- 1Laboratory of Digital Technologies and Modeling, Sarsen Amanzholov East Kazakhstan University, Ust-Kamenogorsk, Kazakhstan
- 2Department of Geoecology, Institute of Geosciences and Geography, Martin Luther University Halle-Wittenberg, Halle (Saale), Germany
- 3Big Data and Blockchain Technologies Research Innovation Center, Astana IT University, Astana, Kazakhstan
Temperate grasslands are called the breadbaskets of the world. Due to most continental climate conditions, humus-rich soils have been developed. These soils are very well suited for grain production. This is why extensive conversions from natural steppe to arable land have been implemented in this biome. The Kulunda Steppe, in Southwest Siberia and Central Asia, occupies large parts of the driest regions of the Eurasian Steppe Belt. It was one of the sites of the Virgin Land Campaign realized in the former Soviet Union in the 1950s and 1960s. Intensive agricultural practices have caused significant soil degradation, mainly through humus loss and soil erosion. This results in the degradation of organic carbon, altering the physical and chemical structure of the chestnut soils and impacting their water storage capacity. Against the background of climatic changes, a further intensification of these processes and conditions is to be expected. To stabilize soil carbon and optimize moisture utilization, it is necessary to extensively introduce worldwide experiences in conservation cropping technologies (such as no-till, min-till, and direct seeding) in the area. This study aimed to determine the effects of different cropping systems on soil water storage and water availability. The study’s initial hypothesis was that the soil conservational cropping system has advantages against the traditional deep tillage (24 cm). This hypothesis was based on extensive global experience studying the effects of different agricultural management systems on soil-water balance. In 2013–2016, an experiment was conducted for the first time in the Kulunda steppe to instrumentally measure soil moisture and matrix potential at 30–60–120 cm depth under traditional and conservation technology using innovative meteorological and soil hydrological stations. Statistically significant advantages of no-till over deep tillage (24 cm) in terms of moisture retention were found, confirming the hypothesis of this study. Besides, this groundbreaking study reveals new possibilities for soil monitoring in the region. The acquired data are applicable for predictive models using remote sensing. Moreover, the results on the management effects for the soil water balance provide basic approaches to soil water monitoring, offering important data for evaluating model results and remote sensing products for the region.
Introduction
Temperate grasslands are known as the breadbaskets of the world. They play an increasing role in sustainable grain production and global food security. Naturally, temperate grasslands are of global importance as carbon sinks. Due to land conversion and intensive agricultural management practices, the soils of this region are affected by severe degradation processes. Conventional, intensive management increases dehumidification and the risk of soil erosion by wind and water. This affects greenhouse gas emissions and, as a result, increases surface air temperature, with a worst-case scenario in 2047 (Mora et al., 2013; FAO, 2021). According to various estimates, agriculture accounts for 30%–35% of global greenhouse gas emissions (Foley et al., 2011; Shukla et al., 2019). These problems are particularly acute within drylands, where the growing season is less than 179 days due to water scarcity (FAO, 2000; Bodner et al., 2015). Of the total amount of degraded land in the world of 1,660 million ha (34% of all agricultural land), 733 million ha are in drylands (FAO, 2021). For the same regions, a high level of “water stress” from 35% to 70% (for comparison, the European average is 8.3%) is also observed (FAO, 2021).
Some authors estimate the world’s historical loss of soil organic carbon (SOC) due to agricultural activities and soil degradation at 133 billion tons (Lal, 2020). At the regional level, the catastrophic consequences of conversion of natural grassland to arable land on an area of 266 million ha (present-day arid regions and edges of Kazakhstan and Russia) occurred during the “virgin land campaign” in the USSR in 1954–1963, which resulted in the loss of 1.6 billion tonnes of SOC. The total loss of soil organic carbon from arable land in the Altai region from 1953 to 2003 from 143.8 million tonnes, with 36.6 million tonnes in the dry steppe zone (Kulunda plain) (Rolinski et al., 2021). Scenario modelling has shown that converting 266 million ha of arable land to fallow land by 2050 could sequester 1.8 billion tonnes of soil carbon (Rolinski et al., 2021).
Conversion of arable land to fallow land is a radical measure. Because of its spatial dimension, the Soviet Virgin Land Campaign is of global significance. Soil degradation and greenhouse gas emissions associated with conversion require solutions for adapted agricultural soil management and soil protection. One of the solutions to stabilize soils is the introduction of conservation agriculture (CA), which is opposed to traditional technologies (Conventional tillage—CT) (Smith, 2004; Blanco-Canqui and Ruis, 2018; Kassam et al., 2019; Wu et al., 2022). Primarily, this refers to “direct seeding” (No-Tillage hereinafter NT), synonymous with “zero-tillage” (Zero- Tillage hereinafter ZT), strip-till seeding (ST), rotational tillage (RT), and minimum tillage (Mini-Till, hereinafter MT) (Baig and Gamache, 2009; Soane et al., 2012; Kassam et al., 2019; Wang et al., 2022). The main focus of research for application of conservation agriculture are in two areas that complement each other: reducing greenhouse gas emissions and increasing water use efficiency (WUE). The studies of water use efficiency are focusing on soil water retention or soil water capacity.
A 10-year study on three tillage technologies (MT, NT, CT) and three fertilizer application rates in winter wheat (Triticum aestivum)—spring wheat (T. aestivum)—maize (Zéa máys) rotation at a Loess Plateau in China revealed that MT showed the highest yield and NT was more optimal in terms of soil moisture management (Zhang et al., 2018).
A field experiment was conducted on a Mollisol to determine the soil water retention curves (SWRC) dynamics of conventional tillage, no-till, and rotational tillage in the maize growing season of 2018 (Huang et al., 2021). The experiment was conducted in Lishu, China. The site has a moderate-temperate and sub-humid monsoon climate. The annual average air temperature is 5.9°C, and the annual mean precipitation is about 556 mm. The maize growing season at the study site is from April to September, with an average air temperature of 18°C and a mean precipitation of 470 mm. The high rigidity of the soil pore system and straw mulch on the soil surface in NT maintained a relatively higher soil water retention capacity. It reduced the temporal variation of SWRC in the maize growing season (Huang et al., 2021).
Changes of soil structure have been assessed by bulk density (BD), penetrometer resistance (PR), water retention curve, least limiting water range, and X-ray computed tomography under different tillage management (NT, CT) in Hebei Province, China (Tian et al., 2022). The average annual temperature and precipitation at the test area are 12.5°C and 494 mm, the texture of topsoil (0–20 cm) is silt loam, with 13.8% sand, 66.3% silt, and 19.9% clay. A crop rotation with Winter wheat (Triticum aestivum), followed by summer maize (Zea mays L.) was established. The experiment showed that NT retained more water, which may offset the higher PR caused by higher BD in the soil-wet condition (Tian et al., 2022).
Results of another field experiment near the town of Jevíčko, Czech Republic are published (Vlček et al., 2022). The average annual temperature at this site equals 8.4°C, and the yearly precipitation is 550–650 mm. The soil was classified as Chernozem. The applied management practices encompassed NT, RT, and CT methods. The plots where reduced tillage was applied were the most beneficial treatments for sustainable water management (Vlček et al., 2022).
Near Raasdorf east of Vienna, Austria, a further study was conducted in 2020 at a long-term field trial and a conventionally managed field (48°14′ N, 16°35′ E; 156 m elevation a.s.l.). The region is characterized by a flat topography and has an annual precipitation of 523 mm and an average temperature of 10.8°C (2000–2019) (Liebhard et al., 2022). The soil type in the experimental field and the conventionally managed field was classified as Chernozem. The yields in this agricultural region are generally restricted by water shortage and heat stress. Results from a field campaign with soybean at a long-term tillage experiment, including partitioning of evapotranspiration with stable isotope technique, show the best crop water productivity for low tillage intensity systems. For the given silt-loam soil and water-limited conditions, NT and RT have higher crop yields than CT. Crop water productivity is best for NT and worst for CT. Furthermore, compared to NT, CT and RT lose more water unproductively by evaporation. The temporal evolution of transpiration rates shows the tillage-induced differences in canopy development with time-delayed development under NT (Liebhard et al., 2022).
A meta-analysis of 147 publications on various (CA) in the world through 2021 found that MT increases N2O and CH4 emissions by 31.0% and 24.7%, respectively, and reduces yield by 17.4% without affecting CO2 emissions or CH4 uptake, while NT reduces CO2, N2O, and CH4 emissions by 15.1%, 7.5%, and 19.8%, on average, without affecting either CH4 uptake or yield (Yue K et al., 2023). A 2-year study in the context of carbon neutrality, water scarcity, and high fertilizer costs conducted in arid northwest China showed that in wheat (Triticum aestivum) cultivation, the minimum greenhouse gas emissions were observed with low irrigation and low nitrogen application rates, but at the expense of significant yield reduction (Kamran et al., 2023). A meta-analysis of publications on the Loess Plateau in China showed that factors that increase WUE ranked in the following order of importance: nitrogen fertilizer use, mulching, drip irrigation, crop residue return, and conservation tillage (Zhang et al., 2023).
Field measurements of the soil water content and matric potential were conducted in 2020 and 2021 in Slovenia (average July temperature 23, rainfall from May to September 668 mm), a depth of 20 cm (TDR - sensors, Delta-T Devices, Ltd., Cambridge, United Kingdom) on Mollic Gleysol on Soybeans [Glycine max (L.) Merr.] and maize (Zea mays L.) with the construction of a soil water retention curve (SWRC). Measurements were made in two growing seasons at different spatial locations on plots with different tillage systems: NT and CT. In both growing seasons, SWRCs reached lower pF values under the NT than under the CT (Pečan et al., 2023).
At the experimental site of Obersiebenbrunn in Lower Austria, ca. 35 km east of Vienna combinations of water content and isotope analysis in soil profiles were performed for 16 plots (Canet-Martí et al., 2023). The plots were planted with winter wheat (Triticum aestivum) and managed with different tillage (CT), (RT), (MT), (NT) and irrigation systems [hose reel boom irrigation with nozzles (BI), sprinkler irrigation (SI), drip irrigation (DI) and no irrigation (NI)]. Annual average precipitation, air temperature and potential evapotranspiration (ET0) were 540 mm, 10.5°C, and 626 mm. The soil was classified as Chernozem, with 4% of organic carbon content in the topsoil. The results indicated that the more intense the tillage, the lower the water content. The resulting evapotranspiration within tillage and irrigation variants decreased in the order CT > RT > MT > NT and SI > BI > DI > NI (Canet-Martí et al., 2023).
However, there are studies that indicate an ambiguous positive effect of the implementation of conservation agriculture on soil physicochemical properties and yields under drought conditions. For example, one study in North China evaluated the effects of three tillage practices (no-till; subsoil tillage—SS and deep tillage—DT) over 5 years on soil physicochemical properties (Yan Q et al., 2023). The results indicated that SS and DT improved grain yield, straw biomass and straw carbon return of wheat compared with NT. Compared with DT, soil available nutrients improved under NT and SS in the 0–20 cm layer (Yue K et al., 2023). There are earlier works that have identified various effects of NT. On the other hand NT systems showed some negative effects, particularly due to soil compaction in different layers (Kotovrasov and Pavlovsky, 1989; Andreini and Steenhuis, 1990; Pikul et al., 1993).
Some studies do not reveal any differences or consider that differences in the dynamics of soil agrophysical properties according to tillage systems are non-significant (Glukhikh and Sobyanin, 2000; Mukherjee and Lal, 2015; Lozano et al., 2016; Soracco et al., 2018). Therefore, it is particularly important to study the management opportunities that help improve the water capacity of soils in relation to plant growth and yields. This study supplements the comprehensive analysis of conditions and barriers to sustainable farming practices adoption in the Kulunda steppe, implemented in 2012–2016 within the Kulunda Project (KULUNDA, 2020) and provides a basis for research within the ongoing East Kazakhstan project started in 2021 (Nugumanova et al., 2021). It has been shown that there was a lack of knowledge regarding the possible benefits of no-till as well as its disadvantages and advantages, which influence farmers’ confidence in the technology’s performance. Thus, our investigation provides scientific support for the adoption of conservation tillage systems in the Kulunda steppe of cross-border Altai under worsened climate aridity, considering environmental factors.
Based on in situ measurements with soil-hydrological measuring stations (SHMS) installed in two adjacent fields, our study investigated 1) the short-term effects of NT and CT cropping systems on soil moisture and 2) the effects of the two different cropping systems on water availability for plant nutrition. Special focus was taken on the comparison of conventional tillage operations (CT), prevalent in the area under the study, and the soil conservation tillage operation (NT) on the potential of water-holding capacity as a steering factor for crop yield and having a high impact in crop production systems in the marginal ecosystems of the Kulunda Steppe. We followed the hypotheses that 1) water capacity in the root zone is under conservational cropping systems higher than under conventional and 2) under conservational cropping systems, soils provide water for plant nutrition during the vegetation period longer than under conventional systems. The hypothesis of our study and conducting direct measurements of soil volumetric moisture (%) and matrix potential (pF) during the growing seasons of 2013–2016 was based on the fact that implementation of CA in the arid zone on sandy loam and light loam soils over time changes the physical properties of soil and helps to increase soil moisture and water holding capacity. Further studies in different rainfall-deficient countries of the temperate Eurasian belt have shown that the introduction of CA has resulted in improved water retention, reduced evapotranspiration, reduced greenhouse gas emissions, and stabilized yields independent of the moisture content of the growing seasons (Blanco-Canqui and Ruis, 2018; Zhang et al., 2018; Huang et al., 2021; Tian et al., 2022; Vlček et al., 2022; Wang et al., 2022; Canet-Martí et al., 2023; Kamran et al., 2023; Pečan et al., 2023; Yan Q et al., 2023; Zhang et al., 2023). The study was the first to investigate complex cropping systems with regard to their effects on soil water balance parameters by means of continuous soil water investigations under field conditions in the region.
Materials and Methods
Investigation Area
To determine soil water under different tilling methods, a pedo-hydrological measuring network was installed in the Kulunda steppe in Southwestern Siberia. The tested field was selected on a farm near to the village Poluyamki in Mikhaylovsky district in Altai Krai. Two adjacent plots were selected from the field, which were managed according to the conventional mode in previous years. The trail plots had an equaled size of 12 m × 30 m with a distance between them of 6 m. Thus, two tested plots had similar soil types and experienced similar climate conditions. In September 2012, one weather station (WS) was installed in the territory of a farm in the village Poluyamki (WS—E 79° 42.786′ N 52° 03.959′). At the same time, two soil-hydrological measuring stations (SHMS) were installed in the trial plots (SHMS 1—N52° 04.180′ E79°54.014′; SHMS 2—N 52° 04.128′ E 79° 54.006′). The entire description of the trial field design is presented in (Belyaev et al., 2020). The tested plots were located at 12 km distance from the village Poluyamki.
The investigated soils are Calcic Chernozems (FAO, 2005), with a 25 cm thick humic horizon (plough zone) and an AC-horizon until a depth of 50 cm below ground level. The subsoil C-horizon is parent material (loess-like calcareous deposits). The grain size distribution (after German soil taxonomy) in the upper part of the profile (0–50 cm) is sandy loamy silt, beneath silt loam and below 70 cm loamy sand occurs (Ad-hoc-AG Boden, 2005). The site has been under intensive agricultural use for 60 years (Balykin et al., 2016).
The Monitoring Network and Experimental Design
The weather station was equipped with a radiometer, multisensory “VAISALA,” measuring wind speed, wind direction, air temperature, air humidity, barometric pressure, and rainfall. Additionally, a standard rain gauge was installed. The soil-hydrological measuring stations were equipped with sensors measuring soil water content (volumetric percentage—Vol. %), soil temperature, osmotic pressure, and electric conductivity at depths of 30, 60, and 120 cm logging data automatically. Measurements of WS were registered in 60-min intervals while SHMSs recorded in 6-h intervals. Supplementary Appendix SA summarizes the specification of installed equipment.
At the experimental plots, the effects of the following tillage operations were analyzed:
a) the conventional technology (CT)—tillage with deep loosener (PG-3–5—“ploskorez glubokorykhlitel”) at 22–24 cm depth and crop rotation was spring wheat (2013)—fallow (2014)—spring wheat (2015)—spring wheat (2016).
b) the no-till technology (NT)—no tillage and the crop rotation were spring wheat (2013)—rape (2014)—spring wheat (2015)—peas (2016).
In the plot under conventional technology, SHMS 1 was installed, while other plot was managed in the NT mode with SHMS 2 installed (Figure 1).
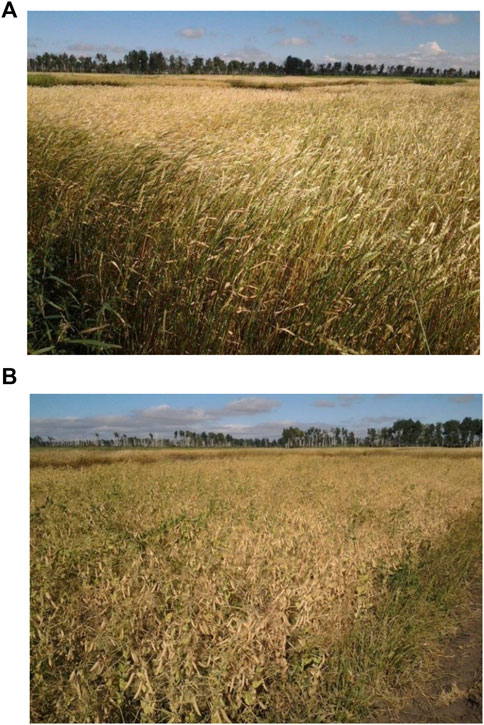
FIGURE 1. Crop growing in the tested plots in August 2016. Spring wheat in the CT plot (SHMS 1) (A) and peas in the NT plot (B) (SHMS 2). Source: A.A. Bondarovich (2016).
Analysis of Soil Water Availability
It is known that there is a relationship between the water holding capacity of soils and the soil type, especially to the grain-size distribution and porosity of soils (Ehlers, 1996). Thus, the values for field capacity (FC) are different for sandy, loamy, and clayey soils and depend on regional characteristics. The pF values from 1.8 to 2.5 refer to the relatively constant soil water content reached after 48 h drainage of water from a saturated soil (Ganjara, 2001). The value of 4.2 pF marks the border of water availability to plants, or the permanent wilting point (PWP), in which plant roots are not able to create proper osmotic pressure to the water tension and the plants lose turgor (Haertge and Horn, 2016). pF values between 4.2 and 2.5 describe the area of plant available water capacity. Available Water Capacity (AWC) is the water available for plant growth held between Field Capacity (FC) and Permanent Wilting Point (PWP) (Ehlers, 1996). With a deep groundwater table, AWC is the maximum possible capillary-suspended moisture. Thus, AWC is the most important soil hydrological quantity characterizing the ability of the soil to accumulate moisture and hold it in the field of gravitational forces (Ganjara, 2001).
According to Ehlers (1996), we assume that it is necessary to allocate a subclass of pF with limited availability of moisture for loamy and humic soils, such as Luvic Chernozem. Ehlers (1996) revealed that for loamy soils, in which “medium pores” occupy a large share (about 18%) of the soil profile, the values of pF 2.0–3.0 correspond to the optimal suitability of water for plants. The study (Ganjara, 2001) on loamy soils showed that FC with optimal water supply corresponds to the value of pF 2.3. There is a small number of studies, showing results of instrumental field observations of soil water-holding capacity under different farming strategies. Therefore, our study firstly allows the discussion of the question of limits of optimal water availability according to pF within territory of the Kulunda plain.
Existing water retention curves that were revealed in laboratory conditions describe, as usual, some ideal soils. We tried to combine the published approaches of water retention and water availability and set the limit of optimal water availability as 1.8–3.0 pF, including the values from 1.8 to 2.5 pF, when water moves slightly and is still available. Furthermore, due to the logic and technique of measurements we supposed that water was going on to the PWP (pF > 4.2), the content of water could not be optimally available on all measurements. In this case, we revealed the limit of “reduced availability” of pF 3.0–4.2. This scale can help on a respectively short row of observations to reveal some differences in the trends between tillage systems. Therefore, we propose the classification, shown in Table 1, to assess the availability of soil moisture for plant nutrition under different tillage operations.
Data Availability
Hourly measurements were registered during vegetation periods (May-September) in 2013–2016. We obtained the full time series of climatic data for the whole period of the study, but the datasets for SHMSs had some data gaps due to different technical reasons. We obtained the longest time series without data gaps from 3 May to 30 September in 2013 for both SHMSs (604 observations). The shortest series of measurements was received for CT technology (SHMS 1) in 2014 from 7 to 30 September (60 observations) while for NT we had a relatively complete dataset from 1 May to 30 September (608 observations). In 2015 and 2016, time series of both CT and NT technologies were relatively complete. There were some data gaps in the time series of CT in May-June-July in 2015 and 2016. Totally, we obtained 369 and 612 hourly observations in 2015, and 201 and 592 measurements in 2016 for CT and NT, respectively. Thus, to compare soil properties under conventional and conservation farming systems we used joint time series from both SHMSs matched by date and time of data registration. Nonetheless, the separated data for CT and NT are also of interest in the study of dynamics of soil hydrological parameters under different farming strategies.
Data Preparation and Statistical Analysis
The general principles of data preparation for WS and SHMSs were similar. First, we converted the precipitation cumulative data of multisensor “Vaisala” (WS) into absolute values per hour. Second, we removed erroneous data due to system error or noticeable outliers from the raw data. We prepared two datasets for CT and NT separately to analyze soil parameters under the studied farming practices during the experiment (2013–2016). Additionally, we joined two hourly time series (SHMS 1 and 2) by the date and the time of observation registration to find out differences of agri-important soil-hydrological parameters under CT and NT and their statistical significance.
Quantification of effects of the conservation farming system was conducted through estimation of differences in probability distribution of the studied parameters between two independent samples for CT and NT based on joint dataset. As both the soil moisture content (Vol. %) and the Matrix potential (pF) were not normally distributed, we employed the two-sample Wilcoxon Rank Sum Test with continuity correction (equivalent to the Mann-Whitney U test) (Haertge and Horn, 2016). Using joint hourly time series, we tested the null hypothesis that distributions of a soil parameter for both samples were identical with equal medians. According to Hollander and Wolfe (Hollander and Wolfe, 1999) and the algorithm described in (Haertge Bauer, 1972), we estimated a median and a mean of differences in the studied soil properties (CT-NT) and its 95% confidence interval. The data processing was performed in R using “Google Colaboratory” tools (RDocumentation, 2020).
Results
Climate Situation
Climate conditions of the Kulunda plain are semi-arid, with average annual precipitation from 220–250 mm in the South-West plains to 350 mm in the North-East (KULUNDA, 1972). The climatic parameters measured through the WS in the village Poluyamki during vegetation periods of 2013–2016 showed general agreement and statistically significant correlation (Schmidt et al., 2016) with the data measured by Roshydromet for the territory of Altai Krai (ROSHYDROMET, 2020). Based on the WS daily data in the village Poluyamki, dates of the start and the end of growing seasons in 2013–2016 were identified. The start and the end of vegetation periods were set at the date when daily average temperature passed +5°C. The longest vegetation period with 202 days was in 2014, and the longest period with a temperature higher than +10°C was in 2015. The summary of weather information for the growing seasons of the experiment is provided in Supplementary Appendix SB.
In “dry years” we observed a more consistent distribution of precipitation from May to September, when in the “provided moistening” years almost half of the precipitation happened in July. Thus, the share of precipitation in July in total sum of May-September in 2013 was 31%, 2015—37%, 2014—46% and in 2016—49%. Dry hot winds in Kulunda steppe continue to reinforce the evaporation effect in May and June. It was associated with an increased solar radiation and higher wind speed in these months. The proportion of precipitation in May-June ranged from 29% in 2014 to 38% in the moistest year 2016. 2014 was the least favorable in this respect, when high solar radiation and minimum rainfall in June were reported for the whole period of the study. According to experts’ opinions (ROSHYDROMET, 2020), the most favorable period for crop production was in 2016, when the proportion of precipitation in May-June was 37.7%, July—49.2% and August–September—13.1%.
Soil Moisture Content
Using the separated daily datasets for CT and NT farming practices, the descriptive statistics of soil moisture content (Vol. %) was computed for 2013–2016 (Supplementary Appendix SC). In the first year of the experiment (2013), differences in the soil water regime between the compared tillage methods could be caused by different soil textures of the tested plots. Moreover, the entire area had been cultivated in conventional mode and the same crop rotation was applied in previous years. The maximum amount of water contained in the soil (34.0 Vol. %) was measured at the depth of 60 cm in the CT plot (22 June 2013). Under NT, at the depth of 60 cm the maximum volume (33.5 Vol. %) had been observed earlier (3 May 2013). However, the maximum values at the depth of 30 cm were recorded on 3 May for both technologies. Minimum values at different depths for both options were registered in 20–27 September 2013. Comparison of medians showed that at the depth of 30 cm there was a higher daily amount of soil moisture under NT (19.3 Vol. %) than under CT (17.1 Vol. %). However, the amplitude had the highest value at the depth of 30 cm under CT (20.0 Vol. %), but under NT it was achieved at the depth of 120 cm (20.4 Vol. %).
The low amplitude usually indicates a more even distribution of the moisture through the season. Generally, the soil moisture in the CT plot was significantly higher than in the NT plot in 2013. The testing of the null hypothesis regarding differences in soil moisture distributions under tested technologies found out that there were positive shifts of medians at each of the depths (Table 2). The medians of differences in soil moisture (CT-NT) were 1.5, 2.6, and 3.4 Vol. % at the depths of 30, 60, and 120 cm, respectively. Thus, the CT plot had statistically significant higher soil moisture content compared to the NT plot. This fact was also confirmed by positive values of differences within 95% confidence intervals.
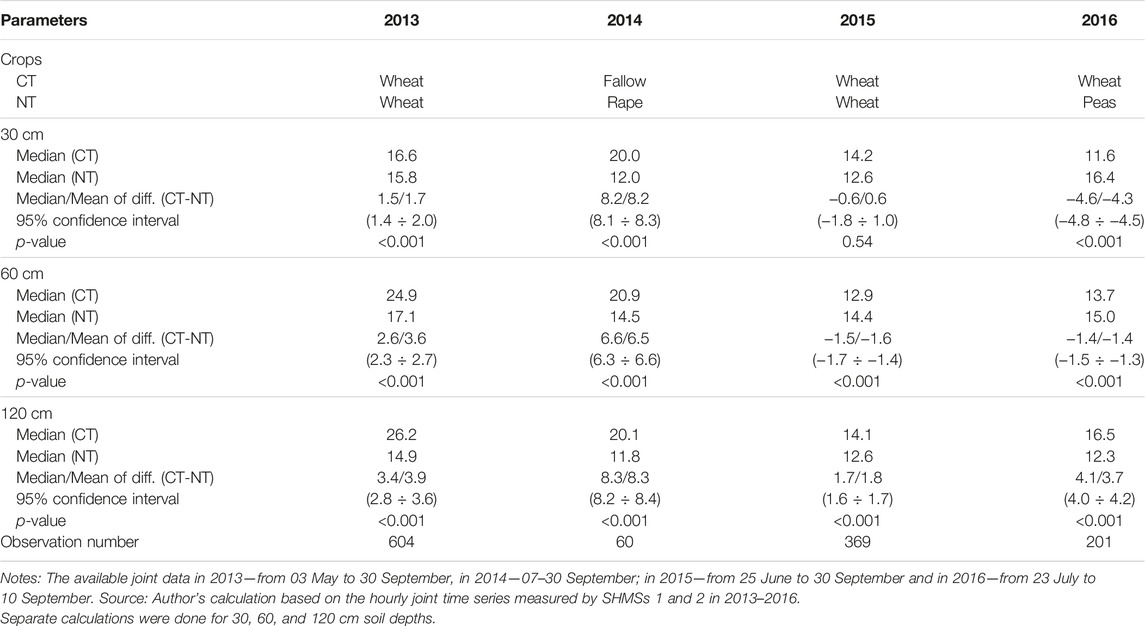
TABLE 2. Quantification of the differences in soil moisture content (Vol. %) in two adjacent trial plots, managed according to CT and NT modes, May–September 2013–2016, Poluyamki, Altai Krai.
We should note, for the next periods from 2014 to 2016, we did not observe soil moisture as high as in 2013. Such large amounts of water measured at different depths in 2013 is not characteristic of the dry steppe generally. The soil moisture content varied from 13 to 34 Vol. % in the CT plot and from 11.9 to 33.5 Vol. % in the NT plot, which can be explained by some natural and artificial reasons. Some of the natural causes were stocks of winter moisture. Thus, water content measurements in the soil by the express method (Table 3) at the sites showed that in early May of 2013 the amount of water was higher than in other years. The exception was May 2016 when the CT field had a water content higher by 7% than in 2013. It is important to note that after the hot and dry season in 2012 from September of 2012 to April of 2013, 232 mm of precipitation fell in this area (ROSHYDROMET, 2020). It is more than usual. Additionally, for better connection with soil, according to the installation guideline, the sensors of both SHMSs were covered with wet clay during the installation process in May 2013. That might have led to inflated values on the first days after sensors installation. The consequences of installation lasted until mid-June 2013. However, we decided to use most of this data in the analysis, because such effects were observed while using both technologies.
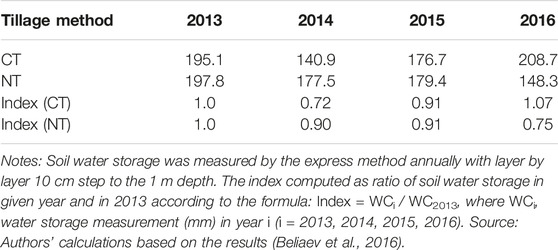
TABLE 3. Soil water storage (mm) in 1 m depth in the tested plots in the first ten days of May and its dynamics during the experiment, Poluyamki, Altai Krai.
In the second year of the experiment, comparisons of tilling methods could be made only at the end of growing season (September 2014) because of a large data gap in SHMS 1 measurements. However, daily data of soil moisture content under no-till are of interest in terms of soil property reactions to this conservation tillage system. The highest value of soil moisture for NT was observed on 4 May at the depth of 120 cm with 23.2 Vol. %, on 4 July at the depth of 30 cm with 16.7 Vol. %, and on 21 July at 60 сm with 22.6 Vol. %. The minimum values were reported in September. The maximum amplitude for NT (11.7 Vol. %) was observed at the depth of 120 cm as well as maximum of the standard deviation, the same as in 2013. At the depths of 60 and 120 cm the distribution became less even and the average values were higher than medians. Testing the differences between soil moisture content of the two farming systems based on joint hourly data showed that in September 2014 water content at all the depths under CT was significantly higher than under NT (Table 2). Most likely, it was not an effect caused only by tilling systems but also by the used crop rotations. There was mechanical fallow in the CT plot and rape in the NT plot. Thus, there were both moisture accumulation in fallow field and high-water consumption in rape field (Beliaev et al., 2016), that led to the decrease of soil water under NT especially at the depth of 30 cm.
In 2015, the cropped species were identical on both plots (spring wheat). The weather conditions were hotter than in the previous growing seasons with lower amount of precipitation (244.6 mm). In general, NT system showed higher daily values of soil moisture in comparison with CT. The maximum water content (23.9 Vol. %) under NT was on 8 May at the depth of 120 cm. Mean and median daily values for NT were higher than for CT at both 30 and 60 cm depths. In the third year of the experiment, difference in soil water content between CT and NT technologies at 30 cm depth was insignificant and varied from negative to positive effects (from −1.8 to 1.0 Vol. %). However, there was a significantly higher water storage under NT at the depth of 60 cm with 1.5 Vol. % of mean value of difference (CT-NT). The opposite effect was at the depth of 120 cm where at the same time hourly data indicated positive both median and mean values of differences in soil moisture between CT and NT.
In the fourth year of the experiment, the difference of soil moisture content became more evident, even though cultivated crops (peas and spring wheat) vary widely in water consumption. According to Geisler (1988) the soil moisture consumption for peas is about 600–700 L/kg of dry mass and for soft wheat—about 500–600 L/kg of dry mass. The NT system showed higher average values of the moisture content at 30 and 60 cm depths with 18.3 and 18.6 Vol. % than CT with 11.9 and 13.0 Vol. %, respectively. The average values were relatively similar for both systems at 120 cm depth with 16.6 and 16.7 Vol. %. Importantly, measurements of soil moisture under CT showed higher standard deviations at the depth of 30 cm compared with NT, the same as in 2013, 2015, and 2016. It was probably caused by a faster movement of water and an increase of evapotranspiration under deep tillage in 30 cm upper layers of soil. At the depths of 60 and 120 cm, the standard deviations of soil moisture were significantly higher under no-till compared to conventional tillage and can indicate, as a proxy indicator, the more intensive water movement. Statistically significant differences in soil moisture content (CT-NT) reached −4.6 and −1.4 Vol. % of median values at the depths of 30 and 60 cm, respectively. Notably, in deep soil layers in the NT field the accumulation of soil water was smaller than in the CT field and median of difference was 4.1 Vol. %.
Matrix Potential (pF)
The most important indicator in crop production, measured by the soil hydrological measuring stations, is soil moisture availability (Matrix potential, pF). To study dynamics of pF during the experiment, we computed the descriptive statistics based on daily data for CT and NT separately (Supplementary Appendix SD). According to mean values of pF, both tillage systems showed basically optimal water availability at all soil layers. However, transitions to the reduced water availability were observed in the NT plot at 30 cm depth in 2014 (3.13 pF) and in 2016 (3.25 pF), at 60 cm depth—in 2015 (3.18 pF). Under CT, there was 3.25 pF in 2016 at the depth of 30 cm, 3.06 pF in 2015 at 60 cm depth and 3.57 and 3.18 pF at 120 cm depth in 2015 and 2016, respectively. Maximum values of pF, which correspond the soil water unavailability (crossing the limit to “permanent wilting”), were also reported mostly under NT at 30 cm depth 4.44 pF (11 September 2014), 4.6 pF (15 August 2015) as well as 4.6 pF (25 September 2016). The pF values of more than 4.2 were also recorded at the 60 cm depth under NT at the end of growing seasons—4.46 pF (27 September 2015) and 4.24 pF (25 September 2016). Besides, there were no pF-values higher than the limit of sustainable wilting under CT.
To improve understanding of dynamics of soil water availability during vegetation seasons for different farming systems, we classified pF measurements according to the pF classes (Table 1). Totally, we obtained 2,425 hourly measurements of pF in the NT plot and 1,169 measurements in the CT plot for the vegetation seasons of 2013–2016. Based on hourly data, we calculated means and standard deviations of measured water holding capacity as well as observation distributions according to pF-classes through months (Figure 2). The class FC (1.8 ≤ pF < 3.0) represents the favorable water regime of biomass growth, AWC (3.0 ≤ pF < 4.2) associates with the maximum potential of soil water-holding capacity and, the limits of unavailable water are presented by classes G and PWP related with pF < 1.8 and pF ≥ 4.2, respectively.
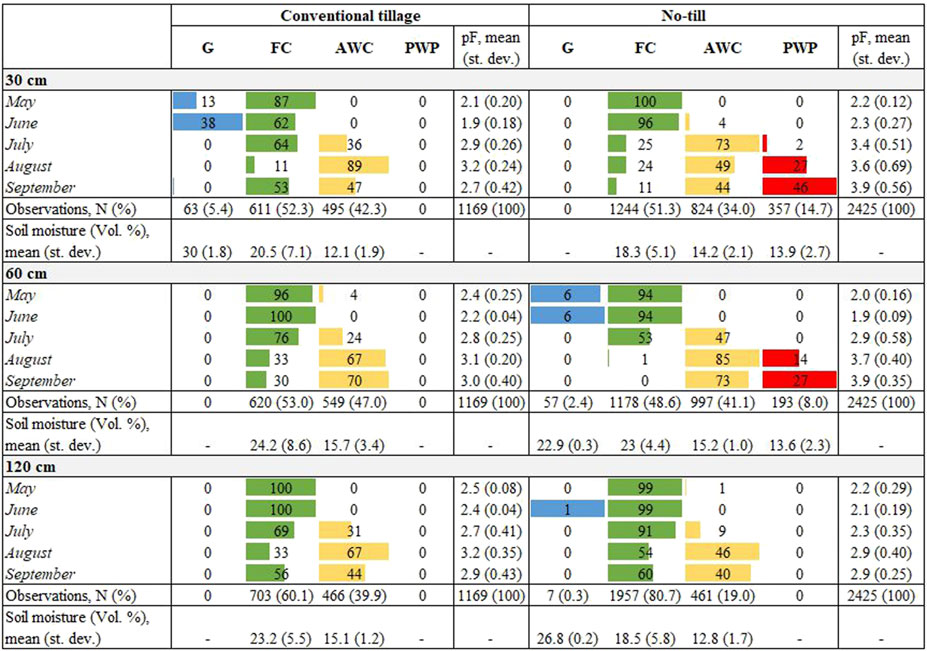
FIGURE 2. Distributions of hourly measurements of water holding capacity (pF) under tested tillage operations during the vegetation periods of 2013–2016, Poluyamki, Altai Krai. A separate analysis was done for the 30, 60, and 120 cm soil depths. Source: Authors’ calculation based on hourly data measured by SHMSs for 2013–2016.
Over the period of the experiment, the NT system had some advantages in the first months of growing seasons (May–June–July). Importantly, in the NT plot, no observation with low limit of unavailable water (the pF-class G) was registered at the depth of 30 cm, but there were 13% of all measurements in May and 38% in June in the CT plot. It is probably due to spring gravitational water registered by SHMS 1 in May–June of 2013. However, this happened only after deep autumn tillage, which definitely increased the water absorption. In the meantime, at 60–120 cm depths there was no gravitational water under CT. Both farming systems almost did not show the transition through the PWP (pF ≥ 4.2) from May to July. The main share of measurements was in the classes FC and AWC for both technologies at all depths. In August-September, when rainfall was not intensive, the pF distributions shifted to AWC and PWP classes that were related with the process of crop water consumption and transpiration. Transition through the PWP at the depth of 30 cm was found out under NT in August-September for 27%–46% of all measurements with average soil water 13.9 Vol. %. It was an effect of the farming systems, namely, crop rotations. In 2014 there was fallow in the CT plot considered as a method of soil water conservation, and rape in the NT plot. In 2016, it was spring wheat in the CT plot and peas in the NT plot, which has higher water consumption compared to spring wheat.
We should note that at the depth of 60 cm there was 6% of observations in May and June under NT in the pF-class G as well as 1% of observations at the depth of 120 cm. We also observed the shifting of measurement distribution during vegetation seasons to AWC and PWP classes in the NT plot at the 60 cm depth. It was getting lower with the depth of soil. There was no observation in G and PWP classes in the CT plot. The share of observations with water content in AWC (3.0 ≤ pF < 4.2) under CT was 47% with an average soil water 15.7 Vol. %, and in the NT plot in this class was registered 41% of measurements with an average soil water of 15.2 Vol. %. Using NT, the share of observations in the class PWP (pF ≥ 4.2) was 8% with an average soil water of 13.6 Vol. %.
The water at 120 cm depth was better to be considered as a potential storage, which is hardly available for the main crops (FAO, 2005). At the same time, the analysis of soil water content at such depth allows to improve knowledge about the regime and movements of soil water in terms of infiltration and water rising under different tillage systems. Using NT, the share of observations in the FC class (1.8 ≤ pF < 3.0) was significantly higher (81%) with an average soil water of 18.5 Vol. % than under CT—60% with an average soil water of 23.2 Vol. %. In the pF-class AWC (3.0 ≤ pF < 4.2), 40% of observations for CT with an average soil water 15.1 Vol. %, and 19% of pF measurements for NT with an average soil water of 12.8 Vol. % were reported. At the depth of 120 cm there were no measurements in pF-classes G and PWP for CT and only 1% of observations under NT in June.
To quantify the differences between cropping systems in the soil water availability during vegetation periods, we tested hypotheses about equal distribution of measurements based on joint hourly time series (Table 4). We found out that differences in mean values of pF and shifting of medians for CT and NT were statistically significant. The mean of pF difference (CT-NT) was reduced through the deepness of soil layers. We also observed increasing differences in pF during a vegetation season.
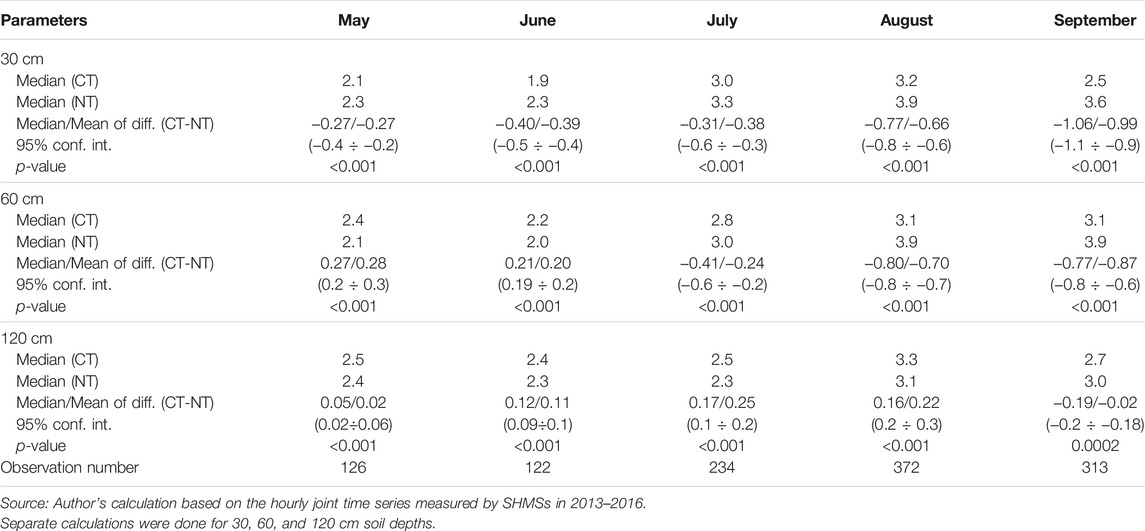
TABLE 4. Quantification of the differences in Matrix potential (pF) in two adjacent trail plots managed according to CT and NT modes, May–September 2013–2016, Poluyamki, Altai Krai.
Discussion
The study attempts to quantify the short-term benefits of no-till compared with conventional tillage on the soil water content and plant water availability in the dry steppe of the Kulunda plain. The short-term analysis is important to find out the effects of the first stage of conservation tillage adoption especially from the point of view of managing small farms. As shown (Bavorova et al., 2018; Bavorova et al., 2019; Bavorová et al., 2020), the adoption of a no-till system in Altai Krai demands large investments and small-scale agricultural business faces limitations of financial sources and needs to shorten the period of payback. Additionally, it is well known that no-till requires accumulation of residues on a field that is difficult to achieve in mixed farm specialization combined crops and livestock production. Small-scale farms cultivated 31.4% of arable land in Altai Krai, most of them practice only crop production (Geisler, 1988). In this point of view, understanding short-term differences in soil properties under the compared farming strategies is an essential factor which influences farmers’ attitude to conservation tillage systems. Revealing possible benefits of no-till will be able to enhance farmers’ confidence and further shape farmers’ intentions to adopt no-till. In general, that point is important for wide spreading and adoption of conservation soil tillage systems in the region and small farms may represent an “engine” of this process.
In our study two adjacent fields, which had a similar soil type and experienced similar climatic conditions, were managed according to no-till and conventional tillage in parallel during the 4-year experiment. The soil hydrological measuring stations and the weather station continuously measured climatic and soil parameters with a time resolution of 60 min. To our knowledge, there are no such comparative studies in the Kulunda plain.
The short-term experiment revealed statistically significant differences in both the soil moisture content and the water-holding capacity between the studied technologies. Comparative analysis was based on joint hourly measurements for the vegetation periods in 2013–2016. The received hourly measurements agreed with existing experimental models which describe the relationships of the water-holding capacity and soil types as well as soil texture (Ehlers, 1996; Ganjara, 2001). At the depth of 30 cm, mean soil water for FC class (1.8 ≤ pF < 3.0) was 20.5 Vol. % for CT and 18.3 Vol. % for NT. At the 60 cm depth, it was 24.2 and 23 Vol. % for CT and NT, respectively. These values correspond to the soils with light loamy physical structure. The soils of investigated plots are the light loamy and medium-humified Luvic Chernozem (Balykin et al., 2016).
Importantly, at the fourth year of the experiment the differences in the soil water regime became more evident despite the different crop rotations in the tested fields. Tested CT system implied the monoculture with spring wheat and fallow while the NT system supposed rotational cropping (with spring wheat, rape, and peas). The significantly higher standard deviation of both water content and pF under NT compared to CT indicates, in our opinion, an accelerated water movement in the soil layer from 0–120 cm. This can be caused by higher preferential flow. As a result of the greatly reduced mechanical stress at NT, the habitat of soil organisms is less disturbed. Thus, the proportion of preferential flow paths in the form of animal passages in the pore space can increase. Tested conventional tillage system, supposed deep tilling, showed significantly lower standard deviation and soil water dynamics in the deep soil layers (60–120 cm). Nonetheless, analysis of pF values showed that CT had a slight advantage at the 30–60 cm soil layer. It is probably because deep ploughing intensifies water movement (infiltration). That fact was proved by presence of the gravitational water measured in the CT plot at 30 cm depth in May 2013.
Both systems showed the decreasing soil water availability during the vegetation seasons, but the most negative trend was observed for NT. In July “reduced availability of soil water” (from 3.0 to 4.2 pF) was reported even at 73% of all measurements under NT, for CT there was only 36%. At 60 cm depth the share of such measurements was also higher for NT (47%) than CT (24%). In August and September, at 30 cm soil layer the share of observations in PWP class was 27% and 46% for NT, at 60 cm depth it was 14% and 27%, while for CN there were no such measurements.
Some confirmations of our findings we found out in previous studies which were conducted in Kulunda plain and areas had similar soil and climatic conditions. Belyaev et al. (2017) revealed higher soil water content at the start of vegetation season in CT fields than in NT fields for 2013–2015, that was due to tested autumn tillage operations for soil moisture conservation. They used soil water measurements according to the express method (the TDR-sonde, Eco-Tech) in the 1 m soil depth in sunflower fields. They also showed that at the end of the vegetation period (August) in NT fields the water storage was higher compared to CT fields. According to the latest results (Belyaev et al., 2022), the moisture consumption per one unit of crop productivity (in the case of sunflower) for NT was 1.5 times lower than for CT that reflected the crop yield which was much higher for CT by 5.2 dt/ha.
Bakirov and Petrova (2014) investigated the effects of NT technology on the southern chernozem in Orenburg region (foothill of Ural Mountains) during the dry weather conditions in 2012 for different crops (spring wheat, barley, chickpea, sun-flower and rape). They found out that mulch (MT) and conventional (CT) technologies have some advantages of the water content in a 1 m soil layer compared to NT. They also showed that the differences among technologies were decreased during the vegetation period. Significant differences in the crop productivity in relation to the tillage systems were not revealed.
Interestingly, some studies showed that in Kastanozems in dry years, the amount of water was higher for NT than for CT, but in wet years it was opposite (Gavrilov et al., 2001; Korchagin and Novikov, 2009). We could not make final conclusions based on our measurements about this effect because 4-years experiment. We should note that there was a drought in 2012 with 104 mm of precipitation for May-August (Russian Federation, 2013; ROSHYDROMET, 2020). Unfortunately, we set up the experiment later, perhaps we could reveal apparent effects of NT even at the start of the experiment (2012–2013). However, at the fourth year of the experiment (2016, was the wet season), the soil moisture was higher under CT (20.8 Vol. %) than under NT (17.7 Vol. %) at the 30 cm depth in the start of vegetation period (May 1, 2016). At the 60 and 120 cm soil layers we had the opposite results—21.3 and 23.0 Vol. % at 60 cm depths, and 20.6 and 23.0 Vol. % for CT and NT, respectively. Despite the starting soil moisture shortage advantages of CT at 30 cm soil depth, the NT system showed positive moisture conservation effects. Reduction of soil moisture during the vegetation period (from 1 May to 1 September 2016) was −9.6 and −2.0 Vol. % at 30 cm depth for CT and NT, correspondingly. Moreover, NT reported higher average soil moisture content in all soil layers during the vegetation period of 2016 (Supplementary Appendix SC). Decreasing of soil moisture at 60 cm depth was similar, but at 120 cm soil depth it was 11.0 Vol.% for NT and only 3.9 Vol. % for CT. The findings within our experiment support the positive effects of moisture conservation for NT stated in hypothesis 1 even in wet years.
In the NT field the soil moisture is distributed more equally throughout depth and the storage of “optimally available soil water” is formed at 100–120 cm. This finding is confirmed in our experiment. In deep soil layers, 81% of measurements in the 120 cm soil layer were classified as optimally available soil water while it was reported at only 60% for CT. Probably because in dry seasons the water is rising from deep soil layers that makes the NT system more resilient during droughts.
Several studies of NT systems in different territories revealed both positive influences of NT to soil properties and disadvantages—lower crop yield compared to other conventional technologies. In Stavropol region of Russia, it was noted that in temperate-humid zones (Leached Chernozem) a positive effect of NT appeared only at 4–5 years, namely, NT showed better water conservation than others, but this advantage did not provide higher wheat productivity (Wolters et al., 2001; Drepa and Golub, 2014). Slyusarev et al. (2017) revealed the influence of NT on the physicochemical properties of leached Chernozem in the territory of Prikubanskaja lowland (Russia) in 2014–2016. The crop rotation in their experiment was 1) spring wheat 2) sunflower 3) maize with different fertilizer schemes. Their findings highlighted a positive effect of NT on physicochemical properties of soil, but, at the same time, they obtained a low crops productivity and high production costs (Slyusarev et al., 2017) that has high impact in terms of NT adoption. We can make comparisons of crop yield within our experiment only in the first and third years of the experiment (2013 and 2015) when a similar crop was grown. In 2013 the spring wheat yield was 2.69 and 2.61 t/ha for CT and NT, respectively, while in 2015, the wheat yield was reported to 1.41 and 1.47 t/ha (Beliaev et al., 2016). Thus, 4 years of experiment did not reveal differences in crop yields.
The advantage of NT that relates to more sustainable water movements during the vegetation period is discounted by the decrease of water availability during the vegetation period, which in turn leads to lower crop productivity. Regarding hypothesis 2 our study showed that water in NT becomes hardly available or not available only in the end of the growing season, basically in August-September. This period is not that important for crop production in the conditions of dry steppe, as almost no winter crops are cultivated. Here, soil water capacity is not directly connected to crop productivity, but to study this relationship is not possible even in experiments conducted under the same climatic conditions. Thus, the results of investigating maize in NT and CT in the Argentine pampa in 2014–2015, demonstrate that many agrophysical properties show temporary changes and different trends during the vegetation period depending on the tillage system (Soracco et al., 2018). But availability of moisture was rather low in both options in the preplanting and growth periods. Also, the authors gave other examples where the productivity was higher when using CT or it was equal in NT (Soracco et al., 2018). Vague results were obtained for winter wheat (Triticum aestivum L.) by NT and in Oklahoma (Omara et al., 2019). Obviously for the revelation of advantages according to the water balance and crop productivity much more time for observations is needed. Thus, a 31-year experiment in the Canadian prairie showed the advantages of NT against other treatment systems. They mentioned that the yield increased due to an increase in soil water in the 0–30 cm soil layer with NT and MT (Lafond et al., 2011).
The decrease of water availability in our experiment with NT at 30–60 cm depth could depend on the gradual soil compaction and density. It was noticed that MT and NT lead to soil compaction (and higher bulk density) in comparison to ploughing and a decrease of water infiltration and root penetration of cultures in the deeper layers (Pikul et al., 1993; Medvedev, 2009; Tolon-Becerra et al., 2011). That is why some studies support the NT treatment but recommend using a plough once every several years (Haertge Bauer, 1972; Derpsch et al., 2010; Lafond et al., 2011; Zhang et al., 2023). Some authors support the solution of this problem in the production of a maximum quantity of field residues, and in a combination of crops that together lead not only to a decrease of loss of water through evaporation but also to the decompaction of upper soil layers (Gavrilov et al., 2001; Slyusarev et al., 2017; Schmidt et al., 2018). Crop residues in the NT treatment was not measured in our experiment. Four years is a too short period for accumulation of optimal field residues in the conditions of dry steppe, considering that rape (2014) was applied in the crop rotation which leads to poor residue production.
In our experiment, data were obtained for a rather short observation period. Therefore, the influence of crops noted by individual authors on the agrophysical properties of the soil and the water content (Schmidt et al., 2018; Soracco et al., 2018) was not observed. However, according to our measurements, the smallest amount of unavailable water (PWP class) of 12%–14% was observed in rapeseed in September 2014 and with pea 16%–18% in August and September 2016 in the NT plot. It is well known that rape and pea have a high level of water consumption (Geisler, 1988).
Conclusion
Our research shows that adapted cropping systems can contribute to an improvement in soil water balance and water supply for plant growth in arid regions. With the results of our field experiments the research hypotheses could be confirmed. Despite the differences in crop rotations, the revealed effects were statistically significant. The main limitation of the study was the short-term period of the experiment. However, we discovered significant differences in soil water regime between conventional and conservational cropping systems. This result represents important evidence of possible short-term benefits of the conservation tillage system under the dry climate conditions of southwestern Siberia.
The authors believe that long-term studies are needed to confirm the findings. Thus, the effects of crop rotation can be neglected for the measurement results.
To sum up, the study contributed to a better understanding of the advantages of conservation tillage in comparison with conventional modes in marginal steppe areas of Eurasia concerning implementation of sustainable food production practices.
Data Availability Statement
The original contributions presented in the study are included in the article/Supplementary Material, further inquiries can be directed to the corresponding author.
Author Contributions
Supervision: PI and GS. Conceptualization, methodology and writing–original draft preparation: AB. Software, investigation, data curation and validation: EP and AB. Writing—review and editing: AN and AM. Visualization: EP and AM. Funding acquisition: AN and MS. All authors contributed to the article and approved the submitted version.
Funding
This research was funded by the Science Committee of the Ministry of Science and Higher Education of the Republic of Kazakhstan, grant number AP09259379.
Conflict of Interest
The authors declare that the research was conducted in the absence of any commercial or financial relationships that could be construed as a potential conflict of interest.
Supplementary Material
The Supplementary Material for this article can be found online at: https://www.frontierspartnerships.org/articles/10.3389/sjss.2023.11493/full#supplementary-material
References
Ad-hoc-AG Boden (2005). Bodenkundliche Kartieranleitung, 5. Auflage, Hannover, Manual of Soil Mapping (KA5). 5th ed.
Andreini, M. S., and Steenhuis, T. S. (1990). Preferential Paths of Flow under Conventional and Conservation Tillage. Geoderma 46, 85–102. doi:10.1016/0016-7061(90)90009-X
Baig, M. N., and Gamache, P. M. (2009). The Economic, Agronomic and Environmental Impact of No-Till on the Canadian Prairies. Canada: Alberta Reduced Tillage Linkages, 134.
Bakirov, F. G., and Petrova, G. V. (2014). The Effectiveness of No-Till Technology on the Chernozems of the Southern Orenburg Preduralye (In Russian). Izv. Orenbg. State Agrar. Univ. 2014, 23–26.
Balykin, D., Puzanov, A., Stephan, E., and Meissner, R. (2016). “Using the Innovative Lysimeter Technology in the German–Russian Research Project KULUNDA,” in Novel Methods for Monitoring and Managing Land and Water Resources in Siberia. Editors L. Mueller, A. K. Sheudshen, and F. Eulenstein (Cham: Springer Water. Springer International Publishing), 387–399. doi:10.1007/978-3-319-24409-9_16
Bavorova, M., Imamverdiyev, N., and Ponkina, E. (2018). Farm-level Economics of Innovative Tillage Technologies: the Case of No-Till in the Altai Krai in Russian Siberia. Environ. Sci. Pollut. Res. 25, 1016–1032. doi:10.1007/s11356-017-9268-y
Bavorová, M., Ponkina, E. V., Herzfeld, T., Imamverdiyev, N., Baisakova, N., Ganzha, S. V., et al. (2020). “Transition of Agriculture in Altai Krai: The Role of Structural Change, Introduction of Modern Soil Cultivation Practices and Agricultural Policy,” in KULUNDA: Climate Smart Agriculture, Innovations in Landscape Research. Editors M. Frühauf, G. Guggenberger, T. Meinel, I. Theesfeld, and S. Lentz (Cham: Springer International Publishing), 231–240. doi:10.1007/978.3030-15927-6_16
Bavorova, M., Ponkina, E. V., Imamverdiyev, N., and Hirschauer, N. (2019). Effect of Adoption of Sustainable Crop Production Systems on Farm Economics. Environ. Dev. Sustain. 22, 6961–6984. doi:10.1007/s10668-019-00522-1
Beliaev, V., Grunwald, L., Meinel, T., Schmidt, G., Bondarovich, A. A., Scherbinin, V. V., et al. (2016). Soil Water Regime and Crop Yields in Relation to Various Technologies of Cultivation in the Kulunda Steppe (Altai Krai). Visnyk Dnipropetrovsk Univ. Biol. Ecol. 24, 531–539. doi:10.15421/011672
Belyaev, V. I., Grunwald, L.-C., Akshalov, K. A., Meinel, T., and Sokolova, L. V. (2020). “Modernization of Current Agricultural Technologies of Grain Production under the Conditions of a Steppe Zone of the Altai Region,” in KULUNDA: Climate Smart Agriculture, Innovations in Landscape Research. Editors M. Frühauf, G. Guggenberger, T. Meinel, I. Theesfeld, and S. Lentz (Cham: Springer International Publishing), 341–354. doi:10.1007/978-3-030-15927-6_25
Belyaev, V. I., Mainel, T., Tissen, R., Rudev, N. V., Kozhanov, N. A., and Sokolova, L. V. (2017). Comparative Estimation of a Water Regime of Soil and Sunflower Yield at Various Technologies of Autumn Tillage under Conditions of Kulunda Steppe of Altay Krai (In Russian). Bull. Altay State Agrar. Univ. 151, 27–34.
Belyaev, V. I., Varlagin, A. V., Dridiger, V. K., Kurganova, I. N., Orlova, L. V., Orlov, S. V., et al. (2022). The Global Climate Agenda. Soil Conservation Resource-Saving (Carbon) Agriculture as a Standard of International and National Strategies for Soil Conservation and Agricultural Carbon Markets. Int. Agric. J. 1, 421–441. doi:10.55186/25876740-2022-6-1-26
Blanco-Canqui, H., and Ruis, S. J. (2018). No-tillage and Soil Physical Environment. Geoderma 326, 164–200. doi:10.1016/j.geoderma.2018.03.011
Bodner, G., Nakhforoosh, A., and Kaul, H. P. (2015). Management of Crop Water under Drought: a Review. Agron. Sustain. Dev. 35, 401–442. doi:10.1007/s13593-015-0283-4
Canet-Martí, A., Morales-Santos, A., Nolz, R., Langergraber, G., and Stumpp, C. (2023). Quantification of Water Fluxes and Soil Water Balance in Agricultural Fields under Different Tillage and Irrigation Systems Using Water Stable Isotopes. Soil Tillage Res. 231, 105732. doi:10.1016/j.still.2023.105732
Derpsch, R., Friedrich, T., Kassam, A., and Hongwen, L. (2010). Current Status of Adoption of No-Till Farming in the World and Some of its Main. Int. J. Agric. Biol. Eng. 3, 1–25.
Drepa, E., and Golub, A. (2014). Physical Properties of Soil Using No-Till Technology (In Russian). Vestn. Agropromyshlennogo Kompleksa Stavropolja 16, 181–185.
Ehlers, W. (1996). Water in Soil and Plant Dynamics of the Water Balance as the Basis of Plant Growth and Yield. Stuttgart: Publisher Eugen Ulmer.
FAO (2005). Drought-resistant Soils: Optimization of Soil Moisture for Sustainable Plant Production. Rome: FAO.
FAO (2000). Land Resource Potential and Constraints at Regional and Country Levels. World Soil Resources Report 90. Rome: FAO.
FAO (2021). The State of the World’s Land and Water Resources for Food and Agriculture – Systems at Breaking Point. Synthesis report. Rome: FAO. doi:10.4060/cb7654en
Foley, J., Ramankutty, N., Brauman, K., Cassidy, E. S., Gerber, J. S., Johnston, M., et al. (2011). Solutions for a Cultivated Planet. Nature 478, 337–342. doi:10.1038/nature10452
Gavrilov, A. M., Levkin, V. N., and Teleychenko, N. I. (2001). Water-physical Properties of Light-Chestnut Soil Depending on the Main Treatment of Black Steam (In Russian). Zemled. Arable Farming 2, 3–27.
Geisler, G. (1988). Crop Production. A Textbook - Biological Foundations and T Echnique of Plant Production. Berlin and Hamburg: Verlag Paul Parey.
Glukhikh, M. A., and Sobyanin, V. B. (2000). Soil Cultivation in the Trans-urals. Arable Farming 2000, 32–33.
Haertge Bauer, D. F. (1972). Constructing Confidence Sets Using Rank Statistics. J. Am. Stat. Assoc. 67, 687–690. doi:10.1080/01621459.1972.10481279
Haertge, K. H., and Horn, R. (2016). Essential Soil Physics: An Introduction to Soil Processes, Functions, Structure and Mechanics. Semantic Sch. Available at: https://www.semanticscholar.org/paper/Essential- Soil-Physics%3A-An-introduction-to-soil-and-Haertge- Horn/8621e683eb340868d76008d6d3066187003171c3#paper-header (Accessed April 4, 2020).
Hollander, M., and Wolfe, D. A. (1999). “Nonparametric Statistical Methods,” in Wiley Series in Probability and Statistics. 2nd ed. (New York: Wiley).
Huang, X., Wang, H., Zhang, M., Rainer, H., and Ren, T. (2021). Soil Water Retention Dynamics in a Mollisol during a Maize Growing Season under Contrasting Tillage Systems. Soil Tillage Res. 209, 104953. doi:10.1016/j.still.2021.104953
Kamran, M., Yan, Z., Ahmad, I., Jia, Q., Muhammad Usman, G., Chen, X., et al. (2023). Assessment of greenhouse gases emissions, global warming potential and net ecosystem e conomic benefits from wheat field with reduced irrigation and nitrogen management in an arid region of China. Agric. Ecosyst. Environ. 341, 108197. ISSN 0167-8809. doi:10.1016/j.agee.2022.108197
Kassam, A., Friedrich, T., and Derpsch, R. (2019). Global Spread of Conservation Agriculture. Int. J. Environ. Stud. 76 (1), 29–51. doi:10.1080/00207233.2018.1494927
Korchagin, B. A., and Novikov, V. G. (2009). Soil-protective and Water-Saving Technological Complexes for the Cultivation of Grain Crops in the Dry Steppe Areas of the Middle Transvolga (In Russian). Sci. Technol. Agro-Ind. Complex 2009, 12–14. Dostizhenija Nauli Tekhniki Agro-Promyshlennogo Kompleksa Achiev.
Kotovrasov, I. P., and Pavlovsky, I. B. (1989). Experience in the Minimization of Soil Cultivation in Ukraine. Arable Farming 1989, 46–47.
KULUNDA (2020). in KULUNDA: Climate Smart Agriculture: South Siberian Agro-Steppe as Pioneering Region for Sustainable Land Use. Editors M. Frühauf, G. Guggenberger, T. Meinel, I. Theesfeld, and S. Lentz (Switzerland: Springer Nature Switzerland AG). doi:10.1007/978-3-030-15927-6
Lafond, G. P., Walley, F., May, W. E., and Holzapfel, C. B. (2011). Long Term Impact of No-Till on Soil Properties and Crop Productivity on the Canadian Prairies. Soil &Tillage Res. 117, 110–123. doi:10.1016/j.still.2011.09.006
Lal, R. (2020). Managing Soils for Negative Feedback to Climate Change and Positive Impact on Food and Nutritional Security. Soil Sci. Plant Nutr. 66 (1), 1–9. doi:10.1080/00380768.2020.1718548
Liebhard, G., Andreas, K., Neugschwandtner Reinhard, W., and Reinhard, N. (2022). Effects of Tillage Systems on Soil Water Distribution, Crop Development, and Evaporation and Transpiration Rates of Soybean. Agric. Water Manag. 269, 107719. ISSN 0378-3774. doi:10.1016/j.agwat.2022.107719
Lozano, L. A., Soracco, C. G., Villarreal, R., Ressia, J. M., Sarli, G. O., and Filgueira, R. R. (2016). Soil Physical Quality and Soybean Yield as Affected by Chiseling and Subsoiling of a No-Till Soil. Rev. Bras. Ciênc. Solo 40. doi:10.1590/18069657rbcs20150160
Mora, C., Frazier, A., Longman, R., Dacks, R. S., Walton, M. M., Tong, E. J., et al. (2013). The Projected Timing of Climate Departure from Recent Variability. Nature 502, 183–187. doi:10.1038/nature12540
Mukherjee, A., and Lal, R. (2015). Tillage Effects on Quality of Organic and Mineral Soils under On-Farm Conditions in Ohio. Environ. Earth Sci. 74, 1815–1822. doi:10.1007/s12665-015-4189-x
Nugumanova, A., Bondarovich, A., Tlebaldinova, A., Shuller, I., Rakhymbek, K., and Maulit, A. (2021). “Spatial Interpolation of Humidity Values in the Fields of East Kazakhstan Agricultural Experimental Station,” in 2021 16th International Conference on Electronics Computer and Computation (ICECCO), Kaskelen, Kazakhstan, 25-26 November 2021.
Omara, P., Aula, L., Eickhoff, E. M., Dhillon, J. S., Lynch, T., Wehmeyer, G. B., et al. (2019). Influence of No-Tillage on Soil Organic Carbon, Total Soil Nitrogen, and Winter Wheat (Triticum aestivum L) Grain Yield. Grain Yield. Int. J. Agron. 2019, 1–9. doi:10.1155/2019/9632969
Pečan, U., Marina, P., and Damijana, K. (2023). Variability of In Situ Soil Water Retention Curves under Different Tillage Systems and Growing Seasons. Soil Tillage Res. 233, 105779. ISSN 0167-1987. doi:10.1016/j.still.2023.105779
Pikul, J. L., Ramig, R. E., and Wilkins, D. E. (1993). Soil Properties and Crop Yield Among Four Tillage Systems in a Wheat-Pea Rotation. Soil Tillage Res. 26, 151–162. doi:10.1016/0167-1987(93)90041-M
RDocumentation (2020). RDocumentation - wilcox.Test Function in R. Available at: https://www.rdocumentation.org/packages/stats/versions/3.6.2/topics/wilcox.test (Accessed April 4, 2020).
Rolinski, S., Prishchepov, A. V., Guggenberger, G., Bischoff, N., Kurganova, I., Schierhorn, F., et al. (2021). Dynamics of Soil Organic Carbon in the Steppes of Russia and Kazakhstan under Past and Future Climate and Land Use. Reg. Environ. Change 21, 73. doi:10.1007/s10113-021-01799-7
ROSHYDROMET (2020). ROSHYDROMET. Available at: http://www.meteorf.ru/ (Accessed May 4, 20).
Russian Federation (2013). Review of Environmental Conditions and Pollution in the Russian Federation for 2012. Moscow: Russian Federation.
Schmidt, E. S., Villamil, M. B., and Amiotti, N. M. (2018). Soil Quality under Conservation Practices on Farm Operations of the Southern Semiarid Pampas Region of Argentina. Soil Tillage Res. 176, 85–94. doi:10.1016/j.still.2017.11.001
Schmidt, G., Bondarovich, A. A., Scherbinin, V. V., Ponkina, E. V., Harlamova, N. F., Matsyura, A. V., et al. (2016). Results of Operation of the International Agricultural Meteorological and Soil Hydrological Monitoring Network in Kulunda Plain (The South of Western Siberia, 2013-2015). Acta Biol. Sib. 2, 89. doi:10.14258/abs.v2i2.1424
Shukla, P. R., Skeg, J., Buendia, E. C., Masson-Delmotte, V., Pörtner, H. O., Roberts, D. C., et al. (2019). Climate Change and Land: An IPCC Special Report on Climate Change, Desertification, Land Degradation, Sustainable Land Management, Food Security, and Greenhouse Gas Fluxes in Terrestrial Ecosystems. Geneva, Switzerland: IPCC.
Slyusarev, V. N., Podkolzin, O. A., and Osipov, A. V. (2017). The Effect of Agrotechnologies Using the Zero-Tillage System on the Physico-Chemical Properties of the Chernozem Leached in the Kuban Lowland and the Productivity of Field Oil Crops (In Russian). Sci. Tech. Bull. - Russ. Res. Inst. Oil-Bear. Cult. 172, 52–60.
Smith, P. (2004). Carbon Sequestration in Croplands: the Potential in Europe and the Global Context. Eur. J. Agron. 20 (3), 229–236. ISSN 1161-0301. doi:10.1016/j.eja.2003.08.002
Soane, B. D., Ball, B. C., Arvidsson, J., Basch, G., Moreno, F., and Roger-Estrade, J. (2012). No-till in Northern, Western and South-Western Europe: A Review of Problems and Opportunities for Crop Production and the Environment. Soil Tillage Res. 118, 66–87. doi:10.1016/j.still.2011.10.015
Soracco, C. G., Lozano, L. A., Villarreal, R., Melani, E., and Sarli, G. O. (2018). Temporal Variation of Soil Physical Quality under Conventional and No-Till Systems. Rev. Bras. Ciênc. Solo 42. doi:10.1590/18069657rbcs20170408
Tian, M., Qin, S., Richard, W. W., Zhou, H., Ren, T., and Gao, W. (2022). Changes of Soil Structure under Different Tillage Management Assessed by Bulk Density, Penetrometer Resistance, Water Retention Curve, Least Limiting Water Range and X-Ray Computed Tomography. Soil Tillage Res. 221 (105420), 105420. ISSN 0167-1987. doi:10.1016/j.still.2022.105420
Tolon-Becerra, A., Tourn, M., Botta, G. F., and Lastra-Bravo, X. (2011). Effects of Different Tillage Regimes on Soil Compaction, Maize (Zea mays L) Seedling Emergence and Yields in the Eastern Argentinean Pampas Region. Soil Tillage Res. 117, 184–190. doi:10.1016/j.still.2011.10.003
Vlček, L., Václav, Š., Nikol, Z., Petr, Č., David, K., and Jan, V. (2022). Water Retention and Infiltration Affected by Conventional and Conservational Tillage on a Maize Plot; Rainfall Simulator and Infiltrometer Comparison Study. Agric. Water Manag. 271, 107800. ISSN 0378-3774. doi:10.1016/j.agwat.2022.107800
Wang, R., Ma, L., Lv, W., and Li, J. (2022). Rotational Tillage: A Sustainable Management Technique for Wheat Production in the Semiarid Loess Plateau. Agriculture 12 (10), 1582. doi:10.3390/agriculture12101582
Wolters, I. A., Vlasova, O. I., and Trubacheva, L. V. (2001). Effect of Winter Wheat Precursors on Agrophysical Fertility Factors and Yield in a Moderately Wet Zone (In Russian). Agrochem. Bull. 16.
Wu, X., Tian, Z., and Guo, J. (2022). A Review of the Theoretical Research and Practical Progress of Carbon Neutrality. Sustain. Operations Comput. 3, 54–66. ISSN 2666-4127. doi:10.1016/j.susoc.2021.10.001
Yan, Q., Wu, L., Dong, F., Shuang-dui, Y., Li, F., Jia, Y., et al. (2023). Subsoil Tillage Enhances Wheat Productivity, Soil Organic Carbon and Available Nutrient Status in Dryland Fields. J. Integr. Agric. 2023, 011. doi:10.1016/j.jia.2023.06.011
Yue, K., Fornara Dario, A., Heděnec, P., Wu, Q., Peng, Y., Peng, X., et al. (2023). No Tillage Decreases GHG Emissions with No Crop Yield Tradeoff at the Global Scale. Soil Tillage Res. 228, 105643. doi:10.1016/j.still.2023.105643
Zhang, G., Zhang, Y., Zhao, D., Liu, S., Wen, X., Han, J., et al. (2023). Quantifying the Impacts of Agricultural Management Practices on the Water Use Efficiency for Sustainable Production in the Loess Plateau Region: A Meta-Analysis. Field Crops Res. 291, 108787. ISSN 0378-4290. doi:10.1016/j.fcr.2022.108787
Zhang, U., Wang, S., Wang, H., Wang, R., Wang, X., and Li, J. (2018). Crop Yield and Soil Properties of Dryland Winter Wheat-Spring Maize Rotation in Response to 10- Year Fertilization and Conservation Tillage Practices on the Loess Plateau. Field Crops Res. 225, 170–179. ISSN 0378-4290. doi:10.1016/j.fcr.2018.07.003
Keywords: no-till, conventional tillage, soil moisture content, soil water-holding capacity, dry steppe
Citation: Bondarovich A, Illiger P, Schmidt G, Ponkina E, Nugumanova A, Maulit A and Sutula M (2023) Effects of Agricultural Cropping Systems on Soil Water Capacity: The Case in Cross-Border Altai. Span. J. Soil Sci. 13:11493. doi: 10.3389/sjss.2023.11493
Received: 18 April 2023; Accepted: 10 July 2023;
Published: 02 August 2023.
Edited by:
Avelino Núñez-Delgado, University of Santiago de Compostela, SpainCopyright © 2023 Bondarovich, Illiger, Schmidt, Ponkina, Nugumanova, Maulit and Sutula. This is an open-access article distributed under the terms of the Creative Commons Attribution License (CC BY). The use, distribution or reproduction in other forums is permitted, provided the original author(s) and the copyright owner(s) are credited and that the original publication in this journal is cited, in accordance with accepted academic practice. No use, distribution or reproduction is permitted which does not comply with these terms.
*Correspondence: Almasbek Maulit, bWF1bGl0LmFsbWFzQGdtYWlsLmNvbQ==