- 1Posgrado en Ciencias de la Tierra, Universidad Nacional Autónoma de México, Ciudad de México, México
- 2Instituto de Geología, Departamento de Ciencias Ambientales y del Suelo, Universidad Nacional Autónoma de México, Ciudad de México, México
- 3Laboratorio Nacional de Geoquímica y Mineralogía, Ciudad de México, México
- 4Departamento de Ecología y Recursos Naturales del Centro Universitario de la Costa Sur, Universidad de Guadalajara, Guadalajara, Jalisco, México
- 5Departamento de Edafología y Química Agrícola, Escuela Politécnica Superior de Ingeniería, Universidad de Santiago de Compostela, Lugo, Spain
Prescribed burn is a tool that must imply low soil burn severity (SBS) levels; however, a wide range of soil impacts have been demonstrated because of the influence of very variable factors. The effects on biological, physical, and chemical soil properties are well reported in numerous studies; nonetheless, there are still questions about the effect of prescribed burns on soils at the micro-scale. As a result, an analysis of the link between micromorphological features and SBS does not currently exist. Thus, the main aim of the present study is to perform a micro-scale evaluation for complementing the SBS visual examination after prescribed burning in a managed pine forest in western Mexico. Morphometry and micromorphology analyses of mineral soil revealed that at low SBS levels, only the soil structure in the first centimeter is affected by prescribed burns. While at high SBS, the prescribed burn affected the first 2 cm, showing soil structure disturbance, ash filling porous, and soil aggregates getting reddish. Therefore, immediate actions have to be made by land managers after applying prescribed burns before the first rain to prevent post-fire surface soil erosion, particularly in bare soil patches where the burned aggregates are more susceptible to rain splash and runoff.
Introduction
The impacts of prescribed burns on soils are spatially variable due to the high heterogeneity of the environmental conditions (Hubbert et al., 2006; Girona-García et al., 2019). Although prescribed burns imply the application of low-intensity fire (Fernandes et al., 2013), different intensities and severities have been reported (Alcañiz et al., 2018). Therefore, their effects on soil properties can be very variable depending on the fire and vegetation type, the previous soil conditions, and their correct execution (Scharenbroch et al., 2012; Badía et al., 2017; Alcañiz et al., 2018; Girona-García et al., 2019). Additionally, the sampling method is another factor that can also influence the variations of soil fire impacts, such as the time elapsed after the fire, the mixture of charred O and Ah horizons, and even the depth of soil sampling with a dilution effect (Armas-Herrera et al., 2016; Lucas-Borja et al., 2019; Pereira et al., 2023). The variability of all these factors for each intervened area can produce distinctive soil impacts, resulting in patches with different soil burn severity levels (Hubbert et al., 2006; Alcañiz et al., 2018).
The degree of soil burn severity determines the modification of biological, chemical, and physical soil properties (Santín and Doerr, 2016). The effect of heating on soil properties such as pH, total organic carbon (TOC), total nitrogen (TN), and total phosphorous (TP) have been extensively studied (Alcañiz et al., 2018; García-Oliva et al., 2018; Merino et al., 2019). It is accepted that pH values, TOC, and TP contents increase after a low-severity prescribed burn (Ulery et al., 1993; Arocena and Opio, 2003; Úbeda et al., 2005; Afif and Oliveira, 2006; Scharenbroch et al., 2012), however, there is an unclear pattern in the case of TN (Alcañiz et al., 2018). After the fire, changes in soil physical properties such as aggregates stability, bulk density, and moisture have been described (Neary et al., 1999; Debano, 2000; Mataix-Solera et al., 2011; Badía et al., 2017). Some authors reported a decrease in soil structure stability because high temperatures disrupted aggregates cement, generating cracks and the disaggregation of soil peds (Badía and Martí, 2003a; Arocena and Opio, 2003; Chief et al., 2012) related to TOC losses and changes in clays or Fe contents (Mataix-Solera and Doerr, 2004; Mataix-Solera et al., 2011). Regarding bulk density, there are also controversial results because after a prescribed burn, it can increase (Hubbert et al., 2006), decrease (Chief et al., 2012), or remain unchangeable (Phillips et al., 2000). These discrepancies are likely due to the different soil burn severity (SBS). Likewise, soil moisture behavior can vary according to the soil moisture conditions and the prescribed burn type (Girona-García et al., 2019). Even depending on the previous soil moisture conditions, this property can protect the soil against the effects of fire (Badía et al., 2017). Soil moisture can have insignificant changes following a prescribed fire (Iverson and Hutchinson, 2002), and under laboratory conditions, soil moisture content can decrease dramatically in the first centimeters of soil depth (Badía et al., 2017).
For evaluating the impacts of burn areas, a classification index has been proposed to estimate the SBS with a five levels scale based on soil organic layer characteristics and mineral soil surface morphology (Ryan and Noste, 1985; Vega et al., 2013). This index is closely related to soil physico-chemical changes from unburned to high burn severity (Vega et al., 2013; Merino et al., 2018). For prescribed burns, this tool must imply low SBS levels (Scharenbroch et al., 2012) corresponding to levels without visible surface soil impact but with charred forest floor (Vega et al., 2013). Although the important contribution of the SBS index, there are still questions about the changes at micro-scale that are not visible to the naked eye, underestimating the fire effect on the soil. Micromorphology is a valuable technique to detect the combustion features (e.g., charcoal, charred plant material, ashes, and reddened aggregates) in soils and sediments, commonly used in archaeological contexts (Alperson-Afil, 2012; Berna et al., 2012; Mallol et al., 2017), making possible to interpret the fire history in a specific place. In the case of prescribed burns, micromorphology has already been used in soils of Australia, United States, and Spain (Greene et al., 1990; Phillips et al., 2000; Badía et al., 2020). Particularly, Badía et al. (2020) observed the formation of coatings of fine sand-size particles mixed with charcoal fragments present in the soil surface and infill pores. However, no studies still associate the micromorphological characteristics of burn soils with different levels of SBS.
In this study, we applied micromorphological and morphometric analyses to complement the information from the field visual examination and soil physico-chemical properties changes after a prescribed burn where different SBS levels occurred. Micromorphology and morphometry are novelty analyses that support the interpretations of the level of affection caused by the fire that can help in the future management of the forest and the understanding of post-fire soil processes.
Materials and Methods
Study Area
The study was conducted in a managed pine forest in Ahuacapán Ejido (19° 36′–19° 41′ N and 104° 16′–104° 21′ W) of Autlán de Navarro, Jalisco, Mexico (Figure 1). This area is located within the upper zone of the Sierra de Manantlán Biosphere Reserve, at 1,900 m a.s.l., where the climate is temperate sub-humid Ca (w2) (w) (e) (g) (Martínez et al., 1991). The mean annual temperature, precipitation, and humidity, from 2011 to 2020, are 15.7°C ± 0.17°C, 1,771 ± 173 mm, and 80.7% ± 20.8%, respectively (Zuloaga-Aguilar, 2021). The soils are classified as Distric Cambisols, developed on Tertiary (Paleocene-Oligocene) intermediate extrusive igneous rocks (Jardel and Moreno, 2000). The forest vegetation is dominated by Pinus douglasiana under silvicultural practices, together with Arbutus xalapensis, Fraxinus uhdei, and Carpinus tropicalis in the underground (Jardel and Moreno, 2000). Because of human pressure and climatic conditions, this forest is considered a fire-prone ecosystem, subjected to moderate and low-intensity fires every 4.2–7.3 years (Rubio, 2007; Cerano-Paredes et al., 2015). Therefore, previous fires might mask part of the results of the present analysis.
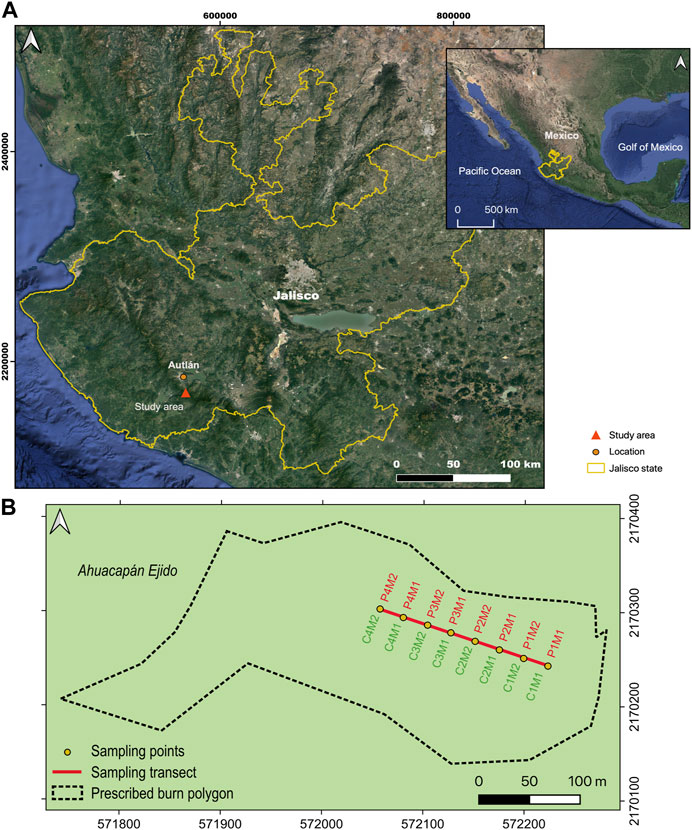
FIGURE 1. Study area (A) location in the SW of Jalisco state, Mexico. (B) The prescribed burn area (7.3 ha) and the sampling design. The sampling was made in the same transect before and after the prescribed burn. Green names correspond to the unburned samples and red names to the burned samples.
Prescribed Burn Event Characteristics
The prescribed burning event was carried out on 21 March 2017, to reduce hazardous fuels and improve pine forest regeneration. Forests firefighters of the National Forestry Commission (CONAFOR), in conjunction with a local forest brigade of the Ahuacapán Ejido, conducted the burning supervised by fire management specialists from the University of Guadalajara (DERN-IMECBIO) and from the Sierra de Manantlán Biosphere Reserve.
The size of the burned area was 7.3 ha (Figure 1). The weather conditions were a relative humidity of 38%–76%, a temperature of 19°C–22°C, and a monthly accumulated precipitation of 3.81 mm from 1 to 21 March. The prescribed burning had a duration of 10 h, with the following observed characteristics: a flame height of 1.01–3.27 m; a rate of spread between 1.4–2.5 m min−1; flame temperature between 439°C and 593°C; and total vertical consumption of 9 cm of fuel (81% of the pre-burn material, see Figure 2) (Pérez-Salicrup, 2018).
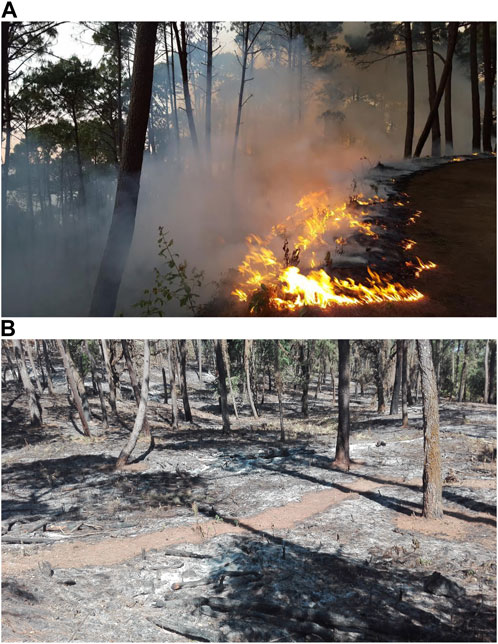
FIGURE 2. (A) The prescribed burn event. (B) Features of the burned site the next day. Photos by Faviola Castillo.
Soil Sampling Design and Soil Burn Severity (SBS) Assessment
A transect randomly oriented from SE to NW 175 m long (Figure 1B) was established within the burned area. Before and after the prescribed burn, a sampling area of 1 m2 every 25 m was delimited. After the fire event, on 22 March 2017, in the center of each square, the soil burn severity (SBS) was evaluated by a visual estimation using a 30 cm × 30 cm sampling metallic frame, following the procedure described by Ryan and Noste. (1985) modified by Vega et al. (2013). This SBS index considered the condition of the remaining soil organic layer, whether it was unburned, partially or wholly charred, or if it was completely consumed remaining only ash. Also, this post-fire assessment considered the visual characteristics of the mineral soil: if this was undisturbed, if the soil structure and the soil organic matter (SOM) were affected, and if there were soil color changes. Then, with these descriptions, a value from 0 to 5 was assigned for defining the SBS level in each sampling point, according to Vega et al. (2013) classification.
Before and after the fire, three types of samples were collected inside the 1 m2 area from the first 5 cm of the mineral soil at each sampling point: 1) five samples integrated into one bulk sample for chemical analyses, stored in a plastic bag, 2) one unaltered sample was taken with a 118.8 cm3 cylindrical steel core (5 cm depth), which was used for bulk density and moisture determinations, and 3) one unaltered sample was taken, from the organic layer and the upper 5 cm of the mineral soil, for micromorphological and morphometric analyses using cylindrical PVC cores.
Soil Analysis
Soil Physical Properties
The color measurements were triplicated with a colorimeter (COLORLITE Sph 870) considering the CIELAB color system (CIE, 1986). In this system, the color is represented by three scalar parameters: L*, luminosity, with values from 0 to 100 (from black to white, respectively); a*, related to changes from redness (a* > 0) to greenness (a* < 0); and b*, related to transitions from yellowness (b* > 0) to blueness (b* < 0) (Cancelo-González et al., 2014). Colors were obtained from sieved (2 mm) and homogenized samples.
Bulk density and moisture were evaluated using the cylinder method (Flores-Delgadillo and Alcalá-Martínez, 2010). Cores filled with soil were weighed fresh and dried at 105°C for 48 h until they reached a constant weight. The mass difference was used for moisture calculation, and the known volume’s dry mass was used to calculate the bulk density.
Chemical Properties
Soil pH was measured in deionized water (1:10 W:V ratio) with a portable pH meter (Corning) in fresh samples. The total organic carbon (TOC), total nitrogen (TN), and total phosphorous (TP) were obtained after dry samples to 105°C until constant weight. The TOC was determined by combustion and coulometric detection (Huffman, 1977) in a UIC CM150 carbon analyzer. The TN and TP were determined in dry samples after acid digestion in H2SO4, H2O2, K2SO4, and CuSO4 mixture at 360°C for 4 h. The TN was determined by the micro-Kjeldahl method (Bremner, 1996) and TP by the colorimetric method with molybdate after ascorbic acid reduction (Murphy and Riley, 1962); both elements were quantified by colorimetry in Seal AA3 segmented continue flow analyzer.
Morphometric and Micromorphological Analyses
The unaltered soil samples were previously dried and then impregnated with polyester resin. Thin sections (30 µm) were prepared at the Institute of Geology (UNAM). Morphometric analysis was done on high-resolution scanned images (2,400 dpi and 24 bits) of the thin sections using an EPSON scanner (Perfection V700 Photo). The Image-Pro Plus 7.0 software was used for processing the images. We selected a representative area (6 cm2) of each thin section for developing the analysis. This analysis visually identified some burn features classified, such as charred elements, reddened zones, and ashes. The unburnt soil matrix and the porous space were also identified. The software separated and grouped the elements into five classes of different colors. Finally, we obtained a reclassified image, from which we calculated the proportion of each group per area.
The micromorphological analysis was made using a petrographic microscope (OLYMPUS). The analysis focused on identifying combustion features in thin sections such as charcoal, ashes, burned shells, charred aggregates, and reddened aggregates, according to Nicosia and Stoops. (2017) and Stoops et al. (2018).
Statistical Analysis
A correlation analysis (significance of p ≤ 0.05) was performed among physical, chemical, and morphometric variables to avoid redundancy in the principal component analysis (PCA). The PCA was done to explain the changes due to the fire effect considering samples before and after the prescribed burn. The selection of the variables for the PCA considered the highest significance among them, demonstrated by their highest correlation. A JMP trial software was used for statistical analyses (JMP Statistical Discovery LLC, Cary, NC, United States).
Results
Soil Burn Severity Levels Recorded After the Prescribed Burn
The prescribed burn event in the Ahuacapán Ejido (Figure 1) left a heterogeneous mosaic of areas with different SBS (Figure 2). Although most of the area was affected by low SBS (levels 2 and 1), high SBS levels (4 and 5) were only found in smaller areas (only in two sampling sites of eight, Table 1; Figures 3C, D). In level 1, the organic layer was partially burned; in the case of level 2, the Oa layer was completely charred, and gray ash was present. In these low SBS, the temperature did not affect the mineral soil and remained undisturbed (Figures 3A, B). On the contrary, in the highest SBS areas, the organic layer was entirely consumed (levels 4 and 5). In SBS 4 level, high temperatures also affected the uppermost mineral soil horizon in the 0–2 upper cm, showed a slightly reddish color, lack of fine roots, and degraded structure. Besides, in SBS 4, a thick layer of white ash covered the mineral soil; while in SBS 5 most of the surface soil was bare, with black and reddish colors (Figures 3C, D).
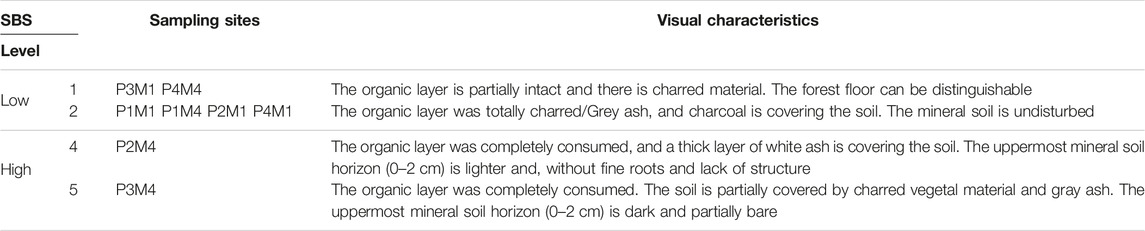
TABLE 1. Visual description of the soil burn severity (SBS) levels found after the prescribed burn according to Vega et al. (2013).
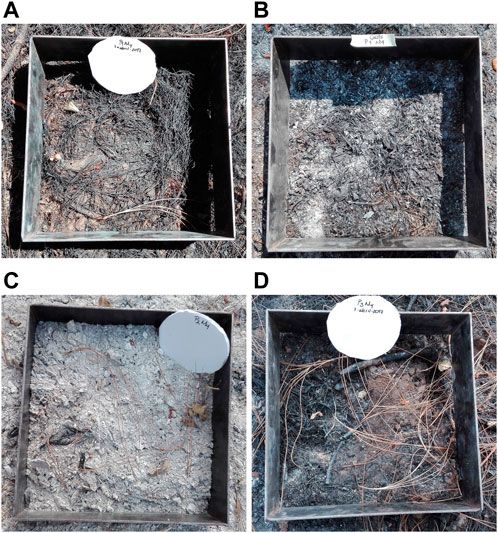
FIGURE 3. Examples of the different levels of SBS after the prescribed burn in Ahuacapán Ejido: (A) low SBS (level 1), sample point P4M4; (B) low SBS (level 2), sample point P1M1; (C) high SBS (level 4), sample point P2M4; (D) high SBS (level 5), sample point P3M4. Photos by Faviola Castillo. The sampling metallic frames are 30 cm × 30 cm.
Changes in Physical and Chemical Soil Properties After the Prescribed Burn
Soil color measurements and mean moisture values for samples collected in the uppermost 5 cm showed changes after the prescribed burn (Figure 4). The mean values of the color parameters (L*, a*, and b*) decreased after soil burning, no matter the severity level. The reduction of luminosity (L*) mean values evidenced a soil darkening after the burn (Figure 4A). The decrease in the mean value of the a* and b* parameters showed fewer reddish and yellowish colors than the unburned condition (Figures 4B, C, respectively). Regarding soil moisture, this property tended to decrease slightly after the fire (Figure 4D). On the other hand, the soil bulk density increased 21.4% in the burned soils affected by low SBS and 22.6% by high SBS (Figure 4E).
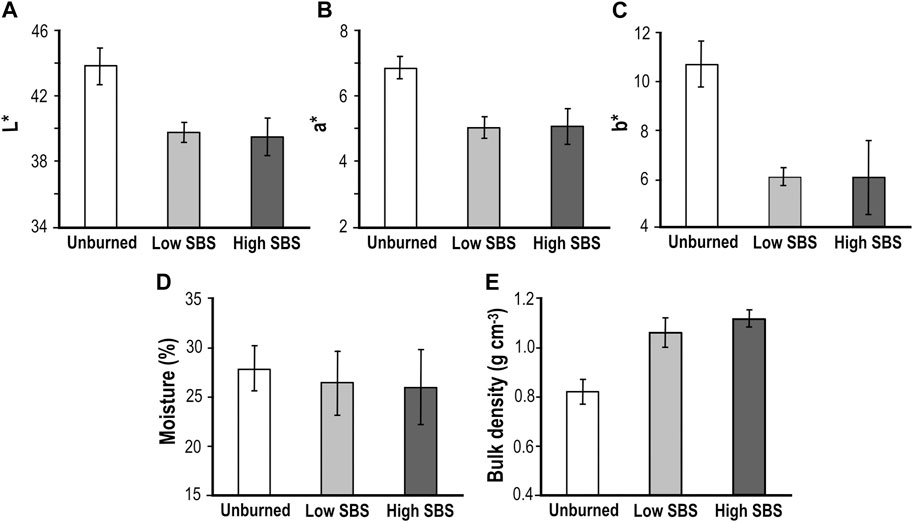
FIGURE 4. Mean values and standard errors of physical properties of the soil samples collected from uppermost soil layer, before (unburned) and after the prescribed burn (low and high SBS). Low SBS corresponds to levels 1 and 2 (n = 6), and high SBS to levels 4 and 5 (n = 2). (A) L*; (B) a*; (C) b*; (D) Moisture; (E) Bulk density.
Concerning the chemical soil properties, the pH values slightly increased after burning, especially in the high SBS (Figure 5A). The total organic carbon (TOC), no clear trend could be distinguished; it was reduced in soils affected by low SBS and increased in high SBS (Figure 5B). The TN concentrations did not show evident changes either (Figure 5C). Different trends in C/N ratios were evident because this ratio decreased in the low SBS. However, remarkable increases were found in the high SBS (Figure 5D). The TP values increased from unburned to burned condition 0.7 mg g−1 (Figure 5E).
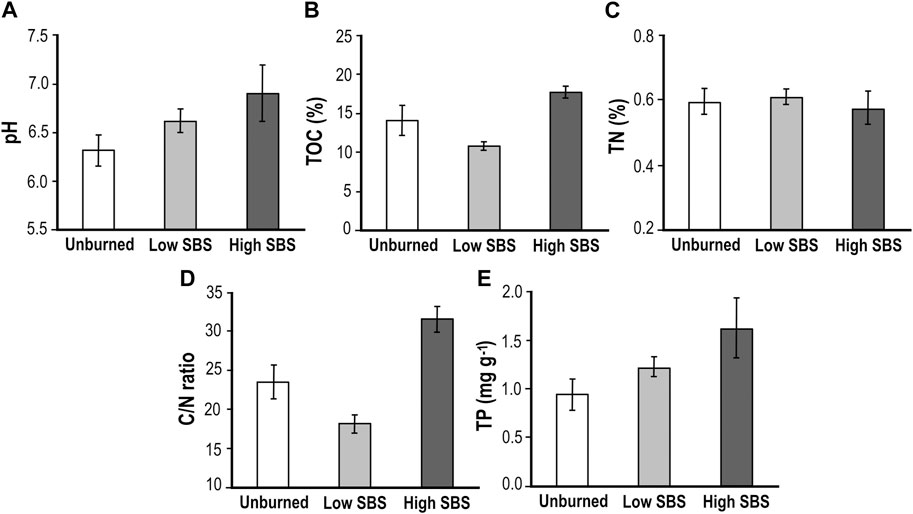
FIGURE 5. Mean values and standard errors of chemical properties of the soil samples collected from uppermost soil layer, before (unburned) and after the prescribed burn (low and high SBS). Low SBS correspond to levels 1 and 2 (n = 6), and high SBS to the levels 4 and 5 (n = 2). (A) pH; (B) TOC; (C) TN; (D) C/N ratio; (E) TP.
Morphometric Analysis in Soils Affected by Different SBS
Images from thin sections with the organic layer and the upper 5 cm mineral soil from unburned and burned samples were morphometrically analyzed (Table 2; Figure 6). As logical, the lowest percentage of charred features was found in soils unaffected by fire (Figure 6A). All the scanned thin sections from the burn samples exhibited a darker layer on top (Figure 6). Samples with SBS level 1 showed the lowest number of unburned elements (e.g., aggregates, seeds, and plant tissues), coinciding with the highest porosity (46.3%, Table 2; Figure 6B) and followed by samples from SBS level 2 (37.5%, Figure 6D); the lowest porosity was found in the soil with SBS level 5 (14.5%). On the other hand, the morphometric analysis demonstrated that the highest number of elements with evidence of burning (e.g., charred and reddened aggregates, burned plant remains, and charcoal) was related to level 5 of the SBS index, reaching 50% of the considered area (Figure 6E; Table 2). Although level 4 showed fewer percentages of burning elements, only here mineral ash was detected (Figure 6E; Table 2).
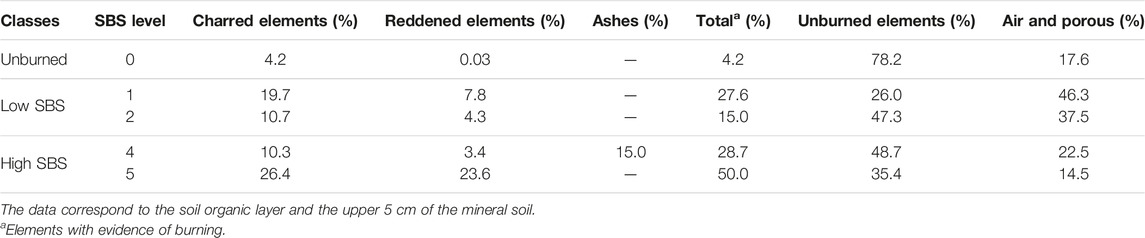
TABLE 2. Soil morphometry results for the unburned and different SBS levels samples, showing the percentages related to the unburned and burned elements in a representative area (6 cm2) of each thin section.
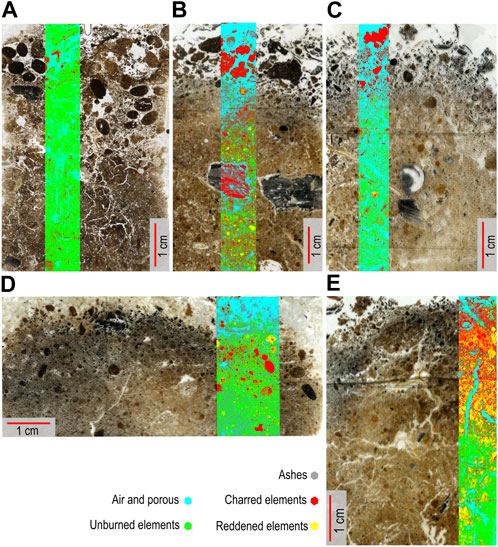
FIGURE 6. Soil thin sections from the soil organic layer and the upper 5 cm of the mineral soil with the selected area used for the morphometry analysis: (A) Unburned topsoil with brown aggregates and fresh plant remains; (B) Sample at low SBS 1, burned aggregates at the surface soil; (C) Sample at low SBS 2, completely charred topsoil; (D) Sample at high SBS 4, ashes covering the burned soil. (E) Sample at high SBS 5, absent organic layer and the mineral soil with charred and reddened aggregates.
In general, we identified a loss of structure and a reduction of the number of unburned elements and porosity with the increasing SBS. Additionally, ashes percentages and reddened aggregates were more significant in the highest SBS levels (4 and 5, respectively, Table 2). The morphometric data showed that the prescribed burn affected the soil organic layer and the first centimeter of the mineral soil for low SBS levels (Figures 6B, C) and the first 2 cm for high SBS levels (Figures 6D, E). The main proportion of burned remains corresponded to charred elements from the organic layer and the upper Ah horizon (Figure 6). It is important to mention that this examination might be including burning residues from previous fire events preserved in the soil. However, this previous evidence was discriminated by micromorphology analysis.
Soil Micromorphological Changes Due to the Prescribed Burn
The following descriptions will relate to the main micromorphological characteristics of unburned and burned samples from the organic layer, the top 5 cm of the mineral soil, and the burned features associated with each SBS level.
In the organic layer of unburned samples, fresh plant and animal residues, such as wood fragments (Figure 7A), seeds (Figure 7B), and shells (Figure 7C), were frequent and could be distinguished. The mineral topsoil showed an organic soil structure (sub-rounded aggregates pigmented by organic matter) (Figure 7B). Although these thin sections belong to unburned soils, a few rounded charcoal fragments were identified, possibly from previous fire events. A zoogenic structure (dark rounded peds) integrated by coprolites was also common (Figure 7D). Brown subangular aggregates were frequent from a depth of 3 cm and onward (Figure 7C).
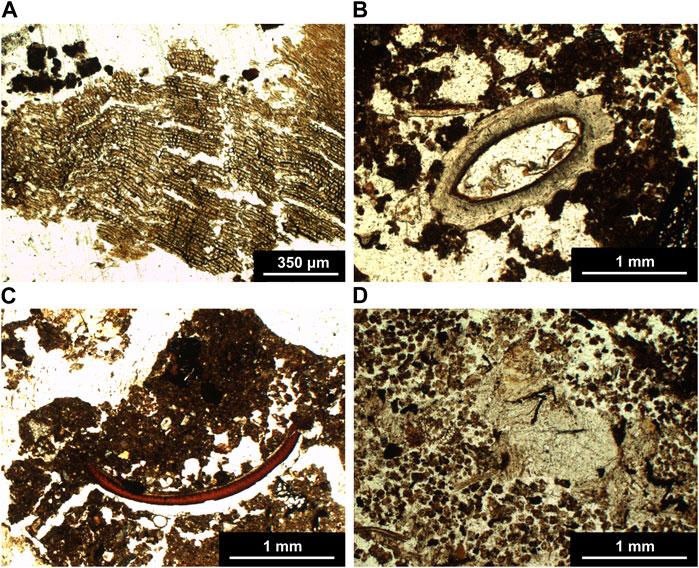
FIGURE 7. Micromorphology characteristics from the unburned organic layer and unburned mineral soil: (A) fresh wood; (B) seed into the organic soil structure; (C) fresh shell with subangular soil aggregates; (D) rounded coprolites and vegetal remains.
At this microscopic scale, samples from low SBS level 1 areas showed an organic layer with partially charred plant tissues and some burned vegetal remains, such as charred wood, roots, and spikes. The mineral soil exhibited an organic structure, where some aggregates get darker after burning in all samples affected by this low SBS level (Figures 8A–D).
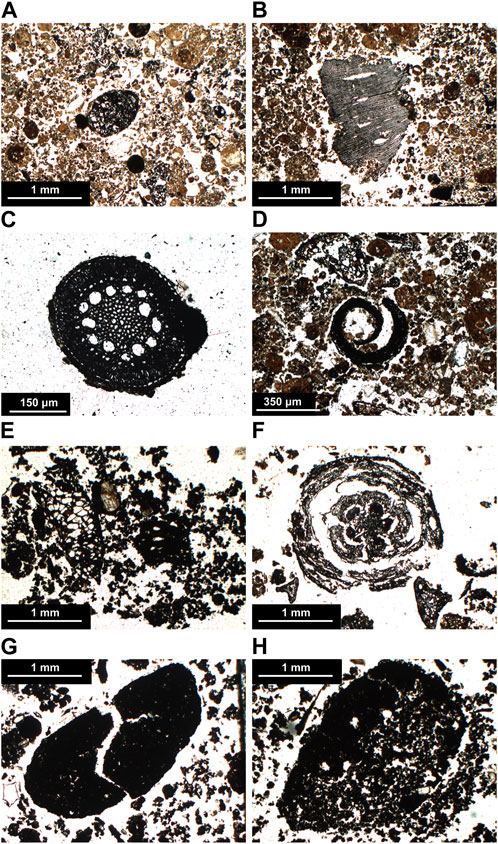
FIGURE 8. Micromorphological combustion features of burned soils at low SBS levels 1 and 2: (A) charred aggregates and charcoal mixed with no burned aggregates, SBS 1; (B) charred wood, SBS 1; (C) charred root, SBS 1; (D) charred spike and needles in soil organic matter, SBS 1; (E) charred topsoil and charcoal, SBS 2; (F) charred pine stem, SBS 2; (G) charred and fractured aggregate, SBS 2; (H) charred partially disintegrated aggregate and soil structure disaggregation by fire, SBS 2.
In the thin sections that showed a low SBS level 2, the prescribed burning heat induced a color change in the first centimeters of the soil, commonly covered by a black organic layer of 1 cm thick (Figure 6C). This layer comprised charcoal fragments from the charred organic layer remains, such as seeds, leaves, needles, stems, roots, and spikes in all samples (Figures 8E–H). These charred organic materials were frequently complete and preserved their cellular structure (Figure 8F). The surface soil aggregates for SBS level 2 was charred, fractured, and partially or totally disaggregated; a loss in the soil structure was also identified (Figures 8G, H, 9B–D).
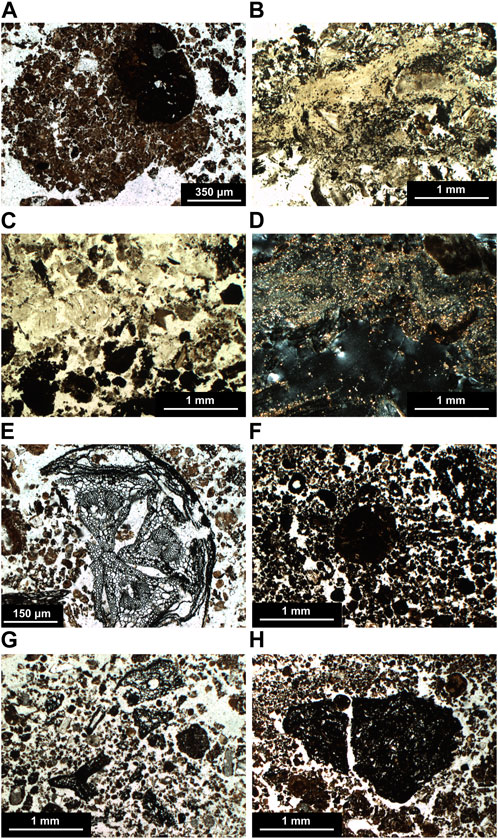
FIGURE 9. Micromorphological combustion features of burned soils at high SBS levels 4 and 5; (A) reddened and rounded aggregate, SBS 4; (B,C) ashes in PPL, SBS 4; (D) ashes in XPL, SBS 4. PPL and XPL refer to plane and cross polarized light, respectively; (E) charred needles with its cellular structure well preserved, SBS 5; (F,G) charred aggregates and disaggregated soil structure, SBS 5; (H) charred and cracked aggregate, SBS 5.
The organic materials were reduced to ash in the samples showing SBS level 4, (gray material in Figure 6D, yellowish material associated with charcoal in Figures 9B, C and small shiny crystals in Figure 9D). Some ash particles fill the pores of the surface soil (Figures 6E, 9C, D). At this level, the combustion process of the organic layer was completed. In the mineral soil, the organic matter in aggregates, fine roots, and seeds were charred or turned to ash; rounded reddened aggregates appeared (Figure 9A); the porosity decreased; and the surface soil lost its structure (Figure 6A). The organic layer was absent in the SBS level 5, while the soil organic matter was charred in the first centimeter (black aggregates) (Figure 6E, 9E–H).
Principal Components Analysis (PCA) of the Unburned and Burned Soils Data
In the PCA model, the first two principal components (PC) together explained 63% of the variance in the data (Figure 10A). The *L and pH are the variables that explain the most variance in PC1, while TOC and TN are in PC 2 (Figure 10B). On PC1, unburned samples are far from burned samples, mainly from those at SBS level 5 (Figure 10A). In contrast, on PC2 is possible to identify the overlaying by SBS level; in the center of the plot, the unburned samples are together to SBS level 1. The SBS 2 samples are in lower values related to an increment of TN concentration and bulk density (Figure 10), while SBS levels 4 and 5 group are in higher values related to the increment of TOC concentration and reddened elements (Figure 10).
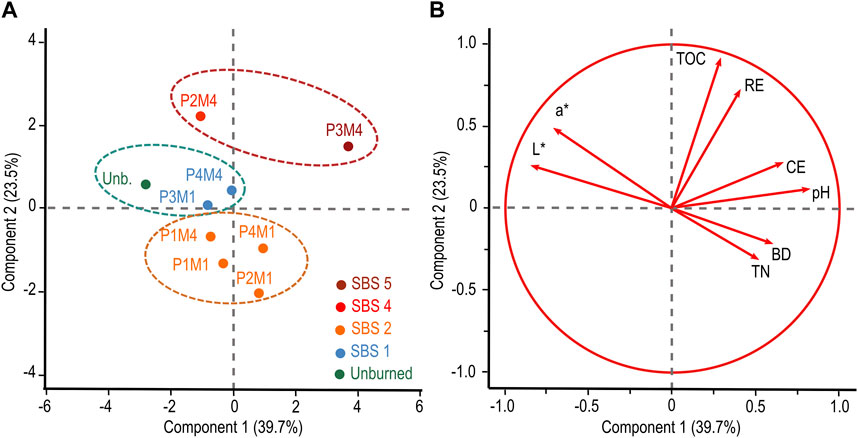
FIGURE 10. (A) Principal components analysis for the unburned (average) and the burned samples before and after the prescribed burn, respectively; the soil burn severity level of the samples are represented in different colors. (B) The right plot showed the correlation between soil variables, considered for the analysis, and the principal components. TOC, total organic carbon; RE, reddened elements; CE, charred elements; BD, bulk density; TN, total nitrogen. L*: luminosity, a*: redness to greenness.
Discussion
Changes in Soil Physical and Chemical Properties and Their Relationship With Micro-Scale Analyses
The effect of fire on soil physical-chemical properties has been described in several studies. Soil organic layer amount and composition can be affected (Nave et al., 2011; Merino et al., 2015; Santín et al., 2016). With respect to mineral soil, important changes in morphological, physical, and chemical properties can happen (Neary et al., 1999; Mataix-Solera et al., 2011), particularly in the uppermost 5 cm, where the highest temperature is reached (DeBano et al., 1979; Neary et al., 1999; Mataix-Solera et al., 2011; Badía et al., 2017). All these perturbations depend on the heating and are reflected as SBS levels. In the case of low-intensity prescribed burns, the heating caused minimal adverse changes in mineral soils (Debano, 2000; Mataix-Solera et al., 2011). The present study’s PCA analysis separates the unburned from the burned samples by burn severity (Figure 10A), which agrees with the findings of Vega et al. (2013). The burned samples generally showed a darker color (L* value decrease), higher bulk density, higher pH values, and a higher percentage of charred and reddened elements than the unburned samples. Mainly, these properties explained 63% of the variance in the PCA analysis (Figure 10A). In addition, we observed modifications in other soil properties, such as moisture, TOC, TN, and TP.
Changes in the mean values of the chromatic parameters (L*, a*, and b*) indicate a darkening and a decrease in the redness and yellowness colors in the burned bulk samples (Figure 4). These results coincide with those obtained by Badía and Martí (2003a), where both value and chroma decrease as temperature increases, suggesting a darkening of the soil color of the charred samples. On the contrary, in the experiment conducted by Cancelo-González et al. (2014), the color parameters increased with the temperature rise in soils with different moisture contents (0%, 25%, and 50%). On the one hand, our unburned samples showed a mean value of soil moisture around 27%, and after the prescribed burn, a slight reduction of moisture was detected due to the water loss by vaporization in the uppermost 5 cm (Badía et al., 2017). On the other hand, Cancelo-González et al. (2014) experiment was done in soils derived from granitic rocks, whose mineralogy differs from that of our study (intermediate extrusive igneous rocks). Another difference was that we evaluated the color in sieved and homogenized samples, while Cancelo-González et al. (2014) measured the post-fire color on the undisturbed surface, which proved to be the best way for the evaluation. Nevertheless, the decrease in the L* parameter coincided with the morphometric and micromorphological analyses of the burn soils, where a dark black layer on the topsoils was identified. The darker color measured on burn samples with the colorimetric method corresponded to the abundant charred elements from the organic layer and the upper Ah horizon. Ketterings and Bigham. (2000) showed that soil became darker after fires at low temperatures (<250°C) of short duration because charred organic materials are dominant in the soil. Also, Cancelo-González et al. (2014) showed that organic matter content, temperature, and fire duration affected the color of burn soils. Concerning the reddened elements identified by micro-scale analyses of the samples with high SBS (Figure 9A), the colorimetric measurements did not detect them because this reddening was punctual, and the colorimeter performed an average of soil bulk samples color.
The observed increase of bulk density with heating (Figure 5) coincides with several studies where prescribed burn has been applied, independently of the kind of vegetation, brushes (Hubbert et al., 2006) or oak forest (Phillips et al., 2000), and for different soils heated under laboratory conditions (Badía and Martí, 2003a). Morphometric and micromorphological results showed the collapse of soil aggregates and the ash presence into the voids (Figures 9G, H), which can be other reasons to elevate the bulk density in the Ahuacapán Ejido. Likewise, Badía and Martí. (2003a) also found a moderate diminution of aggregate stability at 250°C, and Agbeshie et al. (2022) exhibited the breaking of organo-mineral aggregates and the clogging of porous by the ash-like causes of the bulk density increase. Moreover, this increase would indicate soil organic matter composition changes affecting the soil structure. Different studies have revealed carbohydrate decreases with heating, implying lower aggregate stability (Gregorich et al., 1996; Kavdir et al., 2005; Merino et al., 2018). Another process involved in structure stability is the loss of binders, such as fine roots, hyphae, and microorganisms (Mataix-Solera et al., 2011). Additionally, decreases in biological properties in the soil surface after controlled burn have been reported both in the lab (Badía and Martí, 2003b) and in the field (Armas-Herrera et al., 2018; Girona-García et al., 2018; Alfaro-Leranoz et al., 2023).
We observed a decrease in TOC in the low SBS samples but an increase in the soils with a high-level SBS (Figure 5). The critical consumption of organic carbon starts between 200ºC and 250°C and is completed approximately at 460°C (Giovannini et al., 1988; Vega et al., 2013); however, when the temperature is higher than 500°C, a total loss of organic matter occurs (Terefe et al., 2008). Likewise, Badía and Martí. (2003a) found a coefficient of correlation of R = −0.84 (p < 0.01) between the SOM and heat, due to a significant decrease of the organic matter with temperature increase at 250°C and almost the completed consumption at 500°C. Therefore, no conclusive trend could be distinguished with the TOC, probably because of mixed effects: the unfinished combustion process of the organic matter and the integration of charred materials into the first centimeter of the mineral soil (Soto and Díaz-Fierros, 1993; Úbeda et al., 2005; Afif and Oliveira, 2006; Scharenbroch et al., 2012). Thus, it is possible that the light increases in TOC in the area showing high SBS were due to the presence of charcoal, as the high C/N ratios suggest it. This is supported by the important percentage of charred organic fragments integrated into the mineral topsoil detected in thin sections from the high SBS (Figures 6D, E).
Regarding another chemical property, the slight rise in pH registered after the prescribed burn is described by the whole oxidation of organic matter producing ash with high Ca, Mg, K, and carbonate content (Ulery et al., 1993; Arocena and Opio, 2003; Certini, 2005), as well as by the formation of Fe-hydroxides (Schwertmann and Fisher, 1973). Under the microscope, calcite crystals were detected in high-level SBS (Figures 9F–H). Higher pH values in burned samples are often reported (e.g., Alcañiz et al., 2018).
Little change in TN concentrations was noted after the prescribed burn (Figure 5). Similarly, Arocena and Opio. (2003) found no important modifications in the TN; the authors considered that TN loss was mainly restricted to the forest floor, or TN losses were compensated by atmospheric N deposition. However, this last possibility can be considered in the long term. Another explanation for this slight change in TN is that the maximum temperature on the soil surface of the prescribed burn was not enough to allow the organic nitrogen volatilization from the soil (Neary et al., 1999), and only a minor portion of the nitrogen was thermally mineralized and integrated into the soil from the forest floor as NH4+ (Dannenmann et al., 2011; Bird et al., 2015). On the other hand, Badía and Martí. (2003a) found a significant (R = −0.70; p < 0.01) reduction of TN at 500°C for two different soils under laboratory conditions. According to a review of several studies (Alcañiz et al., 2018), the behavior of TN after burning remains unclear. Due to the insignificant TN variations, the mean values of the C/N ratio showed the same trend as TOC concentration, with a reduction of the C/N ratio from the unburned to the burned samples at low SBS and an increment for the burned samples at high SBS.
The increase in the TP content after the prescribed burn (Figure 5) coincides with the results reported by other studies (Wienhold and Klemmedson, 1992; Úbeda et al., 2005; Afif and Oliveira, 2006; Merino et al., 2018). Besides, the available P (Olsen extractable P) showed a significant increase by heating (R = 0.48; p = 0.01) in lab conditions (Badía and Martí, 2003a). This TP enrichment is mainly favored by the mineralization of organic matter (García-Oliva et al., 2018), together with the increase in pH, due to the liberation of basic cations during the organic matter combustion and the ash production (Ulery et al., 1993; Kennard and Gholz, 2001; Arocena and Opio, 2003; Certini, 2005).
Soil Burn Severity Index and Soil Micro-Scale Changes Due to Prescribed Burn
As mentioned before, one of the properties affected by fire is the soil structure. However, changes are not completely visible to the naked eye; consequently, the different SBS degrees are not identified. Micromorphological characterization is a complementary tool to evaluate the effect of the prescribed burns, as shown by Badía et al. (2020). The classification proposed by Vega et al. (2013) considers a macromorphological analysis, where the SBS 1 is associated with partial changes of the organic layer, while the mineral horizon remains unaltered. Our microscopic observations document some charred elements and burned features in the organic and mineral horizons (charred aggregates, partially charred plant tissues, burned roots, wood, and spikes). Although part of these charred materials can be formed during the present burn, it is important to notice that some features might also originated in previous fires (Figure 6B), as they were also identified in the unburned samples (Figure 6A). However, charcoals from prior fire events are easier to identify with micromorphological methods because they are more rounded than the fresh ones due to mechanical breaking during time (Ponomarenko, 1997; Ponomarenko and Anderson, 2001). Hobley et al. (2017) documented that charcoal fragments are quickly integrated into the soil profile after a fire event and can persist in the soil environment for a long time. In many archaeological sites, burnt features, identified at micro-scale, are well preserved, providing information about human impact on the ecosystem (Mallol et al., 2007; Huisman et al., 2019).
Similarly, in the SBS 2 Vega et al. (2013) index, the mineral soil is also undisturbed. However, the thin sections showed that the soil was covered by a 1 cm-thick black organic layer (Figures 3B, 6C). Under the microscope, we observed a loss of the structure while charred aggregates are fractured and partially or completed disaggregated, in the first centimeter of the mineral soil (Figures 8E–G). This observation might explain the increase of the soil bulk density (Figure 4E). Greene et al. (1990) showed that frequent prescribed burnings can break down aggregates surface. These results have been also confirmed by Arocena and Opio. (2003), who demonstrated the presence of cracks on soil aggregates through SEM-EDS observations. They interpreted these cracks as a thermal shock related to the temperature growth. Other studies, however, have not found immediate effects on mineral topsoil aggregates after the low-intensity sand rapid prescribed burn in shrubland in Central Pyrenees, Spain (Badía et al., 2020). In agreement to our findings, DeBano et al. (1998) and Mataix-Solera et al. (2011) mentioned that even low-intensity fires can affect soil structure and aggregate stability due to the combustion and/or transformation of the organic matter compounds that binds soil aggregates; this generates a loss of soil structure, a porosity reduction, and a bulk density increase (DeBano, 1981). Moreover, Badía et al. (2017) revealed that controlled burning only affects the physical and chemical properties of the first centimeter of dry soils. Another explanation for the loss of aggregate stability after prescribed burnings, without significant loss of organic matter, was exposed by Albalasmeh et al. (2013) and Chief et al. (2012), who found that steam pressure can break down aggregates at temperatures of 150ºC and 175°C in soils with high initial moisture. Nevertheless, it is important to mention when the fire temperature rises, an increment in the ped stability is noted because of the low contents of clay and Fe oxides in the soils (Thomaz, 2021). With these findings, we can complement the SBS Vega et al. (2013) index adding a slight perturbation of the first centimeter of the mineral soil at level 2.
For the SBS level 4, the micromorphological and morphometric descriptions agree with the general properties proposed by Vega et al. (2013), where the organic layer was completely consumed and transformed into a thick ash layer. The fire also affected the uppermost mineral soil structure and the surface fine roots and seeds (Figures 3C, 6D, 9A–D). The ash layer resulted from the oxidation of vegetal materials beyond charring during the fire, where the calcium oxalate was transformed into calcium carbonate by loss of TOC (Canti and Brochier, 2017). Calcite crystals were identified in the thin section of the SBS level 4 (Figure 9D). Reddened aggregates were also detected under the microscope. However, they were not recognized in the SBS index of Vega et al. (2013) because it is hard to identify them at a macroscopic scale. Our micro-scale study demonstrated that these mineralogical transformations expressed by reddened aggregates could start even before SBS level 5.
Regarding the Vega et al. (2013) index for the SBS 5, the micro-scale analyses showed that the soil organic layer was absent because it was completely consumed (Figure 6E). This characteristic can result from a higher temperature during the prescribed burn in this specific zone. The forest floor may have reached a temperature of around 460°C, in which the organic carbon consumption is completed (Giovannini et al., 1988), or the organic layer was absent in this area before de fire. In our study, the micromorphological and morphometric results showed that the aggregates and soil structure were slightly affected in the first 2 cm of the mineral soil (Figure 6E). Additionally, soil organic matter was charred and some aggregates were reddened in the surface soil (Figure 6E). The incipient soil reddening is a consequence of Fe oxides transformation due to heating; however, a continuous reddened layer covered by ash is expected only for severe fires and for SBS level 5 (Wells et al., 1979; Ulery and Graham, 1993; Ketterings and Bigham, 2000; Vega et al., 2013).
Conclusion
The physical-chemical characteristics of burned samples showed clear differences with unburned samples. After the prescribed burn, soils exhibited a darker color with a decrease in redness and yellowness, a slight reduction in soil moisture, an increase in bulk density, higher pH values, an inconclusive trend for TOC content, insignificant variation of TN, and an increase in TP content. Besides these changes in the physical and chemical properties, already well documented in other studies, micromorphological and morphometrical characteristics are useful techniques for revealing how deep the effect of prescribed burning reached into the soil and for complementing the diagnosis of post-fire soil physical alterations that SBS assessment cannot identify due to the evaluation scale.
Some of our results agree with to the SBS Vega et al. (2013) classification, particularly for the organic layer. However, the microscale analyses of mineral soil revealed that even from the SBS 2, the first centimeter of soil structure was affected by a prescribed burn. This might be attributed to changes in soil organic matter content and composition and to the thermal shock and steam pressure suffered by the aggregates due to temperature increase. Despite these features, some living seeds and fine roots could be distinguished, indicating a potential for plant and microorganism regeneration. Additionally, the Fe oxides transformations that reddened soil aggregates can start even before the soil gets the SBS 5. For high SBS levels, the micro-scale analyses revealed that the prescribed burn affected the first 2 cm of soil depth, disrupting soil structure, porous clogging with ash, and the reddening of soil aggregates. These effects resulted in higher bulk density and changes in surface soil porosity.
Land managers must consider these soil alterations at low and high SBS after applying prescribed burns to avoid post-fire erosion of the first-centimeters mineral soil. The actions to protect the soil must be applied following the prescribed burn and before the first rain, especially in the bare soil areas where aggregates disrupted by the fire can be susceptible to dispersion and erosion by the rain splash and runoff. Therefore, the SBS assessment can include an additional section about the microscopic characteristics of burn soils. Likewise, a micro-scale characterization of different SBS levels for wildfires could be interesting because it can reveal soil perturbation that we cannot see directly on the field.
Data Availability Statement
The raw data supporting the conclusion of this article will be made available by the authors, without undue reservation.
Author Contributions
BC-V conceived the general idea and led the project. SQ-G and BC-V designed the sampling and took the samples. BC-V and MR-R performed the soil physicochemical analysis. MR-R prepare the thin sections. SM-R carried out the morphometric and micromorphological examination. SM-R and BC-V performed the statistical analysis. ES-R supervised the project. AM, BC-V, and ES-R contributed to the analysis of the results and the discussion section. SM-R wrote the manuscript with support of BC-V, ES-R, AM, and SQ-G. All authors contributed to the article and approved the submitted version.
Conflict of Interest
The authors declare that the research was conducted in the absence of any commercial or financial relationships that could be construed as a potential conflict of interest.
Acknowledgments
We appreciate the financial support provided by the projects: UDG-PTC-1356, PAPIIT—UNAM (IA209716) and PAPIIT—UNAM (IN103617). We thank to the Ahuacapán Ejido, MSc. Rodrigo Velázquez, Miss Faviola Castillo, MSc. Ofelia Beltrán Paz, MSc. Jaime Díaz, Dr. Sergey Sedov, Dr. Yazmín Rivera, MSc. Oscar Balcazar, Eng. Erick López and Ms. Silvia Martínez for the support provided in the different stages of this research. We also appreciate the contributions of the reviewer to improve the manuscript.
References
Afif, E., and Oliveira, J. A. (2006). Efectos del Fuego Prescrito Sobre el Matorral en las Propiedades del Suelo. Investigaciones Agrarias. Sist. Recur. For. 15 (3), 262–270.
Agbeshie, A. A., Abugre, S., Atta-Darkwa, T., and Awuah, R. (2022). A Review of the Effects of Forest Fire on Soil Properties. J. For. Res. 33, 1419–1441. doi:10.1007/s11676-022-01475-4
Albalasmeh, A. A., Berli, M., Shafer, D. S., and Ghezzehei, T. A. (2013). Degradation of Moist Soil Aggregates by Rapid Temperature Rise Under Low Intensity Fire. Plant Soil 362, 335–344. doi:10.1007/s11104-012-1408-z
Alcañiz, M., Outeiro, L., Francos, M., and Úbeda, X. (2018). Effects of Prescribed Fires on Soil Properties: A Review. Sci. Total Environ. 613–614, 944–957. doi:10.1016/j.scitotenv.2017.09.144
Alfaro-Leranoz, A., Badia-Villas, D., Marti-Dalmau, C., Emran, M., Conte-Dominguez, A. P., and Ortiz-Perpiña, O. (2023). Long-Term Evolution of Shrub Prescribed Burning Effects on Topsoil Organic Matter and Biological Activity in the Central Pyrenees (NE-Spain). Sci. Total Environ. 888, 163994. doi:10.1016/j.scitotenv.2023.163994
Alperson-Afil, N. (2012). Archaeology of Fire: Methodological Aspects of Reconstructing Fire History of Prehistoric Archaeological Sites. Earth-Science Rev. 113, 111–119. doi:10.1016/j.earscirev.2012.03.012
Armas-Herrera, C. M., Martí, C., Badía, D., Ortiz-Perpiñá, O., Girona-García, A., and Mora, J. L. (2018). Short-Term and Midterm Evolution of Topsoil Organic Matter and Biological Properties After Prescribed Burning for Pasture Recovery (Tella, Central Pyrenees, Spain). Land Degrad. Dev. 29, 1545–1554. doi:10.1002/ldr.2937
Armas-Herrera, C. M., Martí, C., Badía, D., Ortiz-Perpiñá, O., Girona-García, A., and Porta, J. (2016). Immediate Effects of Prescribed Burning in the Central Pyrenees on the Amount and Stability of Topsoil Organic Matter. CATENA 147, 238–244. doi:10.1016/j.catena.2016.07.016
Arocena, J. M., and Opio, C. (2003). Prescribed Fire-Induced Changes in Properties of Sub-Boreal Forest Soils. Geoderma 113, 1–16. doi:10.1016/S0016-7061(02)00312-9
Badía, D., Esteban, J., Girona, A., Ortiz, O., and Poch, R. M. (2020). Topsoil Microstructure Changes After a Shrubland Prescribed Burn (Central Pyrenees, NE Spain). Sci. Total Environ. 748, 141253. doi:10.1016/j.scitotenv.2020.141253
Badía, D., López-García, S., Martí, C., Ortíz-Perpiñá, O., Girona-García, A., and Casanova-Gascón, J. (2017). Burn Effects on Soil Properties Associated to Heat Transfer Under Contrasting Moisture Content. Sci. Total Environ 601–602, 1119–1128. doi:10.1016/j.scitotenv.2017.05.254
Badía, D., and Martí, C. (2003b). Effect of Simulated Fire on Organic Matter and Selected Microbiological Properties of Two Contrasting Soils. Arid Land Res. Manag. 17, 55–69. doi:10.1080/15324980301594
Badía, D., and Martí, C. (2003a). Plant Ash and Heat Intensity Effects on Chemical and Physical Properties of Two Contrasting Soils. Arid Land Res. Manag. 17, 23–41. doi:10.1080/15324980301595
Berna, F., Goldberg, P., Horwitz, L. K., Brink, J., Holt, S., Bamford, M., et al. (2012). Microstratigraphic Evidence of In Situ Fire in the Acheulean Strata of Wonderwerk Cave, Northern Cape Province, South Africa. Proc. Natl. Acad. Sci. U.S.A. 109, E1215–E1220. doi:10.1073/pnas.1117620109
Bird, M. I., Wynn, J. G., Saiz, G., Wurster, C. M., and McBeath, A. (2015). The Pyrogenic Carbon Cycle. Annu. Rev. Earth Planet. Sci. 43, 273–298. doi:10.1146/annurev-earth-060614-105038
Bremner, J. M. (1996). “Nitrogen-Total,” in Methods of Soil Analysis (Madison, Wisconsin: Soil Science Society of America, Inc. and American Society of Agronomy, Inc.), 1085–1121. doi:10.2136/sssabookser5.3.c37
Cancelo-González, J., Cachaldora, C., Díaz-Fierros, F., and Prieto, B. (2014). Colourimetric Variations in Burnt Granitic Forest Soils in Relation to Fire Severity. Ecol. Indic. 46, 92–100. doi:10.1016/j.ecolind.2014.05.037
Canti, M. G., and Brochier, J. É. (2017). “Plant Ash,” in Archaeological Soil and Sediment Micromorphology. Editors C. Nicasio, and G. Stoops (Hoboken, NJ: John Wiley & Sons, Ltd), 147–154. doi:10.1002/9781118941065.ch17
Cerano-Paredes, J., Villanueva-Díaz, J., Cervantes-Martínez, R., Fulé, P., Yocom, L., Esquivel-Arriaga, G., et al. (2015). Historia de Incendios en un Bosque de Pino de la Sierra de Manantlán, Jalisco, México. Bosque (Valdivia) 36, 41–52. doi:10.4067/S0717-92002015000100005
Certini, G. (2005). Effects of Fire on Properties of Forest Soils: A Review. Oecologia 143, 1–10. doi:10.1007/s00442-004-1788-8
Chief, K., Young, M. H., and Shafer, D. S. (2012). Changes in Soil Structure and Hydraulic Properties in a Wooded-Shrubland Ecosystem Following a Prescribed Fire. Soil Sci. Soc. Am. J. 76, 1965–1977. doi:10.2136/sssaj2011.0072
Dannenmann, M., Willibald, G., Sippel, S., and Butterbach-Bahl, K. (2011). Nitrogen Dynamics at Undisturbed and Burned Mediterranean Shrublands of Salento Peninsula, Southern Italy. Plant Soil 343, 5–15. doi:10.1007/s11104-010-0541-9
DeBano, L. F., Eberlein, G. E., and Dunn, P. H. (1979). Effects of Burning on Chaparral Soils: I. Soil Nitrogen. Soil Sci. Soc. Am. J. 43, 504–509. doi:10.2136/sssaj1979.03615995004300030015x
DeBano, L. F., Neary, D. G., and Folliott, P. F. (1998). Fire’s Effects on Ecosystems. New York: J. Wiley.
DeBano, L. F. (2000). The Role of Fire and Soil Heating on Water Repellency in Wildland Environments: A Review. J. Hydrology 231–232, 195–206. doi:10.1016/S0022-1694(00)00194-3
DeBano, L. F. (1981). Water Repellent Soils: A State-Of-The-Art (No. PSW-GTR-46). Berkeley, CA: U.S. Department of Agriculture, Forest Service, Pacific Southwest Forest and Range Experiment Station. doi:10.2737/PSW-GTR-46
Fernandes, P. M., Davies, G. M., Ascoli, D., Fernández, C., Moreira, F., Rigolot, E., et al. (2013). Prescribed Burning in Southern Europe: Developing Fire Management in a Dynamic Landscape. Front. Ecol. Environ. 11. doi:10.1890/120298
Flores-Delgadillo, L., and Alcalá-Martínez, J. R. (2010). Manual de Procedimientos Analíticos. Mexico City: Instituto de Geología, Universidad Nacional Autónoma de México.
Gregorich, E. G., Monreal, C. M., Schnitzer, M., and Schulten, H. R. (1996). Transformation of Plant Residues Into Soil Organic Matter: Chemical Characterization of Plant Tissue, Isolated Soil Fractions, and Whole Soils. Soil Sci. 161, 680–693. doi:10.1097/00010694-199610000-00005
García-Oliva, F., Merino, A., Fonturbel, M. T., Omil, B., Fernández, C., and Vega, J. A. (2018). Severe Wildfire Hinders Renewal of Soil P Pools by Thermal Mineralization of Organic P in Forest Soil: Analysis by Sequential Extraction and 31P NMR Spectroscopy. Geoderma 309, 32–40. doi:10.1016/j.geoderma.2017.09.002
Giovannini, G., Lucchesi, S., and Giachetti, M. (1988). Effect of Heating on Some Physical and Chemical Parameters Related to Soil Aggregation and Erodibility. Soil Sci. 146, 255–261. doi:10.1097/00010694-198810000-00006
Girona-García, A., Badía-Villas, D., Martí-Dalmau, C., Ortiz-Perpiñá, O., Mora, J. L., and Armas-Herrera, C. M. (2018). Effects of Prescribed Fire for Pasture Management on Soil Organic Matter and Biological Properties: A 1-Year Study Case in the Central Pyrenees. Sci. Total Environ. 618, 1079–1087. doi:10.1016/j.scitotenv.2017.09.127
Girona-García, A., Ortiz-Perpiñá, O., and Badía-Villas, D. (2019). Dynamics of Topsoil Carbon Stocks After Prescribed Burning for Pasture Restoration in Shrublands of the Central Pyrenees (NE-Spain). J. Environ. Manag. 233, 695–705. doi:10.1016/j.jenvman.2018.12.057
Greene, R. S. B., Chartres, C. J., and Hodgkinson, K. C. (1990). The Effects of Fire on the Soil in a Degraded Semiarid Woodland.I. Cryptogam Cover and Physical and Micromorphological Properties. Soil Res. 28, 755. doi:10.1071/SR9900755
Hobley, E. U., Le Gay Brereton, A. J., and Wilson, B. (2017). Forest Burning Affects Quality and Quantity of Soil Organic Matter. Sci. Total Environ. 575, 41–49. doi:10.1016/j.scitotenv.2016.09.231
Hubbert, K. R., Preisler, H. K., Wohlgemuth, P. M., Graham, R. C., and Narog, M. G. (2006). Prescribed Burning Effects on Soil Physical Properties and Soil Water Repellency in a Steep Chaparral Watershed, Southern California, USA. Geoderma 130, 284–298. doi:10.1016/j.geoderma.2005.02.001
Huffman, E. W. D. (1977). Performance of a New Automatic Carbon Dioxide Coulometer. Microchem. J. 22, 567–573. doi:10.1016/0026-265X(77)90128-X
Huisman, D. J., Niekus, M. J. L. T., Peeters, J. H. M., Geerts, R. C. A., and Müller, A. (2019). Deciphering the Complexity of a ‘Simple’ Mesolithic Phenomenon: Indicators for Construction, Use and Taphonomy of Pit Hearths in Kampen (the Netherlands). J. Archaeol. Sci. 109, 104987. doi:10.1016/j.jas.2019.104987
Iverson, L., and Hutchinson, T. (2002). Soil Temperature and Moisture Fluctuations During and After Prescribed Fire in Mixed-Oak Forests, USA. Nat. Areas J. 22, 296–304.
Jardel, J. P., and Moreno, G. S. (2000). Programa de Manejo Forestal Para el Aprovechamiento del Ejido Ahuacapán, Municipio de Autlán, Jalisco. Autlán, Jalisco, México: IMECBIO- Universidad de Guadalajara/ECOFOR.
Kavdır, Y., Ekinci, H., Yüksel, O., and Mermut, A. R. (2005). Soil Aggregate Stability and 13C CP/MAS-NMR Assessment of Organic Matter in Soils Influenced by Forest Wildfires in Çanakkale, Turkey. Geoderma 129, 219–229. doi:10.1016/j.geoderma.2005.01.013
Kennard, D. K., and Gholz, H. L. (2001). Effects of High-Intensity Fires on Soil Properties and Plant Growth in a Bolivian Dry Forest. Plant Soil 234, 119–129. doi:10.1023/A:1010507414994
Ketterings, Q. M., and Bigham, J. M. (2000). Soil Color as an Indicator of Slash-And-Burn Fire Severity and Soil Fertility in Sumatra, Indonesia. Soil Sci. Soc. Am. J. 64, 1826–1833. doi:10.2136/sssaj2000.6451826x
Lucas-Borja, M. E., Miralles, I., Ortega, R., Plaza-Álvarez, P. A., Gonzalez-Romero, J., Sagra, J., et al. (2019). Immediate Fire-Induced Changes in Soil Microbial Community Composition in an Outdoor Experimental Controlled System. Sci. Total Environ. 696, 134033. doi:10.1016/j.scitotenv.2019.134033
Mallol, C., Marlowe, F. W., Wood, B. M., and Porter, C. C. (2007). Earth, Wind, and Fire: Ethnoarchaeological Signals of Hadza Fires. J. Archaeol. Sci. 34, 2035–2052. doi:10.1016/j.jas.2007.02.002
Mallol, C., Mentzer, S. M., and Miller, C. E. (2017). “Combustion Features,” in Archaeological Soil and Sediment Micromorphology. Editors C. Nicosia, and G. Stoops (Hoboken, NJ: John Wiley & Sons, Ltd), 299–330. doi:10.1002/9781118941065.ch31
Martínez, L. M., Sandoval, J. J., and Guevara, R. D. (1991). El Clima en la Reserva de la Biosfera Sierra de Manantlan (Jalisco-Colima, México) y en su área de Influencia. Agrociencia, Agua-Suelo-Clima 2, 1–14.
Mataix-Solera, J., Cerdà, A., Arcenegui, V., Jordán, A., and Zavala, L. M. (2011). Fire Effects on Soil Aggregation: A Review. Earth-Science Rev. 109, 44–60. doi:10.1016/j.earscirev.2011.08.002
Mataix-Solera, J., and Doerr, S. H. (2004). Hydrophobicity and Aggregate Stability in Calcareous Topsoils From Fire-Affected Pine Forests in Southeastern Spain. Geoderma 118, 77–88. doi:10.1016/S0016-7061(03)00185-X
Merino, A., Chávez-Vergara, B., Salgado, J., Fonturbel, M. T., García-Oliva, F., and Vega, J. A. (2015). Variability in the Composition of Charred Litter Generated by Wildfire in Different Ecosystems. CATENA 133, 52–63. doi:10.1016/j.catena.2015.04.016
Merino, A., Fonturbel, M. T., Fernández, C., Chávez-Vergara, B., García-Oliva, F., and Vega, J. A. (2018). Inferring Changes in Soil Organic Matter in Post-Wildfire Soil Burn Severity Levels in a Temperate Climate. Sci. Total Environ. 627, 622–632. doi:10.1016/j.scitotenv.2018.01.189
Merino, A., Jiménez, E., Fernández, C., Fontúrbel, M. T., Campo, J., and Vega, J. A. (2019). Soil Organic Matter and Phosphorus Dynamics After Low Intensity Prescribed Burning in Forests and Shrubland. J. Environ. Manag. 234, 214–225. doi:10.1016/j.jenvman.2018.12.055
Murphy, J., and Riley, J. P. (1962). A Modified Single Solution Method for the Determination of Phosphate in Natural Waters. Anal. Chim. Acta 27, 31–36. doi:10.1016/S0003-2670(00)88444-5
Nave, L. E., Vance, E. D., Swanston, C. W., and Curtis, P. S. (2011). Fire Effects on Temperate Forest Soil C and N Storage. Ecol. Appl. 21, 1189–1201. doi:10.1890/10-0660.1
Neary, D. G., Klopatek, C. C., DeBano, L. F., and Folliott, P. F. (1999). Fire Effects on Belowground Sustainability: A Review and Synthesis. For. Ecol. Manag. 122, 51–71. doi:10.1016/S0378-1127(99)00032-8
C. Nicosia, and G. Stoops (Editors) (2017). Archaeological Soil and Sediment Micromorphology (Hoboken, NJ: Wiley).
Pereira, J. S., Badía, D., Martí, C., Mora, J. L., and Donzeli, V. P. (2023). Fire Effects on Biochemical Properties of a Semiarid Pine Forest Topsoil at Cm-Scale. Pedobiologia 96, 150860. doi:10.1016/j.pedobi.2022.150860
Pérez-Salicrup, D. R. (2018). Caracterización y Clasificación de Combustibles Para Generar y Validar Modelos de Combustibles Forestales Para México (No. 251694). Mexico City: Fondo sectorial CONAFOR-CONACYT.
Phillips, D. H., Foss, J. E., Buckner, E. R., Evans, R. M., and FitzPatrick, E. A. (2000). Response of Surface Horizons in an Oak Forest to Prescribed Burning. Soil Sci. Soc. Am. J. 64, 754–760. doi:10.2136/sssaj2000.642754x
Ponomarenko, E. V., and Anderson, D. W. (2001). Importance of Charred Organic Matter in Black Chernozem Soils of Saskatchewan. Can. J. Soil. Sci. 81, 285–297. doi:10.4141/S00-075
Ponomarenko, E. V. (1997). Seasonality, Paleoenvironments and Stratigraphic Correlations of Archaeological Components in the Borden Dune Complex. Saskatoon, SK: Report on file with Western HeritageServices Inc.
Rubio, E. A. (2007). Frecuencia de Incendios Forestales en Bosques de Pinus Douglasiana del Ejido Ahuacapán, Jalisco, México. (Bachelor degree thesis). Jalisco, México: Departamento de Ecología y Recursos Naturales, Universidad de Guadalajara.
Ryan, K. C., and Noste, N. V. (1985). “Evaluating Prescribed Fires,” in Tech Coord Workshop of Wilderness Fire. Editors J. E. Lotan, B. M. Kilgore, W. C. Fischer, and R. W. Mutch (Missoula, Montana: U.S. Department of Agriculture (USDA)).
Santín, C., and Doerr, S. H. (2016). Fire Effects on Soils: The Human Dimension. Philosophical Trans. R. Soc. B Biol. Sci. 371, 20150171. doi:10.1098/rstb.2015.0171
Santín, C., Doerr, S. H., Merino, A., Bryant, R., and Loader, N. J. (2016). Forest Floor Chemical Transformations in a Boreal Forest Fire and Their Correlations With Temperature and Heating Duration. Geoderma 264, 71–80. doi:10.1016/j.geoderma.2015.09.021
Scharenbroch, B. C., Nix, B., Jacobs, K. A., and Bowles, M. L. (2012). Two Decades of Low-Severity Prescribed Fire Increases Soil Nutrient Availability in a Midwestern, USA Oak (Quercus) Forest. Geoderma 183–184, 80–91. doi:10.1016/j.geoderma.2012.03.010
Schwertmann, U., and Fischer, W. R. (1973). Natural “Amorphous” Ferric Hydroxide. Geoderma 10, 237–247. doi:10.1016/0016-7061(73)90066-9
Soto, B., and Diaz-Fierros, F. (1993). Interactions Between Plant Ash Leachates and Soil. Int. J. Wildland Fire 3, 207–216. doi:10.1071/WF9930207
Stoops, G., Marcelino, V., and Mees, F. (2018). Interpretation of Micromorphological Features of Soils and Regoliths. 2nd ed. Amsterdam: Elsevier.
Terefe, T., Mariscal-Sancho, I., Peregrina, F., and Espejo, R. (2008). Influence of Heating on Various Properties of Six Mediterranean Soils. A Laboratory Study. Geoderma 143, 273–280. doi:10.1016/j.geoderma.2007.11.018
Thomaz, E. L. (2021). Effects of Fire on the Aggregate Stability of Clayey Soils: A Meta-Analysis. Earth-Science Rev. 221, 103802. doi:10.1016/j.earscirev.2021.103802
Úbeda, X., Lorca, M., Outeiro, L. R., Bernia, S., and Castellnou, M. (2005). Effects of Prescribed Fire on Soil Quality in Mediterranean Grassland (Prades Mountains, North-East Spain). Int. J. Wildland Fire 14, 379–384. doi:10.1071/WF05040
Ulery, A. L., Graham, R. C., and Amrhein, C. (1993). Wood-Ash Composition and Soil pH Following Intense Burning. Soil Sci. 156, 358–364. doi:10.1097/00010694-199311000-00008
Ulery, A. L., and Graham, R. C. (1993). Forest Fire Effects on Soil Color and Texture. Soil Sci. Soc. Am. J. 57, 135–140. doi:10.2136/sssaj1993.03615995005700010026x
Vega, J. A., Fontúrbel, T., Merino, A., Fernández, C., Ferreiro, A., and Jiménez, E. (2013). Testing the Ability of Visual Indicators of Soil Burn Severity to Reflect Changes in Soil Chemical and Microbial Properties in Pine Forests and Shrubland. Plant Soil 369, 73–91. doi:10.1007/s11104-012-1532-9
Wells, C. G., Campbell, R. E., DeBano, L. F., Lewis, C. E., Fredriksen, R. L., Franklin, E. C., et al. (1979). Effects of Fire on Soil: A State of Knowledge Review (General Technical Report No. WO-07). Washington, DC: U.S. Deparment of Agriculture Forest Service.
Wienhold, B. J., and Klemmedson, J. O. (1992). Effect of Prescribed Fire on Nitrogen and Phosphorus in Arizona Chaparral Soil-Plant Systems. Arid Soil Res. Rehabilitation 6, 285–296. doi:10.1080/15324989209381323
Keywords: prescribed burn, fire, soil burn severity, micromorphology, morphometry
Citation: Moreno-Roso S, Chávez-Vergara B, Solleiro-Rebolledo E, Quintero-Gradilla S, Merino A and Ruiz-Rojas M (2023) Soil Burn Severities Evaluation Using Micromorphology and Morphometry Traits After a Prescribed Burn in a Managed Forest. Span. J. Soil Sci. 13:11488. doi: 10.3389/sjss.2023.11488
Received: 18 April 2023; Accepted: 27 September 2023;
Published: 11 October 2023.
Edited by:
Irene Ortiz-Bernad, University of Granada, SpainCopyright © 2023 Moreno-Roso, Chávez-Vergara, Solleiro-Rebolledo, Quintero-Gradilla, Merino and Ruiz-Rojas. This is an open-access article distributed under the terms of the Creative Commons Attribution License (CC BY). The use, distribution or reproduction in other forums is permitted, provided the original author(s) and the copyright owner(s) are credited and that the original publication in this journal is cited, in accordance with accepted academic practice. No use, distribution or reproduction is permitted which does not comply with these terms.
*Correspondence: S. Moreno-Roso, c29sZGptb3Jlbm9AZ21haWwuY29t