire in Ecosystems: Effects of Fire on Soils and Water. Gen. Tech. Rep. RMRS-GTR-42-vol.4. Ogden, UT: U.S. Department of Agriculture, Forest Service, Rocky Mountain Research Station, 250.
Nelson, D. V., and Sommers, L. E. (1982). “Total Carbon, Organic Carbon, and Organic Matter,” in Methods of Soil Analysis, Part 2. Chemical and Biological Methods. Agronomy Monographs. Editor A. L. Page (Madison, WI: American Society of Agronomy, Inc., Soil Science Society of America, Inc.), 816.
Paul, E. A., and Clark, F. E. (1996). Soil Microbiology and Biochemistry. New York: Academic Press, 340.
Pausas, J. G., and Keeley, J. E. (2009). A Burning Story: the Role of Fire in the History of Life. Bioscience 59, 593–601. doi:10.1525/bio.2009.59.7.10
Pearsons, A., Robichaud, P. R., Lewis, S. A., Napper, C., and Clark, J. T. (2010). Field Guide for Mapping Post-Fire Soil Burn Severity. Gen. Tech. Rep. RMRS-GTR-243. Fort Collins, CO: U.S. Department of Agriculture, Forest Service, Rocky Mountain Research Station, 49.
Peña, M. A., and Ulloa, J. (2017). “Mapping the Post-Fire Vegetation Recovery by NDVI Time Series,” in First IEEE International Symposium of Geoscience and Remote Sensing (GRSS-CHILE), Valdivia, Chile, June 15–16, 2017 (Geoscience & Remote Sensing Society), 1–8. doi:10.1109/GRSS-CHILE.2017.7996002
Pisano, E. (1974). Estudio ecológico de la región continental sur de área andino patagónica. II. Contribución a la fitogeografía de la zona del Parque Nacional “Torres del Paine” N° 1 y 2. Anales Instituto de la Patagonia V, 59–104.
Robichaud, P. R., Beyers, J. L., and Neary, D. G. (2000). Evaluating the Effectiveness of Post Fire Rehabilitation Treatments. Gen. Tech. Rep. RMRS-GTR-63. Fort Collins: U.S. Department of Agriculture, Forest Service, Rocky Mountain Research Station, 85.
Roldán, A., García-Orenes, F., and Lax, A. (1994). An Incubation Experiment to Determine Factors Involving Aggregation Changes in an Arid Soil Receiving Urban Refuse. Soil Biol. Biochem. 26, 1699–1707. doi:10.1016/0038-0717(94)90323-9
RStudio Team. (2020). RStudio. Boston, MA: Integrated Development for R. RStudio, IncAvailable at: http://www.rstudio.com/ (Accessed February 15, 2021).
Salas, C., Donoso, P. J., Vargas, R., Arriagada, C. A., Pedraza, R., and Soto, D. P. (2016). The Forest Sector in Chile: An Overview and Current Challenges. J. For. 114, 562–571. doi:10.5849/jof.14-062
Scott, D. F. (1992). “The Influence of Vegetation Type on Soil Wettability,” in Proceedings of the 17th Congress of the Soil Science Society of South Africa, University of Stellenbosch, January 28–30, 1992, 10B21–10B26.
Shakesby, R., and Doerr, S. (2006). Wildfire as a Hydrological and Geomorphological Agent. Earth Sci. Rev. 74, 269–307. doi:10.1016/j.earscirev.2005.10.006
Soto, B., Benito, E., and Diaz-Fierros, F. (1991). Heat-Induced Degradation Processes in Forest Soils. Int. J. Wildland Fire 1, 147–152. doi:10.1071/WF9910147
Teramura, A. H. (1980). Relationships Between Stand Age and Water Repellency of Chaparral Soils. Bull. Torrey Bot Club 107, 42–46. doi:10.2307/2484849
Terefe, T., Mariscal-Sancho, I., Peregrina, F., and Espejo, R. (2008). Influence of Heating on Various Properties of Six Mediterranean Soils. A Laboratory Study. Geoderma 143, 273–280. doi:10.1016/j.geoderma.2007.11.018
Úbeda, X., and Sarricolea, P. (2016). Wildfires in Chile: A Review. Glob. Planet Change 146, 152–161. doi:10.1016/j.gloplacha.2016.10.004
Varela, M. E., Benito, E., and de Blas, E. (2005). Impact of Wildfires on Surface Water Repellency in Soils of Northwest Spain. Hydrol. Process. 19, 3649–3657. doi:10.1002/hyp.5850
Vidal, O. (2012). Torres del Paine on Fire. Pat. J. Available at: http://www.patagonjournal.com (Accessed January 15, 2015).
Wallis, M. G., Horne, D. J., and McAuliffe, K. W. (1990). A Study of Water Repellency and Its Amelioration in a Yellow-Brown Sand. N. Z. J. Agric. Res. 33, 139–144. doi:10.1080/00288233.1990.10430670
Wallis, M. G., Horne, D. J., and Palmer, A. S. (1993). Water Repellency in a New Zealand Development Sequence of Yellow Brown Sands. Soil Res. 31, 641–654. doi:10.1071/SR9930641
Wessel, A. T. (1988). On Using the Effective Contact Angle and the Water Drop Penetration Time for Classification of Water Repellency in Dune Soils. Earth Surf. Process. Landf. 13, 555–561. doi:10.1002/esp.3290130609
Weyman-Kaczmarkowa, W., and Pędziwilk, Z. (2000). The Development of Fungi as Affected by pH and Type of Soil, in Relation to the Occurrence of Bacteria and Soil Fungistatic Activity. Microbiol. Res. 155, 107–112. doi:10.1016/S0944-5013(00)80045-2
Zavala, L. M., Granged, A. J. P., Jordán, A., and Bárcenas-Moreno, G. (2010). Effect of Burning Temperature on Water Repellency and Aggregate Stability in Forest Soils Under Laboratory Conditions. Geoderma 158, 366–374. doi:10.1016/j.geoderma.2010.06.004
Zegers, G., Arellano, E., and Östlund, L. (2020). Using Forest Historical Information to Target Landscape Ecological Restoration in Southwestern Patagonia. Ambio 49, 986–999. doi:10.1007/s13280-019-01232-8
Keywords: wildfire, Patagonia, soil degradation, aggregate stability, soil water repellency, Torres del Paine
Citation: Mataix-Solera J, Arellano EC, Jaña JE, Olivares L, Guardiola J, Arcenegui V, García-Carmona M, García-Franco N and Valenzuela P (2021) Soil Vulnerability Indicators to Degradation by Wildfires in Torres del Paine National Park (Patagonia, Chile). Span. J. Soil Sci. 11:10008. doi: 10.3389/sjss.2021.10008
Received: 25 April 2021; Accepted: 31 May 2021;
Published: 02 July 2021.
Edited by:
Agustin Merino, University of Santiago de Compostela, SpainCopyright © 2021 Mataix-Solera, Arellano, Jaña, Olivares, Guardiola, Arcenegui, García-Carmona, García-Franco and Valenzuela. This is an open-access article distributed under the terms of the Creative Commons Attribution License (CC BY). The use, distribution or reproduction in other forums is permitted, provided the original author(s) and the copyright owner(s) are credited and that the original publication in this journal is cited, in accordance with accepted academic practice. No use, distribution or reproduction is permitted which does not comply with these terms.
*Correspondence: Jorge Mataix-Solera, am9yZ2UubWF0YWl4QHVtaC5lcw==