- 1Soil and Water Research Group, University of Lleida, Lleida, Spain
- 2isardSAT SL, Barcelona, Spain
When irrigation is introduced in a region, it adds salts and mobilizes those already present in the soil, changing the soil-plant-atmosphere continuum. These changes may lead to higher salt loads in the drainage water which, in turn, can have an impact on waters further downstream. Knowing the dynamics of these loads at the sub-basin scale is key to accounting for the possible impact that irrigation may have and to determining what improvements could be applied. This study aimed to characterise the different salt types present and to investigate drainage salt loads and their dependence upon irrigation input and their implications in irrigation management in a new, and well-managed, 8000 ha irrigation district located in the Ebro basin, NE Spain. In addition, it is relevant to highlight that the Ebro basin suffered a period of significant drought in 2023. As a result, irrigation restrictions were applied in many irrigation districts. We sought to investigate how these reductions influenced irrigation return flows and salt discharges within a selected irrigation district. The present study was undertaken during the irrigation periods of 2021, 2022, and 2023. We monitored water inputs and outputs in two representative sub-basins belonging to the Algerri-Balaguer irrigation district (Ebro basin, NE Spain). We also analysed water inputs and outputs in order to characterise and establish the salt balances in both of the sub-basins that we studied. Our results showed that during 2023, a reduction in irrigation delivery of 31% led to a reduction in drainage volume of 73%, resulting in a decrease in salt exports through drainage of 70%. These data revealed that the application of irrigation restrictions not only led to an increased availability of downstream water resources, but also to a decrease in drainage volume and salt load, resulting in an improvement in irrigation management by farmers. However, it should also be underlined that this drastic reduction in irrigation was, in turn, responsible for a reduction in yield. Re-utilising drainage waters and/or improving irrigation management at the field scale may help to find this point of balance in areas with high water demands.
Introduction
The introduction of irrigation in a region has a significant impact on the natural dynamics of its environment, including its soils (Murray and Grant, 2007; Hillel et al., 2008; Cox et al., 2018). In Mediterranean areas, irrigation plays a crucial role in enabling high crop yields and promoting social and economic development (FAO, 2002). These areas typically have soils that are rich in ions, because the dry climate prevents them from being washed out. The aridity of their climates is characterized by low levels of precipitation (P) compared to evapotranspiration (ET) (Soil Survey Staff, 2022). Under dry-land conditions, the balance between evapotranspiration and precipitation leads to a deficit in water availability in the soil and there is little possibility of salt leaching. The application of irrigation water leads to a significant change in soil water dynamics. Irrigation, which may also contain high levels of dissolved salts, allows excess water to carry salts away from the root zone more efficiently. The movement of water from the soil to lower areas, aided by drainage systems, facilitates the removal of salts from both the irrigated fields and the entire irrigated area. This, helps to prevent soil salinisation and water-logging (Fereres et al., 2011).
Numerous studies have been conducted to address this issue. In irrigated Mediterranean regions, and specifically in the Ebro basin, salt removal in irrigated land has been reported to range from 0.5 (Andrés and Cuchí, 2014) to 14.5 t.
Understanding the quality and quantity of drainage water within an irrigation district is crucial for determining opportunities for water reuse, evaluating potential impacts on water bodies, and assessing the sustainability of irrigation practices related to soil and water quality.
It is on these questions that this study is focused. We wanted to investigate how a reduction in irrigation water use affects salinity and volume of drainage water in new irrigated areas where land consolidation has been carried out and a drainage network has been designed to allow for monitoring. To do so, this study focused on an irrigation district that, due to the drought in the Ebro basin, suffered a 25% restriction (from a theoretical maximum allocation of 48
Material and Methods
Area Studied
Land Use and Irrigation Management in the Area Studied
The research area is located in the Algerri-Balaguer irrigation district, which is part of the River Ebro basin in northeastern Spain. This district was established in 1998 and has a total irrigable area of 8,000 ha.
The maximum water usage in this district is 48
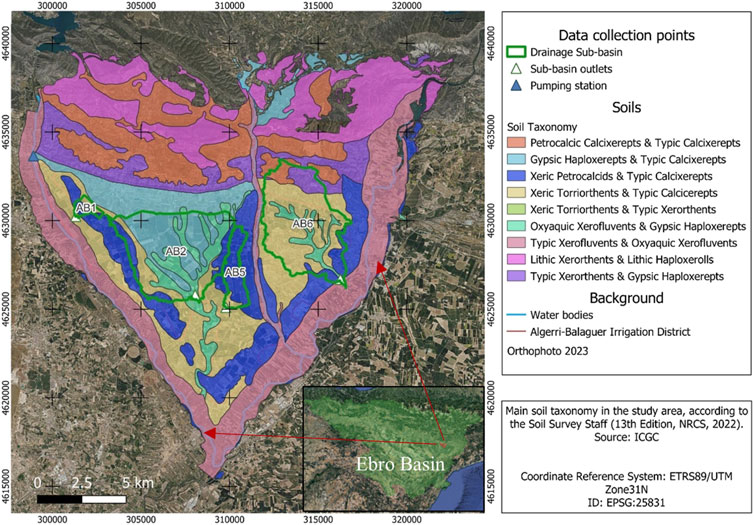
Figure 1. The sub-basins studied, main soil mapping units in the study area, and data collection points.
The predominant land use in this district is double cropping, with winter cereals or peas (to a lesser degree) being grown in winter, followed by maize in summer. Double cropping accounts for over 50% of land use in the irrigated area. Orchard crops, such as apples, almonds and olives, are also grown in this district.
To conduct the study within the irrigation district, four drainage sub-basins were selected. These were defined using the “Fill sinks” (Wang and Liu, 2006) algorithm in QGIS 3.24.3 software. The digital elevation model of the study zone used for this purpose was obtained from Institut Cartogràfic i Geològic de Catalunya (2020). The “SAGA Terrain Analysis: Channel Network and Drainage Basins” toolbox was then used to obtain the final drainage sub-basin areas. The specific drainage sub-basins can be seen in Figure 1; Table 1.
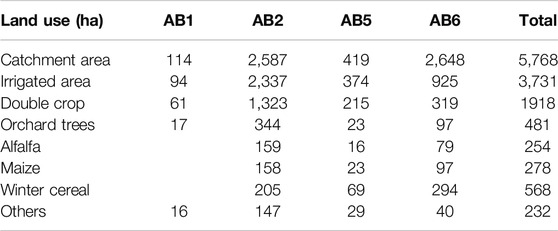
Table 1. Catchment area and land use in the four sub-basins studied within the Algerri-Balaguer irrigation district.
The extension, land use and acreage of the sub-basins which were studied are shown in Table 1. Land use data were obtained from the DUN-SIGPAC data set provided by the Departament d’Acció Climàtica (2021) and validated, for each study year, using in situ data provided by technicians working in the Algerri-Balaguer irrigation district.
Weather and Climate
The study area has a dry, continental Mediterranean climate, with cold winters and hot, dry summers. Its average air temperature is 14.4°C. Its average annual rainfall and reference evapotranspiration are 378 mm and 1,072 mm, respectively (2000–2022), as measured at the agrometeorological station of Albesa (RuralCat Departament d’Acció Climàtica Alimentació i Agenda Rural, 2023). Rainfall is predominantly concentrated during the spring and autumn seasons. During the study period, annual rainfall was below average, with values of 333, 298, and 237 mm, for 2021, 2022, and 2023, respectively (Figure 2).
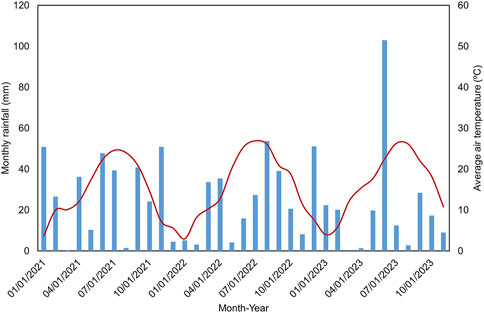
Figure 2. Monthly rainfall and average air temperature in the study area during the study period, according to the agrometeorological station at Albesa.
Geology and Soils
From a geological point of view, the study site is located in the Piedmont zone, between the expansive Pre-Pyrenean Mountain system, which is characterised by imposing mountain ranges, and the Tertiary depression, which is dominated by the Ebro Valley. Serra Llarga marks its northern boundary, corresponding to the southern slopes of the Barbastro-Balaguer Anticline, with the Alfarràs plateau connecting it to lower-lying areas through fluvial deposits and glacis. To the south, the study area is bordered by the central-eastern depression, which is traversed by the Segre and Noguera Ribagorçana rivers, which have formed prominent, stepped terraces.
Geologically, the region’s stratigraphy comprises Tertiary materials, which progress from a gypsum base and include two detrital units. The lower unit includes stratified limestones, marls, flint intercalations, sandstones alternating with silts and clays, and carbonate levels. Upon this lies the upper detrital unit, which features paleochannel deposits of sandstones, silts, and red clays, alternating with sandstone bars and conglomerates. Quaternary deposits, characterised by terraces and colluvial deposits have also been identified in the study area (Gil et al., 1998).
Hydrologically, the study region falls within the basins of the rivers Segre and Noguera Ribagorçana. In terms of hydrogeology, the aquifer system of the Ebro’s alluvial terraces, is divided into two aquifers: the lower alluvial terrace of the River Noguera Ribagorçana, with its current floodplain, and the last alluvial terrace of the River Segre. In both cases, the impermeable substrate consists of tertiary lithologies, such as clays, gypsum, and silts. Recharge mechanisms encompass direct infiltration from rivers, rainwater infiltration, limited infiltration from lateral torrents, and predominant infiltration from irrigation returns (Mensua et al., 1977).
However, this aquifer system is not extensive in the studied sub-basins, as it is confined to the terraces of rivers Noguera Ribagorçana, Farfanya, and Segre (areas limited by Typic Xerofluvents and Oxyaquic Xerofluvents, in Figure 1). Thus, there is no interaction with groundwater in the study area.
The soils in the study area have been widely studied. Field studies dating from 1984–1986; 1990–1991; 1994; and 2017–2018 resulted in a detailed 1:25,000 soil map (Institut Cartogràfic i Geològic de Catalunya, 2019), which is available online.
The soils in the Algerri Balaguer irrigation district have pHs ranging from of 7.6–8.5, with high levels of calcium carbonate (15%–50%) and low levels of organic matter (0.2%–3%). The soil textures are mainly loam, clay-loam, silt, and silty-clay-loam. Soil depths vary, being generally deep (
Secondary accumulations of calcium carbonate often manifest as nodules (calcic horizons), and in cases where coarse elements are abundant, petrocalcic horizons may form. It is also common to find crystals and secondary accumulations of vermiform gypsum, which have formed gypsic horizons. This has occurred in soils that have developed on detrital materials originating from the residual platforms of alluvial deposits in the foothills of the Serra Llarga range. According to the parental material, there are also distinctive sequences of horizons, with the most common ones being Ap - Bwkn(y)/Bw/Bkm/By - 2C/2R.
The soils in the study area are mainly classified as Gypsic Haploxerepts, Typic Calcixerepts, Xeric Torriorthents or Xeric Petrocalcids, among others, according to the Soil Survey Staff (Soil Survey Staff, 2022). Further information on the different soil taxonomies present in the area can be found in Figure 1.
Sampling Methodology
Throughout 2021, 2022, and 2023, the volumes of irrigation water used, precipitation and the amount of water drained were measured. Complete water analyses were also carried out periodically in order to determine the main quality parameters.
Irrigation Water
As already mentioned in 2.1.1, the Algerri-Balaguer irrigation district has a maximum water use of 48
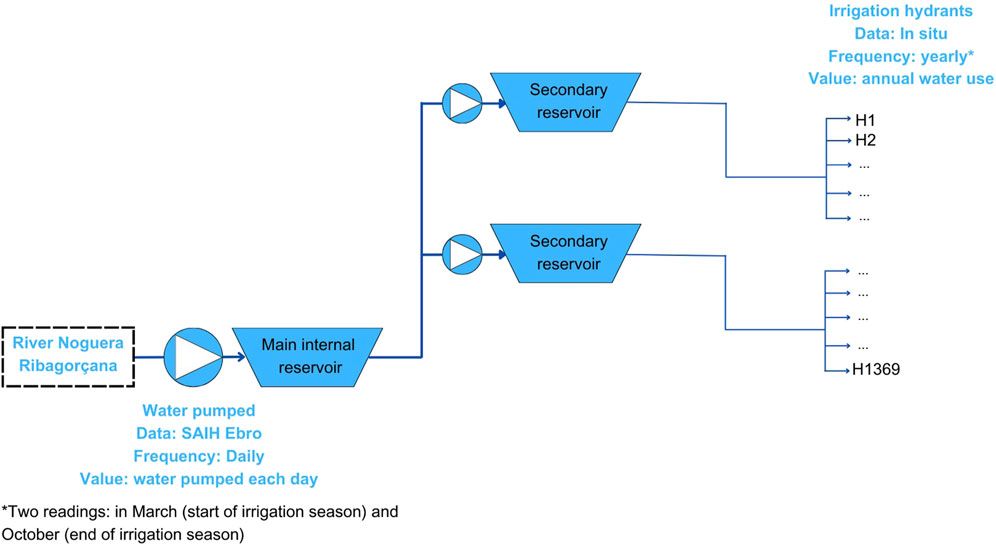
Figure 3. Diagram showing the irrigation network of the Algerri Balaguer irrigation district and the points from which the water volume data were collected.
The farmers connect their systems to the irrigation district network of hydrants, which are equipped with flow-measuring devices. A total of 1,369 hydrants were installed in the Algerri Balaguer irrigation district during its development. Non-revenue water (NRW), which is also known as unregistered water, is the difference between the volume of water pumped into the water distribution system and that which reaches the hydrant. It has been estimated as representing 8% in 2021 and 2022 (Olivera-Guerra et al., 2023) and 7% in 2023.
The Algerri-Balaguer irrigation season starts on 5th March and ends on 15th October. To monitor yearly water use and provide a tool for water accounting, each hydrant is checked before the start and after the end of the irrigation season. This enables the irrigation district technicians to accurately monitor the amount of irrigation water used from each hydrant and its associated field. The irrigation district makes two charges for supplying water. One is fixed and depends on the total acreage irrigated and the other is variable and depends on the total volume of water used.
With this information, and knowing the location of each hydrant, it is possible to determine the total irrigation water input for each sub-basin and year. In addition, it is possible to obtain average values of water consumption for each crop as recorded at the hydrant.
The total amount of water used per sub-basin and day can be determined by considering both the daily volume of pumped water and the yearly use per hydrant. We assume that daily pattern water consumption is consistent across all the sub-basins studied.
Monitoring Drainage Volume and Water Quality
During the 2021, 2022, and 2023 irrigation seasons, the water level and electrical conductivity (EC) at the outlets from the four sub-basins (Figure 1) were continuously monitored, using a Hydros21 sensor (METERGroup, USA). This sensor provides hourly average data about water level (mm), electrical conductivity (EC, dS·
However, among the four sub-basins, reliable flow estimates are only available for outlets AB1 and AB2, which were used for determining the salt and water balances. When characterising the quality of the drainage water, data from all of the four monitored sub-basin were used.
Drainage water quality was monitored in two different ways. On the one hand, continuous hourly EC (dS·
Salt Balances
A total of six salt balances were carried out. Three were obtained for sub-basin AB1 and another three for sub-basin AB2. They were obtained for the irrigation periods in the years 2021, 2022, and 2023: from 5th March to 15th October in each year.
The salt balance (
In the case of the present study, the main salt inputs were irrigation (
It should be noted that salt exports (SO) correspond exclusively to the salt load of the drainage water, as other components of the balance, such as salt removal by crop uptake, are considered negligible. In the case of groundwater, there was no evidence of the aquifers in the sub-basins studied (Agència Catalana de l’Aigua, 2013; Instituto Geológico y Minero de España and Dirección General del Agua, 2010; Ministerio para la Transición Ecológica y el Reto Demográfico, 2022). In these cases, the resulting salt balance would be provided by Equation 1.
Water and salt balance calculations were conducted for the entire basin. The results were then divided by the irrigated area, yielding the average salt balance per field, expressed in kg.
We also performed a separate balance calculation for each ion in order to know which ions were exported through drainage water and which remained in the soil.
Leaching Fraction
The leaching fraction (LF) represents the depth of water that leached below the root zone compared to the total depth of water applied (Ayers and Wescot, 1985) and is provided by Equation 2. In the case of irrigated agriculture, the total volume of water applied is the sum of irrigation and rainfall. In the present study, the amount of leached water accounts for drainage water.
Irrigation adds salts to the soil, which can potentially decrease crop yields in the medium term. Additionally, in calcareous soils, irrigation can dissolve existing salts in the soil. To maintain soil quality, these salts must be removed, a process defined as leaching requirements (LR) (Rhoades, 1974; Doorenbos and Pruitt, 1977; Ayers and Wescot, 1985). As long as the leaching fraction (LF) is greater than or equal to the leaching requirement (LR), there will be no accumulation of salts from the irrigation water, and existing salts in the soil will be leached away. If this situation persists for extended periods, salts from irrigation water will accumulate in the soil, leading to processes known as secondary soil salinisation. Amongst other things, this will adversely affect the yields of non-salt-tolerant crops.
In this study, the leaching fractions (LF) were estimated and compared with the leaching requirements (LR), which can be calculated using models. We employed the model proposed by Rhoades (1974) for these estimations, following Equation 3,
With
According to the Rhoades model, the salt leaching requirement with an irrigation water electrical conductivity of 0.37 dS·
Results and Discussion
Water Quality Data
Figure 4 presents the Piper diagram (Piper, 1944) for the four sub-basins studied, along with the irrigation water used in the Algerri-Balaguer.
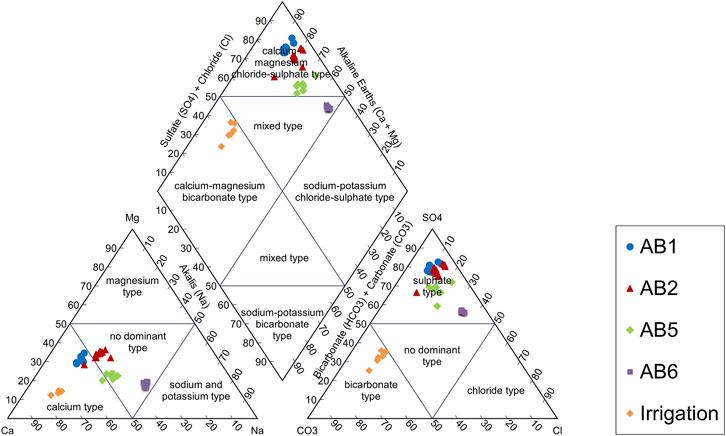
Figure 4. Piper diagram of irrigation water and the different drainage water in the sub-basins studied. Template by (Stosch, 2022).
The Piper diagram is a graphic procedure for geochemical interpretation of water analysis. It is useful for observing and describing possible differences between samples and sources, in terms of their chemistry. It shows the relative proportions of cations and anions and their hydrochemical characteristics.
The Piper diagram illustrates the differences in ionic composition between the irrigation water and the drainage water. In the case of the cations, the irrigation water exhibited a predominance of calcium. In the drainage water, there is no dominant cation type. This discrepancy was primarily due to the elevated levels of

Table 2. Mean and standard deviation of the main ions analysed in the different sample points. Values for ions, expressed in
The irrigation water showed characteristics of a calcium-magnesium bicarbonate type. Conversely, the drainage water exhibited a composition dominated by calcium, magnesium, chlorine, and sulfate ions. However, in the case of AB6, there was a mixed type, attributable to the presence of elevated levels of Na+ and Cl−. These ions are present in the soils classified as Xeric Torriorthents that predominate in this sub-basin (Figure 1).The differences in composition between the irrigation and drainage waters was a result of the dissolution of salts present in the soil; this process is intensified with higher water inputs, such as the application of irrigation.
On the other hand, we established a relationship between the total dissolved ions
When assessing most salt balances, it is common to use total dissolved solids (TDS) for this purpose, establishing the relationship at 640 mg·
In Figure 5 we show the TDI-EC relationship for water in the four sub-basins studied and for the irrigation water.
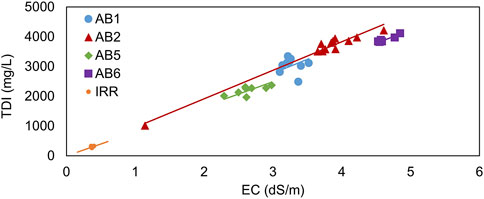
Figure 5. Relationship between total dissolved ions (TDI, mg·
All the relationships were higher than 640 mg·
In Table 2, we also indicate the SAR (sodium adsorption ratio) and EC (electrical conductivity) of each sample site. It is important to note that in AB1, AB,2 and AB5, the SAR values did not exceed 3, while the TDI values ranged between 1,000 and 4,500 mg·
This, combined with the relatively low EC values—especially for drainage waters—suggests that in certain cases, this water could be reused for irrigation, with appropriate irrigation management. However, in the case of AB6, we observed higher SAR values (6.9) accompanied by slightly higher EC values (4.6 dS·
These data were compared to a previous study conducted in the area between 2006 and 2008 by Villar et al. (2015). They monitored outlets AB1 and AB2 when the irrigated area had been newly stablished (and covered 4,700 ha in the AB district compared to the present 7,000 ha). In Table 3 we present some of the water quality results obtained in their study, and compared with the present one for sub-basin AB2. In Table 3 we can observe a reduction in CE and in SAR values, accompanied with a reduction of concentration in Na+ and
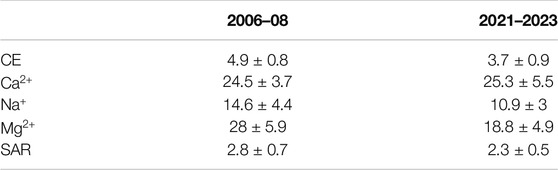
Table 3. Comparison between drainage water quality in the sub-basin AB2 for the years of the study of Villar et al. (2015) and the present study. Values of electrical conductivity (EC) in dS.
Drainage Volumes and Salt Balances
Figure 6 illustrates the daily dynamics of irrigation water inputs, drainage water amounts, and the estimated salt export from the irrigable area in the two studied sub-basins (AB1 and AB2). In 2021 and 2022, the impact of irrigation on the quantity of drainage water and the salts exported is clearly evident. As expected for the type of crops in the area, the majority of the irrigation water was used during the summer months, with average values ranging from 50 to 80
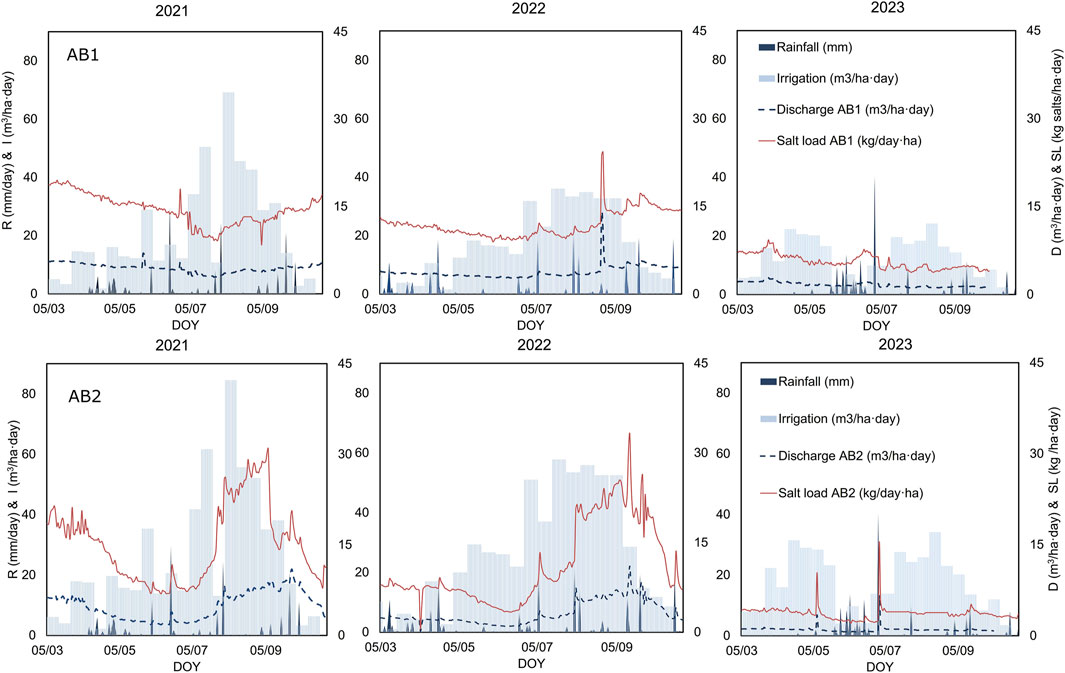
Figure 6. Daily dynamics of irrigation (I), rainfall (R) drainage discharge (D) and salt loads (SL) for the two outlets studied (AB1, AB2) during the three study periods.
A delay was observed between irrigation application and drainage discharge. Despite the highest irrigation water usage occurring in July and August, peak drainage and salt discharge were recorded in September. This suggests that at the onset of the “second” irrigation period for maize, the soil was initially dry, resulting in minimal drainage. Over time, as the soil moisture increased, excessive drainage occurred from July to September, aligning with the period of peak irrigation demand. This indicates that irrigation management may have been less effective during periods of high irrigation demand compared to periods of lower demand.
Regarding the response to precipitation events, it was observed (dark blue bars in Figure 6) that the influence in AB1 was much smaller than in AB2, with the former having a significantly larger drainage basin (2587 ha vs. 114 ha). In AB2, there was a clear increase in the salt load in the days leading up to a rainfall event. In AB2, salt load values ranged from 20 to 30 kg salt·
The main differences between these studies were related to the geological and soil types, and also to the irrigation types (surface or sprinkler). This complicates the comparison of results across basins beyond salt exports and highlights the importance of studying each irrigated area individually. It also underscores the potential for improvements in water management and their overall impact.
In 2023, there was a noticeable decrease in irrigation water application compared to 2021: by 40% in AB1 and by 32% in AB2. The reduction in irrigation water application was mandated by the basin administrator (Confederación Hidrográfica del Ebro, CHE) in response to the hydrological drought experienced in the basin in 2023 Confederación Hidrográfica del Ebro (2024). Water availability for irrigation across the entire irrigable area was reduced to 75% compared to a normal year. However, through optimized water management at the irrigation district level, the actual irrigation water applied amounted to 70% of the amount applied in the previous 2 years (personal communication, 2023).
We observed the effects of reduced irrigation water availability in both sub-basins. As shown in Figure 6, peak irrigation application occurred during the summer months in 2021 and 2022 but this level was not reached in 2023. In that year, maximum average daily irrigation inputs did not exceed 40
Moreover, during the first irrigation period application in 2023 (spring months), which corresponded to the winter cereal cycle, we observed a lower drainage discharge despite a higher water input through irrigation compared to 2021 and 2022, as seen in Figure 6. This suggests that when restrictions were imposed in the irrigation district, farmers improved their water management, possibly due to greater concerns about water usage.
An increase in irrigation during the spring months did not result in any corresponding increase in drainage during these months. Furthermore, despite a greater irrigation input, we observed a reduction in the leaching fraction in AB2 in 2022. This decrease in drainage was attributed to higher reference evapotranspiration (
At the same time, the reduction observed in 2023 resulted in a lower LF, with the value approaching the optimal level (LR), with this being even lower in the case of AB2 (Figure 6). The LF decreased from 15.6% in AB1 and 14.8% in AB2, to 7.5% and 3.9%. These results suggest that most of the excess drainage occurred during the summer months, coinciding with peak irrigation activity.
In has been observed that the salt balances varied across the 3 years analyzed (Table 4), with the amount of irrigation water applied being the determining factor. During the periods of unrestricted irrigation in 2021 and 2022, the salt balance shows that salt outputs through drainage were consistently high, ranging between 996 kg·

Table 4. Irrigation (I,
It is important to note that the drainage measures discussed in this research are those registered exclusively during the irrigation periods of the three study years. Consequently, references to salt exports pertain specifically to those occurring within this time-frame. Although there is reduction in drainage water flow during the winter period, salt exports still occurred during this season; however, these are not reflected in this study.
Ion Balance Assessment
Individual assessments of the predominant ions were conducted for each irrigation period within the two sub-basins studied. Figure 7 provides a synthesis of the acquired data. The figure indicates that salt is exported in excess in most cases, except for sub-basin AB2 in 2023, where salt is retained in the soil. Sulfate emerged as the primary ion exported in substantial quantities, averaging −1,200 kg.
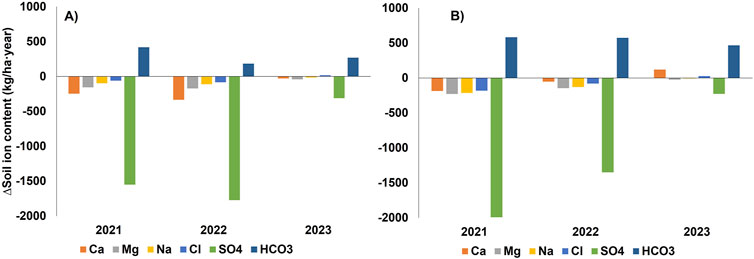
Figure 7. Soil ion increase/decrease for each irrigation season in the two sub-basins studied, AB1 subfigure (A) and AB2 subfigure (B). More detailed data are available in the Supplementary Material.
Considering Equation 5, and based on an average annual increase of 416 kg·
As noted by Entry et al. (2004), irrigation can serve as a tool to augment the sequestration of carbon within the soil, working in conjunction with organic C sequestration. Furthermore, this mechanism provides a valuable means of enhancing carbon sequestration in semi-arid regions, where soil organic matter (SOM) is scarce and challenging to increase. This is particularly important in arid and semi-arid regions with low soil organic matter (SOM) levels.
Further research could focus on accounting the total amount of C sequestrated through irrigation in a certain irrigated area.
Future Recommendations
In view of the results obtained in the present study, some remedial measures can be carried out in the study area, which are described below.
From one side, an improvement in irrigation management is necessary to reduce the existing leaching fraction and reduce the downstream impact. As shown in Table 4, in years with normal irrigation (i.e. 2021-2022), leaching fractions between 9.8% and 17% were obtained during the irrigation period. This indicates that LF during the whole year exceeds these values, being five times higher than those necessary to maintain soil quality. To understand the excess LF in the study area, it is necessary to know the existing irrigation dynamics.
As indicated in Table 1, double crop (barley + maize) is the main land use in all the studied sub-basins, followed with alfalfa and maize (as in the whole studied irrigation district as well as in others irrigation districts in the Ebro basin).
Those crops are usually classified as summer crops, as its growing cycle occurs mainly during summer months. This fact, together with the reduced rainfall during this season, makes irrigation indispensable to obtain profitable productions.
At the same time, Salvador et al. (2011) observed that summer crops in the Ebro basin are usually over-irrigated. In sprinkler irrigation, they reported 20% and 25% higher irrigation water use with respect to the net irrigation requirement values in alfalfa and maize, respectively. This indicates an irrigation water use that may be leached on excess.
This results are similar to ones obtained in a previous study developed in the sub-basin AB5. Altés et al. (2023) found that maize and double crop fields were being over-irrigated between a 9%–12% with respect to the gross irrigation requirements, according to Allen et al. (1998).
One of the strategies to reduce LF is to adjust the irrigation depths to the irrigation requirements of the crops. As can be seen in Figure 6, September accounts for the highest drainage volume discharge. It is in this late period of the crop cycle when irrigation requirements begin to decrease. However, according to Altés et al. (2024), irrigation depths in the area are not reduced correspondingly, being responsible for this excess leaching.
On the other hand, water quality parameters indicate that three sub-basins drainage water can be easily reused (AB1, AB2, AB5). According to Ayers and Wescot (1985), these are medium-low quality water in therms of EC, but with low sodium content. This, combined with the use of good quality water (as is currently being used), can be a tool to reduce the total use of irrigation water diverted upstream, and in turn reduce the impact of the irrigable area downstream, without a reduction in the soil quality of the irrigable area. At the same time, it increases water resources downstream available for other users.
Conclusion
This study focused on monitoring drainage water quality in various sub-basins within a modern irrigation district located in the Ebro basin, northeastern Spain, over three consecutive irrigation periods. Our findings demonstrate distinct variations in drainage water quality among sites irrigated with identical water sources. This underscores the need to discern soil types at each site prior to evaluating potential water reuse and/or its leaching requirements. While drainage water in three of the sub-basins which were studied presented suitability for irrigation reuse without any significant issues, one site exhibited high levels of electrical conductivity and sodium adsorption ratios, pointing to its unsuitability. Analyses of salt balances over the study period also revealed a consistent trend of greater salt outputs than inputs, with drought years changing this dynamic due to insufficient water inputs for maintaining the minimum levels required for leaching. It is significant that in 2023, which was a year characterised by irrigation restrictions, salt accumulations occurred in the soil. In the case of ion concentrations, high sulfate levels in drainage water were primarily attributed to the abundance of gypsum in the soils in the study area. This altered the relationship between electrical conductivity and total dissolved ions. This study also suggest the significance of accounting for inorganic carbon accumulation in calcareous soils. This could be attributable to the precipitation of calcium carbonate resulting from irrigation involving bicarbonate-type water. Reducing irrigation doses at the end of the vegetative cycle might lower the leaching fraction reported in this study. Moreover, the reuse of the drainage water may promote sustainable water use in the region.
Data Availability Statement
The raw data supporting the conclusions of this article will be made available by the authors, without undue reservation.
Author Contributions
VA: conceptualisation, data curation, formal analysis, methodology, visualisation, writing–original draft. MP: formal analysis, supervision, writing–review and editing. JMV: funding acquisition, resources, supervision, methodology, writing–review and editing. All authors contributed to the article and approved the submitted version.
Funding
The author(s) declare that financial support was received for the research, authorship, and/or publication of this article. This research was undertaken under project PCI2020–112030, which was funded by the Agencia Estatal de Investigación, of the Ministerio de Ciencia e Innovación: MCIN/AEI/10.13039/501100011033 and by the European Union NextGenerationEU/PRTR and supported by IDEWA project ANR-19-P026–003.
Conflict of Interest
Author VA was employed by isardSAT SL.
The remaining authors declare that the research was conducted in the absence of any commercial or financial relationships that could be construed as a potential conflict of interest.
Acknowledgments
We are grateful to the Algerri- Balaguer irrigation district (Comunitat de Regants del Canal Algerri- Balaguer) for their collaboration with this research.
Supplementary Material
The Supplementary Material for this article can be found online at: https://www.frontierspartnerships.org/articles/10.3389/sjss.2024.13522/full#supplementary-material
References
Abrahao, R., Causapé, J., García-Garizábal, I., and Merchán, D. (2011). Implementing Irrigation: Salt and Nitrate Exported From the Lerma Basin (Spain). Agric. Water Manag. 102, 105–112. doi:10.1016/j.agwat.2011.10.011
Agència Catalana de l’Aigua (2013). Cartografia d’aqüífers de Catalunya. Barcelona: Tech. rep., Agència Catalana de l’Aigua.
Allen, R. G., Pereira, L. S., Raes, D., and Smith, M. (1998). Crop Evapotranspiration. Guidelines for Computing Crop Water Requirements. FAO Irrigation and Drainage Paper 56. Rome: FAO.
Altés, V., Bellvert, J., Pascual, M., and Villar, J. M. (2023). Understanding Drainage Dynamics and Irrigation Management in a Semi-arid Mediterranean Basin. WaterSwitzerl. 15, 16–23. doi:10.3390/w15010016
Altés, V., Pascual, M., Escorihuela, M. J., and Villar, J. M. (2024). Assessing Irrigation Impact on Water Quality Conditions: A Case Study in the River Noguera Ribagorçana (NE Spain). Agric. Water Manag. 296, 108809. doi:10.1016/j.agwat.2024.108809
Andrés, R., and Cuchí, J. A. (2014). Salt and Nitrate Exports from the Sprinkler-Irrigated Malfarás Creek Watershed (Ebro River Valley, Spain) During 2010. Environ. Earth Sci. 72, 2667–2682. doi:10.1007/s12665-014-3174-0
Andrés, R., Martín-Ramos, P., and Cuchí, J. A. (2020). Water Balance and Nitrate and Salt Exports from a Saline–Sodic Irrigation District in Castelflorite (Huesca, NE Spain). Agronomy 10, 165 [pp. 16]. doi:10.3390/agronomy10020165
Ayers, R., and Wescot, D. (1985). Water Quality for Agriculture, 1. Rome: FAO Irrigation and Drainage Paper 29 Rev.
Barros, R., Isidoro, D., and Aragüés, R. (2015). Three Study Decades on Irrigation Performance and Salt Concentrations and Loads in the Irrigation Return Flows of La Violada Irrigation District (Spain). Agric. Ecosyst. Environ. 151, 44–52. doi:10.1016/j.agee.2012.02.003
Boixadera, J., Danés, R., and Porta, J. (1989). “Sistema d’informació de sòls de Catalunya (CatSIS),” in Comunicacions de la XVI Reunion de la Sociedad Española de la Cienca del Suelo (Lleida).
Causapé, J., Quílez, D., and Aragüés, R. (2004a). Assessment of Irrigation and Environmental Quality at the Hydrological Basin Level: I. Irrigation Quality. Agric. Water Manag. 70, 195–209. doi:10.1016/J.AGWAT.2004.06.005
Causapé, J., Quílez, D., and Aragüés, R. (2004b). Assessment of Irrigation and Environmental Quality at the Hydrological Basin Level: II. Salt and Nitrate Loads in Irrigation Return Flows. Agric. Water Manag. 70, 211–228. doi:10.1016/j.agwat.2004.06.006
Confederación Hidrográfica del Ebro, M. P. L. T. E. (2024). Informe de la Sequía 2023 (Año Hidrológico 2022-2023). Tech. Rep.
Cox, C., Jin, L., Ganjegunte, G., Borrok, D., Lougheed, V., and Ma, L. (2018). Soil Quality Changes Due to Flood Irrigation in Agricultural Fields Along the Rio Grande in Western Texas. Appl. Geochem. 90, 87–100. doi:10.1016/j.apgeochem.2017.12.019
Doorenbos, J., and Pruitt, W. (1977). Guidelines for Predicting Crop Water Requirements. Rome: FAO Irrigation and Drainage. Paper 24.
Entry, J. A., Sojka, R. E., and Shewmaker, G. E. (2004). Irrigation Increases Inorganic Carbon in Agricultural Soils. Environ. Manag. 33. doi:10.1007/s00267-003-9140-3
Fereres, E., Orgaz, F., and Gonzalez-Dugo, V. (2011). Reflections on Food Security under Water Scarcity. J. Exp. Bot. 62, 4079–4086. doi:10.1093/jxb/err165
García-Garizábal, I., Abrahao, R., and Causapé, J. (2012). El Manejo del Riego y la Contaminación por Sales y Nitrato: Un Caso De Inundación Vs. Aspersión. Inf. TÉCNICA ECONÓMICA Agrar. 108, 482–500.
García-Garizábal, I., Valenzuela, J. C., and Abrahão, R. (2009). Evolution of the Efficiency and Agro-Environmental Impact of a Traditional Irrigation Land in the Middle Ebro Valley (2001–2007). Span. J. ofAgricultural Res. 7, 465–473. doi:10.5424/sjar/2009072-1499
Gil, C., Santos, J., and Esnaola, J. (1998). Mapa Geológico y Memoria de la Hoja no 359 (Balaguer). Mapa Geológico de España E. 1:50.000. Madrid: Tech. rep., Instituto Tecnológico Geominero de España.
Hart, B. (1974). A Compilation of Australian Water Quality Criteria. (Australian Water Resources Council Techn Paper Nr 7). Canberra: Tech. rep., Australian Government Publishing Service.
Hillel, D., Braimoh, A. K., and Vlek, P. L. (2008). “Soil Degradation under Irrigation,” in Land Use and Soil Resources. Editors V. P. Braimoh, and A. K. Dordrecht doi:10.1007/978-1-4020-6778-5{_}6
Instituto Geológico y Minero de España and Dirección General del Agua (2010). Identificación y caracterización de la interrelación que se presenta entre aguas subterráneas, cursos fluviales, descargas por manantiales, zonas húmedas y otros ecosistemas naturales de especial interés hídrico. 091.061 Aluvial del Bajo Segre. Madrid: Tech. rep., Instituto Geológico y Minero de España, Ministerio de Ciencia e Innovación, Gobierno de España.
Isidoro, D., Quílez, D., and Aragüés, R. (2006). Environmental Impact of Irrigation in La Violada District (Spain). J. Environ. Qual. 35, 766–775. doi:10.2134/jeq2005.0064
Jiménez-Aguirre, M. T., and Isidoro, D. (2018). Hydrosaline Balance in and Nitrogen Loads from an Irrigation District before and after Modernization. Agric. Water Manag. 208, 163–175. doi:10.1016/j.agwat.2018.06.008
McNeil, V. H., and Cox, M. E. (2000). Relationship between Conductivity and Analysed Composition in a Large Set of Natural Surface-Water Samples, Queensland, Australia. Environ. Geol. 39, 1325–1333. doi:10.1007/s002549900033
Mensua, S., Fernández, S., Ibáñez, M., Marcellán, M., Yetano, M., and Ruiz, M. (1977). Sector Central de la Depresión del Ebro, Mapa de terrazas fluviales y glacis. 1st ed. Zaragoza: Universidad de Zaragoza, Departamento de Geografía.
Merchán, D., Casalí, J., Del Valle de Lersundi, J., Campo-Bescós, M. A., Giménez, R., Preciado, B., et al. (2018). Runoff, Nutrients, Sediment and Salt Yields in an Irrigated Watershed in Southern Navarre (Spain). Agric. Water Manag. 195, 120–132. doi:10.1016/j.agwat.2017.10.004
Merchán, D., Causapé, J., and Abrahão, R. (2013). Impact of Irrigation Implementation on Hydrology and Water Quality in a Small Agricultural Basin in Spain. Hydrological Sci. J. – J. des Sci. Hydrologiques 58, 1400–1413. doi:10.1080/02626667.2013.829576
Ministerio para la Transición Ecológica y el Reto Demográfico (2022). Caracterización adicional de las masas de agua subterránea MSBT:ES091MSBT061 - Aluvial del Bajo Segre. Tech. rep., Ministerio para la Transición Ecológica y el Reto Demográfico.
Murray, R., and Grant, C. (2007). Impact Of Irrigation On Soil Structure. Tech. Rep. Canberra: Australian Government, Land and Water Australia.
Olivera-Guerra, L.-E. E., Laluet, P., Altés, V., Ollivier, C., Pageot, Y., Paolini, G., et al. (2023). Modeling Actual Water Use under Different Irrigation Regimes at District Scale: Application to the FAO-56 Dual Crop Coefficient Method. Agric. Water Manag. 278, 108119. doi:10.1016/j.agwat.2022.108119
Piper, A. (1944). A Graphic Procedure in the Geochemical Interpretation of Water-Analyses. os, Trans. Am. Geophys. Union 25, 914–928. doi:10.1029/TR025i006p00914
Quílez, D. (1985). Descripción, análisis y aplicación de un modelo hidrosalino del sistema flujos de retorno de riego. Bachelor thesis. Tech. rep. Zaragoza: Universidad de Zaragoza.
Rhoades, J. (1974). “Drainage for Salinity Control,” in Drainage for Agriculture, 433–468. J. van Schilfgaarde (Agronomy Monographs). doi:10.2134/agronmonogr17.c21
Rhoades, J., Chanduvi, F., and Lesch, S. (1999). Soil Salinity Assessment. Methods and Interpretation Fo Electrical Conductivity Measurements. Rome: FAO Irrigation Drainage Paper.
Rusydi, A. F. (2018). Correlation between Conductivity and Total Dissolved Solid in Various Type of Water: A Review. IOP Conf. Ser. Earth Environ. Sci. 118, 012019. doi:10.1088/1755-1315/118/1/012019
Salvador, R., Martínez-Cob, A., Cavero, J., and Playán, E. (2011). Seasonal On-Farm Irrigation Performance in the Ebro Basin (Spain): Crops and Irrigation Systems. Agric. Water Manag. 98, 577–587. doi:10.1016/J.AGWAT.2010.10.003
Soil Survey Staff (2022). Keys To Soil Taxonomy (USDA Natural Resources Conservation Service). 13th ed.
Stosch, H.-G. (2022). Excel Template to Plot Hydrochemical Data into a Piper Diagram (1.0). doi:10.5281/zenodo.5994293
Tedeschi, A., Beltrán, A., and Aragüés, R. (2001). Irrigation Management and Hydrosalinity Balance in a Semi-arid Area of the Middle Ebro River Basin (Spain). Agric. Water Manag. 49, 31–50. doi:10.1016/S0378-3774(00)00117-7
Villar, J., Pascual, M., Rufat, J., and Villar, P. (2015). El impacto del riego en la calidad del agua de drenaje en una nueva zona regable. Ing. del agua 19, 241–253. doi:10.4995/ia.2015.4113
Keywords: return flows, water quality, drought, calcareous soils, environmental impact
Citation: Altés V, Pascual M and Villar JM (2024) Impact of Irrigation Management on Salinity and Volume of Drainage Water in an 8000 ha Irrigation District in the Ebro Basin (NE Spain) . Span. J. Soil Sci. 14:13522. doi: 10.3389/sjss.2024.13522
Received: 12 July 2024; Accepted: 05 November 2024;
Published: 14 November 2024.
Edited by:
Avelino Núñez-Delgado, University of Santiago de Compostela, SpainCopyright © 2024 Altés, Pascual and Villar. This is an open-access article distributed under the terms of the Creative Commons Attribution License (CC BY). The use, distribution or reproduction in other forums is permitted, provided the original author(s) and the copyright owner(s) are credited and that the original publication in this journal is cited, in accordance with accepted academic practice. No use, distribution or reproduction is permitted which does not comply with these terms.
*Correspondence: Víctor Altés, dmljdG9yLmFsdGVzQHVkbC5jYXQ=