- Sustainable Use, Management and Reclamation of Soil and Water Research Group, Escuela Técnica Superior Ingeniería Agronómica, Universidad Politécnica de Cartagena, Cartagena, Spain
There is a need to assess alternative cropping systems for climate change mitigation. Hence, we aimed to evaluate if cowpea, a legume crop with high climate adaptability and active rhizodeposition, can reduce GHG emissions when intercropped with melon, if different intercropping patterns can affect these soil GHG emissions, and elucidate if GHG emissions are related by soil and crop properties. We compared a cowpea and melon monocultures with different melon-cowpea intercropping patterns during two crop cycles. The different melon-cowpea intercropping patterns were: row intercropping 1:1 (melon:cowpea), row intercropping 2:1 (melon:cowpea) and mixed intercropping (alternate melon/cowpea plants within the same row), receiving 30% less fertilizers than monocrops. Results showed that CO2 emission rates were higher in the row 2:1 and row 1:1 intercropping systems compared to mixed intercropping, melon monocrop and cowpea monocrop, with the lowest emissions, likely due to the highest density of both plant species, which may stimulate microbial communities. Soil N2O emission rates were not affected by crop diversification, with very low values. Soil CO2 and N2O emissions were not correlated with environmental factors, soil properties or crop yield and quality, suggesting that crop management and plant density and growth were the main factors controlling GHG emissions. When the GHG emissions were expressed on a crop production basis, the lowest values were observed in mixed intercropping, owing to higher crop production. However, the 1:1 and 2:1 cowpea intercropping systems, with the lowest overall crop production, showed higher values of GHG emissions per unit of product, compared to cowpea monocrop. Thus, intercropping systems, and mostly mixed intercropping, have the potential to contribute to sustainable agriculture by increasing land productivity, reducing the need for synthetic fertilizers and decreasing GHG emissions per unit of product. These results highlight the importance of considering both agricultural productivity and greenhouse gas emissions when designing and implementing intercropping systems.
Introduction
Annual greenhouse gas (GHG) emissions continue to globally increase unabatedly (Boden et al., 2009). Conventional agriculture in the last decades has caused in many situations water scarcities, soil and water pollution, low biodiversity, high levels of GHG emissions, high erosion rates, loss of soil organic matter (SOM) and high incidence of pests and diseases (FAO, 2017). One-third of out GHG emissions come from agriculture (Gilbert, 2012). The three GHGs associated with agriculture are CO2, CH4, and N2O. Natural sources of CO2 in agriculture are related to machinery use, soil respiration or above ground plant respiration (Oertel et al., 2016). Methane is generated in soils via methanogenesis, a process that occurs in the absence of oxygen, and is subsequently consumed by microorganisms known as methanotrophs. (Dutaur and Verchot, 2007). N2O emissions result from nitrification, which involves the conversion of NH4+ to NO3− via NO2−, and denitrification, a process that reduces NO3− to N2O and N2 (Oertel et al., 2016). N2O soil emissions have shown an increasing trend after addition of crop residues or application of inorganic fertilizers (Baggs et al., 2000). As a consequence, the priority in current farming systems is maintaining high crop yields and social welfare while decreasing negative effects to the agroecosystems (Duhamel and Vandenkoornhuyse, 2013). In the last years, an interest in organic farming and crop diversification is growing as a feasible alternative to counteract the impact of conventional intensive monocropping, through crop divesification and an optimized use of natural resources. Crop diversification includes one, several or all of: (i) diversification of crops grown on the same field in successive growing seasons (crop rotation); (ii) diversification of crops grown on the same land within a growing season (multiple cropping); and (iii) diversification of crops grown in proximity in the same field (mixed intercropping, strip intercropping, row intercropping) (Mao et al., 2015). Intercropping, in general, includes one cash crop and one or several partner crops, where the highest possible yields in the cash crop is intended (Maitra et al., 2021). Resources are supposed to be better used in intercropping systems in comparison to its respective monocrop leading to greater productivity (Li et al., 2006; Bedoussac et al., 2015). In terms of mitigating GHG emissions, intercropping has been proposed as an effective strategy (Senbayram et al., 2015; Wang et al., 2021).
Melon (Cucumis melo L.) is among the most common species cultivated in regions with warm climates (Aldoshin et al., 2020). Melon monocrops under conventional farming, like many others monocrops, tend to reduce SOM and soil microbial and invertebrate biodiversity, contributing to soil and water pollution by pesticides and excessive use of fertilizers (Hijri et al., 2006; Jiao et al., 2011). Under arid and semiarid conditions, the cowpea (Vigna unguiculata (L.) Walp.) is the most common legume cropped owing to its high climate adaptability, low nutrient needs, and its active rhizodeposition, improving soil fertility (Sánchez-Navarro et al., 2019; Amorim et al., 2022). However, the use of cowpea intercropped with melon has not been previously study in terms of climate change mitigation strategy. It has been previously reported that legumes can be a suitable alternative for adaptation to climate change because they decrease GHG emissions and favour C sequestration and storage (Lüscher et al., 2014). The introduction of legumes in intercropping systems has helped reduce fertilizer rates and machinery used, and so reduce GHG emissions (Murphy-Bokern et al., 2017). Legumes can reduce the emission of CO2 derived from fossil fuels compared to cropping systems that use synthetic N fertilizers, as they are able to fix atmospheric N through symbiosis with rhizobia (Jensen et al., 2012). Under this context, Cuartero et al. (2022a) assessed the soil bacterial community of a melon-cowpea intercropping system; they verified that the practice of intercropping changed the structure of the soil bacterial community, and these changes were linked to higher levels of total N and available P, with an increase in melon crop yield. In this context, the use of intercropping strategies that involve both melon and cowpea crops has the potential to address the challenges of achieving high yields while reducing negative environmental practices such as soil GHG emmissions.
The objectives of this study were to: i) assess how intercropping between melon (C. melo), and cowpea (V. unguiculata) can contribute to soil GHG emissions compared with conventional melon and cowpea monocultures; ii) evaluate if different intercropping patterns can affect these soil GHG emissions; and iii) elucidate if GHG emissions are related by soil and crop properties. Thus, our initial hypothesis was that the use of cowpea in intercropping with melon may increase soil CO2 and N2O emissions by higher rhizodeposition and activation of soil microbial communities. Nonetheless, the growth of cowpea in the same space than melon should enhance overall crop yields by the collection of new products, with no negative effect on the cash crop. This would be associated to lower CO2e per unit of crop production.
Materials and Methods
Study Site and Experimental Design
Briefly, this experiment was carried out in Cartagena, South-East of Spain, at Tomás Ferro Experimental Farm of the Universidad Politécnica de Cartagena (37° 41′ N; 0° 57′ E). Climate is semiarid Mediterranean with a total annual precipitation of 275 mm and a mean annual temperature of 18°C. Annual potential evapotranspiration surpasses 900 mm. Soil is classified as Haplic Calcisol (loamic, hypercalcic) (WRB, 2015), with clay loam texture.
We compared a melon (Cucumis melo L. var. Sacharinus Naud) monocrop and a cowpea (Vigna unguiculata (L.) Walp. var. fradel) monocrop with different melon-cowpea intercropping patterns during two crop cycles (2019 and 2020). Melon (seedlings with two leaves) and cowpea were planted and sown, respectively, on the 07/05/2019 and 04/06/2020 for both cycles. The different melon-cowpea intercropping patterns were: row intercropping 1:1 (melon:cowpea), row intercropping 2:1 (melon:cowpea) and mixed intercropping (alternate melon/cowpea plants within the same row). The field experiment was previously explained in Marcos-Pérez et al. (2023). Briefly (Figure 1), it was established as a completely randomized design with three replications, and each plot had 150 m2. Melon seedlings were planted in a density of 4,000 plants ha−1, with a spacing of 200 cm between rows and 120 cm between plants in all plots (monocrop and intercropped systems). Cowpea seeds in the monocrop were sown in a density of 50,000 plants ha−1, with a spacing of 100 cm between rows and 20 cm between plants. Cowpea seeds were sown between two rows of melon in the row intercropped patterns, spacing 100 cm between melon and cowpea rows; the separation of cowpea plants within the same row was 20 cm. Thus, the density of plants was 25,000 plants ha−1 and 15,000 plants ha−1 in the row 1:1 and row 2:1 patterns, respectively. In the mixed system, cowpea was sown in all melon rows between two melon plants, and so in a density of 4,000 plants ha−1, with a spacing of 200 cm between rows and 120 cm between plants. So, density of melon was the same in the different treatments, but the density of cowpea changed. In all systems, compost was added annually at the beginning of each cycle, as a traditional practice in the region (Sánchez-Navarro et al., 2019), with a dose of 14,000 kg ha−1. This compost derived from animal manures and had the following characteristics: moisture = 20%; pH = 6.5; electrical conductivity = 20 dS m−1; total nitrogen content = 1.1%; C:N ratio = 17.4; P2O5 content = 1.7% and K2O content = 1.9%. No herbicides were added, and the weed control was done by hand-hoeing. For monocultures, plots were tilled before sowing/planting up to 30–40 cm depth, following the traditional practice in the region. For intercropping, tillage was reduced up to 15–20 cm. All cropping systems received drip irrigation and were managed under organic practices. During each cycle, we applied in all plots 3,100 m3 ha−1 of irrigation water. As organic fertilizers, we applied as fertigation Norgan (NPK: 3-0-7) and Zen (NPK: 5-4-0) (Fyneco SL, Spain). In the intercropped system, under all patterns, we reduced the fertilizers rate by 30% compared to the melon monoculture. Thus, in the melon monoculture we applied 3437 L ha−1 of organic fertilizers with fertigation, in the cowpea monocrop we applied 2187 L ha−1 and in the three intercrop patterns 2453 L ha−1. Fertigation started after 2 weeks of planting/sowing and finished with harvest, applying 3–4 irrigation events per week. Reduction of fertilization in intercropping started with melon blossom. Harvest of melon took place on 24–31/07/2019 and 24/08/2020, while cowpea was harvested on 04–05/05/2019 and 08/09/2020. Harvest of these two species is manual in commercial farms, and there was no need for special machinery. After harvest, melon crop residues in the monoculture were removed from the plots, as traditional practice in the region, while in the cowpea monoculture and intercropped plots, crop residues were incorporated into the soil at 15–20 cm.
Soil Greenhouse Gas Measurements
Measurements of CO2, N2O and CH4 were made every 7 days in all replicated treatments from 20/06/2019 to 17/09/2019 and from 11/06/2020 to 03/09/2020, between 9:00 and 12:00, following the procedure explained in (Sánchez-Navarro et al., 2022). The basic experimental procedure used in this study was the dynamic gas chamber technique (Álvaro Fuentes et al., 2019). The chamber was made of non-oxidisable steel, with a diameter of 7.5 cm and a height of 20 cm, with one inlet and one outlet connected to a photoacoustic infrared spectroscopy multi-gas analyser with ultra-sensitive cantilever pressure sensor (Gasera One, Gasera Ltd). The dynamic system with inlet and outlet in the chamber permits a continuous flow and avoids pressure fluctuations. Three chambers per plot were inserted into the bare soil to a depth of 10 cm, within two melon/cowpea plants in the monocultures. For the patterns row 1:1 and row 2:1, measures were performed in both melon and cowpea rows, inserting three chambers per plot between two melon or cowpea plants. For the mix intercropping pattern, the three chambers per plot were located equidistant to a cowpea plant and to a melon plant. CO2, N2O, and CH4 emissions were quantified every 1 min for a period of 5 min to assess the linear trend. Nonetheless, no CH4 emissions were recorded during the experimental period. CO2 and N2O emissions rates were expressed as the difference between the quantification at the end and the beginning of the measure period divided by the time. CO2 and N2O cumulative emissions for each treatment were estimated by numerical integration (Chen et al., 2013). GHG emissions were converted into CO2 equivalent (CO2e), and then cumulative emission data (g m−2) were also expressed on a production basis (g kg−1) for the experimental period to assess the emissions per product of each treatment. For this, N2O emissions were converted into CO2e according to their global warming potential, which is 265 (Vasconcelos et al., 2022).
Meteorological data were measured using an automatic weather station located in the experimental farm. Soil temperature (T) and soil moisture (M) were measured using a ProCheck and 5TM sensors (Decagon Devices, USA) introduced at 15 cm depth adjacent to the place where GHG measures were done.
Soil and Plant Sampling and Soil Analyses
Soil was sampled at the beginning of the trial, and at the end of each cycle, as previously explained in Marcos-Pérez et al. (2023). Briefly, all plots were sampled at 0–10 cm and 10–30 cm depth. Two composite soil samples derived from 5 sampling points per plot were collected avoiding the border effect. Soil was collected in the crop line, between two plants. Each sample was divided into two aliquots. First aliquot was air dried for 7 days, sieved <2 mm and kept at room temperature. The other aliquot was sieved <2 mm and kept at 4°C for the determination of NH4+ and NO3−. We used for correlations, regressions and multivariate analyses, soil properties measured and explain in Marcos-Pérez et al. (2023).
Statistical Analysis
Data were checked to ensure normal distribution using the Kolmogorov-Smirnov test at p > 0.05. Homoscedasticity was checked by the Levene test. All GHG data were submitted to two-way repeated measures ANOVA, with measurement date as within-subject factor, and treatment (monoculture/diversification) as between-subject factor. GHG emission values for the experimental period were also submitted, independently for each date, to a one-way ANOVA and Tukey’s post hoc test (p < 0.05) to compare significant differences between treatments. Relationships among soil properties and gases were studied using Pearson correlations. A principal components analysis (PCA) was performed with all data to study the structure of dependence and correlation established among the variables studied. Statistical analyses were performed in R version 4.1.2 for Windows.
Results
Greenhouse Gas Emission Rates
As an average, soil temperature was 32.3°C in all crop systems during both crop cycles, with no significant differences between them. Soil moisture was significantly highest in the melon monocrop (p < 0.05), with an average value of 15.9%, compared to all the other treatments, with an average value of 13.3%, with no significant differences between them (Figure 2). Soil CO2 emission rates did not follow soil moisture and temperature trends, without significant correlations between CO2 emission rates and these environmental parameters. Soil CO2 emission rates were significantly affected by time (p < 0.001) and diversification (p < 0.05) treatments, with the interaction time x diversification treatments being also significant (p < 0.001). There were significantly higher CO2 emission rates in the row 2:1 and row 1:1 intercropping systems, with an average value for the experimental period of 91.88 g m−2 h−1 and 91.77 g m−2 h−1 respectively, compared to cowpea monocrop, with the lowest emissions (52.98 g m−2 h−1), melon monocrop with 59.78 g m−2 h−1 and mixed intercropping system with an average of 66.99 g m−2 h−1. In this line, five out of the 23 CO2 emission rate measures were significantly different with respect treatments (marked with * in Figure 2), with highest values of CO2 emission rate under melon growth, one for melon monocrop and the other four ones for intercropped melon (two for 1:1 and the other two for 2:1).
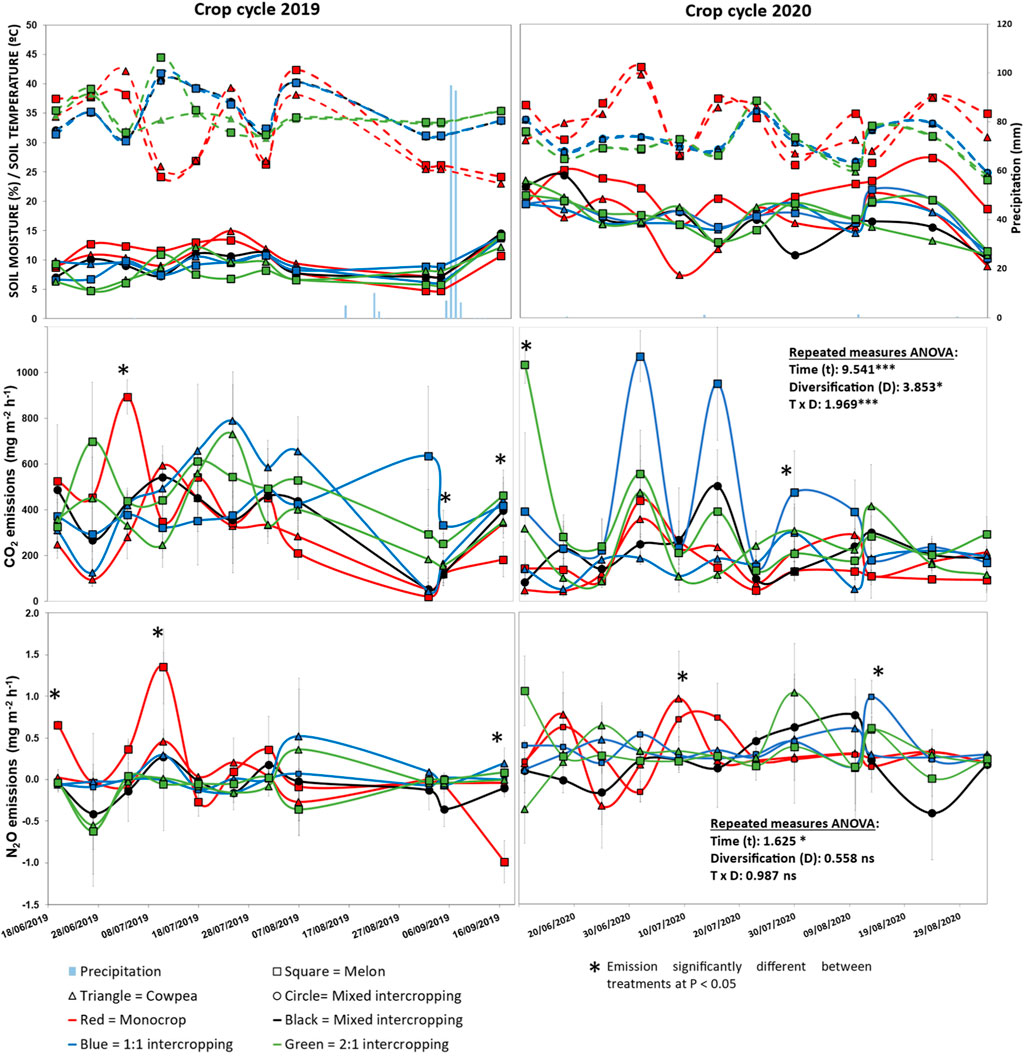
FIGURE 2. Environmental conditions during the duration of the experiment (top), soil CO2 emission rates (centre) and soil N2O emission rates (bottom) from the crops cycles 2019 (left) and 2020 (right). Values are mean ± standard error (n = 6). For environmental conditions, dashed lines represent soil temperature, while solid lines represent soil moisture. For repeated measures ANOVA data (performed on the entire data set from both crop cycles): significant at ***p < 0.001; *p < 0.05; ns: not significant (p > 0.05).
Soil N2O emission rates were not correlated to soil moisture nor temperature, with oscillations up and down of 0.00 mg m−2 h−1 average (Figure 2). Soil N2O emission rates were only significantly affected by time (p < 0.05), with no significant effect by crop diversification. However, five out of the 23 N2O emission rate measures were significantly different between treatments, with lowest values under row 2:1 and mixed intercropping (Figure 2). As an average, N2O emission rates were 0.0211 mg m−2 h−1 in melon monocrop, 0.008 mg m−2 h−1 in cowpea monocrop, −0.016 mg m−2 h−1 in mixed intercropping, 0.011 mg m−2 h−1 in 1:1 intercropping and −0.001 mg m−2 h−1 in 2:1 intercropping system for the entire experimental period.
Overall Cumulative Emissions
There were no significant correlations between soil properties, crop yield and cumulative gases. Soil cumulative CO2 emissions showed significant differences (p < 0.05) between treatments over the entire experimental period (Table 1). Cumulative CO2 emissions were significantly highest under the melon growth in the row 2:1 and row 1:1 intercropping systems, with an average value for the experimental period of 1716 g m−2 h−1 and 1,655 g m−2 h−1, respectively. However, these values were not significantly different from the melon monocrop (1,083 g m−2 h−1). The lowest significant cumulative CO2 emissions were observed in the cowpea monocrop (945 g m−2 h−1), with no significant differences with the cowpea growth in the intercrops (Table 1). Soil cumulative N2O and CO2e emissions were, in general, not significantly different between systems over the entire experimental period (Table 1). Nonetheless, when the GHG emissions were expressed on a crop production basis there were significant differences (p < 0.001) between treatments. In this line, there were significantly highest values in the soil under cowpea growth in the row 2:1 and row 1:1 cowpea intercropping systems, with average values of 9,038 g kg−1 and 7,342 g kg−1, respectively. Mixed intercropping showed the lowest value with 187 g kg−1 (Table 1).
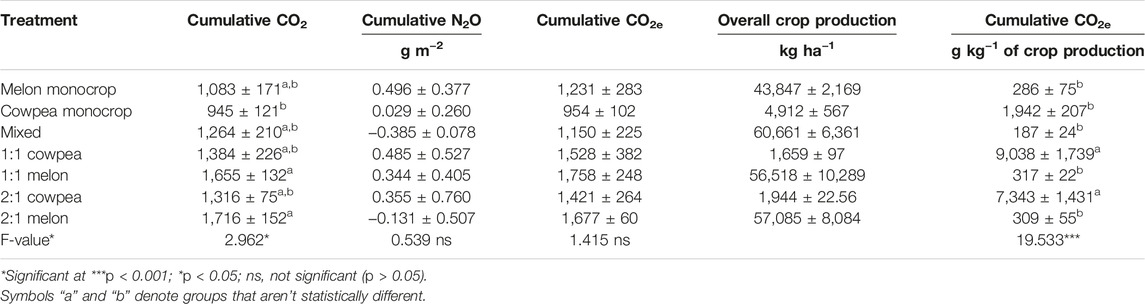
TABLE 1. Cumulative soil CO2, N2O, CO2 equivalent (CO2e) and CO2 equivalent expressed on a production basis for melon and cowpea crops in monoculture and intercropping patterns for two crop cycles.
Interrelationships Between GHG and Soil Properties
The PCA performed with the cumulative GHG emissions and soil physicochemical properties showed that 73.3% of the total variation could be explained by the first three PCs (Table 2). PC1 (35.8% of the variation) was positively related to EC, Fe, POC, Mn, Cu and Na and negatively related to cumulative CO2 and pH. PC1 separated samples of the first crop cycle (negative scores) from the second crop cycle (positive scores) (Figure 3A). PC2 (22.3% of the variation) was positively related to K, CEC, Mg, Ca and Nt. PC2 significantly separated melon monocrop (negative values) from all the other systems (positive values) (Figure 3B). PC2 also significantly separated the cowpea monocrop, with highest factor scores, from both row intercroppings. PC3 (15.2% of the variation) was positively related with P, Zn and negatively related to NO3−. PC3 separated monocrops, with negative scores, from intercropping systems, with positive scores (Figure 3B).
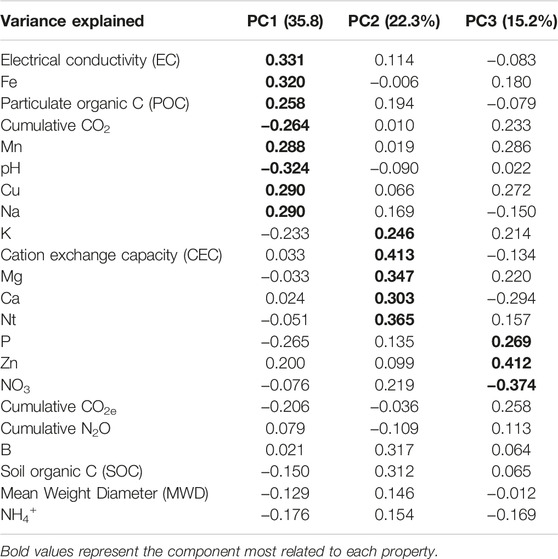
TABLE 2. Matrix of the PCA obtained with soil properties and GHG in monocrops and intercropping patterns over the entire experimental period.
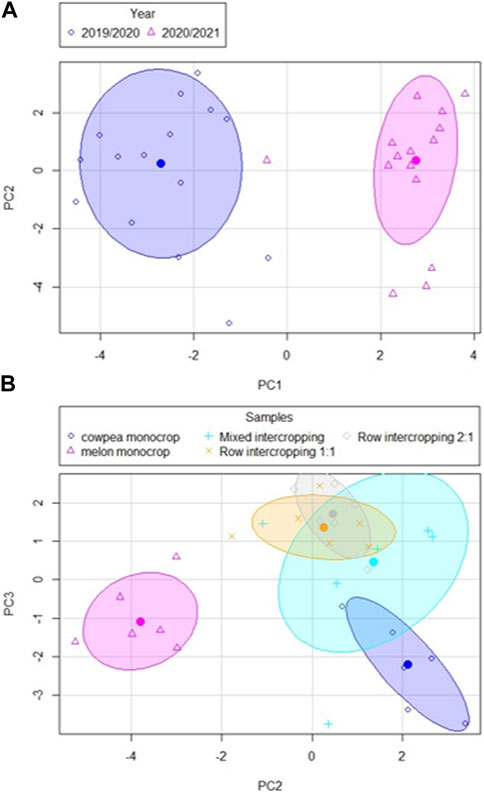
FIGURE 3. PCA factor scores of soil properties and GHG for monocultures and the different intercropping patterns over the entire experimental period. (A) Distribution of PC1 and PC2 over time. (B) Distribution of PC2 and PC3 across different samples. Ellipses of concentration represent 70% of the data.
Discussion
Intercropping between melon and cowpea successfully performed, with improvements in overall crop yields, but not clear patterns concerning GHG emissions. The Mediterranean climate of the study site, with high temperatures during both crop cycles, may have played a role in the lack of correlation between GHG emissions and soil temperature (Oertel et al., 2016). This is because soil temperature was >25°C in all cases, which favours high microbial activity and so no limitation by low temperatures (Dilekoğlu and Sakin, 2017). Water was not a limiting factor for microbial activity either. It seems that microorganisms were not affected by changes in soil moisture, confirmed by the lack of correlations between soil moisture and GHG emissions, since irrigation maintained high soil water levels to achieve high productivity (Sánchez-Navarro et al., 2023). Steinweg et al. (2012) suggested that soil temperature is the most important factor for microbial activity when soil water content is not limiting factor. In addition, previous research has indicated the high control of soil temperature on GHG emissions under Mediterranean climate, but when GHG emissions were monitored during the entire year, and so there was an effect of seasons (Zornoza et al., 2018; Sánchez-Navarro et al., 2022). Thus, in our experiment, the summer season with high temperatures for the melon crop cycles and the irrigated conditions can explain the lack of effect of temperature and moisture on GHG emissions. Therefore, changes in GHG emissions may likely be affected by the availability of nutrients, crop development and crop management. However, Sánchez-Navarro et al. (2023) also observed, as in this study, an absence of relationships between CO2 emissions and soil properties such as TOC, Nt, pH, texture, EC, nitrates and ammonium, which might indicated that crop management (tillage, weed control, disease/pest control) and crop growth are the main factors controlling GHG emissions.
Intercropped systems, independently of the pattern, showed higher values of CO2 emission rates in both cycles comparing to melon monocrop (Figure 2). No effect of precipitation, fertilization event or harvest was observed in relation with the CO2 emission rate measures. In this sense, the interaction between the two different plant species in the intercropping system may have increased the quantity and diversity of root exudates in the soil, which in turn can attract a greater diversity and quantity of soil microorganisms, with higher activity, and so, CO2 emissions (Zhang et al., 2018; Tang et al., 2021; Cuartero et al., 2022a). Additionally, the crop residues from the different intercropped crops incorporated in the soil can provide different sources of nutrients for microorganisms, which can also increase soil microbial diversity and activity (Li et al., 2020), leading to an increasing in decomposition and mineralization of organic matter (Yerlikaya et al., 2020). Soil cumulative CO2 emissions were higher in row 2:1 and 1:1 intercropping systems compared to monocrops (Table 1). This could be explained by the effect of higher density of melon and cowpea plants in these two systems, and thus with higher rhizodeposition and stimulation of soil microbial communities. Higher density of plants could affect the spatial root distribution of the intercropped species, favoring lateral spread, and so higher interaction between both species (Yerlikaya et al., 2020). Thus, it seems that soil CO2 emission rates mostly depended on the growth and biomass of the living plant community (Chen et al., 2013). Relationships between higher CO2 emissions and higher soil microbial activity have been previously identified (Wu et al., 2017; Usyskin-Tonne et al., 2021). Accordingly, Cuartero et al. (2022b) assessed the bacterial community of the soil samples coming from the first sampling of the present study, and confirmed that intercropping systems, independently of the pattern, increased the abundance of beneficial microorganisms. This corroborates the hypothesized affirmation that the inclusion of cowpea in intercropping with melon may increase soil CO2 emission by active rhizodeposition and stimulation of soil microbial communities.
In this agroecosystem, N2O emissions were low, which highlights an effective N fertilization under organic management. Consequently, no significant influence of temperature, soil moisture, tillage practices, or the adoption of intercropping systems could be established on the emission of N2O. Nonetheless, it has been reported that intercropping can contribute to reduce N2O emissions (Huang et al., 2019). This is because intercropped crops can improve the efficiency of nutrient use and decrease the dependence for synthetic fertilizers. In previous studies, N2O emissions from intercropping were lower than in monocrops, like maize-peanut and maize-soybean systems (Jian-xiong et al., 2014). However, although not significant, intercropping systems showed lower values of N2O emission rates, in line with previous research. Furthermore, the reduction of tillage and the incorporation of crop residues like done in the intercropping systems tends to minimize N2O emissions by reducing the accumulation of inorganic N forms (Amours et al., 2023).
Hence, the lack of significant relationships between GHG emissions and soil or plant properties may imply that vegetation composition, growth and density (Ward et al., 2013), the presence of specific microorganisms (Cavicchioli et al., 2019) and crop management may have greater control. Nonetheless, it is important to highlight that the lack of significant correlations in this study does not necessarily mean that there is no relationship between the variables, but rather than the relationship may be more complex than expected, or more data and analyses are needed to understand the relationship.
The PCA results showed that GHGs were not key factors explaining the dynamics of variation of the soil samples. In this line, only CO2 emissions explained differences, and not between systems, but between years (Figure 3A). So, there was a temporal variability not related to management with regard to CO2 emissions, with no key effect of CO2 at explaining the variability of cropping systems. This is probably because CO2 is strongly influenced by the activity of soil microorganisms, which are in turn affected by environmental factors such as moisture and temperature, with higher environmental temperature, as average, during the first crop cycle. As environmental factors change between years, soil CO2 emissions can vary temporally (Li et al., 2008; Panosso et al., 2009). Besides, there was a negative relationship in PC1 between POC and cumulative CO2. This may indicate that owing to higher decomposition of organic compounds, the labile organic fraction decreased in those places with higher CO2 emissions (Paustian et al., 2000). This highlights the importance of including different organic C pools, which are more sensitive than SOC at explaining changes derived by management or environmental factors (Rocci et al., 2021). CO2 cumulative emissions were also negatively with EC, Na, Fe, Mn and Cu. Elevated level of metals and salts in soil can negatively affect soil microbial activity (Stuczynski et al., 2003). Moreover, a high content of salts in the soil can affect the availability of nutrients for plants (Fageria et al., 2011), which can reduce the release of organic compounds which activate microbial communities.
Although the cowpea contributed to less cumulative CO2e (Table 1), when expressed on a production basis, CO2e increased due to the lower overall crop production compared to melon. In this sense, intercropping systems have been significantly more productive and so efficient than cowpea monoculture. This is because in our experiment, intercropping helped improve soil fertility and nutrient use efficiency, leading to increased yields (Cuartero et al., 2022a; Cuartero et al., 2022b). Thus, the intercropping system can provide a more sustainable and profitable alternative to monoculture systems. Besides, intercropping can reduce costs by decreasing the need for external inputs such as synthetic fertilizers while increasing yield per unit area (Marcos-Pérez et al., 2023). According to our results, not all intercropping systems behave equally regarding GHG emissions per unit of product. The 1:1 and 2:1 cowpea intercropping systems, with lower overall crop production, showed significantly higher GHG emissions per unit of product compared to the cowpea monocrop. In contrast, mixed intercropping seems more efficient concerning GHG emissions per unit of product because it increases production and reduces GHG emissions with respect to the other two intercropping patterns. Therefore, melon growth based on intercropping with cowpea, mainly as mixed intercropping, reduced tillage and addition of crop residues can be a cost-effective and sustainable alternative for agricultural production with lower levels of greenhouse gas emissions per unit of product.
Conclusion
Our study shows that the implementation of intercropping systems can have important implications for both agricultural ecosystem and greenhouse gas emissions. The inclusion of cowpea in intercropping with melon increased soil CO2 emission rates likely due to active rhizodeposition and stimulation of soil microbial communities by the interaction of roots of different species. However, the growth of cowpea in the same space than melon increased land productivity by the harvest of new products, with no negative effect on the main crop, which was associated to lower CO2e per unit of melon. This can lead to a cost-effective and sustainable alternative for agricultural production with lower levels of GHG emissions. In this study, mixed intercropping led to a more efficient pattern because it increased production and reduced GHG emissions compared to the others. No effect was observed on N2O emissions in terms of cropping systems. The lack of correlations between GHG emissions, environmental factors and soil and plant properties in this study could be attributed to several factors, including the high temperatures owing to summer time, the high soil moisture level owing to irrigation, the availability of nutrients for microbial and plant growth, the organic management practices or the density and development of plants. Nonetheless, future research should delve deeper into these factors to better understand their influence on GHG emissions in agricultural systems.
Data Availability Statement
The original contributions presented in the study are included in the article/supplementary material, further inquiries can be directed to the corresponding author.
Author Contributions
RZ and VS-N contributed to the study conception and design. Material preparation, data collection and analysis were performed by MM-P, RZ, and VS-N. The first draft of the manuscript was written by MM-P and RZ commented on previous versions of the manuscript. All authors contributed to the article and approved the submitted version.
Funding
This research was funded by the Project AsociaHortus granted by the Spanish Ministry of Science and Innovation (AGL2017-83975-R).
Conflict of Interest
The authors declare that the research was conducted in the absence of any commercial or financial relationships that could be construed as a potential conflict of interest.
Acknowledgments
MM-P acknowledges the financial support from the Spanish Ministry of Science, Innovation and Universities through the “Ayudas para contratos predoctorales para la formación de doctores 2018” Program (PRE2018-085702). We also acknowledge the technicians of the Tomás Ferro Experimental Farm of the Universidad Politécnica de Cartagena for their support in sowing/planting, monitoring the crops and harvest.
References
Aldoshin, N., Mamatov, F., Ismailov, I., and Ergashov, G. (2020). Development of Combined Tillage Tool for Melon Cultivation. Eng. Rural. Dev. 19, 767–772. doi:10.22616/ERDev.2020.19.TF175
Álvaro Fuentes, J., Lóczy, D., Thiele-Bruhn, S., and Zornoza Belmonte, R. (2019). Handbook of Plant and Soil Analysis for Agricultural Systems. Spain: Universidad Politécnica de Cartagena.
Amorim, M. R., Mendes, L. W., Antunes, J. E. L., Oliveira, L. M. S., Melo, V. M. M., Oliveira, F. A. S., et al. (2022). Cowpea Nodules Host a Similar Bacterial Community Regardless of Soil Properties. Appl. Soil Ecol. 172, 104354. doi:10.1016/j.apsoil.2021.104354
Amours, J. D., Pelster, D. E., Gagn, G., Anne, J., Chantigny, M. H., Angers, D. A., et al. (2023). Combining Reduced Tillage and Green Manures Minimized N2O Emissions From Organic Cropping Systems in a Cool Humid Climate. Agric. Ecosyst. Environ. 341, 108205. doi:10.1016/j.agee.2022.108205
Baggs, E. M., Rees, R. M., Smith, K. A., and Vinten, A. J. A. (2000). Nitrous Oxide Emission From Soils After Incorporating Crop Residues. Soil use Manag. 16, 82–87. doi:10.1111/j.1475-2743.2000.tb00179.x
Bedoussac, L., Journet, E. P., Hauggaard-Nielsen, H., Naudin, C., Corre-Hellou, G., Jensen, E. S., et al. (2015). Ecological Principles Underlying the Increase of Productivity Achieved by Cereal-Grain Legume Intercrops in Organic Farming. A Review. Agron. Sustain. Dev. 35, 911–935. doi:10.1007/s13593-014-0277-7
Boden, T. A., Marland, G., and Andres, R. J. (2009). “Global, Regional, and National Fossil-Fuel CO2 Emissions,” in Carbon dioxide Inf. Anal. Center, Oak ridge Natl. Lab. Oak Ridge, Tenn., USA: U.S. Department of Energy.
Cavicchioli, R., Ripple, W. J., Timmis, K. N., Azam, F., Bakken, L. R., Baylis, M., et al. (2019). Scientists’ Warning to Humanity: Microorganisms and Climate Change. Nat. Rev. Microbiol. 17, 569–586. doi:10.1038/s41579-019-0222-5
Chen, W., Wang, Y., Zhao, Z., Cui, F., Gu, J., and Zheng, X. (2013). The Effect of Planting Density on Carbon Dioxide, Methane and Nitrous Oxide Emissions From a Cold Paddy Field in the Sanjiang Plain, Northeast China. Agric. Ecosyst. Environ. 178, 64–70. doi:10.1016/j.agee.2013.05.008
Cuartero, J., Pascual, J. A., Vivo, J.-M., Özbolat, O., Sánchez-Navarro, V., Weiss, J., et al. (2022a). Melon/Cowpea Intercropping Pattern Influenced the N and C Soil Cycling and the Abundance of Soil Rare Bacterial Taxa. Front. Microbiol. 13, 1004593. doi:10.3389/fmicb.2022.1004593
Cuartero, J., Pascual, J. A., Vivo, J. M., Özbolat, O., Sánchez-Navarro, V., Egea-Cortines, M., et al. (2022b). A First-Year Melon/Cowpea Intercropping System Improves Soil Nutrients and Changes the Soil Microbial Community. Agric. Ecosyst. Environ. 328, 107856. doi:10.1016/j.agee.2022.107856
Dilekoğlu, M. F., and Sakin, E. (2017). Effect of Temperature and Humidity in Soil Carbon Dioxide Emission. J. Anim. Plant Sci. 27, 1596–1603.
Duhamel, M., and Vandenkoornhuyse, P. (2013). Sustainable Agriculture: Possible Trajectories From Mutualistic Symbiosis and Plant Neodomestication. Trends Plant Sci. 18, 597–600. doi:10.1016/j.tplants.2013.08.010
Dutaur, L., and Verchot, L. V. (2007). A Global Inventory of the Soil CH4 Sink. Glob. Biogeochem. Cycles 21, 2734. doi:10.1029/2006GB002734
Fageria, N. K., Gheyi, H. R., and Moreira, A. (2011). Nutrient Bioavailability in Salt Affected Soils. J. Plant Nutr. 34, 945–962. doi:10.1080/01904167.2011.555578
FAO (2017). The Future of Food and Agriculture: Trends and Challenges. Rome, Italy: Food and Agriculture Organization. Annu Rep 296, 1–180.
Gilbert, N. (2012). One-Third of Our Greenhouse Gas Emissions Come From Agriculture. Nature 2012, 1–2. doi:10.1038/nature.2012.11708
Hijri, I., Sýkorová, Z., Oehl, F., Ineichen, K., Mäder, P., Wiemken, A., et al. (2006). Communities of Arbuscular Mycorrhizal Fungi in Arable Soils are Not Necessarily Low in Diversity. Mol. Ecol. 15, 2277–2289. doi:10.1111/j.1365-294X.2006.02921.x
Huang, J., Sui, P., Gao, W., and Chen, Y. (2019). Effects of Maize-Soybean Intercropping on Nitrous Oxide Emissions From a Silt Loam Soil in the North China Plain. Pedosphere 29, 764–772. doi:10.1016/S1002-0160(17)60389-8
Jensen, E. S., Peoples, M. B., Boddey, R. M., Gresshoff, P. M., Henrik, H. N., Alves, B. J. R., et al. (2012). Legumes for Mitigation of Climate Change and the Provision of Feedstock for Biofuels and Biorefineries. A Review. Agron. Sustain. Dev. 32, 329–364. doi:10.1007/s13593-011-0056-7
Jian-xiong, H., Yuan-quan, C., Peng, S. U. I., Sheng-wei, N. I. E., and Wang-sheng, G. A. O. (2014). Soil Nitrous Oxide Emissions Under Maize-Legume Intercropping System in the North China Plain. J. Integr. Agric. 13, 1363–1372. doi:10.1016/S2095-3119(13)60509-2
Jiao, H., Chen, Y., Lin, X., and Liu, R. (2011). Diversity of Arbuscular Mycorrhizal Fungi in Greenhouse Soils Continuously Planted to Watermelon in North China. Mycorrhiza 21, 681–688. doi:10.1007/s00572-011-0377-z
Li, L., Sun, J., Zhang, F., Guo, T., Bao, X., Smith, F. A., et al. (2006). Root Distribution and Interactions Between Intercropped Species. Oecologia 147, 280–290. doi:10.1007/s00442-005-0256-4
Li, N., Gao, D., Zhou, X., Chen, S., Li, C., and Wu, F. (2020). Intercropping With Potato-Onion Enhanced the Soil Microbial Diversity of Tomato. Microorganisms 8, 834–915. doi:10.3390/microorganisms8060834
Li, Y. L., Otieno, D., Owen, K., Zhang, Y., Tenhunen, J., and Rao, X. Q. (2008). Temporal Variability in Soil CO2 Emission in an Orchard Forest Ecosystem. Pedosphere 18, 273–283. doi:10.1016/S1002-0160(08)60017-X
Lüscher, A., Mueller-Harvey, I., Soussana, J. F., Rees, R. M., and Peyraud, J. L. (2014). Potential of Legume-Based Grassland-Livestock Systems in Europe: A Review. Grass Forage Sci. 69, 206–228. doi:10.1111/gfs.12124
Maitra, S., Hossain, A., Brestic, M., Skalicky, M., Ondrisik, P., Gitari, H., et al. (2021). Intercropping—A Low Input Agricultural Strategy for Food and Environmental Security. Agronomy 11, 343. doi:10.3390/agronomy11020343
Mao, L. L., Zhang, L. Z., Zhang, S. P., Evers, J. B., van der Werf, W., Wang, J. J., et al. (2015). Resource Use Efficiency, Ecological Intensification and Sustainability of Intercropping Systems. J. Integr. Agric. 14, 1542–1550. doi:10.1016/S2095-3119(15)61039-5
Marcos-Pérez, M., Sánchez-Navarro, V., and Zornoza, R. (2023). Intercropping Systems Between Broccoli and Fava Bean Can Enhance Overall Crop Production and Improve Soil Fertility. Sci. Hortic. Amst. 312, 111834. doi:10.1016/j.scienta.2023.111834
Murphy-Bokern, D., Stoddard, F. L., and Watson, C. A. (2017). Legumes in Cropping Systems. United Kingdom: CABI.
Oertel, C., Matschullat, J., Zurba, K., Zimmermann, F., and Erasmi, S. (2016). Greenhouse Gas Emissions From Soils—A Review. Chem. Erde 76, 327–352. doi:10.1016/j.chemer.2016.04.002
Panosso, A. R., Marques, J., Pereira, G. T., and La Scala, N. (2009). Spatial and Temporal Variability of Soil CO2 Emission in a Sugarcane Area Under Green and Slash-And-Burn Managements. Soil Tillage Res. 105, 275–282. doi:10.1016/j.still.2009.09.008
Paustian, K., Six, J., Elliott, E. T., and Hunt, H. W. (2000). Management Options for Reducing CO2 Emissions From Agricultural Soils. Biogeochemistry 48, 147–163. doi:10.1023/A:1006271331703
Rocci, K. S., Lavallee, J. M., Stewart, C. E., and Cotrufo, M. F. (2021). Soil Organic Carbon Response to Global Environmental Change Depends on its Distribution Between Mineral-Associated and Particulate Organic Matter: A Meta-Analysis. Sci. Total Environ. 793, 148569. doi:10.1016/j.scitotenv.2021.148569
Sánchez-Navarro, V., Martínez-Martínez, S., Acosta, J. A., Almagro, M., Martínez-Mena, M., Boix-Fayos, C., et al. (2023). Soil Greenhouse Gas Emissions and Crop Production With Implementation of Alley Cropping in a Mediterranean Citrus Orchard. Eur. J. Agron. 142, 126684. doi:10.1016/j.eja.2022.126684
Sánchez-Navarro, V., Shahrokh, V., Martínez-Martínez, S., Acosta, J. A., Almagro, M., Martínez-Mena, M., et al. (2022). Perennial Alley Cropping Contributes to Decrease Soil CO2 and N2O Emissions and Increase Soil Carbon Sequestration in a Mediterranean Almond Orchard. Sci. Total Environ. 845, 157225. doi:10.1016/j.scitotenv.2022.157225
Sánchez-Navarro, V., Zornoza, R., Faz, Á., and Fernández, J. A. (2019). Does the Use of Cowpea in Rotation With a Vegetable Crop Improve Soil Quality and Crop Yield and Quality? A Field Study in SE Spain. Eur. J. Agron. 107, 10–17. doi:10.1016/j.eja.2019.03.007
Senbayram, M., Wenthe, C., Lingner, A., Isselstein, J., Steinmann, H., Kaya, C., et al. (2015). Legume-Based Mixed Intercropping Systems May Lower Agricultural Born N2O Emissions. Energy. Sustain. Soc. 6, 2–9. doi:10.1186/s13705-015-0067-3
Steinweg, J. M., Dukes, J. S., and Wallenstein, M. D. (2012). Modeling the Effects of Temperature and Moisture on Soil Enzyme Activity: Linking Laboratory Assays to Continuous Field Data. Soil Biol. Biochem. 55, 85–92. doi:10.1016/j.soilbio.2012.06.015
Stuczynski, T. I., McCarty, G. W., and Siebielec, G. (2003). Response of Soil Microbiological Activities to Cadmium, Lead, and Zinc Salt Amendments. J. Environ. Qual. 32, 1346. doi:10.2134/jeq2003.1346
Tang, X., Zhang, Y., Jiang, J., Meng, X., Huang, Z., Wu, H., et al. (2021). Sugarcane/Peanut Intercropping System Improves Physicochemical Properties by Changing N and P Cycling and Organic Matter Turnover in Root Zone Soil. PeerJ 9, 108800–e10928. doi:10.7717/peerj.10880
Usyskin-Tonne, A., Hadar, Y., Yermiyahu, U., and Minz, D. (2021). Elevated CO2 and Nitrate Levels Increase Wheat Root-Associated Bacterial Abundance and Impact Rhizosphere Microbial Community Composition and Function. ISME J. 15, 1073–1084. doi:10.1038/s41396-020-00831-8
Vasconcelos, A. L. S., Cherubin, M. R., Cerri, C. E. P., Feigl, B. J., Borja Reis, A. F., and Siqueira-Neto, M. (2022). Sugarcane Residue and N-Fertilization Effects on Soil GHG Emissions in South-Central, Brazil. Biomass Bioenergy 158, 106342. doi:10.1016/j.biombioe.2022.106342
Wang, X., Chen, Y., Yang, K., Duan, F., Liu, P., Wang, Z., et al. (2021). Effects of Legume Intercropping and Nitrogen Input on Net Greenhouse Gas Balances, Intensity, Carbon Footprint and Crop Productivity in Sweet Maize Cropland in South China. J. Clean. Prod. 314, 127997. doi:10.1016/j.jclepro.2021.127997
Ward, S. E., Ostle, N. J., Oakley, S., Quirk, H., Henrys, P. A., and Bardgett, R. D. (2013). Warming Effects on Greenhouse Gas Fluxes in Peatlands are Modulated by Vegetation Composition. Ecol. Lett. 16, 1285–1293. doi:10.1111/ele.12167
WRB (2015). World Reference Base for Soil Resources 2014, International Soil Classification System for Naming Soils and Creating Legends for Soil Maps. Rome, Italy: Food and Agriculture Organization.
Wu, H. S., Chen, S. Y., Li, J., Liu, D. Y., Zhou, J., Xu, Y., et al. (2017). An Approach to Mitigating Soil CO2 Emission by Biochemically Inhibiting Cellulolytic Microbial Populations Through Mediation via the Medicinal Herb Isatis Indigotica. Atmos. Environ. 158, 259–269. doi:10.1016/j.atmosenv.2017.03.046
Yerlikaya, B. A., Ömezli, S., and Aydoğan, N., 2020. Climate Change Forecasting and Modeling for the Year of 2050. in Environment, Climate, Plant and Vegetation Growth. Berlin, Germany: Springer, 109–122. doi:10.1007/978-3-030-49732-3_5
Zhang, M. M., Wang, N., Hu, Y. B., and Sun, G. Y. (2018). Changes in Soil Physicochemical Properties and Soil Bacterial Community in Mulberry (Morus Alba L)/Alfalfa (Medicago Sativa L) Intercropping System. Microbiologyopen 7, 005555–e611. doi:10.1002/mbo3.555
Keywords: intercropping, legumes, CO2 emissions, N2O emissions, crop diversification
Citation: Marcos-Pérez M, Sánchez-Navarro V and Zornoza R (2023) Soil Greenhouse Gas Emissions in Intercropped Systems Between Melon and Cowpea. Span. J. Soil Sci. 13:11368. doi: 10.3389/sjss.2023.11368
Received: 17 March 2023; Accepted: 31 July 2023;
Published: 11 August 2023.
Edited by:
Avelino Núñez-Delgado, University of Santiago de Compostela, SpainCopyright © 2023 Marcos-Pérez, Sánchez-Navarro and Zornoza. This is an open-access article distributed under the terms of the Creative Commons Attribution License (CC BY). The use, distribution or reproduction in other forums is permitted, provided the original author(s) and the copyright owner(s) are credited and that the original publication in this journal is cited, in accordance with accepted academic practice. No use, distribution or reproduction is permitted which does not comply with these terms.
*Correspondence: Mariano Marcos-Pérez, bWFyaWFuby5tYXJjb3NAdXBjdC5lcw==