- 1Centro Interdisciplinar de Investigación en Tecnologías Ambientales, Departamento de Edafoloxía e Química Agrícola, Facultade de Bioloxía, Universidade de Santiago de Compostela, Santiago de Compostela, Spain
- 2Centro de Ciências Agrárias, Ambientais e Biológicas (CCAAB), Universidade Federal do Recôncavo da Bahia, Cruz das Almas, Brazil
- 3Departamento de Ciências do Solo, Centro de Ciências Agrárias (CCA), Universidade Federal do Ceará, Fortaleza, Brazil
- 4Department of Soil Science, College of Agriculture Luiz de Queiroz, University of São Paulo, Escola Superior de Agricultura “Luiz de Queiroz” da Universidade de São Paulo, Piracicaba, Brazil
- 5Rede de Estacións Biolóxicas da Universidade de Santiago de Compostela (REBUSC), Estación de Bioloxía Mariña da Graña, Ferrol, Spain
Crab and soil samples were seasonally collected at four mangrove sites (Cacha Prego, CP; Ponta Grossa, PG; Ilha de Maré, IM; and Pitinga, PT) in Todos os Santos Bay, in NE Brazil. Total Cu levels in soils ranged from 2.5 to 89.4 mg kg−1, while in crab organs (muscles, hepatopancreas, and gills), Cu ranged from 20 to 1,320 mg kg−1, with the highest concentrations found in the Ilha de Maré population, where soils showed a clear Cu enrichment. Total Cu content did not explain the abnormally high Cu concentrations observed in crabs from Pitinga, where total content in soil was low. Geochemical partitioning indicated that most of the potentially bioavailable Cu was found in the oxidizable fractions, pyrite and organic matter. Pyrite oxidation during the dry season led to increased Cu bioavailability. This process was of relevance in soils of the Pitinga mangrove, where sulfide oxidation led to soil acidification and to an increase in Cu associated with the most labile fractions.
Introduction
Mangroves, which are coastal ecosystems located in transitional areas between the terrestrial and marine environments and subjected to tidal regimes, are highly productive environments associated with the provision of a wide range of ecosystem products and services (Barbier et al., 2011; Trégarot et al., 2021). Mangrove soils are characterized by pH values close to neutral, suboxic conditions on the surface and anoxic conditions in depth, and generally high contents of organic matter (OM), Fe oxyhydroxides on the surface, and Fe sulfides in depth (Otero and Macías, 2010). These properties and composition lead to a high reactivity, which promotes fixation of trace elements (Otero et al., 2010), turning these ecosystems into major sinks for pollutants (Marchand et al., 2016).
Todos os Santos Bay (BTS), the second largest bay in Brazil (Hatje and Andrade, 2009), hosts vast mangrove areas (Hadlich et al., 2008), many of them close to urban and industrial areas, oil refineries, ports, and sea terminals (Hatje et al., 2009). Previous studies have shown high concentrations of pollutants in this area, including hydrocarbons (Queiroz and Celino, 2008a, b; Silva et al., 2014) and trace elements, mostly copper (Hatje and Barros, 2012; Andrade et al., 2017). Despite pollution having been reported in the BTS (Milazzo et al., 2014; Rocha et al., 2016; Andrade et al., 2017), most of the studies carried out focused on total levels in soil and sediments, with works on bioavailability based mainly on the quantification of acid-volatile sulphides and metals extracted simultaneously (AVS-Me; Silva-Júnior et al., 2016; Brito et al., 2020). This technique has limitations with regard to the quantification of sulfides, with underestimation of concentrations in the system as well as of metallic bioavailability (Cooper and Morse, 1998; Luther, 2005), since it disregards other associations established between metals and other geochemical phases of soils/sediments (Huerta-Días and Morse, 1992; Otero and Macías, 2003). Unlike the AVS-Me technique, the sequential extraction of metals (Tessier et al., 1979; Rauret et al., 1998) allows analyzing the distribution of trace elements among the different geochemical fractions of the soil/sediment and favors a more comprehensive analysis of the mobility of each element and the processes that regulate its availability to the biota.
Copper can be found in association with different fractions, depending on geochemical conditions and the factors that control its speciation processes under conditions of higher or lower availability to the biota (Otero et al., 2000a; Chakraborty et al., 2015). Among the shellfish species extracted in the BTS, the crab Ucides cordatus is one of the main crustaceans exploited in this region (Soares et al., 2009; Mota et al., 2023) and one of the most consumed throughout the country (Pinheiro et al., 2016). It is considered a bioindicator of pollution in mangroves, as it bioaccumulates both organic pollutants and trace elements in its tissues (Nudi et al., 2007; Almeida et al., 2014; Silva et al., 2018; Ramos and Leite, 2022).
Considering the scarce information about Cu distribution among the geochemical fractions and its bioavailability, along with the importance of Ucides cordatus in the BTS, the objectives of this study were 1) to understand the geochemical behavior of this element in mangrove soils using a sequential extraction method and 2) to study the transfer of Cu to biota using the crab Ucides cordatus as a potential bioindicator species in this region.
Material and Methods
The study was carried out in four mangrove sites (Figure 1). Two of them were located at the entrance of the bay (Cacha Prego, CP; and Ponta Grossa, PG), both in more undeveloped areas; another one located in the northeast region (Ilha de Maré, IM), close to industrial areas and maritime ports, and another one in the northern region (Pitinga, PT), in the Subaé River estuary, an area with a history of metal pollution associated with an abandoned metallurgical factory (Hatje et al., 2009; Rocha et al., 2012).
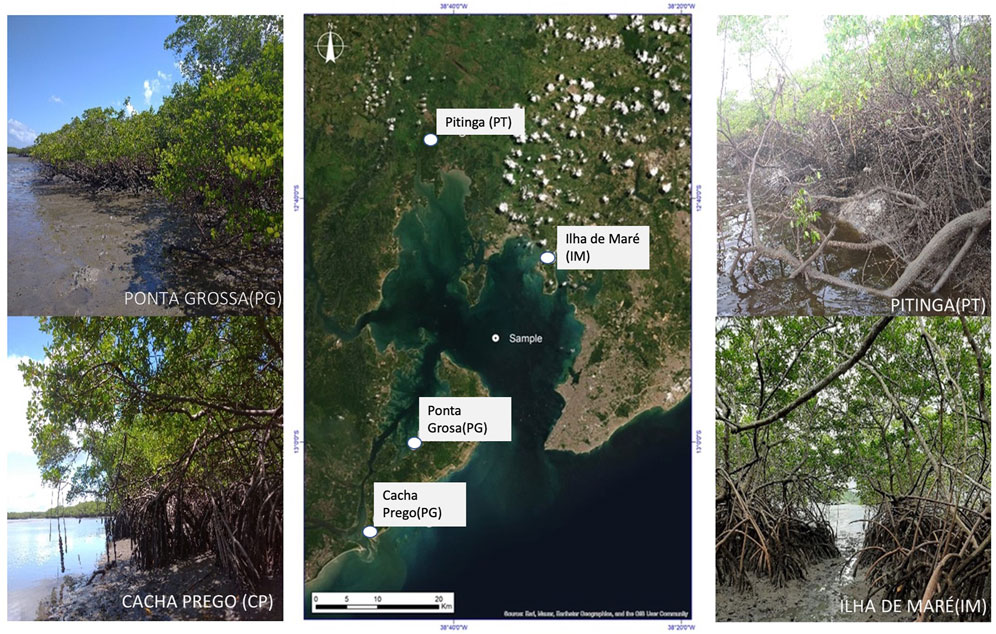
FIGURE 1. Location of sampling sites in the Todos os Santos Bay and general view of the four studied mangroves.
Mangroves in IM and CP are comprised of monospecific Rhizophora mangle (L.) forests, while PT and PG show mixed patches composed of R. mangle and Laguncularia racemosa (L.). These sites were selected to cover different sections of the BTS, thus analyzing potential spatial variations according to the degree of exposure to anthropogenic stressors, the composition of the different forests, and soil characteristics in each mangrove, both to establish baseline values and to better understand soil-to-biota transfer processes.
Twelve soil samples, each consisting of a pool of three individual samples, were collected at low tide during the dry season (DS: dry season 2020/2021) and during the rainy season (WS: wet season 2021) at depths of 0–5 cm (surface - S) and 15–30 cm (depth - D). Determinations of pH and Eh were performed in situ using a portable device (HI8424, Hanna Instruments) (Souza et al., 2007). The contents of sand and fine fraction (silt + clay) were determined by sifting through a 2 mm sieve to obtain the fine Earth fraction, then through a 0.05 mm sieve to separate the fine sand from the fine fraction (silt and clay particles) (Almeida et al., 2012. Souza et al. (2007), while total organic carbon (TOC) content was determined using an elemental analyzer (FlashEA1112, ThermoFinnigan). For TOC analysis, all soil samples had been previously subjected to acid attack using 10 mL HCl (1N) to remove carbonates (Schumacher, 2002; Nobrega et al., 2015). After adding the acid solution (HCl), samples were shaken for 1 h, then centrifuged to remove HCl, washed five times in deionized water, and dried in an oven at 60°C. All analyzes were carried out on the bulk sample, without separating the granulometric fractions.
To analyze total metal contents, 0.5 g of each sample were weighed and digested using 9 mL ultrapure nitric acid (HNO3 65%) and 3 mL ultrapure hydrochloric acid (HCl 37.5%) in a closed-vessel microwave system (Milestone; ETHOS EASY). Geochemical partitioning of Cu was performed using the BCR method (Rauret et al., 1998) and based on the sequence proposed by Tessier et al. (1979), as described below:
• Fraction 1: soluble, exchangeable fraction associated with carbonates (ExCa), extracted using 30 mL acetic acid (0.11 mol L−1; pH = 4.5) and shaking for 16 h at 25°C.
• Fraction 2: fraction associated with amorphous iron oxyhidroxides (Am), extracted using 20 mL of solution containing 20 g ascorbic acid +50 g sodium citrate +50 g bicarbonate +1 L ultrapure deoxygenated H2O (pH 8) and shaking for 24 h at 25°C.
• Fraction 3: fraction associated with crystalline iron oxyhidroxides (Cri), extracted using 20 mL of solution containing 73.925 g sodium citrate +9.24 g NaHCO3 in 1 L ultrapure H2O, adding 3 g sodium dithionite to each sample, and shaking for 30 h at 75°C.
• Fraction 4: reduced forms associated with organic matter and metal sulfides (Red), extracted using 10 mL H2O2 (8.8 M) and warming in a bath at 85°C until evaporated to 3 mL, followed by a second addition of 10 mL H2O2. Samples were kept warm in a bath at 85°C until evaporated to 1 mL, at which point 50 mL of AcNH4 solution were added and samples were shaken for 16 h at 25°C.
The residual fraction (FR) value was calculated as the difference between the total concentration after microwave digestion and the sum of the bioavailable fractions (Σ F1 -> F4).
Twelve U. cordatus specimens were collected from each mangrove, washed in distilled water, and subsequently dissected to extract muscle, hepatopancreas, and gills. Tissues were freeze-dried for 72 h, ground, and digested on a digestion block with 7 mL ultrapure HNO3 and 2 mL ultrapure H2O2. Mili-Q water was added to the digested material up to a total volume of 25 mL. Copper concentrations in each fraction, total content in soil, and contents in animal tissues were determined by inductively coupled plasma-mass spectrometry (Varian 820-MS ICP-MS). The analytical method used was validated using certified reference material for soil (SO-3, Canadian Certified Reference Material Project, Canada), leaves (NIST-1547), and animal samples (NIST-1577b, bovine liver), with recovery rates over 85%. The limit of detection for Cu was 0.005 ± 0.001 mg L−1, determined as 2.5 times the standard error of the blank (Bruland et al., 1979).
The results were analyzed using descriptive statistics (Sigmaplot 12.0), and a Kruskal-Wallis non parametric test was applied at a 5% level of significance (XLSTAT 2014) to compare the results obtained from the different study sites. This non-parametric test was selected due to its higher robustness and lower statistical assumption requirements (Reimann et al., 2008). Likewise, discriminant analysis was applied to verify possible relationships between the studied variables.
Results
Soil Attributes and Components
Texture differed significantly between Ilha de Maré (IM), where soils showed higher proportions of silt and clay (52% ± 8.6%), and the remaining three sites (Table 1). Values for pH ranged from 4.5 to 7.7, with mean soil values close to neutral and without seasonal variations except for Pitinga (PT = 4.5–7.3), where values during the dry season were lower than during the rainy season and oscillated between highly and moderately acidic (PT–DS: surface 4.8 ± 0.4; depth: 5.9 ± 0.4).
The redox potential during the dry season varied between +92 mV and +188 mV (Table 1), with mean values typical of suboxic soils in all the mangroves (generally Eh: 200–300 mV), except for IM, where conditions in the deep soil layer were predominantly anoxic (+97 ± 6 mV). During the rainy season, values varied between +30 and +155 mV, with most of the soils showing values within the suboxic range, the deep layer being predominantly anoxic in IM, PG, and CP (IM: 93 ± 27 mV; PG: 96 ± 15; CP: 90 ± 31 mV). Seasonal differences were observed in the surface and deep soil layers in IM, as well as in the deep layer in PT, with higher values during the dry season (p < 0.05).
TOC ranged between 0.8% and 6.6% (Table 1), without seasonal variations, but with significant spatial differences, with the IM site showing higher values, both during the dry (S: 6.0% ± 0.58%; D: 4.8% ± 0.3%) and the rainy season (S: 5.9% ± 1.0%; D: 4.8% ± 0.4%), compared with the remaining areas (0.8%–3.9%). Total Fe ranged from 0.1% to 2.8% and showed the highest concentrations in IM (Table 1), both during the dry (S: 2.3% ± 0.5%; D: 2.6% ± 0.1%) and the rainy season (S: 2.3% ± 0.5%; D: 2.6% ± 0.1%). Contents in CP during the rainy season (S: 1.3% ± 0.3%; D: 1.2% ± 0.2%) were also higher than those found in PT and PG for both depth levels (p < 0.05). Seasonal differences were only observed for the deep soil layer in IM and CP, where contents were higher during the rainy season (p < 0.05). Fe contents in the different fractions varied between 12.4 and 133 mg kg−1 for fraction FeExCa, 0.30–944 mg kg−1 for fraction FeAm, 115–3,035 mg kg−1 for fraction FeCri, and 85.0–9,233 mg kg−1 for fraction FeRed (Figure 2). The majority of non-residual Fe was present in the form of FeCri oxyhydroxides and in the reduced fraction, except for PT, where contents in the dry season were mostly present in the FeAm phase, followed by fractions FeCri and FeRed, with no significant differences in contents among the different fractions (p > 0.05).
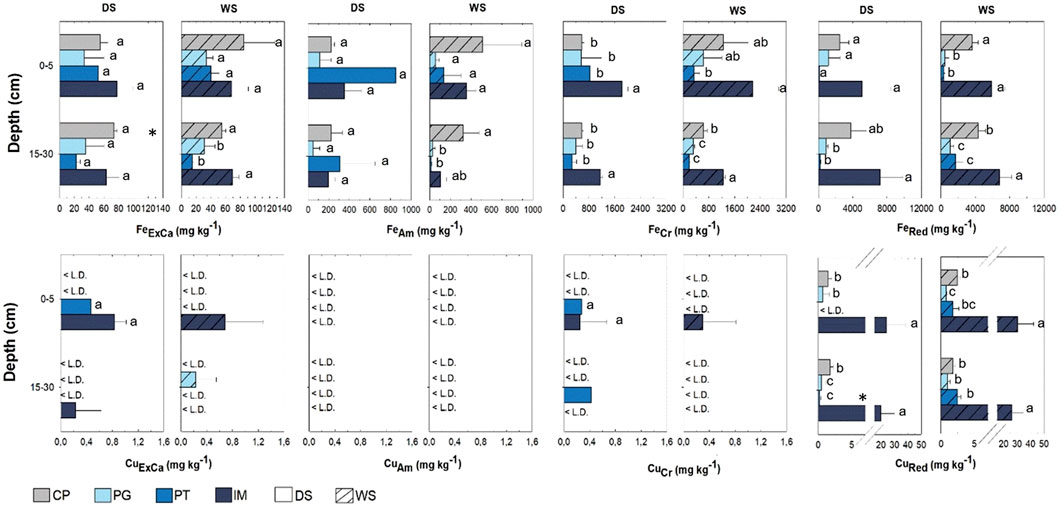
FIGURE 2. Geochemical Fe fractions in soils from mangroves in Ilha de Maré (IM), Pitinga (PT), Ponta Grossa (PG), and Cacha Prego (CP) during the dry (DS) and rainy (WS) seasons in Todos os Santos Bay. Different letters indicate spatial differences within each season, while * indicates significant seasonal differences (p < 0.05); ns: no differences.
Concentrations showed significant spatial differences for all fractions. FeExCa and FeAm contents during the rainy season were higher in the deep soil layer in IM and CP. Likewise, FeCri was higher in IM, except for the values observed in the surface soil layer during the rainy season in this area, which did not differ from the concentrations found in CP and PG (p > 0.05). FeRed was higher in IM and CP, except for the values observed in the surface soil layer during the dry season, where no significant spatial variations were found. Seasonal variations were only observed in the CP mangrove, where FeExCa concentrations at depth were higher during the dry season. In PT, even though no significant differences were detected (p < 0.05), FeRed contents during the rainy season (1,721 ± 853 mg kg−1) were higher than during the dry season (160 ± 106 mg kg−1).
Geochemical Fractionation and Bioavailability of Cu
Total Cu varied between 2.5 and 89.4 mg kg−1 and showed the highest values in IM (mean value: 52.5 ± 16.7 mg kg−1), followed by PT and CP (mean values: 5.9 ± 1.8 mg kg−1 and 4.3 ± 1.1 mg kg−1, respectively), with the lowest contents found in PG (mean value: 2.9 ± 0.4 mg kg−1) (Table 2).
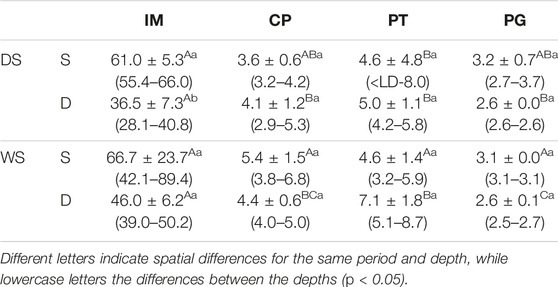
TABLE 2. Total Cu concentration in soils of Ilha de Maré (IM), Pitinga (PT), Ponta Grossa (PG), and Cacha Prego (CP) during the dry (DS) and rainy (WS) seasons in Todos os Santos Bay.
Contents showed seasonal variability in CP (only in the surface layer), IM, and PT (at a depth of 15–30 cm), with higher values during the rainy season (p < 0.05). Contents also differed according to depth, with higher contents in surface soils in IM for both seasons (p < 0.05) and higher contents at depth in PT and CP (p < 0.05) for the rainy and the dry seasons, respectively.
Contents in non-residual fractions varied between <limit of detection (LD = 0.02 mg kg−1) and 40.1 mg kg−1, without differences between depths (p > 0.05) and with the highest contents found in the oxidizable fraction (Figure 2). A different pattern was observed in PT during the dry season, when no differences were observed among CuExCa, CuCri, and CuRed concentrations (p > 0.05). CuExCa contents during the dry season were detected only in PT (<0.02–0.5 mg kg−1) and IM (<0.02–1.0 mg kg−1), while during the rainy season, values above the LD were found in IM (<0.02–1.1 mg kg−1) and in PG (<0.05–0.5 mg kg−1). CuCri was only detected in PT during the dry season (<0.02–0.9 mg kg−1) and in IM for both seasons (DS: <0.02–0.7 mg kg−1; WS: <0.02–0.9 mg kg−1), with CuAm values below the limit of detection in all mangroves.
Copper concentrations in the oxidizable fraction showed spatial variations, with the highest values observed in IM, both during the dry (S: 24.1 ± 13.8 mg kg−1; D: 19.7 ± 10.3 mg kg−1) and the rainy season (S: 30.3 ± 11.9 mg kg−1; D: 26.1 ± 8.5 mg kg−1). Seasonal variations were only observed in PT, where the CuRed contents found at depth were significantly higher during the rainy season (DS: 0.15 ± 0.21 mg kg−1; WS: 2.41 ± 0.51 mg kg−1).
Cu contents in muscle of Ucides cordatus (Figure 3) oscillated between 33.4 and 104.2 mg kg−1 and did not show a common pattern regarding seasonality, with higher contents during the rainy season in PT (p < 0.05) and during the dry season in CP (p < 0.05), with no significant differences detected in the remaining areas. Values in hepatopancreas ranged between 20.2 and 1,133 mg kg−1, with significant seasonal variability (p < 0.05) and with the highest contents found during the dry season. Concentrations found in the gills varied between 76.8 and 1,320 mg kg−1, with seasonal variations in IM and with the highest contents also found in the dry season (p < 0.05). Spatial variability in concentrations was observed within the three types of tissue, with the highest contents found in IM and PT (p < 0.05).
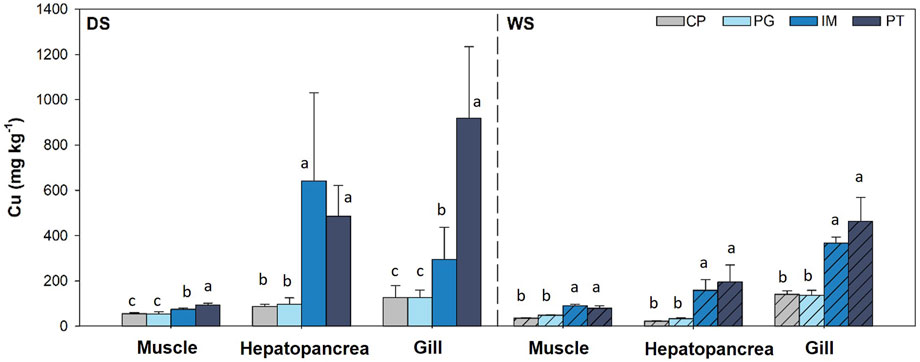
FIGURE 3. Cu concentrations in muscle, hepatopancreas, and gills of Ucides cordatus. DS: dry season, WS: rainy season. For the same stat ion (DS, WS) and the same organ, different letters indicate significant differences (p < 0.05).
Discriminant analysis of mangroves (Figure 4) based on physicochemical parameters of soils and on Cu contents revealed that factors 1 and 2 explained 93.9% of data variability. Axis 1 of the discriminant analysis differentiated between IM and the remaining areas based on its lower total Fe and Cu, TOC, and CuRed contents, while axis 2 discriminated among mangroves in CP, PG, and PT, with the highest pH values associated with CP and the highest Eh values observed in PT. Moreover, positive correlations were detected among contents of TOC, FeRed, and crystalline oxides, as well as between CuTotal and Cu in the exchangeable fraction (CuExCa) and between CuTotal and reduced sulfur forms and organic matter (CuRed).
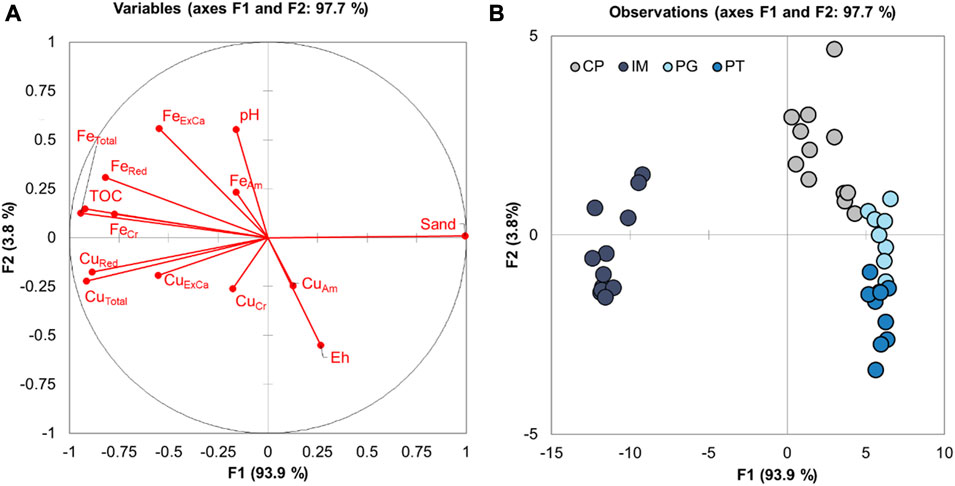
FIGURE 4. Discriminant function analysis of the mangroves in Pitinga (PT), Cacha Prego (CP), Ilha de Maré (IM), and Ponta Grossa (PG) based on soil parameters, considering factors F1 and F2, which explain 93.9% of data variability. ExCa: metal (Fe, Cu) exchangeable fraction; Am: metal associated to amorphous iron oxyhidroxides; Cr: metal associated to cristalline Fe oxyhydroxides; Red: metal associated to the oxidable fraction (further details see Material and Methods section).
Discussion
The results of the discriminant analysis indicate hydrodynamic differences among mangroves, with higher sedimentation of organic matter, silt, clay and Fe oxides and hydroxides in lower energy environments such as IM (Lessa et al., 2000; Hatje and Andrade, 2009). Mangroves exhibited spatial variability, both in terms of soil parameters and of Cu contents in soils and biota. Except for PT, mean pH values were close to neutral, similarly to the results found in other study areas (Otero et al., 2017; Araújo et al., 2022), and redox conditions varied between anoxic (deep soil layers in IM, PG, and CP) and suboxic, within the commonly observed range in northeastern mangroves (Bomfim et al., 2015; Ferreira et al., 2022). A different temporal pattern was observed in PT, with an effect of seasonality on pH and redox potential, showing highly acidic (pH < 5) and neutral values during the dry and the rainy season, respectively, and with significantly higher redox conditions during the dry season. This seasonal behavior may be associated with its more sandy texture and higher relief with respect to the riverbed, factors that could promote faster drainage during drought periods and higher levels of soil aeration (Du Laing et al., 2009; Ferreira et al., 2022).
The seasonal pattern observed in this mangrove influenced geochemical partition of Fe and Cu, with higher contents in reduced fractions observed during the rainy season, consistently with the lower Eh values (Otero et al., 2000a; Otero et al., 2000b; Thanh-Nho et al., 2019). The more reduced conditions observed during this period may have promoted reduction of Fe and precipitation of sulfides, mainly pyrite (Berner et al., 1985; Huerta-Díaz and Morse, 1992; Otero et al., 2009), as well as gradual Cu pyritization (Huerta-Díaz and Morse, 1992; Morse and Luther, 1999). Cu contents in more mobile phases, such as Cu associated with CuExCa fractions or easily reducible Cu (CuCr), were only detected during the dry season, consistently with sulfide oxidation during this period, leading to soil acidification and to higher Cu solubility (Otero et al., 2000b; Machado et al., 2014; Araújo-Júnior et al., 2016). IM soils showed significantly higher total Cu contents than the remaining areas, and the values were above the background value for the BTS proposed by the Bahia Environmental Resources Center (Centro de Recursos Ambientais da Bahia, CRA) (23.0 ± 5.2 mg kg−1, CRA, 2004), as well as above the legally established preventative threshold in Brazil (60 mg kg−1, CONAMA, 2009), above the TEL (threshold effects level, or the minimum threshold for potential risk of toxic effects to biota: 18.7 mg kg−1), and above the ERL (effects range low: 34.0 mg kg−1) established by NOAA et al. (1999). These results are related to the location of the IM mangrove, close to the Port of Aratu, in Aratu Bay, through which a wide variety of products are transported, including fertilizers and Cu concentrates associated with the mineral exploitation of copper sulfides, thus leading to abnormally high Cu concentrations in this bay and its environment (Rocha et al., 2016; Andrade et al., 2017).
Additionally, the location of this mangrove in the middle section of the BTS implies that hydraulic energy conditions are low (Lessa et al., 2000), thus promoting the deposit of fine particles and, consequently, the accumulation of metals (Kumar and Ramanathan, 2015; Bastakoti et al., 2018). The results from partitioning demonstrated that the majority of Cu is found in the oxidizable fraction, consistently with the high tendency of Cu to form stable complexes with organic matter, even forming strong complexes in reducing conditions (Zhou et al., 2010; Chakraborty et al., 2015), or/and to precipitate as sulfides in sulfate-reducing environments (Huerta-Díaz and Morse, 1992; Otero et al., 2000a; Otero et al., 2000b; Thanh-Nho et al., 2019). IM soils showed a higher concentration of Fe and Cu associated with the oxidizable fraction than the other mangroves. However, seasonal changes in redox status may interfere with the stability of this fraction and its role as a metal sink, since pyrite can undergo oxidative dissolution, thus releasing a major portion of the adsorbed elements into more labile phases (Otero and Macías, 2002; Araújo Júnior et al., 2016). However, our results, despite showing a lower percentage of Fe and Cu associated with the reducible fraction, did not show any significant seasonal changes (Figure 2). More specifically, factors such as local flooding patterns and activity of the biota may promote sulfide oxidization and Cu release in a readily available form (Machado et al., 2014; Araújo-Júnior et al., 2016). Likewise, oxygen flow through the aerenchyma of mangrove plants into the rhizosphere substantially affects redox conditions, promoting soil aeration and, consequently, the system’s evolution towards oxic environments (Pi et al., 2009; Sarker et al., 2021).
Consistently with Cu values in mangrove soils, high concentrations were detected in U. cordatus tissues in IM, mainly in the gills and hepatopancreas and during the dry season, which suggests higher Cu bioavailability in this area and during this season. The higher Eh values recorded for this period are consistent with higher soil aeration levels leading to sulfide oxidation and system acidification, with increased Cu bioavailability (Otero et al., 2000a). Moreover, the greater activity levels of U. cordatus during the dry season, related to its breeding period (Ferreira et al., 2007b; Pinheiro et al., 2016), could have promoted higher levels of burrowing activity (bioturbation) and, consequently, greater soil aeration, with an impact on biogeochemical conditions and on the distribution of metals among fractions (Ferreira et al., 2007a; Araújo-Júnior et al., 2016), which could also have contributed to higher Cu bioavailability and its consequent assimilation. High Cu concentrations were also found in crustaceans collected in PT, again in the gills and hepatopancreas and mainly during the dry season, although total contents in soils were low. Moreover, values in the more mobile fractions were highest during the dry season, related to higher Eh values, which suggests a higher bioavailability during this period (Otero et al., 2000a; Araújo-Júnior et al., 2016). The results observed in this mangrove suggest that the risks associated with bioavailability are mainly likned to biogeochemical processes in soils controlling metal partition instead of being only related to total contents in soils, with contents in the more labile fractions acting as better environmental indicators than total metal contents with respect to the risk of bioaccumulation.
The high values observed both in gills and in hepatopancreas are consistent with other studies (Vedolin et al., 2020; Ramos and Leite, 2022), that have also demonstrated a tendency towards a high accumulation of Cu in these tissues in environments with high exposure to metals. Firat et al. (2008), in Iskenderun Bay, an area with an intense agricultural and industrial activity, found high Cu concentrations in gills (827 ± 92.6 µg g−1) and in hepatopancreas (935 ± 50.5 μg g−1) in the crab Charybdis longicolis, with a distribution pattern and concentrations similar to those observed in IM and PT. Cu is present in the pigment hemocyanin and is required in small concentrations for the physiological processes associated with breathing (Taylor and Anstiss, 1999), and for this reason, it is found in greater amounts in branchial tissue. Excess Cu, due to its toxic potential, is usually detoxified by these organisms in the hepatopancreas in order to avoid its presence in high concentrations in a metabolically active condition in the body (Rainbow, 2002).
In summary, the presence of total Cu at concentrations above background levels and the high concentrations found in Ucides cordatus tissues in IM suggest a clear enrichment of this metal, which increases its bioavailability. However, the abnormally high Cu concentrations in crab tissues in PT indicate that total content is not a suitable proxy to assess Cu bioavailability in these intertidal systems (Otero et al., 2000a) and that bioturbation and seasonal changes substantially affect geochemical conditions in soils (Araújo-Júnior et al., 2016; Ferreira et al., 2021), thus promoting environmental acidification and increased concentrations of Cu associated with the most labile and bioavailable fractions during the dry season.
Although a large proportion of Cu is associated with the oxidizable fraction, seasonal factors associated with biota and physicochemical parameters of soils could compromise the stability of geochemical fractions, making this metal available to resident fauna. On the other hand, contents detected in crabs from IM and PT suggest potential ecotoxicological risks in these mangroves; this warrants the need to assess the consumption of Ucides cordatus and its associated impacts on human and environmental health in these areas.
Data Availability Statement
The raw data supporting the conclusion of this article will be made available by the authors, without undue reservation.
Ethics Statement
Ethical approval was not required for the study involving animals in accordance with the local legislation and institutional requirements.
Author Contributions
Conceptualization: MR, GN, and XO. Methodology: MR, GN, and XO. Formal analysis: all authors. Writing—original draft preparation: all authors. All authors contributed to the article and approved the submitted version.
Funding
This research was co-funded by the Conselho Nacional de Desenvolvimento Científico e Tecnológico (CNPq) do Ministério da Ciência, Tecnologias e Inovações do Brasil, Project titled “Desenvolvimento do Índice de Qualidade das Florestas de manguezais na Baía de Todos Santos (BTS) Bahia”, (no. 441389/2017-1), and the Consellería de Educación, Universidade e Formación Profesional Xunta de Galicia (Axudas á consolidación e estruturación de unidades de investigación competitivas do SUG do Plan Galego IDT, Ambiosol Group ref. ED431C; [2022/40]).
Conflict of Interest
The authors declare that the research was conducted in the absence of any commercial or financial relationships that could be construed as a potential conflict of interest.
Acknowledgments
Thanks are due to María José Santiso for her assistance with laboratory work.
References
Almeida, B. G., Donagemma, G. K., Ruiz, H. A., Braida, J. A., Viana, J. H. M., Reichert, J. M. M., et al. (2012). Padronização de Métodos para Análise Granulométrica no Brasil. EMBPARA, 1–11.
Almeida, E. V., Kütter, V. T., and Silva-Filho, E. V. (2014). “Metais traço em caranguejos de mangue Ucides cordatus (L., 1763) Do leste da baía de Guanabara (Sudeste Do Brasil),” in Procesos Geoquímicos Superficiales en Iberoamérica. Editors J. E. Marcovecchio, S. E. Botté, and R. H. Freije (Bahía Blanca: Sociedad Iberoamericana de Física y Química Ambiental), 243–260.
Andrade, R. L. B., Hatje, V., Masqué, P., Zurbrick, C. M., Boyle, E. A., and Santos, W. P. C. (2017). Chronology of Anthropogenic Impacts Reconstructed from Sediment Records of Trace Metals and Pb Isotopes in Todos Os Santos Bay (NE Brazil). Mar. Pollut. Bull. 125, 459–471. doi:10.1016/j.marpolbul.2017.07.053
Araújo Júnior, J. M. C., Ferreira, T. O., Suarez-Abelenda, M., Nóbrega, G. N., Albuquerque, A. G. B. M., Bezerra, A. C., et al. (2016). The Role of Bioturbation by Ucides Cordatus Crab in the Fractionation and Bioavailability of Trace Metals in Tropical Semiarid Mangroves. Mar. Pollut. Bull. 111, 194–202. doi:10.1016/j.marpolbul.2016.07.011
Araújo, P. R. M., Biondi, C. M., Nascimento, C. W. A., Silva, F. B. V., Ferreira, T. O., and Alcântara, S. F. (2022). Geospatial Modeling and Ecological and Human Health Risk Assessments of Heavy Metals in Contaminated Mangrove Soils. Mar. Pollut. Bull. 177, 113489. doi:10.1016/j.marpolbul.2022.113489
Barbier, E. B., Hacker, S. D., Kennedy, C., Koch, E. W., Stier, A. C., and Silliman, B. R. (2011). The Value of Estuarine and Coastal Ecosystem Services. Ecol. Monogr. 81, 169–193. doi:10.1890/10-1510.1
Bastakoti, U., Robertson, J., and Alfaro, A. C. (2018). Spatial Variation of Heavy Metals in Sediments within a Temperate Mangrove Ecosystem in Northern New Zealand. Mar. Pollut. Bull. 135, 790–800. doi:10.1016/j.marpolbul.2018.08.012
Berner, R. A., Leeuw, J. W., Spiro, B., Murchison, D. G., and Eglinton, G. (1985). Sulphate Reduction, Organic Matter Decomposition and Pyrite Formation. Phil. Trans. R. Soc. Lond. A 315, 25–38.
Bomfim, M. R., Santos, J. A. G., Costa, O. V., Otero, X. L., Boas, G. S. V., Capelão, V. S., et al. (2015). Genesis, Characterization, and Classification of Mangrove Soils in the Subaé River Basin, Bahia, Brazil. Solo 39, 1247–1260. doi:10.1590/01000683rbcs20140555
Brito, G. B., Silva-Júnior, J. B., Dias, L. C., Santos, A. S., Hadlich, G. M., and Ferreira, S. L. C. (2020). Evaluation of the Bioavailability of Potentially Toxic Metals in Surface Sediments Collected from a Tropical River Near an Urban Área. Mar. Pollut. Bull. 156, 111215. doi:10.1016/j.marpolbul.2020.111215
Bruland, K. W., Franks, R. P., Knauer, G., and Martin, J. H. (1979). Sampling and Analytical Methods for the Determination of Copper, Cadmium, Zinc, and Nickel at the Nanogram Per Liter Level in Sea Water. Anal. Chim. Acta 105, 233–245. doi:10.1016/S0003-2670(01)83754-5
Centro de Recursos Ambientais - CRA (2004). “Diagnóstico da concentração de metais pesados e hidrocarbonetos de petróleo nos sedimentos e biota da Baía de Todos os Santos,” in Consórcio BTS Hydros CH2MHILL (Salvador: Governo do Estado da Bahia).
Chakraborty, P., Ramteke, D., and Chakraborty, S. (2015). Geochemical Partitioning of Cu and Ni in Mangrove Sediments: Relationships with Their Bioavailability. Mar. Pollut. Bull. 93, 194–201. doi:10.1016/j.marpolbul.2015.01.016
Conselho Nacional do Meio Ambiente – CONAMA (2009). Resolução no 420, de 28 de dezembro de 2009. Dispõe sobre critérios e valores orientadores de qualidade do solo quanto à presença de substâncias químicas e estabelece diretrizes para o gerenciamento ambiental de áreas contaminadas por essas substâncias em decorrência de atividades antrópicas. Brasília: The National Council for the Environment.
Cooper, D. C., and Morse, J. W. (1998). Extractability of Metal Sulfide Minerals in Acidic Solutions: Application to Environmental Studies of Trace Metal Contamination within Anoxic Sediments. Environ. Sci. Technol. 32, 1076–1078. doi:10.1021/es970415t
Du Laing, G., Rinklebe, J., Vandecasteele, B., Meers, E., and Tack, F. M. G. (2009). Trace Metal Behaviour in Estuarine and Riverine Floodplain Soils and Sediments: A Review. Sci. Total Environ. 407, 3972–3985. doi:10.1016/j.scitotenv.2008.07.025
Ferreira, T. O., Nóbrega, G. N., Queiroz, H. M., Souza-Júnior, V. S., Barcellos, D., Ferreira, A. D., et al. (2021). Windsock Behavior: Climatic Control on Iron Biogeochemistry in Tropical Mangroves. Biogeochemistry 156, 437–452. doi:10.1007/s10533-021-00858-9
Ferreira, T. O., Otero, X. L., Vidal-Torrado, P., and Macías, F. (2007b). Effects of Bioturbation by Root and Crab Activity on Iron and Súlfur Biogeochemistry in Mangrove Substrate. Geoderma 142, 36–46. doi:10.1016/j.geoderma.2007.07.010
Ferreira, T. O., Otero, X. L., Vidal-Torrado, P., and Macías, F. (2007a). Redox Processes in Mangrove Soils under Rhizophora Mangle in Relation to Different Environmental Conditions. Soil Sci. Soc. Am. J. 71, 484–491. doi:10.2136/sssaj2006.0078
Ferreira, T. O., Queiroz, H. M., Nóbrega, G. N., Souza Júnior, V. S., Barcellos, D., Ferreira, A. D., et al. (2022). Litho-climatic Characteristics and its Control over Mangrove Soil Geochemistry: A Macro-Scale Approach. Sci. Total Environ. 811, 152152. doi:10.1016/j.scitotenv.2021.152152
Firat, Ö., Gök, G., Çoğun, H. Y., Yüzereroğlu, T. A., and Kargin, F. (2008). Concentrations of Cr, Cd, Cu, Zn and Fe in Crab Charybdis Longicollis and Shrimp Penaeus Semisulcatus from the Iskenderun Bay, Turkey. Environ. Monit. Assess. 147, 117–123. doi:10.1007/s10661-007-0103-7
Hadlich, G. M., Ucha, J. M., and Celino, J. J. (2008). “Apicuns na Baía de Todos os Santos: distribuição espacial, descrição e caracterização física e química,” in Avaliação de ambientes na Baía de Todos os Santos: aspectos geoquímicos, geofísicos e biológicos. Editor A. F. S. Celino, and J. J. Queiroz (Salvador: UFBA: ResearchGate), 59–72. (Coords).
Hatje, V., and Andrade, J. B. (2009). Baía de Todos os Santos: aspectos oceanográficos. Salvador: EDUFBA.
Hatje, V., and Barros, F. (2012). Overview of the 20th Century Impact of Trace Metal Contamination in the Estuaries of Todos Os Santos Bay: Past, Present and Future Scenarios. Mar. Pollut. Bull. 64, 2603–2614. doi:10.1016/j.marpolbul.2012.07.009
Hatje, V., Bícego, M. C., Carvalho, G. C., and Andrade, J. B. (2009). “Contaminação Química,” in Baia de Todos os Santos: aspectos oceanográficos. Editor V. J. B. HatjeAndrade (Salvador: EDUFBA).
Huerta-Díaz, M. A., and Morse, J. W. (1992). Pyritization of Trace Metals in Anoxic Marine Sediments. Geochimica et Cosmochimica Acta 56, 2681–2702. doi:10.1016/0016-7037(92)90353-k
Kumar, A., and Ramanathan, A. (2015). Speciation of Selected Trace Metals (Fe,Mn, Cu and Zn) with Depth in the Sediments of Sundarban Mangroves: India and Bangladesh. J. Soils Sediments 15, 2476–2486. doi:10.1007/s11368-015-1257-5
Lessa, G. C., Bittencourt, A. C. S. P., Brichta, A., and Dominguez, J. M. L. (2000). A Reevaluation of the Late Quaternary Sedimentation in Todos Os Santos Bay (BA), Brazil. An. Acad. Bras. Ciênc. 72 (4), 573–590. doi:10.1590/s0001-37652000000400008
Luther, G. W. (2005). Acid Volatile Sulfide — A Comment. Mar. Chem. 97, 198–205. doi:10.1016/j.marchem.2005.08.001
Machado, W., Borrelli, N. L., Ferreira, T. O., Marques, A. G. B., Osterrieth, M., and Guizan, C. (2014). Trace Metal Pyritization Variability in Response to Mangrove Soil Aerobic and Anaerobic Oxidation Processes. Mar. Pollut. Bull. 79, 365–370. doi:10.1016/j.marpolbul.2013.11.016
Marchand, C., Fernandez, J.-M., and Moreton, B. (2016). Trace Metal Geochemistry in Mangrove Sediments and Their Transfer to Mangrove Plants (New Caledonia). Sci. Total Environ. 562, 216–227. doi:10.1016/j.scitotenv.2016.03.206
Milazzo, A. D. D., Silva, A. C. M., Oliveira, D. A. F., and Cruz, M. J. M. (2014). The Influence of Seasonality (Dry and Rainy) on the Bioavailability and Bioconcentration of Metals in an Estuarine Zone. Estuarine Coast. Shelf Sci. 149, 143–150. doi:10.1016/j.ecss.2014.08.013
Morse, J. W., and Luther, G. W. (1999). Chemical Influences on Trace Metal-Sulfide Interactions in Anoxic Sediments. Geochimica Cosmochimica Acta 63, 3373–3378. doi:10.1016/s0016-7037(99)00258-6
Mota, T. A., Pinheiro, M. A. A., Evangelista-Barreto, N. S., and Rocha, S. S. (2023). Density and Extractive Potential of “Uçá”-crab, Ucides Cordatus (Linnaeus, 1763), in Mangroves of the “Todos Os Santos” Bay, Bahia, Brazil. Fish. Res. 265, 106733. doi:10.1016/j.fishres.2023.106733
NOAA; National Oceanic and Atmospheric Administration (1999). Screening Quick Reference Tables. Seattle, USA: National Oceanic and Atmospheric Administration, 12.
Nóbrega, G. N., Ferreira, T. O., Artur, A. G., Mendonça, E. S., Leão, R. A. O., Teixeira, A. S., et al. (2015). Evaluation of Methods for Quantifying Organic Carbon in Mangrove Soils from Semi-Arid Region. J. Soils Sediments 15, 282–291. doi:10.1007/s11368-014-1019-9
Nudi, A. H., Wagener, A. L. R., Francioni, E., Scofield, A. L., Sette, C. B., and Veiga, A. (2007). Validation of Ucides Cordatus as a Bioindicator of Oil Contamination and Bioavailability in Mangroves by Evaluating Sediment and Crab PAH Records. Environ. Int. 33, 315–327. doi:10.1016/j.envint.2006.11.001
Otero, X. L., Ferreira, T. O., Huerta-Díaz, M. A., Partiti, C. S. M., Souza, V., Vidal-Torrado, P., et al. (2009). Geochemistry of Iron and Manganese in Soils and Sediments of a Mangrove System, Island of Pai Matos (Cananeia — SP, Brazil). Geoderma 148, 318–335. doi:10.1016/j.geoderma.2008.10.016
Otero, X. L., Huerta-Díaz, M. A., and Macías, F. (2000b). Heavy Metal Geochemistry of Saltmarsh Soils from the Ria of Ortigueira (Mafic and Ultramafic Areas, NW Iberian Peninsula). Environm. Pollut. 110, 285–296. doi:10.1016/s0269-7491(99)00302-4
Otero, X. L., and Macías, F. (2010). Biogeochemistry and Pedogenetic Processes in Mangrove and Saltmarsh Systems (New York: Nova Science Publisher).
Otero, X. L., and Macías, F. (2003). Spatial Variation in Pyritization of Trace Metals in Salt-Marsh Soils. Biogeochemistry 62, 59–86. doi:10.1023/a:1021115211165
Otero, X. L., and Macías, F. (2002). Variation with Depth and Season in Metal Sulfides in Salt Marsh Soils. Biogeochemistry 61, 247–268. doi:10.1023/a:1020230213864
Otero, X. L., Méndez, A., Nóbrega, G. N., Ferreira, T. O., Meléndez, W., and Macías, F. (2017). High Heterogeneity in Soil Composition and Quality in Different Mangrove Forests of Venezuela. Environ. Monit. Assess. 189, 511. doi:10.1007/s10661-017-6228-4
Otero, X. L., Sánchez, J. M., and Macías, F. (2000a). Bioaccumulation of Heavy Metals in Thionic Fluvisols by a Marine Polychaete: the Role of Metal Sulfides. J. Environ. Qual. 29, 1133–1141. doi:10.2134/jeq2000.00472425002900040014x
Otero, X. L., Souza, M. L., and Macías, F. (2010). “Iron and Trace Metals Geochemistry in Mangrove Soils,” in Pedogenetic Processes in Mangrove Soils. Editors X. L. Otero, and F. Macías (New York: Nova Science Publishers), 25–50.
Pi, N., Tam, N. F. Y., Wu, Y., and Wong, M. H. (2009). Root Anatomy and Spatial Pattern of Radial Oxygen Loss of Eight True Mangrove Species. Aquat. Bot. 90, 222–230. doi:10.1016/j.aquabot.2008.10.002
Pinheiro, M. A. A., Santos, L. C. M., Souza, C. A., João, M. C. A., Neto, J. D., and Ivo, C. T. C. (2016). “Avaliação Do Caranguejo-Uçá (Ucides Cordatus) (Linnaeus, 1763) (Decapoda: Ucididae),” in Livro vermelho dos crustáceos do Brasil. Avaliação 2010–2014. Sociedade Brasileira de Carcinologia, Porto Alegre. Editors M. A. A. Pinheiro, and H. Boos (Porto Alegre: Sociedade Brasileira de Carcinologia), 441–456.
Queiroz, A. F. S., and Celino, J. J. (2008a). Impacto ambiental da indústria petrolífera em manguezais da região norte da Baía de Todos os Santos (Bahia, Brasil). Bol. Parana. Geociências 62, 23–34. doi:10.5380/geo.v62i0.4278
Queiroz, A. F. S., and Celino, J. J. (2008b). “Manguezais e ecossistemas marinhos da Baía de Todos os Santos,” in Avaliação de ambientes na Baía de Todos os Santos: aspectos geoquímicos, geofísicos e biológicos. Editors A. F. S. Queiroz, and J. J. Celino (Salvador: UFBA), 59–72. (Coords).
Rainbow, P. S. (2002). Trace Metal Concentrations in Aquatic Invertebrates: Why and So what? Environ. Pollut. 120, 497–507. doi:10.1016/s0269-7491(02)00238-5
Ramos, R. J., and Leite, G. R. (2022). Disposition of Trace Elements in the Mangrove Ecosystem and Their Effects on Ucides Cordatus (Linnaeus, 1763) (Crustacea, Decapoda). Biometals 35, 853–873. doi:10.1007/s10534-022-00408-2
Rauret, G., López-Sánchez, J. F., Sahuquillo, A., Rubio, R., Davidson, C., Ureb, A., et al. (1998). Improvement of the BCR Three Step Sequential Extraction Procedure Prior to the Certification of New Sediment and Soil Reference Materials. J. Environ. Monit. 1, 57–61. doi:10.1039/a807854h
Reimann, C., Filzmoser, P., Garrett, R. G., and Dutter, R. (2008). Statistical Data Analysis Explained, Statistical Data Analysis Explained: Applied Environmental Statistics with R. Chichester, UK: John Wiley & Sons, Ltd.
Rocha, G. O., Guarieiro, A. L. N., Andrade, J. B., Eça, G. F., Aragão, N. M., Aguiar, R. M., et al. (2012). Contaminação na Baía de Todos os Santos. Rev. Virtual Quím. 5, 583–610.
Rocha, T. S., Sales, E. A., Beretta, M., and Oliveira, I. B. (2016). Effects of Dredging at Aratu Port in All Saints Bay, Brazil: Monitoring the Metal Content in Water and Sediments. Environ. Monit. Assess. 188, 394. doi:10.1007/s10661-016-5396-y
Sarker, S., Masud-Ul-Alam, M., Hossain, M. S., Chowdhury, S. R., and Sharifuzzaman, S. M. (2021). A Review of Bioturbation and Sediment Organic Geochemistry in Mangroves. Geol. J. 56, 2439–2450. doi:10.1002/gj.3808
Schumacher, B. A. (2002). “Methods for the Determination of Total Organic Carbon (TOC) in Soils and Sediments,” in Ecological Risk Assessment Support Center. Washington, DC: U.S. EPA.
Silva, B. M. S., Morales, G. P., Gutjahr, A. L. N., Faial, K. C. F., and Carneiro, B. S. (2018). Bioacumulation of Trace Elements in the Crab Ucides Cordatus (Linnaeus, 1763) from the Macrotidal Mangrove Coast Region of the Brazilian Amazon. Environ. Monit. Assess. 190, 214. doi:10.1007/s10661-018-6570-1
Silva, S. M. T., Beretta, M., and Tavares, T. M. (2014). Diagnóstico da Contaminação por Hidrocarbonetos Policíclicos Aromáticos nos Sedimentos de Mesolitoral da Baía de Todos os Santos, Brasil. Gesta 2, 193–204. doi:10.9771/gesta.v2i2.10449
Silva-Júnior, J. B., Nascimento, R. A., Oliva, S. T., Oliveira, O. M. C., and Ferreira, S. L. C. (2016). Bioavailability Assessment of Toxic Metals Using the Technique “Acid-volatile Sulfide (AVS)-simultaneously Extracted Metals (SEM)” in Marine Sediments Collected in Todos Os Santos Bay, Brazil. Environ. Monit. Assess. 188, 554. doi:10.1007/s10661-016-5562-2
Soares, L. S. H., Salles, A. C. R., Lopez, J. P., Muto, E. Y., and Giannini, R. (2009). “Pesca e produção pesqueira,” in Baia de Todos os Santos: aspectos oceanográficos. Editor V. Andrade, and J. B. Hatje (Salvador: EDUFBA). (Org).
Souza, V. S., Vidal-Torrado, P., Tessler, M. G., Pessenda, L. C. R., Ferreira, T. O., Otero, X. L., et al. (2007). Evoluccao Quaternaria, Distribuiccao de Particulas nos solos e Ambientes de Sedimentacao em Manguezais do Estado de Sao Paulo. Rev. Bras. Cienc. Solo 31, 753–769.
Taylor, H. H., and Anstiss, J. M. (1999). Copper and Haemocyanin Dynamics in Aquatic Invertebrates. Mar. Freshw. Res. 50, 907–931. doi:10.1071/mf99117
Tessier, A., Campbell, P. G. C., and Bisson, M. (1979). Sequential Extraction Procedure for the Speciation of Particulate Trace Metals. Anal. Chem. 51, 844–851. doi:10.1021/ac50043a017
Thanh-Nho, N., Marchand, C., Strady, E., Vinh, T., and Nhu-Trang, T. (2019). Metals Geochemistry and Ecological Risk Assessment in a Tropical Mangrove (Can Gio, Vietnam). Chemosphere 219, 365–382. doi:10.1016/j.chemosphere.2018.11.163
Trégarot, E., Cailland, A., Cornet, C. C., Taureau, F., Catry, T., Cragg, S. M., et al. (2021). Mangrove Ecological Services at the Forefront of Coastal Change in the French Overseas Territories. Sci. Total Environ. 763, 143004. doi:10.1016/j.scitotenv.2020.143004
Vedolin, M. C., Trevizani, T. H., Angeli, J. L. F., Petti, M. A. V., and Figueira, R. C. L. (2020). Assessment of Metal Concentration in Goniopsis Cruentata (Latreille,1803) (Decapoda, Grapsidae) from Two Brazilian Mangroves under Different Anthropogenic Influences. Regional Stud. Mar. Sci. 36, 101305. doi:10.1016/j.rsma.2020.101305
Keywords: geochemistry, Cu partitioning, bioaccumulation, mangrove forest, Brazil
Citation: Ramos MAV, Nóbrega GN, Ferreira TO and Otero XL (2023) Impact of Seasonality on Copper Bioavailaility to Crabs (Ucides cordatus, Linnaeus, 1763) in Mangrove Soils of Todos os Santos Bay (Bahia, North Eastern Brazil). Span. J. Soil Sci. 13:11737. doi: 10.3389/sjss.2023.11737
Received: 24 June 2023; Accepted: 27 July 2023;
Published: 30 August 2023.
Edited by:
Avelino Núñez-Delgado, University of Santiago de Compostela, SpainCopyright © 2023 Ramos, Nóbrega, Ferreira and Otero. This is an open-access article distributed under the terms of the Creative Commons Attribution License (CC BY). The use, distribution or reproduction in other forums is permitted, provided the original author(s) and the copyright owner(s) are credited and that the original publication in this journal is cited, in accordance with accepted academic practice. No use, distribution or reproduction is permitted which does not comply with these terms.
*Correspondence: Xosé L. Otero, eGwub3Rlcm9AdXNjLmVz