- 1Area de Edafoloxía e Química Agrícola, Departamento de Bioloxía Vexetal e Ciencia do Solo, Facultade de Ciencias, Universidade de Vigo, Ourense, Spain
- 2Misión Biológica de Galicia del Consejo Superior de Investigaciones Científicas (MBG-CSIC), Santiago de Compostela, Spain
- 3Centre de Ciência i Tecnologia Forestal de Catalunya, Solsona, Spain
- 4BIOPOLIS Program in Genomics, Biodiversity and Land Planning, CIBIO/InBIO (Research Centre in Biodiversity and Genetic Resources), Vairão, Portugal
Wildfires are recognized as major contributors to forest loss and soil degradation on a global scale. Understanding the cumulative effects of fire regimes on forest ecosystems and soil dynamics necessitates a deeper exploration of wildfire-vegetation-soil interactions over the long term. This study delves into the wildfire-landscape dynamics within the “Baixa Limia Serra do Xurés” Natural Park, a region prone to fires in Galicia, Spain. By analyzing available statistical and remote sensing data, we identified significant shifts in fire regimes and landscape dynamics between the periods of 2000–2010 and 2010–2020. Our findings indicate a potential extension of the fire season, reflecting the impacts of climate change. Despite improvements in firefighting capabilities, the occurrence of large fires is on the rise in the Natural Park, underscoring the need for proactive management strategies in such areas. Notably, significant fire events in 2011, 2016, 2017, and 2020 extensively affected wooded areas, constituting the majority of the burned area. Shrubs and forests emerged as particularly vulnerable, with varying degrees of burn severity influencing post-fire vegetation recovery rates. While shrublands expanded their coverage between 2000 and 2010, rocky areas with sparse vegetation showed an increase over the subsequent decade (2010–2020), indicating soil degradation and potential desertification in areas affected by recurrent and severe fires, especially within zones designated for the highest levels of protection (with fire rotation periods of less than 1 year). In conclusion, this study provides valuable insights into the impacts of wildfires, changes in land cover, and post-fire soil-vegetation dynamics, which can inform management and conservation efforts in fire-prone mountainous regions. Leveraging advanced remote sensing techniques enables the monitoring of cumulative soil degradation resulting from repeated wildfires over extended periods.
Introduction
Wildfires are known to be one of the main drivers of forest loss and soil degradation worldwide (Pausas and Fernández-Muñoz, 2012; Díaz-Raviña et al., 2021; Tyukavina et al., 2022). High-intensity and recurrence wildfires strongly impact vegetation cover, increasing soil erosion and loss (Chandler et al., 1983; Shakesby, 2011; Díaz-Raviña et al., 2012; Regos and Díaz-Raviña, 2023). In Europe, four Mediterranean countries—Italy, France, Portugal, and Spain—rank among the top six nations grappling with the highest numbers of wildfires (San-Miguel-Ayanz et al., 2021). Spain and Portugal, in particular, stand out as the countries most affected by both the frequency of fires and burned areas. Official European statistics underscore the persistence of severe fire seasons in the last years—three of the most catastrophic fire seasons on record occurred within the past 6 years. The 2022 fire season is the second worst for EU, speaking about fire burnt area (San-Miguel-Ayanz et al., 2023). Spain and Portugal, respectively showing 267,947 ha and 110,097 ha, and Romania presenting 1,599 as the percentage of average, were the most affected regions. Moreover, large wildfires (those larger than 500 hectares) began occurring as early as June–July, preceding the traditional fire season (Rodrigues et al., 2023). Longer fire seasons and increased burnt area in the autumn season have been also recorded over the past decade. These deviations from the norm were correlated with unprecedented levels of fuel dryness, atmospheric water demand, and pyrometeorological conditions. Large wildfires accounted for 82% of the total burnt area, 47% of which took place within protected areas (Rodrigues et al., 2023).
Since 2017, approximately 35% of the total burnt area, exceeding 11,600 km2, has occurred within the Natura 2000 network (San-Miguel-Ayanz et al., 2021), highlighting the vulnerability of these conservation zones. Socioeconomic factors, including the traditional use of fire for land management and the abandonment of traditional agricultural practices, together with more adverse fire-weather conditions due to climate warming have exacerbated wildfire hazards in many mountainous regions (Moreira et al., 2011). This escalating wildfire hazard underscores the pressing need to comprehend the spatial interactions among various factors driving wildfire-landscape dynamics in fire-prone regions, particularly in protected areas that serve as “living labs” for sustainability.
Forest ecosystems consist of two interconnected components: vegetation and soil. Wildfires impact both the aboveground vegetation and the belowground soil components, which are strongly interdependent (Zhang and Biswas, 2017; Pressler et al., 2019; Yuan et al., 2020; Dove et al., 2022). Forests play a crucial role in protecting soils from degradation and enhancing their functions, such as carbon sequestration, provision of food, fiber and fuel, water purification and soil contaminant reduction, climate regulation, nutrient cycling, habitat for organisms, flood regulation among others (Pereira et al., 2018). Additionally, forests provide a multitude of services that are directly or indirectly dependent on soil, including the provision of food products such as wild berries and mushrooms, timber and biomass for fuel, medicinal plants, support for pollination, and the regulation of oxygen and clean water (Pereira et al., 2018). Wildfires have the potential to induce a range of physical, chemical, and biological degradation processes in soil, leading to significant modifications in soil functions and the overall quality, quantity, and sustainability of burned forest areas. These alterations can have far-reaching implications for ecosystem health and resilience, as well as the climate change mitigation potential of affected landscapes (Neary et al., 1999; Certini, 2005; Martín et al., 2012; Lombao et al., 2015a; Zhang and Biswas, 2017). The alterations caused by fires to soil quality, microbial biodiversity, water availability, and post-fire erosion create a cycle of environmental degradation. This cycle jeopardizes the natural values that protected areas aim to preserve (Martín et al., 2009; Díaz-Raviña et al., 2012; Barreiro and Díaz-Raviña, 2021; Girona-García et al., 2021). Soil microorganisms, involved in over 95% of soil processes, play a crucial role for ecosystem recovery (Villar et al., 2004; Mataix-Solera et al., 2009; Barreiro and Díaz-Raviña, 2021; Certini et al., 2021). While these effects primarily impact the uppermost layers of the soil profile, high-frequency fires lead to the progressive thinning of soils. In extreme cases, this can result in complete soil destruction, leading to desertification and the formation of bare soils and rocky landscapes (Shakesby, 2011; Perez-Rey et al., 2023).
Numerous studies have demonstrated that the impact of wildfires on soils and the subsequent recovery of burned areas depend on various factors, including soil type, vegetation, topography, meteorological conditions during and after the fire, and the characteristics of the fire regime (such as severity, duration, and recurrence) (Pausas and Fernández-Muñoz, 2012; Lombao et al., 2015b; Francos et al., 2018; Dove et al., 2020; Lombao et al., 2020; Agbeshie et al., 2022). The impact of fire regimes on forest ecosystems is site-specific, meaning that conclusions drawn from studies conducted in one area may not necessarily apply to other locations with different conditions. Many studies focusing on the effects of wildfires on soils primarily examine short- or medium-term impacts following the most recent fire event. However, these studies often do not monitor the long-term evolution of post-fire properties or record the severity of the fires to which ecosystems are exposed, which are critical aspects for understanding and predicting ecosystem recovery. Thus, due to the limitations of time, data availability, logistics and investment, research concerning the long-term impact of fire regime on forest ecosystems is scarce (see, e.g., Francos et al., 2018). It is necessary to better understand the long-term effects of wildfires on soil properties, especially in fire-prone ecosystems, in order to develop greater insights into their resilience and capacity to respond to such repeated perturbations. These studies are especially relevant considering the climate change impacts on global fire activity (Moritz et al., 2012).
To better understand the long-term impact of fire regimes on forest ecosystems, particularly regarding soil and vegetation dynamics, it is essential to characterize the historic fire regime. Integrating this historical context with updated information gathered through remote sensing technologies holds significant promise for elucidating the long-term consequences of wildfire disturbances on soil degradation. This study focuses on assessing the cumulative impact of wildfires on soil-vegetation dynamics within the Natural Park “Baixa Limia–Serra do Xurés,” a region known for its high susceptibility to fires in Galicia (Northwest Spain). Our approach considers the interactions and changes between soil properties and vegetation over time within an ecosystem (i.e., soil-vegetation dynamics). This concept encompasses the various processes and feedback mechanisms that occur between the soil and plant communities, directly affected by wildfires. To achieve this, we integrated statistical data with remotely sensed analyses on burn severity and land-cover changes to identify: 1) the vegetation types predominantly impacted by fires, 2) the primary land-cover transitions occurring in recently burned areas, and 3) the spatial distribution of fires occurring between 2010 and 2020, considering the administrative and protective designations of the natural park. We particularly focus on areas designated as having a high level of protection, characterized by heathlands and grasslands that are highly susceptible to frequent wildfires and potential soil erosion.
Material and Methods
Study Area
The Natural Park “Baixa Limia-Serra do Xurés” (hereafter referred to as BL-SXNP) stands as the largest Natural Park in Galicia, covering nearly 30,000 hectares spread across six municipalities: Bande, Calvos de Randín, Entrimo, Lobeira, Lobios, and Muíños (see Figure 1).
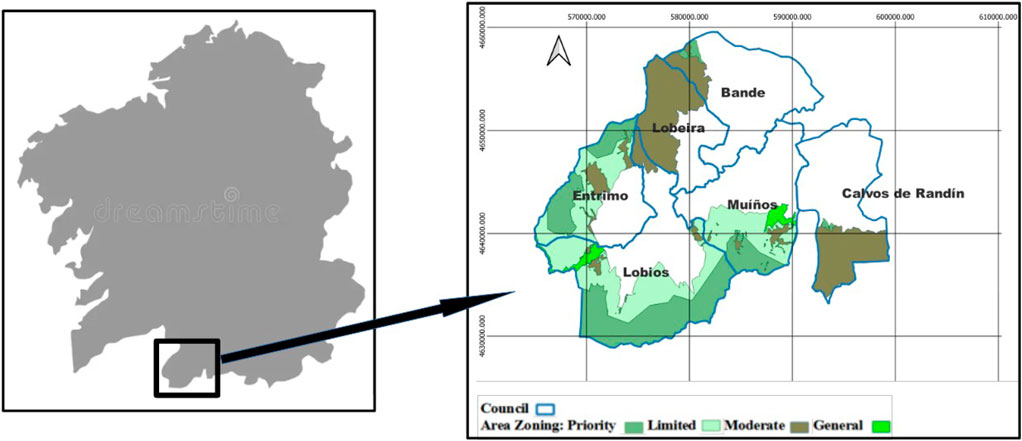
Figure 1. Map illustrating the zoning of the different protection levels within the “Baixa Limia–Serra do Xurés” Natural Park, spanning six municipalities: Bande, Calvos de Randin, Entrimo, Lobeira, Lobios, and Muíños. Green colors depicts the level of protection according to the natural resources management plan of the Natural Park.
Designated under DECREE 64/2009 on February 19, this Natural Park boasts a multifaceted conservation status. It forms an integral part of the Natura 2000 Network, earning recognition as a special conservation area (ZEC ES1130001 “Baixa Limia”) and a special protection area for birds (ZEPA ES0000376 “Baixa Limia – Serra do Xurés”). Notably, UNESCO declared it a Transboundary Biosphere Reserve, together with the “Peneda–Gerês” National Park on 27 May 2009 (Macedo et al., 2009). This designation underscores the area’s commitment to fostering a sustainable relationship between its inhabitants and the natural environment, aiming to balance the conservation of biological and cultural diversity with economic and social development.
The climate in the BL-SXN is temperate oceanic sub-Mediterranean, with a mean annual temperature of 8°C–12°C and a mean annual precipitation of 1,200–1,600 mm, which involves a significant water shortage in summer. According to the Köpen-Geiger classification system, it corresponds to the “Csb” class. The soil, developed over granitic rocks, had a sandy texture and the range values of all physical and chemical properties are associated with poor soils (acid pH and low nutrient availability and organic matter content) (Rodríguez-Lado et al., 2018). The study area encompasses climatic conditions characteristic of both the Mediterranean and Atlantic regions, boasting a diverse array of 26 distinct habitat types, six of which are designated as priority habitats. Of particular significance are the shrub habitats, notably EU priority habitat 4020 “Atlantic wet heaths of Erica ciliaris and Erica tetralix,” which are particularly vulnerable to wildfires and are therefore prioritized for conservation efforts. The preservation of shrubs and rocky formations from an ecological standpoint carries considerable weight, establishing this area as one of Galicia’s most compelling regions of interest (Decree 401/2009 of October 22) (Macedo et al., 2009).
Wildfires pose a significant threat across all municipalities within the park. To mitigate the impact of fires throughout Galicia, zoning based on spatial fire risk has been implemented since 18 April 2007. This zoning classifies territories into areas of low, medium, and high fire risk, with heightened measures in high-risk zones. Additionally, Law 3/2007 of 9 April defines high fire risk areas and parishes of high incendiary activity, where extraordinary measures are warranted to prevent fires and protect forests from their impacts. In alignment with the Prevention and Defense Plan against Forest Fires of 2023 (de Galicia, 2023), 40 parishes of high incendiary activity have been identified, including four within the natural park and one in close proximity.
For effective management of activities within the Natural Park, the territory is delineated into various zones with differing levels of protection, operationalized through zoning. These zones, categorized based on the significance of natural values, serve as a pivotal management tool, regulating permissible and prohibited uses:
• Zone I. Priority Conservation Interest (Reserve): These are areas that require a high degree of protection due to harboring the highest natural, scientific, and landscape values, as well as the uniqueness of their habitats, species, and communities.
• Zone II. Limited Use: It consists of areas that have a high degree of naturalness and can support a certain level of public use, oriented towards research, education, environmental interpretation, and controlled nature viewing. The conservation of its resources and values is guaranteed while allowing certain primary uses.
• Zone III. Moderate Use: This category includes lands where natural formations, generally of medium quality and uniqueness, have undergone a higher degree of humanization, or have good capacity to support more intense public use. In these areas, agricultural and livestock uses, and traditional exploitation is allowed.
• Zone IV. General Use: These are areas that have lower quality within the protected natural space. They may be used for locating facilities for public use.
Fire Regime Characterization
The available fire perimeter dataset (collected on the ground via GPS) for the entire Autonomous Community of Galicia starts from the year 2010. The numerical datasets utilized in this study, spanning from years 1983–2020, are sourced from two distinct origins. Data spanning from 1983 to 2010 are derived from the official statistics of the Ministry for the Ecological Transition and Demographic Challenge at the municipal level (MITECO, 2023). Fire data statistics for the period 2010–2020 are gathered from the Department of Rural Environment of the Xunta de Galicia (hereafter, the DGDM database).
The dataset from the DGDM comprises annual records of fire incidents for each municipality. Each entry includes information such as the onset date, duration, parish where the fire originated, burned forest area, and total affected area, all expressed in hectares, for each year between 2010 and 2020. The DGDM database encompasses fire events affecting areas larger than 0.01 hectares. However, incidents where the burned area is less than 1 hectare are not categorized as fires unless they affect more than 0.5 hectares of wooded terrain.
In our study, we classified the fire events based on their size, considering the fire-weather conditions prevailing during ignition and development:
1) Fire events smaller than 1 hectare typically denote burning of agricultural or forestry debris that did not get out of control.
2) Fires spanning 1–10 hectares often indicate incidents that momentarily escaped control but were swiftly contained due to favorable environmental conditions.
3) Incidents ranging from 10 to 100 hectares typically signify fires that unfolded under challenging meteorological conditions and prolonged previous drought, presenting very difficult-to-control fire-weather situations.
4) Fires surpassing 100 hectares, and up to 500 hectares, are characterized by adverse environmental conditions and several secondary fire outbreaks. Large Forest Fires, exceeding 500 hectares, manifest under exceptionally unfavorable meteorological conditions, often taxing firefighting resources due to their potential threat to inhabited areas.
To characterize the fire regime, we computed several statistics for the forest and shrubland land cover types, number of fires and burnt areas throughout the specified period across municipalities, and different protection levels. We also estimated fire recurrence, defined as the number of times an area is burned, and fire rotation, which is the time required to burn the equivalent of a specified area. Fire rotation was calculated as follows (Bond and Keeley, 2005; Agee, 2016):
In addition, we used burn severity maps derived from García-Redondo et al. (2023). In particular, Landsat 5 imagery was used for 2010 and 2011, while Landsat 7 was used for 2012 due to cloud cover rendering Landsat 5 imagery unusable. From 2013 onwards, Landsat 8 and later Sentinel 2 data were employed. All images were processed to correct for geometric distortions and atmospheric effects using the “Dark Object Subtraction” methodology. This correction enhanced the reliability of the data by converting top-of-atmosphere reflectance to surface reflectance.
The NBR is a widely used index derived from satellite imagery that measures the difference in reflectance between pre- and post-fire conditions, specifically capturing changes in vegetation and soil characteristics. In our study, burn severity maps were produced using the NBR calculated from pre- and post-fire satellite images. The NBR effectively highlights areas of high burn severity where vegetation and organic matter have been significantly altered, thus providing insights into the impact on both soil and canopy levels. High NBR values indicate areas with severe canopy damage and soil exposure, while lower values correspond to less affected regions. Normalized Burn Ratio (NBR) was calculated for each year, which measures the ratio between near-infrared (NIR) and short-wave infrared (SWIR) radiation. NBR helps identify burned areas as NIR and SWIR bands of satellite sensors respond differently to burned vegetation (Eq. 1):
The NBR captures changes in vegetation and soil characteristics, indicating the degree of ecological change caused by fire. High NBR values correspond to areas with significant canopy damage and soil exposure, while lower values indicate less affected areas (all details in García-Redondo et al., 2023). The fire recurrence (defined as the number of fire events per spatial unit), was estimated by overlapping the annual burnt area maps. Thus, for each grid cell, we counted the number of times a fire event has occurred within the defined time frame.
Land-Cover Change Analysis
To analyze landscape changes, we used the Land Use and Land Cover (LC) maps derived from Cánibe et al. (2022). These maps were obtained from satellite images captured by Landsat 5 TM, Landsat 7 ETM+ and Landsat 8 OLI/TIRS sensors. These images were sourced from the USGS (United States Geological Survey) Earth Explorer database. Landsat Collection 1 Level-1 imagery was chosen since they are already geometrically corrected, ensuring consistent geo-registration with image-to-image tolerances of ≤12 m radial root mean square error (RMSE). All images were then calibrated and radiometrically corrected by using the “Dark Object Subtraction” methodology (see Cánibe et al., 2022).
Supervised classification methods were applied to generate LC maps. Training and validation areas were established for six LC classes, including 1) water bodies, 2) deciduous forests, 3) evergreen forests, 4) shrublands, 5) croplands and grasslands, and 6) bare soil with sparsely vegetated areas. Training and validation areas for each habitat class were established by on-screen digitizing in QGIS software, and consisted of a set of pixels identified over well-known homogeneous areas in each Landsat image, thus providing a reference spectral signature for each class. Two images per year were utilized to enhance spectral separability among LC classes, especially for deciduous forests. Four different classification algorithms were employed: Random Forest, Least Squared Support Vector Machines with Radial Basis Function Kernel, Monotone Multi-Layer Perceptron Neural Network, and Adaptative Boosting. Accuracy assessment was conducted using confusion matrices to calculate sensitivity and positive predictive power.
We analyzed the changes in the extent of each LC class for the period 2000–2010, and for the period 2010–2020, both inside and outside the burnt areas, to infer land cover conversions from one LC class to another caused by wildfires that took place in last 10 years. In particular, we quantified the spatial extent (in ha) of each LC class per year (2000, 2010, and 2020) from the different LC maps obtained from each classification algorithm. Boxplots were constructed using the R package “ggplot2” (Wickham, 2009). The contribution of each LC class to the change (i.e., conversion from one LC class to another) was shown through a transition matrix obtained by cross-tabulation of an ensemble LCC map (i.e., “majority vote” rule across the methods used for the supervised classification). Transition matrices were computed with the R package “lulcc” v.1.0.2 (Moulds, 2017). Statistical analyses and graphical representations were conducted using RStudio. Additionally, maps were generated utilizing both the RStudio software application and the QGIS application.
Results
Fire Regime Characterization
The analysis of fire occurrences spanning from the 1980s to the 2020s reveals distinct trends (Figure 2). Initially, there were few fires in the 1980s, but this number increased exponentially in the 1990s, reaching up to 700 fires in 1997, before decreasing throughout the 2000s, 2010s, and 2020s, eventually returning to levels comparable to or even lower than those seen in the 1980s (Figure 2). This fire regime shift is attributed to the implementation of the Prevention and Defense Plan against Forest Fires by Xunta de Galicia in 1999. Interestingly, the number of fires does not correlate with the burnt area, with many years showing relatively low burnt areas despite varying fire frequencies, mainly due to the prevalence of small fires (<100 hectares) that are effectively contained. Surveillance and management strategies are crucial in combating fires. The implementation of the Prevention and Defense Plan against Forest Fires, which encompasses both aspects, has been the primary factor in reducing the number of fires and the area burned. This reduction has been consistently maintained over time.
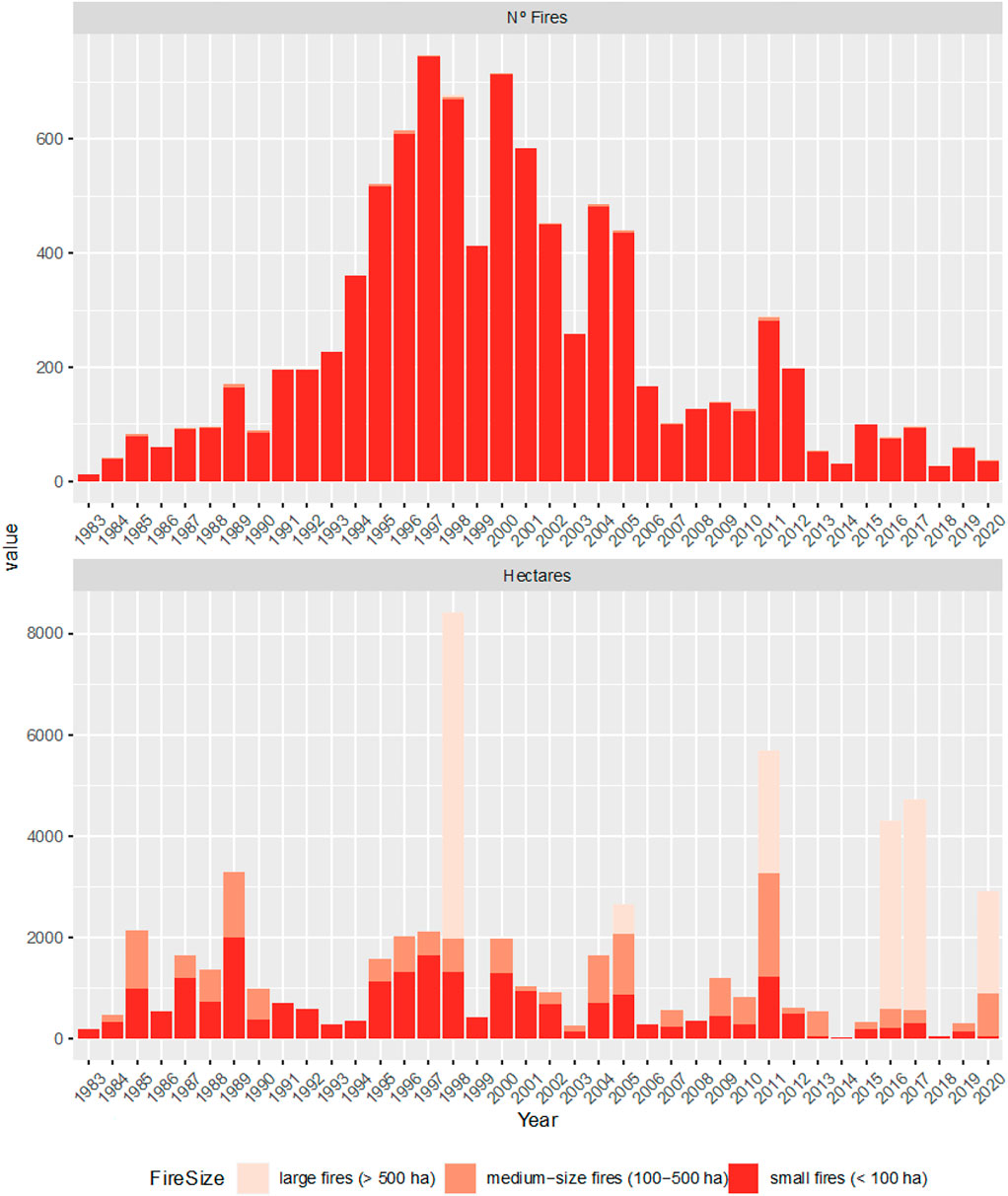
Figure 2. Fire frequency (number of fires) and burnt area (hectares) in the municipalities within the Natural Park from 1983 to 2020. The burnt area is represented by fire sizes. Additionally, a dotted black line indicates the fire regime observed in the last decade, which was subjected to more detailed analysis.
Exceptions to this pattern occurred in 1985, 1989, 1998, 2005, 2011, 2015, 2016, and 2020, with 1998 showing the most significant deviation, where over 8,000 hectares were burned (Figure 2). Over the period from 1983 to 2020, there was an overall increase in fire size, with small and medium fires dominating during the 1980s and 1990s, aside from 1998, which showed a large fire. However, the contribution of medium-sized fires increased during the 2000s, and by the 2010s, medium and large fires became more prevalent, leading to larger burnt areas, particularly evident in 2011, 2015, 2016, and 2020.
In terms of fire seasonality, the number of fires remained relatively stable, with spring consistently recording the highest values, followed by autumn and summer (see Figure 3). However, statistics indicate a concerning trend: an increase in the burnt area during the autumn season over the past decade. This observation suggests an extension of the fire season (Figure 3).
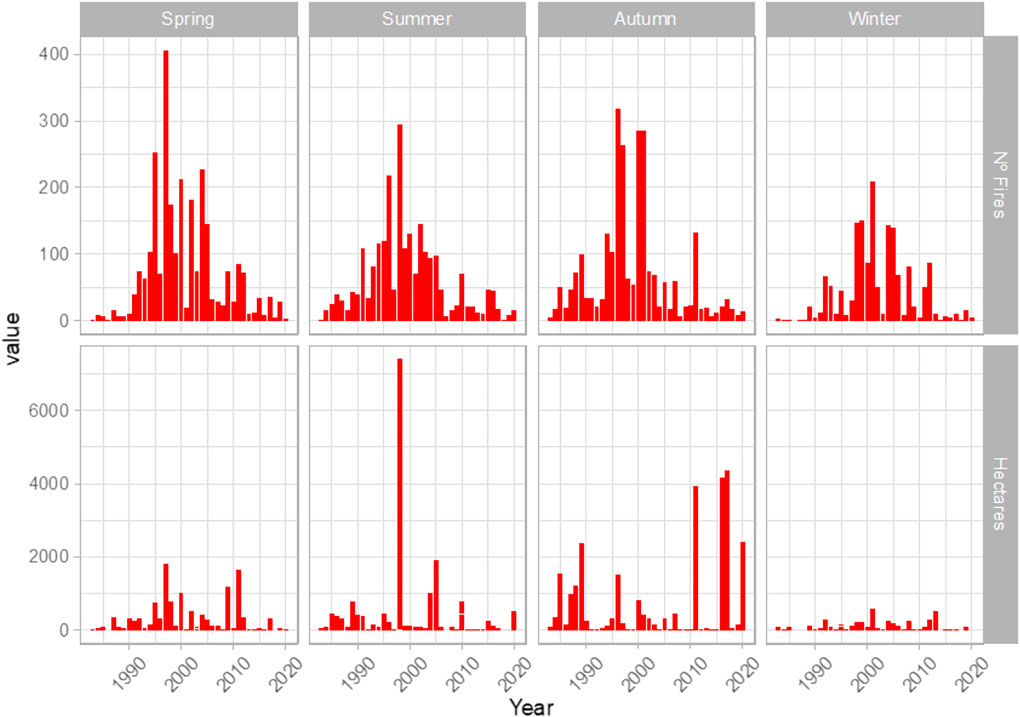
Figure 3. Fire frequency (number of fires) and burnt area (hectares) in the municipalities within the Natural Park from 1983 to 2020, categorized by each season.
Additionally, fire occurrence also varied across municipalities, with “Muíños” experiencing the highest number of fires following by “Entrimo,” “Lobeira,” and “Lobios” with the largest burnt areas due to large fires (see Supplementary Figures S1, S2 for fire prevalence at the municipal and parish level, respectively). Although the proportion of fires remains relatively consistent across seasons, the burnt area is significantly larger during the high-risk season, typically from July to October. During the period from 2010 to 2020, the burnt area in the high-risk season is nearly five times greater than the area burned throughout the rest of the year, despite a similar total number of fires (Table 1). The most notable difference is observed in fire-size group G5, with the number of fires in G4 close to doubling (Table 1).
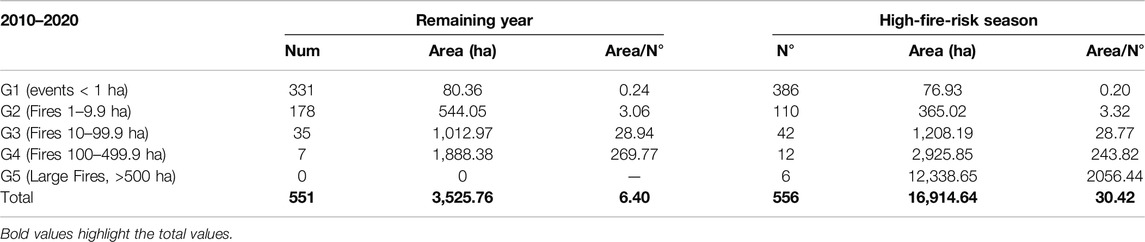
Table 1. Number of fires produced according to the size of the fire surface, and total surface area affected by each group, in the six municipalities in the period 2010–2020 during the months of maximum risk (July–October) and the rest of the year (November–June).
Fire Impacts on Vegetation
Official fire statistics indicate that wildfires have primarily affected shrubland covers within the six municipalities of the Natural Park (Figure 4). Exceptions to this trend were observed only in the years 2016 and 2017, during which the extent of forested areas affected by wildfires exceeded 1,500 hectares (Figure 4).
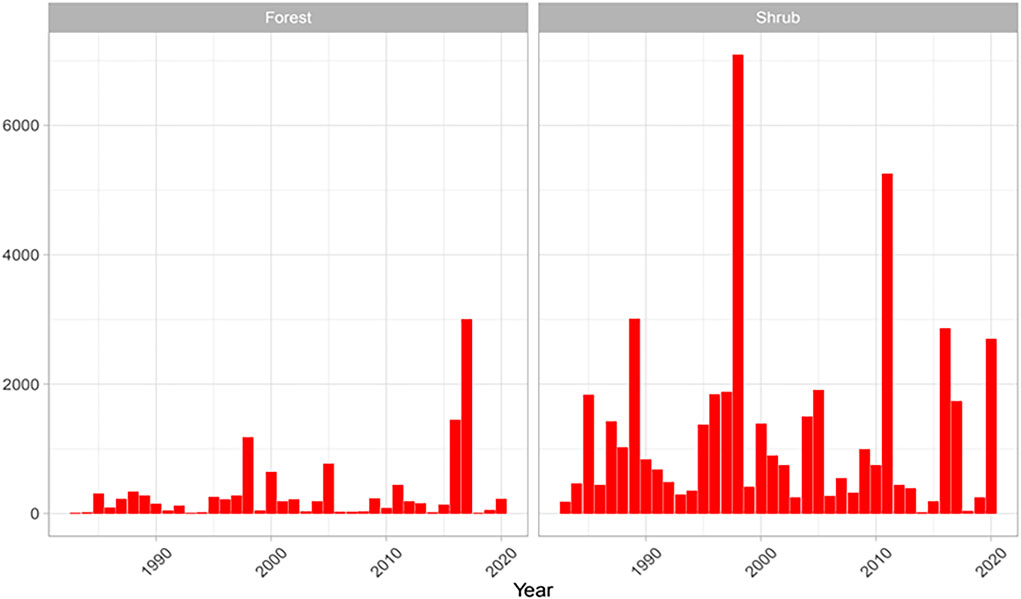
Figure 4. Area burnt (in hectares) over the period 1983–2020 in forests and shrublands in the six municipalities included in the Natural Park “Baixa Limia Serra do Xurés.”
In the last 10 years (2010–2020), wildfires in the Natural Park affected over 14,642 hectares dominated by shrublands (representing 71.63% of the municipality) and 5,798 hectares of forested areas (approximately 28.37%, see Table 2).
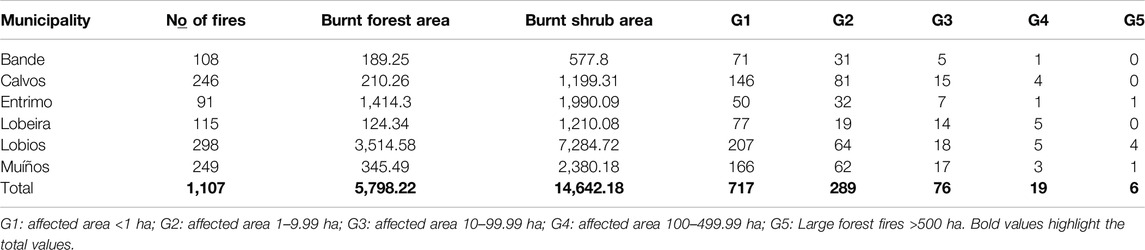
Table 2. Number of fires by fire-size group and total area, wooded and open, affected by group, in the six municipalities during the years 2010–2020.
In the past 5 years, wooded areas have shown increased vulnerability within the Natural Park. Of particular significance are the years 2016 and 2017, during which burnt wooded areas experienced the most significant degradation compared to shrubland areas during that period (Figure 4). Regarding fire recurrence, a large proportion of the Natural Park has burned at least once, with some areas burning up to five times in the last 10 years (approximately every 2 years) (Figure 7).
Land-Cover Changes in Burnt Areas
The analysis of land cover change reveals significant shifts between the periods 2000–2010 and 2010–2020 (see Figure 5). During the latter period, there was a notable increase in shrubland coverage, accompanied by a decrease in shallow soils with sparse vegetation (hereafter: “rocky areas”). However, this trend reversed over the subsequent decade, with a decrease in shrublands in favor of rocky areas. Similar patterns were observed in evergreen forest cover, albeit to a lesser extent than shrubland. The observed pattern was consistent within the areas affected by wildfire between 2010 and 2020 compared to the rest of the Natural Park.
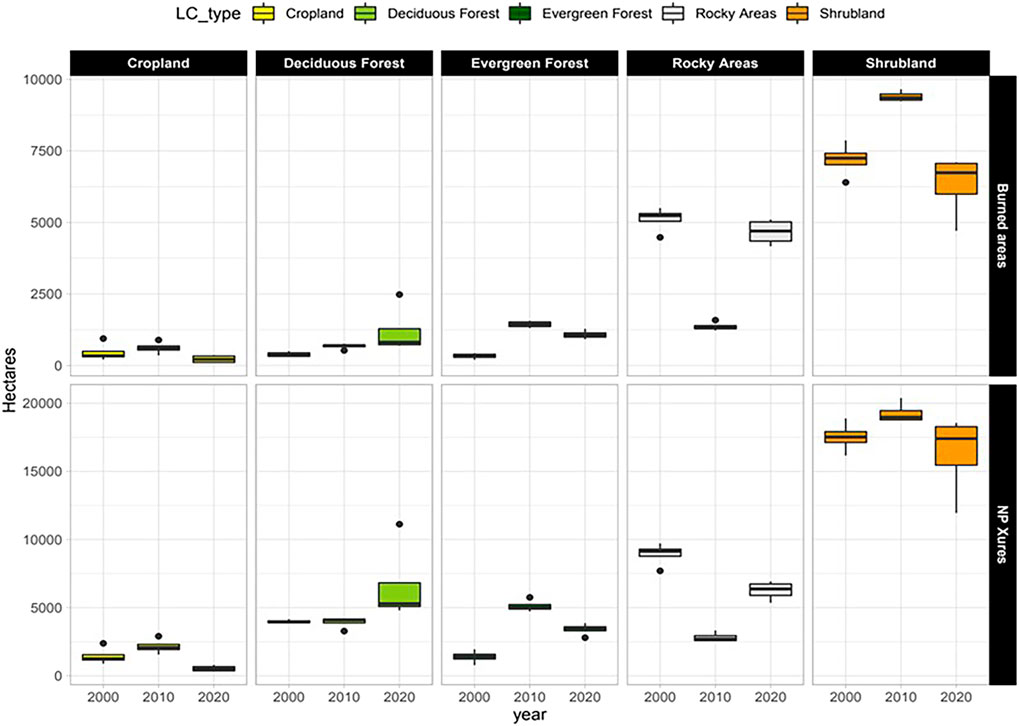
Figure 5. Extent (in hectares) of each land cover class per year. The boxplots display the median, the 50th percentile (box), and 95th percentile (whiskers) confidence intervals. The intra-plot variability arises from the different estimations obtained from each classification algorithm.
The intra-box plot variability illustrates that the land cover type most affected by the algorithm used for classifying satellite images was “shrubland.” Despite this uncertainty, the period from 2000 to 2020 showed a predominant transition from rocky areas (i.e., shallow soils with sparse vegetation) to shrublands (see Figure 6). However, the subsequent decade a reversal in this trend was found, marked by a conversion from shrubland to rocky areas, and to a lesser extent, from evergreen forest (predominantly pine plantations) to shrubland. Notably, a significant portion of rocky areas in 2000 and 2010 remained unchanged after 10 years, despite natural successional processes that would typically favor vegetation encroachment in those areas.
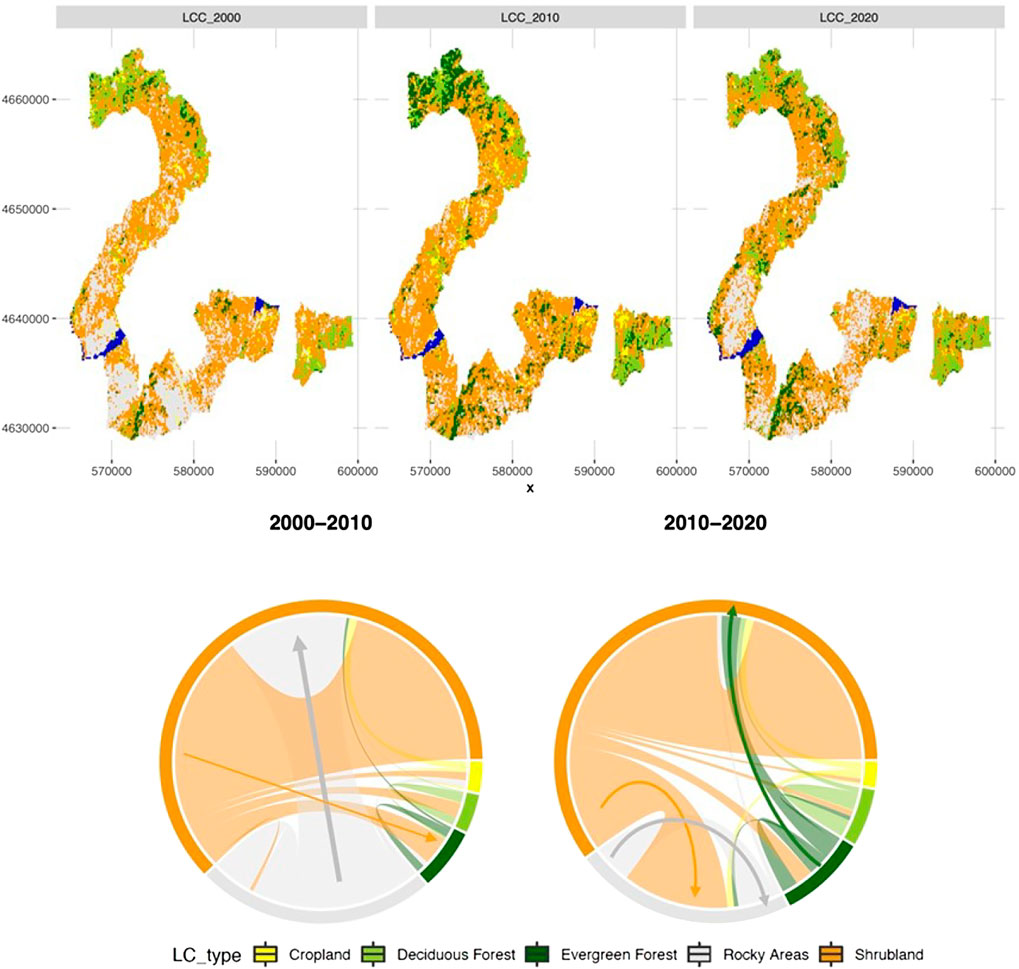
Figure 6. Land cover maps derived from the ensemble of all supervised classifications for each year. Circular plots illustrating the changes in land cover between 2000 and 2010 and between 2010 and 2020 within the areas burnt between 2010 and 2020. The width of the lines is proportional to the contribution of each land cover type to the change. Different colors represent different types of land cover, and arrows indicate the direction of the change.
The primary land cover transitions are spatially linked with areas previously affected by varying degrees of burn severity. There is a heterogeneous distribution of burn severity, which determines an uneven impact on vegetation, as reflected in the land cover composition of the year 2020 (see Figure 7). In fact, some of these areas have experienced multiple fire events, although the high rates of post-fire vegetation recovery might partially mask the spatial patterns of vegetation.
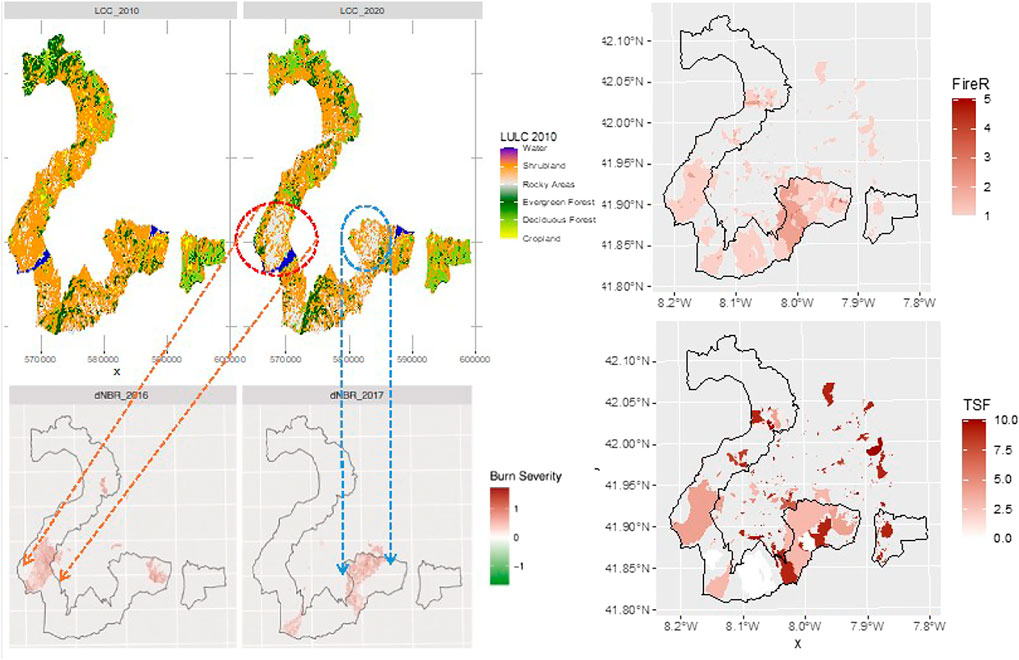
Figure 7. Land cover maps for year 2000 and 2010. Circular plots illustrate rocky areas previously affected by wildfires. Burn severity: maps derived from the dNBR, for years 2016 and 2017. FireR, Fire Recurrence map; TSF, Time Since last Fire, from year 2020 (year 10) to 2010 (year 0).
Fire Impacts in Protected Areas
The analysis of the spatial overlap between the areas burned during the period 2010–2020 and the zoning with different levels of protection revealed considerable variability in the burned area across different municipalities. Remarkably, wildfires impacted the areas with the highest levels of protection in the municipality of “Lobios” (approximately 3,423 and 3,097 hectares in priority and limited use zones, respectively; Figure 8). These areas, characterized by the highest levels of protection and restricted use, were also heavily affected by wildfires in the municipality of “Muíños” (burning approximately 646 and 2,213 hectares in priority and limited use zones, respectively; Figure 8). In “Entrimo,” the priority areas experienced significant burning, totaling around 520 hectares, while those with limited use burned approximately 1,200 hectares (Figure 8). All these areas exhibited a fire rotation of less than 1 year, indicating that their entire extent burns annually (Figure 8). Conversely, Bande was the municipality least affected by fire, particularly in areas with the lowest protection levels, with fire rotations exceeding 200 years (Figure 8).
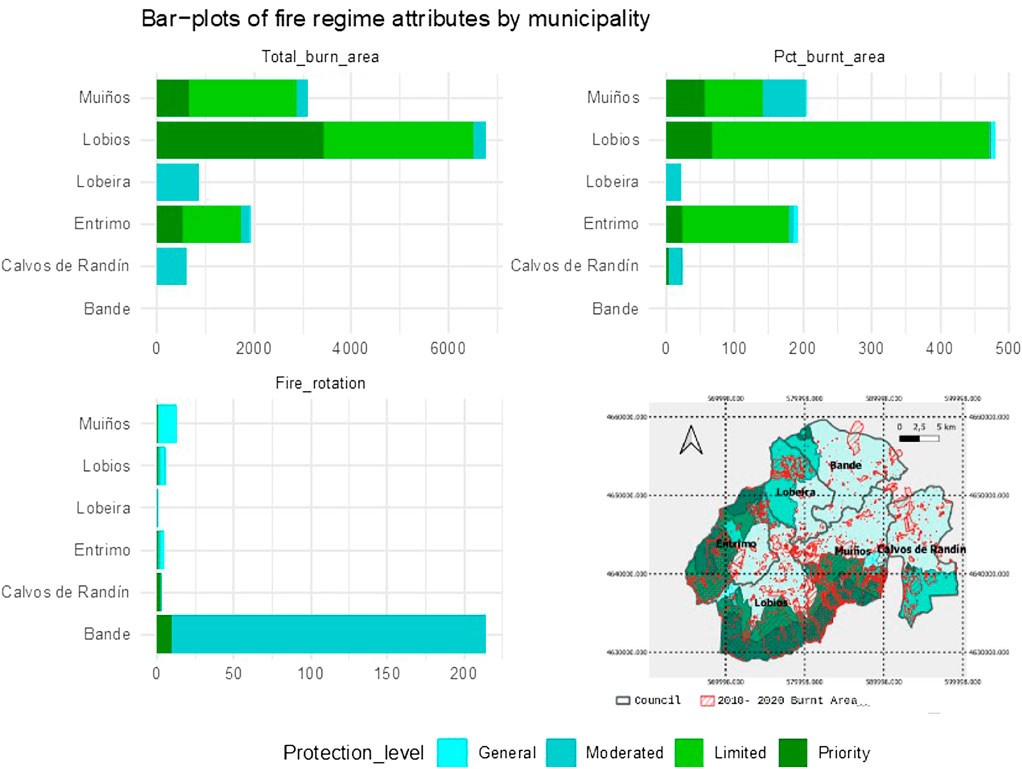
Figure 8. Fire rotation, total burned area, the percentage of the area burnt in each zone of the natural resources managing plan of the Baixa Limia Serra do Xurés Natural Park by municipality.
Discussion
Overall, our findings underscore the complex interplay between wildfire occurrences, burn severity, land cover transitions, soil degradation and post-fire vegetation recovery rates within the Natural Park “Baixa Limia Serra do Xurés.” Understanding these dynamics is crucial for effective wildfire management and conservation efforts in the Natural Park.
During the 2010–2020 period, six large wildfires (i.e., larger than 500 ha) occurred in 2011, 2016, 2017 and 2020. The largest among the six large wildfires occurred during 2017 and affected 3,485.70 hectares, of which 2,100.00 were wooded areas and 1,385.50 were open areas. The results show us that the majority of the burned area (60.08%, Table 1) is caused by these large fire events. These results are in line with the proportion of 75%–80% of the burned areas that can be attribute to large fires in the Mediterranean area (San-Miguel-Ayanz and Camia, 2009). Our data showed that over the 1983–2000 period, the size and the extent of wildfires have been increasing in the Natural Park, and thereafter, during the 2001–2020 period, the trend shows a progressive reduction in fires and area burned (see Figure 1). Despite this reduction, the risk of having large and intense fires in the Natural Park increased notably, which is consistent with wildfire patterns observed in Europe (Fernandez-Anez et al., 2021) and United States (Iglesias et al., 2022a).
These large wildfires are often characterized by being developed under in high wind and dry vegetation conditions, related to the increase in available fuel and climate change (Bowman et al., 2011). They are considered to be increasingly frequent and virulent, being very difficult to control and affecting large areas (Hinojosa et al., 2016). This behavior is observed in the Natural Park, in some areas and certain years: although they cannot be considered as “mega-fires” due to their extension, they can be considered as extreme events due to its behavior (Tedim et al., 2013; (Linley et al., 2022). At the level of extinction, a fire can affect large areas since, when the flame front advances in a fire, not only does the length of the front that needs control increase, but it also increases the possibility of threat to population centers and the generation of secondary outbreaks. This requires an increase in fire-fighting resources to address these new situations, so the efforts of the resources that are fighting fire in the forests must abandon their defense to be used in the defense of the built structures. Our findings revealed that the areas designated with the highest levels of protection (reserve and limited use) experienced the most significant extent of burning (see Figure 8). This trend can be attributed to firefighting priorities, which are often hindered by challenging topography and limited accessibility in these regions. Steep slopes and difficult access can impede effective fire suppression efforts. Besides, the areas with the highest levels of protection are more vulnerable to wildfires because they are zones with minimal intervention. This approach aims to minimize disturbances to endangered habitat and species. Consequently, these protected areas often have denser vegetation and higher fuel loads, increasing their susceptibility to wildfires.
The spatial distribution of wildfires within the park demonstrates a pronounced impact on shrubland covers, with exceptions noted in the years 2016 and 2017 where forested areas experienced significant degradation, exceeding 1,500 hectares (see Figure 4). This underscores the vulnerability of both shrublands and forests to wildfire events within the park. These results are in line with other regions in NW Iberia. In north of Portugal, shrublands are also the land cover type most affected by wildfires, following by forest types (Calheiros et al., 2022). Furthermore, the examination of land cover transitions highlights a clear association between areas previously affected by wildfires and subsequent changes in land cover composition (see Figures 6, 7). The observed heterogeneity in burn severity across these areas plays a crucial role in shaping the post-fire vegetation recovery rates, which has been previously found across different fire-prone ecosystems (Chu et al., 2017; Viana-Soto et al., 2017; Guz et al., 2022). While some regions have undergone multiple fires, the rapid recovery of vegetation following wildfires suggests a resilient ecosystem within the park (Torres et al., 2018). However, it is worth noting that the high rates of post-fire vegetation recovery may partially mask the spatial patterns of vegetation, potentially complicating efforts to accurately assess the long-term impacts of wildfires on soils. Previous studies suggested that an indicator-based approach based on satellite time-series of vegetation indices can effectively cover various facets of post-fire recovery (Torres et al., 2018). Therefore, the application of these new advances in remote sensing could improve the monitoring and prediction of post-fire recovery dynamics, with valuable applications in fire hazard management and post-fire ecosystem restoration and soil monitoring in the natural park.
Moreover, the analysis of fire seasonality reveals a notable increase in the area burnt during the autumn season over the last decade (see Figure 3), which suggests a potential lengthening of the fire season within the park. This observation aligns with the widely recognized transformation of the fire regime due to climate change, impacting not only the size and intensity of fires but also the duration of the fire season (Flannigan et al., 2013; Marlon et al., 2013; Moreno et al., 2019; Keeley and Pausas, 2022). Traditionally, the fire season was confined to the summer months. However, the extended duration of the fire season, attributed to climate change, has prompted revisions in fire management strategies in Galicia. This is evidenced by Law 3/2007, enacted on 9 April, which focuses on the prevention and defense against forest fires in Galicia. Notably, the fire season was extended from late June, July, August, and September to include early October (de Galicia, 2018). These regulatory changes reflect the need to adapt to fire regime shifts and highlight the importance of proactive measures to mitigate wildfire risks in fire-prone regions like the Natural Park “Baixa Limia Serra do Xurés.” In addition, and despite the increase in budgets and technology for extinction, the area burnt by large fires is increasing in the natural park (Figure 2), as reported in other areas of the Mediterranean climate (Seijo, 2005). Many authors place the drastic change in fire regime in Spain around the 1970s due to the land abandonment produced by the rural exodus (Salis et al., 2013; Moreno et al., 2014; Pausas and Fernández-Muñoz, 2012). In the Natural Park, we found a steep decrease in the number of fires from 2000 onward, but an increase in the area burnt by large fire especially in the last decade (see Figure 2).
Considering the ratio of the total burned area in each season divided by the total number of fires in that season, we observed a ratio of 6.40 for July to October and 30.42 for November to June. In other words, interpreting this figure as the average surface area per fire during these periods, the maximum risk area would be approximately 4.75 times greater. However, if we discount the total surface area affected by fires from groups G4 and G5 during these periods, considering that firefighting resources were diverted to protecting populations rather than combating fires as usual, and then add the average suppressed area (those with a burned area greater than 100 hectares), we find a different perspective. These results reveal that firefighting resources operate with great effectiveness under normal conditions, as evidenced by similar ratios during low and high-risk times (when fire-size groups G4 and G5 are excluded). Even when faced with harsh conditions to contain fires, their effectiveness remains high, with a ratio of 3.07, close to the baseline ratio of 3.01 (see Table 1). The significant drop in the ratio during high-fire-risk situations (from 30.42 to 3.07) is attributed to the distribution of the available firefighting resources, both personnel and materials. It is only when these resources are diverted to attend to more urgent needs that the forest suffers the consequences, resulting in a higher ratio of 30.42. This underscores the crucial importance of managing fuel quantity and continuity as key strategies for addressing the heightened wildfire risk resulting from climate change and rural abandonment (Moreno et al., 2014).
Throughout the period from 2010 to 2020, a significant portion of the 29,379.4 hectares within the Natural Park has endured multiple fire incidents, evident from the cumulative area burned, totaling 13,261.46 hectares. This extensive area represents almost half of the size of the Natural Park itself. While this calculation suggests that, theoretically, the entirety of study area could burn enterally over the next decade, it is essential to acknowledge that fires do not spread uniformly across the landscape. As depicted in Figure 8, certain areas have been subjected to repeated burns, exacerbating the impact on soils. The majority of the affected area within the six municipalities studied, except for the year 2016 y 2017, predominantly comprises herbaceous and shrubland areas (as illustrated in Figure 4). Within the shrublands, the area affected by fires approximates 14,642 hectares, contrasting with approximately 5,798 hectares forest areas burned within the municipalities. When firefighting efforts are deployed in open fields, priority is often given to safeguarding wooded areas, not only due to their intrinsic ecological value but also recognizing the challenge in preventing these regions from becoming engulfed in flames, particularly given the practice of using controlled burns to clear brush. The vegetation composition reflects the adaptation to the region’s fire history, predominantly composed of pyrophytic shrubs (Buján, 2010). However, these species, while resilient to fire, neither impede fire spread nor facilitate the movement of ground-based firefighting resources.
The analysis of land cover change provides valuable insights into the dynamics of vegetation within the study area. The observed shifts between the periods 2000–2010 and 2010–2020 indicate significant alterations in land cover composition, with implications for ecosystem resilience and fire susceptibility. During the first period, there was a noteworthy increase in shrubland coverage, accompanied by a reduction in rocky areas (Figures 5, 6). This trend suggests a potential encroachment of vegetation into previously bare soil or sparsely vegetated areas, likely influenced by factors such as post-fire conditions promoting plant growth (increased nutrient availability, favorable moisture and temperature conditions, etc.) (Chungu et al., 2020; Fernández-García et al., 2021; Blanco-Rodríguez et al., 2023). However, the subsequent decade showed a reversal of this trend, with a decrease in shrublands and a corresponding increase in rocky areas (Figure 6). In fact, some rocky areas were not able to evolve to shrubland, which might be suggesting a long-term loss of soil. Similar patterns were observed in evergreen forest cover, albeit to a lesser extent than shrubland. This indicates that forested areas within the study region are also undergoing dynamic changes, albeit at a slower pace compared to shrublands. The observed patterns were consistent within areas affected by wildfire between 2010 and 2020, suggesting that fire events may play a role in shaping land cover dynamics over time.
Fires effects on vegetation cover are coupled with changes belowground systems, in other words, soils. Soil quality and depth is essential for supporting a forest with herbaceous, shrub and tree vegetation. Besides destruction of vegetation cover, wildfires provoke physical, chemical and biological soil degradation as well as soil, C and nutrient losses by lixiviation and post-fire erosion (Dove et al., 2020; Agbeshie et al., 2022). Under unfavorable conditions (high severity fire, area highly susceptible to post-fire erosion due to high slope and abundant precipitations), wildfire can cause the loss of 2–2.5 cm of soil and repeated fire can lead to progressive thinning of soil and even to an irreversible total soil loss (Díaz-Raviña et al., 2012). These accumulative soil degradation processes provoked by altered fire regime could partially explain the observed land cover changes. During the 2000–2010 period, an increase in the bare soils with sparse vegetation was observed in the burnt areas, potentially reducing soil quality. In addition, during the 2010–2020 period, the intensification of fire severity and frequency has exposed the soil to progressive thinning and degradation. Consequently, the landscape can be modified by the increase of rockfalls associated with the occurrence of wildfires (Cristóbal et al., 2024). This issue is of particular concern in areas with the highest levels of protection, which are often exposed to large wildfires, and frequented by tourists, over the fire season.
The analysis also emphasized the impact of burn severity on land cover transitions. Regions experiencing higher burn severity exhibited a greater propensity for vegetation loss, resulting in shifts in land cover composition (see Figure 7). Nevertheless, the substantial rates of post-fire vegetation recovery observed in certain areas underscored the resilience of natural ecosystems to fire disturbances. Conversely, in other areas, the presence of shallow soil and sparse vegetation indicated severe soil degradation and even desertification attributed to the altered fire regime of the last decades.
Conclusion
Overall, our findings highlight the intricate relationships among wildfires and post-fire soil-vegetation dynamics within the Natural Park “Baixa Limia Serra do Xurés.” These dynamics are critical for effective wildfire management and conservation efforts, particularly in fire-prone regions.
Large fire events, particularly notable in 2011, 2016, 2017, and 2020, significantly impacted the landscape within the Natural Park, with the largest fire occurring in 2017 and affecting predominantly wooded areas. Large fires accounted for the majority of the burned area, emphasizing their significance in shaping landscape dynamics and posing challenges for fire suppression efforts. Spatial analysis revealed a substantial impact on shrubland covers, with exceptions noted in 2016 and 2017 where forested areas were significantly affected, highlighting the vulnerability of both shrublands and forests to wildfire events. Land cover transitions showed a clear association between areas previously affected by wildfires and subsequent changes in land cover composition, with burn severity influencing post-fire vegetation recovery rates. The surface area of rocky areas with sparse vegetation tends to increase, and the cover of shrublands and forests tends to decrease over time in areas affected by recurrent fires. This pattern suggests that erosion and soil degradation are key factor influencing post-fire vegetation composition. Advanced remote sensing techniques hold promise for improving the monitoring and prediction of post-fire recovery dynamics, aiding in fire hazard management and ecosystem restoration efforts. Analysis of fire seasonality suggests a potential lengthening of the fire season within the park, reflecting broader shifts in fire regimes attributed to climate change. Despite increased budgets and technology for fire suppression, the areas burnt by large fires are increasing in the natural park, underscoring the need for effective fire management strategies and landscape control to mitigate wildfire risks.
Our study provides a strong long-term and comprehensive analysis of the effects of fires on soil-vegetation dynamics, and their significance for protected areas. Such insights could prove invaluable for policymakers and stakeholders, guiding the development of more effective management strategies in fire-prone regions like the Natural Park “Baixa Limia Serra do Xurés.”
Data Availability Statement
The original contributions presented in the study are included in the article/Supplementary Material, further inquiries can be directed to the corresponding author.
Author Contributions
AR and MD-R designed the study; CG-R and AR conducted the analysis; CG-R, AR, and MD-R interpreted the results; CG-R wrote the first version of the manuscript later revised by MD-R and AR. All authors contributed to the article and approved the submitted version.
Funding
The author(s) declare that financial support was received for the research, authorship, and/or publication of this article. AR is supported by the ‘Juan de la Cierva’ and ‘Ramón y Cajal’ fellowship programs of the Spanish Ministry of Science and Innovation (IJC2019-041033-I and RYC2022-036822-I).
Conflict of Interest
The authors declare that the research was conducted in the absence of any commercial or financial relationships that could be construed as a potential conflict of interest.
Supplementary Material
The Supplementary Material for this article can be found online at: https://www.frontierspartnerships.org/articles/10.3389/sjss.2024.13103/full#supplementary-material
References
Agbeshie, A. A., Abugre, S., Atta Darkwa, T., and Awuah, R. (2022). A Review of the Effects of Forest Fire on Soil Properties. J. For. Res. 33, 1419–1441. doi:10.1007/s11676-022-01475-4
Barreiro, A., and Díaz-Raviña, M. (2021). Fire Impacts on Soil Microorganisms: Mass, Activity, and Diversity. Curr. Opin. Environ. Sci. Health 22 (August), 100264. doi:10.1016/j.coesh.2021.100264
Blanco-Rodríguez, M. Á., Ameztegui, A., Gelabert, P., Rodrigues, M., and Coll, L. (2023). Short-Term Recovery of Post-Fire Vegetation Is Primarily Limited by Drought in Mediterranean Forest Ecosystems. Fire Ecol. 19 (1), 68. doi:10.1186/s42408-023-00228-w
Bond, W. J., and Keeley, J. E. (2005). Fire as a Global ‘herbivore’: The Ecology and Evolution of Flammable Ecosystems. Trends Ecol. Evol. 20 (7), 387–394. doi:10.1016/j.tree.2005.04.025
Bowman, D. M. J. S., Balch, J., Artaxo, P., Bond, W. J., Cochrane, M. A., Antonio, C. M. D., et al. (2011). The Human Dimension of Fire Regimes on Earth, 2223–2236. doi:10.1111/j.1365-2699.2011.02595.x
Buján, E. (2010). Relaciones Suelo-Planta en Ecosistemas de Brezales Atlánticos de Galicia Tesis doctorales. Universidade de Santiago de Compostela, Servizo de Publicacións e Intercambio Científico.
Calheiros, T., Benali, A., Pereira, M., Silva, J., and Nunes, J. (2022). Drivers of Extreme Burnt Area in Portugal: Fire Weather and Vegetation. Nat. Hazards Earth Syst. Sci. 22 (12), 4019–4037. doi:10.5194/nhess-22-4019-2022
Cánibe, M., Titeux, N., Domínguez, J., and Regos, A. (2022). Assessing the Uncertainty Arising From Standard Land-Cover Mapping Procedures When Modelling Species Distributions. Divers. Distributions 28 (4), 636–648. doi:10.1111/DDI.13456
Certini, G. (2005). Effects of Fire on Properties of Forest Soils: A Review. Oecologia 143 (1), 1–10. doi:10.1007/s00442-004-1788-8
Certini, G., Moya, D., Lucas-Borja, M. E., and Mastrolonardo, G. (2021). The Impact of Fire on Soil-Dwelling Biota: A Review. For. Ecol. Manag. 488, 118989. doi:10.1016/j.foreco.2021.118989
Chandler, C., Cheney, P., and Thomas, P. (1983). Forest Fire Behavior and Effects. John Wiley & Sons.
Chu, T., Guo, X., and Takeda, K. (2017). Effects of Burn Severity and Environmental Conditions on Post-Fire Regeneration in Siberian Larch Forest Effects of Burn Severity. Forest 8, 66. doi:10.3390/f8030076
Chungu, D., Ng’andwe, P., Mubanga, H., and Chileshe, F. (2020). Fire Alters the Availability of Soil Nutrients and Accelerates Growth of Eucalyptus Grandis in Zambia. J. For. Res. 31 (5), 1637–1645. doi:10.1007/s11676-019-00977-y
Cristóbal, A., Rigueira, X., Pérez-Rey, I., Estévez-Ventosa, X., Pazo, M., Napoli, M. L., et al. (2024). Automatic Characterization of Block-In-Matrix Rock Outcrops Through Segmentation Algorithms and Its Application to an Archaeo-Mining Case Study. Geosciences 14, 29. doi:10.3390/geosciences14020029
Díaz-Raviña, M., Fontúrbel, M., Martín, A., and Fernández, C. (2021). “Rehabilitation of Forest Soils Affected by Wildfires,” in Recarbonizing Global Soils: A Technical Manual of Recommended Management Practices, Volume 5. Forestry, Wetlands, Urban Soils – Practices Overview (Rome: FAO), 91–109. doi:10.4060/cb6606enç
Díaz-Raviña, M., Martín, A., Barreiro, A., Lombao, A., Iglesias, L., Díaz-Fierros, F., et al. (2012). Mulching and Seeding Treatments for Post-Fire Soil Stabilisation in NW Spain: Short-Term Effects and Effectiveness. Geoderma 191, 31–39. doi:10.1016/j.geoderma.2012.01.003
Dove, N. C., Klingeman, D. M., Carrell, A. A., Cregger, M. A., and Schadt, C. W. (2022). Fire Alters Plant Microbiome Assembly Patterns: Integrating the Plant and Soil Microbial Response to Disturbance. New Phytol. 230, 2433–2446. doi:10.1111/nph.17248
Dove, N. C., Safford, H. D., Bohlman, G. N., Estes, B. L., and Hart, S. C. (2020). High-Severity Wildfire Leads to Multi-Decadal Impacts on Soil Biogeochemistry in Mixed-Conifer Forests. Ecol. Appl. 30 (4), e02072. doi:10.1002/eap.2072
Fernandez-Anez, N., Krasovskiy, A., Müller, M., Vacik, H., Baetens, J., Hukić, E., et al. (2021). Current Wildland Fire Patterns and Challenges in Europe: A Synthesis of National Perspectives. Air, Soil Water Res. 14, 117862212110281. doi:10.1177/11786221211028185
Fernández-García, V., Marcos, E., Huerta, S., and Calvo, L. (2021). Soil-Vegetation Relationships in Mediterranean Forests After Fire. For. Ecosyst. 8 (1), 18. doi:10.1186/s40663-021-00295-y
Flannigan, M., Cantin, A. S., de Groot, W. J., Wotton, M., Newbery, A., Gowman, L. M., et al. (2013). Global Wildland Fire Season Severity in the 21st Century. For. Ecol. Manag. 294, 54–61. doi:10.1016/j.foreco.2012.10.022
Francos, M., Úbeda, X., Pereira, P., and Alcañiz, M. (2018). Long-Term Impact of Wildfire on Soils Exposed to Different Fire Severities. A Case Study in Cadiretes Massif (NE Iberian Peninsula). Sci. Total Environ. 615, 664–671. doi:10.1016/j.scitotenv.2017.09.311
de Galicia, X. D. (2023). Memoria del Pladiga 2023. Retrieved from: https://ficheiros-web.xunta.gal/transparencia/plans/medio-rural/01_Memoria_Pladiga_2023_Cast.pdf.
Galicia, X. D. (2018). Memoria del Pladiga 2023. Retrieved from: https://ficheiros-web.xunta.gal/transparencia/plans/medio-rural/01_Memoria_Pladiga_2018_Cast.pdf.
García-Redondo, C., Fernández-Moure, P., Cánibe, M., Tapia, L., Gil-Carrera, A., Lombao, A., et al. (2023). Burn Severity and Land-Use Legacy Influence Bird Abundance in the Atlantic-Mediterranean Biogeographic Transition. Environ. Res. 233, 116510. doi:10.1016/j.envres.2023.116510
Girona-García, A., Vieira, D. C. S., Silva, J., Fernández, C., Robichaud, P. R., and Keizer, J. J. (2021). Effectiveness of Post-Fire Soil Erosion Mitigation Treatments: A Systematic Review and Meta-Analysis. Earth-Science Rev. 217, 103611. doi:10.1016/j.earscirev.2021.103611
Guz, J., Sangermano, F., and Kulakowski, D. (2022). The Influence of Burn Severity on Post-Fire Spectral Recovery of Three Fires in the Southern Rocky Mountains. Remote Sens. 14, 1363. doi:10.3390/rs14061363
Hinojosa, M. B., Parra, A., Laudicina, V. A., and Moreno, J. M. (2016). Post-Fire Soil Functionality and Microbial Community Structure in a Mediterranean Shrubland Subjected to Experimental Drought. Sci. Total Environ. 573, 1178–1189. doi:10.1016/j.scitotenv.2016.03.117
Iglesias, V., Balch, J. K., and Travis, W. R. (2022a). U.S. Fires Became Larger, More Frequent, and More Widespread in the 2000s. Sci. Adv. 8 (11), eabc0020–18. doi:10.1126/sciadv.abc0020
Keeley, J. E., and Pausas, J. G. (2022). Evolutionary Ecology of Fire. Annu. Rev. Ecol. Evol. Syst. 53, 203–225. doi:10.1146/annurev-ecolsys-102320-095612
Linley, G. D., Jolly, C. J., Doherty, T. S., Geary, W. L., Armenteras, D., Belcher, C. M., et al. (2022). What do you mean, ‘megafire’?Glob. Ecol. Biogeogr. 31 (10), 1906–1922.
Lombao, A., Barreiro, A., Cancelo-González, J., Martín, A., and Díaz-Raviña, M. (2015a). Impact of Thermal Shock on Forest Soils Affected by Fires of Different Severity and Recurrence. Span. J. Soil Sci. 5, 165–179. doi:10.3232/sjss.2015.v5.n2.06
Lombao, A., Barreiro, A., Carballas, T., Fontúrbel, M. T., Martín, A., Vega, J. A., et al. (2015b). Changes in Soil Properties After a Wildfire in Fragas Do Eume Natural Park (Galicia, NW Spain). Geoderma 135, 409–418. doi:10.1016/j.catena.2014.08.007
Lombao, A., Barreiro, A., Fontúrbel, M. T., Martín, A., Carballas, T., and Díaz-Raviña, M. (2020). Key Factors Controlling Microbial Community Responses After a Fire: Importance of Severity and Recurrence. Sci. Total Environ. 741, 140363. doi:10.1016/j.scitotenv.2020.140363
Macedo, A., Tavares, A., Fontes, A., Pinto, C., Rodrigues, C., Machado, C., et al. (2009). Propuesta para la creación de la Reserva de la Biosfera Transfronteriza Gerês/Xurês. Retrieved from: http://www.ibader.org/archivos/docs/mab geres xures 09pdf (Accessed February 15, 2023)
Marlon, J. R., Bartlein, P. J., Daniau, A.-L., Harrison, S. P., Maezumi, S. Y., Power, M. J., et al. (2013). Global Biomass Burning: A Synthesis and Review of Holocene Paleofire Records and Their Controls. Quat. Sci. Rev. 65, 5–25. doi:10.1016/j.quascirev.2012.11.029
Martín, A., Díaz-Raviña, M., and Carballas, T. (2009). Evolution of Composition and Content of Soil Carbohydrates Following Forest Wildfires. Biol. Fertil. Soils 45, 511–520. doi:10.1007/s00374-009-0363-1
Martín, A., Díaz-Raviña, M., and Carballas, T. (2012). Short- and Medium-Term Evolution of Soil Properties in Atlantic Forest Ecosystems Affected by Wildfires. Land Degrad. Dev. 23, 427–439. doi:10.1002/ldr.1078
Mataix-Solera, J., Guerrero, C., García-Orenes, F., Bárcenas, G. M., and Torres, M. P. (2009). “Forest Fire Effects on Soil Microbiology,” in Fire Effects on Soil and Restoration Strategies. Editors A. Cerdá, and P. R. Robichaud (Enfield, USA: Science Publishers), 133–175.
MITECO (2023). URL Estadísticas de Incendios Forestales. Available at: https://www.miteco.gob.es/es/biodiversidad/estadisticas/Incendios_def ault.aspx (Accessed June 25, 2023).
Moreira, F., Viedma, O., Arianoutsou, M., Curt, T., Koutsias, N., Rigolot, E., et al. (2011). Landscape-Wildfire Interactions in Southern Europe: Implications for Landscape Management. J. Environ. Manag. 92, 2389–2402. doi:10.1016/j.jenvman.2011.06.028
Moreno, J. M., Urbieta, R. I., Franquesa, M., and Viedma, O. (2019). Fire Activity and Burned Forest Lands Decreased During the Last Three Decades in Spain. Ann. For. Sci. 76, 90. doi:10.1007/s13595-019-0874-3
Moreno, M. V., Conedera, M., Chuvieco, E., and Pezzatti, G. B. (2014). Fire Regime Changes and Major Driving Forces in Spain From 1968 to 2010. Environ. Sci. Policy 37, 11–22. doi:10.1016/j.envsci.2013.08.005
Moritz, M. A., Parisien, M. A., Batllori, E., Krawchuk, M. A., Dorn, J. V., Ganza, D. J., et al. (2012). Climate Change and Disruptions to Global Fire Activity. Ecosphere 3, 1–22. doi:10.1890/es11-00345.1
Neary, D. G., Klopatek, C. C., DeBano, L. F., and Ffolliott, P. F. (1999). Fire Effects on Belowground Sustainability: A Review and Synthesis. For. Ecol. Manag. 122, 51–71. doi:10.1016/s0378-1127(99)00032-8
Pausas, J. G., and Fernández-Muñoz, S. (2012). Fire Regime Changes in the Western Mediterranean Basin: From Fuel-Limited to Drought-Driven Fire Regime. Clim. Change 110 (1–2), 215–226. doi:10.1007/s10584-011-0060-6
Pereira, P., Bogunovic, I., Muñoz-Rojas, M., and Brevick, E. C. (2018). Soil Ecosystem Services, Sustainability, Valuation and Management. Curr. Opin. Environ. Sci. Health 5, 7–13. doi:10.1016/j.coesh.2017.12.003
Perez-Rey, I., Sarro, R., Tomas, R., Alejano, L., Hernandez Gutierrez, L., Mateos, R., et al. (2023). A Brief Review of the Effect of Wildfires on Rockfall Occurrence. IOP Conf. Ser. Earth Environ. Sci. 1124, 012122. doi:10.1088/1755-1315/1124/1/012122
Pressler, Y., Moore, J. C., and Cotrufo, M. F. (2019). Belowground Community Responses to Fire: Meta-Analysis Reveals Contrasting Responses of Soil Microorganisms and Mesofauna. Oikos 128, 309–327. doi:10.1111/oik.05738
Regos, A., and Díaz-Raviña, M. (2023). A Storyboard of Wildfires in Galicia. in The Environment in Galicia: A Book of Images: Galician Environment Through Images. Editors A. Núñez-Delgado, E. Álvarez-Rodríguez, and D. Fernández-Calviño (Springer International Publishing), 551–596). doi:10.1007/978-3-031-33114-5_26
Rodrigues, M., Cunill Camprubí, À., Balaguer-Romano, R., Coco Megía, C. J., Castañares, F., Ruffault, J., et al. (2023). Drivers and Implications of the Extreme 2022 Wildfire Season in Southwest Europe. Sci. Total Environ. 859 (February), 160320–160326. doi:10.1016/j.scitotenv.2022.160320
Rodríguez-Lado, L. M., Perez-Rodríguez, M., Taboada-Rodríguez, T., Martínez Cortizas, A., and Macias Vazquez, F. (2018). Atlas digital de Propiedades de Suelos de Galicia. Servizo de Publicacións e Intercambio Científico Campus Vida. doi:10.15304/9788416533848
Salis, M., Ager, A., Finney, M., Arca, B., and Spano, D. (2013). Analyzing Spatiotemporal Changes in Wildfire Regime and Exposure Across a Mediterranean Fire-Prone Area. Nat. Hazards 71, 1389–1418. doi:10.1007/s11069-013-0951-0
San-Miguel-Ayanz, J., and Camia, A. (2009). “Forest Fires at a Glance: Facts, Figures and Trends in the EU,” in Living With Wildfires: What Science Can Tell Us. Editor Y. Birot (European Forest Institute).
San-Miguel-Ayanz, J., Durrant, T., Boca, R., Maianti, P., Libertà, G., Artés Vivancos, T., et al. (2021). Forest Fires in Europe, Middle East and North Africa 2020. Publications Office of the European Union. doi:10.2760/216446
San-Miguel-Ayanz, J., Durrant, T., Boca, R., Maianti, P., Libertà, G., Oom, D., et al. (2023). Forest Fires in Europe, Middle East and North Africa 2022. Publications Office of the European Union. doi:10.2760/348120
Seijo, F. (2005). The Politics of Fire: Spanish Forest Policy and Ritual Resistance in Galicia, Spain. Environ. Polit. 14 (3), 380–402. doi:10.1080/09644010500087665
Shakesby, R. A. (2011). Post-Wildfire Soil Erosion in the Mediterranean: Review and Future Research Directions. Earth-Science Rev. 105 (3–4), 71–100. doi:10.1016/j.earscirev.2011.01.001
Tedim, F., Remelgado, R., Borges, C., Carvalho, S., and Martins, J. (2013). Exploring the occurrence of mega-fires in Portugal. For. Ecol. Manag. 294, 86–96.
Torres, J., Gonçalves, J., Bruno, M., and Horando, J. (2018). Indicator-Based Assessment of Post-Fire Recovery Dynamics Using Satellite NDVI Time-Series. Ecol. Indic. 89, 199–212. doi:10.1016/j.ecolind.2018.02.008
Tyukavina, A., Potapov, P., Hansen, M. C., Pickens, A. H., Stehman, S. V., Turubanova, S., et al. (2022). Global Trends of Forest Loss Due to Fire From 2001 to 2019. Front. Remote Sens. 3 (March), 1–20. doi:10.3389/frsen.2022.825190
Viana-Soto, A., Aguado, I., and Martínez, S. (2017). Assessment of Post-Fire Vegetation Recovery Using Fire Severity and Geographical Data in the Mediterranean Region (Spain). Environments 4, 90. doi:10.3390/environments4040090
Villar, M. C., Petrikova, V., Díaz-Raviña, M., and Cslarballas, T. (2004). Changes in Soil Microbial Biomass and Aggregate Stability Following Burning and Soil Rehabilitation. Geoderma 122, 73–82. doi:10.1016/j.geoderma.2003.12.005
Wickham, H. (2009). ggplot2: Elegant Graphics for Data Analysis. Springer New York, NY. doi:10.1007/978-0-387-98141-3
Yuan, Z., Ali, A., Ruiz-Benito, P., Jucker, T., Mori, A. S., Loreau, M., et al. (2020). Above- and Below-Ground Biodiversity Jointly Regulate Temperate Forest Multifunctionality Along a Local-Scale Environmental Gradient. J. Ecol. 108, 2012–2024. doi:10.1111/1365-2745.13378
Zhang, Y., and Biswas, A. (2017). The Effects of Forest Fire on Soil Organic Matter and Nutrients. in Boreal Forests of North America: A Review. BT - Adaptive Soil Management: From Theory to Practices. Editors A. Rakshit, P. C. Abhilash, H. B. Singh, and S. Ghosh (Springer), 465–476. doi:10.1007/978-981-10-3638-5_21
Keywords: burn severity, fire recurrence, protected areas, remote sensing, soil degradation
Citation: García-Redondo C, Díaz-Raviña M and Regos A (2024) Long-Term Cumulative Effects of Wildfires on Soil-Vegetation Dynamics in the “Baixa Limia–Serra do Xurés” Natural Park. Span. J. Soil Sci. 14:13103. doi: 10.3389/sjss.2024.13103
Received: 07 April 2024; Accepted: 23 July 2024;
Published: 07 August 2024.
Edited by:
Diana Vieira, Joint Research Centre, ItalyCopyright © 2024 García-Redondo, Díaz-Raviña and Regos. This is an open-access article distributed under the terms of the Creative Commons Attribution License (CC BY). The use, distribution or reproduction in other forums is permitted, provided the original author(s) and the copyright owner(s) are credited and that the original publication in this journal is cited, in accordance with accepted academic practice. No use, distribution or reproduction is permitted which does not comply with these terms.
*Correspondence: Adrián Regos, aregos@mbg.csic.es
†ORCID: Adrián Regos, orcid.org/0000-0003-1983-936X