- Escuela Politécnica Superior de Huesca, Instituto de Investigación en Ciencias Ambientales (IUCA), GEOFOREST Research Group, Huesca, Spain
Prescribed burns and selective shrub clearing are widely implemented as management strategies to stop the shrub encroachment of grasslands, decrease fuel loads and fire risks, and improve biodiversity and ecosystem functionality in mountain environments. While the short-term effects of burns on soil have been extensively studied, the impact of mechanical treatments on soil has received comparatively less attention. This study aims to: i) evaluate the physical, chemical, and biological characteristics of subalpine soils influenced by prescribed burns and selective clearing, and ii) assess the effectiveness of these interventions by examining the changes in vegetation cover 2 years after implementation. The research was conducted in the Central Pyrenees, where three plots were selected according to their management type: a prescribed burn plot (B), a clearing plot (CL), and a shrubland control plot (C). The results highlight how both treatments increased soil pH and reduced other properties (EC, BD, moisture, GLU) after 2 years of study, with burned and cleared plots showing similar trends in all cases. The carbon source utilization patterns of soil microbial communities (CLPP) remained unchanged by either treatment, which may indicate the short-term resilience of microbial communities. However, differences in soil microbial activity, as measured by basal soil respiration (bSR), were observed. An increase in bSR was found with shrub removal via mechanical clearing, as evidenced by the constants of the single-compartment model and the average residence time (ART) of organic matter. These changes were primarily driven by the indirect effects of vegetation cover alteration. Shrub cover remained low 2 years after the application of both methods, although prescribed burning resulted in more bare soil and lower plant diversity compared to the cleared plot.
Introduction
Grassland communities in mountain areas, one of the most important ecosystems for plant diversity, are being replaced by expanding shrubland due to land-use changes driven by a decline in grazing pressure, resulting from rural depopulation and land abandonment (Schirpke et al., 2017; Tokarczyk, 2017; Lasanta, 2019). This issue is part of Global Change, alongside vegetation cover transformations and climate changes linked to global warming. These processes negatively impact natural mountain ecosystems, leading to irreversible changes in ecosystem functionality and services, alterations in landscape primary productivity, reductions in biodiversity, and changes in soil and vegetation properties (Alados et al., 2019; Vadillo et al., 2023).
Woody encroachment on former pastures is especially suitable in the Pyrenees where it is estimated that 2/3 have been affected in recent decades (Gelabert et al., 2021). Among these bushes stands Echinospartum horridum (Vahl) Rothm, a spiny, cushion-shaped species, endemic to the Pyrenees. This species is strictly calcicolous, well-adapted to dry air, intense sunlight, stony and shallow soils, and is highly resistant to grazing (Montserrat et al., 1984). Its expansion is prominent in the Pre-Pyrenean mountains and the central Pyrenees of Aragón, preferably on south facing slopes, where it forms large, dense, monotypic stands that can cover several hectares (Mora et al., 2022; Komac et al., 2011). Moreover, there is higher seedling survival of this species after burns. Fires kill the plant but trigger the simultaneous germination of seeds (Montserrat et al., 1984). Alados et al. (2019) compared clearing vs burning treatments for restoring subalpine grasslands after shrub encroachment in Tella (Pyrenees); they found, 5 years after treatment, as burn had higher E. horridum cover and abundance (more than double) than clear cut treatment.
Historically, fire has been used as a management tool to control shrub expansion and promote grassland regeneration. However, selective shrub clearing is being used as an alternative measure that not only reduces fuel build-up and the risk of summer wildfires but also helps conserve the diversity and functionality of mountain ecosystems (Alados et al., 2019; Fontúrbel et al., 2016; Castillo-Garcia et al., 2024; Pereira et al., 2023). Many studies also propose integrating these management strategies with pyric herbivory, a traditional practice which combines fire and guided grazing. This approach is considered a potentially more effective strategy for mitigating grassland shrub encroachment and promoting plant species diversity (Alados et al., 2019; Cortijos-López et al., 2023). It is also important to include rest periods between herbivory treatments, as grazing animals may consume the regrowth of desired species, delaying recovery. However, other studies have shown that continuing with current control and grazing practices will not achieve pastures typical of the Central Pyrenees due to the reduced livestock density within these ecosystems. Therefore, it is still necessary to implement additional strategies to prevent shrub encroachment in pastures (Cottani and Sabattini, 2006; San Emeterio et al., 2016; Amsten et al., 2024; Clarke et al., 2013; Mora et al., 2022).
It has been demonstrated that mechanical clearing practices promote an increase of soil organic carbon storage, acting as carbon sinks as shrub clearing process increases the soil organic carbon (SOC) about 40% compared to shrubland cover (Cortijos-López et al., 2023; Nadal-Romero et al., 2018; 2021; Berninger et al., 2015). During the initial stage of woody invasion in the grasslands of the subalpine zone of the Central Spanish Pyrenees, the SOC stock in the mineral fraction decreases by 38%, from 147.9 Mg C/ha to 91.7 Mg C/ha, due to reduced carbon inputs from herbaceous vegetation and alterations in the decomposition and stabilization processes of soil carbon. Over time, as woody vegetation becomes established and matures, SOC stocks may recover or even increase due to new organic matter contributions from shrub and tree vegetation (Nadal-Romero et al., 2018). Furthermore, it has been shown that clear cutting can modify soil microbial communities in the long term (Hynes and Germida, 2013). Initially, microbial communities may thrive due to the decomposition of roots, but over time, the availability of these nutrients declines, leading to a reduction in microbial biomass growth rates. Additionally, mechanical clearing has been shown to increase soil temperature (Hashimoto and Suzuki, 2004), with associated modifications on the biological community and soil properties.
The effects of burning practices on soil are highly variable and depend on numerous factors, including climate, type and amount of fuel, fire intensity, duration, size of the burned area, time since the last fire, fire history, soil type, moisture content, and slope (Bodí et al., 2014). Among these, fire duration is one of the most impactful characteristics: longer fires result in greater soil impacts (Alfaro-Leranoz et al., 2024). Even low-temperature fires can reduce microbial biomass and soil respiration (Francos et al., 2018), affecting the diversity and structure of microbial communities. This is particularly important because soil bacterial communities play a critical role in edaphic structure and ecosystem recovery following disturbances. Microorganisms contribute significantly to ecosystem regeneration and recovery (Moya et al., 2022; Fontúrbel et al., 2024). However, in general, bacteria tend to be more resilient to post-fire edaphic conditions than fungi (De la Rosa et al., 2014). During the first month after a fire, fungal populations decrease significantly and take a long time to recover to pre-fire levels, largely due to the loss of soil organic matter caused by the fire. The destruction of soil mycological flora has severe consequences for vegetation, as the lack of mycorrhizae reduces plants' ability to absorb water and nutrients (Martínez et al., 2006; Fernández de Ana-Magán, 2000). In the Central Pyrenees, numerous studies have already been conducted on the impact of prescribed burns on various soil properties (Armas-Herrera et al., 2016; Girona-García, et al., 2018; Alfaro-Leranoz, et al., 2023). Among the findings, a decrease in β-D-glucosidase (GLU) activity was observed immediately after the burn, and this activity does not recover over time. In contrast, other properties did not show an immediate reduction but experienced declines over time, such as total soil organic carbon (SOC), labile carbon (DOC), total nitrogen (TN), and basal soil respiration (bSR).
These two management practices produce both direct and indirect effects on the soil, highlighting the importance of distinguishing between them. In the case of burns, direct effects are a result of the temperatures reached during the fire and the combustion process of surface biomass and organic matter. Indirect effects are associated with the post-treatment period following both fire and mechanical clearing. During this time, changes in ecosystem components, such as the reduction of vegetation and litter cover, the presence of ash and burned plant material (in the case of burns), or shredded plant material (in the case of clearing), influence soil microbial populations and, consequently, edaphic characteristics and vegetation recovery (Santín and Doerr, 2016; Zavala et al., 2014; Bodí et al., 2014).
While numerous studies have focused on the single effects of prescribed fire on soil, particularly in the immediate aftermath (Fontúrbel et al., 2024; Girona-García et al., 2019; Agbeshie et al., 2022; González-Pérez et al., 2004) and clearing effects on soil and vegetation response (Pietikäinen and Fritze, 1995; Alados et al., 2019), there is limited information comparing the effects of mechanical treatments and prescribed burns, especially about soil properties. The aim of this study is to evaluate the effects of key management practices on mountain pasture ecosystems 2 years after their implementation. Specifically, the objectives are: i) to characterize the physical, chemical, and biological properties of soils in mountain pastures subjected to different management treatments, and ii) to assess the effectiveness of these treatments by analyzing changes in vegetation cover over time in the experimental plots.
Two years after the application of both treatments, ashes had either been leached or incorporated into the soil, similar to the behavior of shredded plant material. However, large-sized burned vegetation, such as mature E. horridum remains in the ecosystem due to its robust shrub structure with thick trunks and branches. Consequently, assessing the effects of fire and mechanical clearing primarily relies on indirect measurements of changes in soil properties and vegetation cover.
This study hypothesizes that no significant differences will be observed in the physical, chemical, and biological properties of the soils 2 years after the implementation of the two E. horridum management treatments (prescribed burning and mechanical clearing) as previous studies on prescribed burns have shown that the effects of fire on soil are typically confined to the top centimeter of the soil profile. Additionally, it is expected that the regeneration of E. horridum will be differently affected by the management treatments, with lower cover observed in the prescribed burn treatment compared to other treatments, because of fire on vegetation.
Materials and Methods
Experimental Design and Treatments
The study area is in a subalpine mountain passes in Asín de Broto (Sobrarbe, Huesca, Spain) (Figure 1). The climate is characterized by a mean annual temperature of 8.8°C and an average annual precipitation of 1,120 mm/year (Girona-García et al., 2019). The slope faces west, with an inclination between 10% and 15%. The most common soils in this area correspond to Eutric Cambisols and Calcaric Cambisols (IUSS Working Group WRB, 2022), developed over Eocene turbidites (IGME, 1972). Non-shrub grasslands in this area are classified as priority habitat 6,210, “Semi-natural dry grasslands and scrubland facies on calcareous substrates (Festuco-Brometalia)” (Girona-García et al., 2019).
Three plots were selected in this area (Figure 2) at elevations ranging from 1,606 to 1,644 m a.s.l., according to their management practices: one subjected to a prescribed burn in 2021 (B) covering 0.38 ha, another subjected to mechanical clearing in the same year (CL) covering 1.28 ha, and a control plot (C) of 0.77 ha situated between the other two, where no interventions were conducted and shrub dominance persists.
Prior to the interventions, both managed plots were dominated by E. horridum. Prescribed burning was performed when the environmental conditions met the prescribed parameters set by firefighters of the EPRIF (Wildfire Prevention Team) of Huesca and BRIF (Reinforcement Brigades against Wildfires) of Daroca units. Similar burns (Girona-García et al., 2018; Alfaro-Leranoz et al., 2023) result in temperatures of 812°C at the soil surface, which decrease at ambient temperature below 2 cm depth. Despite this, the litter layer was not completely consumed by the fire. Following the treatments, the soil was covered with biomass remains from burning and clearing, with the cleared residue reaching a thickness of 4 cm.
The study area was also subject to continuous, free grazing across approximately 100 ha, including the three study plots. The grazing regime is as follows: cattle grazing occurred from 20 April to 2 July, with a load of 100 cows, and from 1 November to 1 January, with a reduced load of 40 cows. Sheep grazing was more extensive, with 1,500 sheep present from April to 21 July, followed by 500 sheep grazing from July to September. From September to November, sheep numbers started at 1,300 and decreased gradually, with 15 fewer sheep per day until the end of the period. Additionally, 20 goats grazed year-round, except from October to December, while a single donkey grazed concurrently with the sheep.
Vegetation Monitoring and Soil Sampling
To assess the effectiveness of the management treatments in promoting vegetation recovery and to evaluate vegetation structure and species composition, a total of four 15-m transects were established in each plot. Vegetation cover was measured at consistent intervals of 10 cm along the entire length of each transect, ensuring detailed and uniform data collection across all plots. Cover was established using the Line-Intercept Sampling method (Cummings and Smith, 2000; Eberhardt, 1978) and categorized into four functional groups: grasses, legumes, E. horridum (thorny cushion), other species, and bare soil, or litter. Additionally, four litter samples were collected from 30 × 30 cm quadrats along each transect in the cleared and burned plots to quantify the litter present on the surface of each plot (kg/ha).
To perform soil sampling, in each plot, four representative and equidistant 30 × 30 cm surfaces were established along the four transects used for vegetation analysis. At each point, five surface soil samples (0–3 cm) were collected and combined to form a composite sample per point. Subsequently, the composite samples from the four points within each transect were further mixed, resulting in a four composite soil sample representative of each treatment (Figure 3). A similar sampling was repeated in unburned plots, collecting the soil samples next to shrubs. This sampling approach was based on prior observations indicating that the immediate effects of wildfires on soil properties are generally confined to the top few centimeters due to the high thermal inertia of soil (Alfaro-Leranoz et al., 2024; Pereira et al., 2023). All samples were collected in plastic bags to avoid desiccation and were rapidly transported and stored at 4°C to maintain the fresh conditions. In addition, two undisturbed surface cores were collected for each transect for bulk density determination. In Figure 3, the samples are represented within a square geometry reflecting the method used during collection. Litter was first removed from a 30 × 30 cm surface area and soil was subsequently extracted using soil coring cylinders.
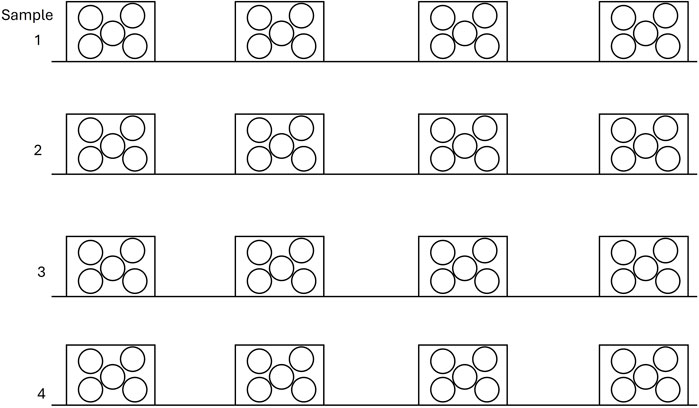
Figure 3. Soil sampling scheme: Four transects were carried out in each area subjected to the different treatments studied: C, B, CL, from which four composite samples were collected. Each composite sample consisted of five surface soil measurements taken at a depth of 0–3 cm.
Determination of Soil Properties
A fresh soil subsample was stored at 4°C for biological analyses. The rest of the soil samples were air-dried to constant weight and sieved to 2 mm for physical and chemical analysis. Additionally, a portion of the air-dried sample was ground into a fine powder.
Field capacity (FC) was determined using the method of Klute. (1986), permanent wilting point (PWP) with a pressure membrane equipment, aggregate stability (SAS) according to Kemper and Koch (1966) methodology, bulk density (BD) following the cylinder method of Blake and Hartge (1986), soil moisture was calculated gravimetrically until constant weight, and the persistence of soil water repellency was calculated with water drop penetration test (WDPT) proposed by Bisdom et al. (1993) using the classes of Doerr et al. (2000) on air-dried and 2-mm sieved soil.
The current pH was determined potentiometrically in a 1:2.5 (w/v) soil water mixture, and electrical conductivity (EC) was measured in a 1:5 (w/v) soil extract following Page et al. (1982) procedures.
Oxidizable organic carbon (OOC) was measured trough chromic oxidation (Page et al., 1982), total organic matter (TOM) was obtained by dry combustion (Heiri et al., 2001), dissolved organic matter (DOM) extracted with a soil/water suspension (1:5 w/v) at 80°C and measured trough wet oxidation method (Ghani et al., 2003), And recalcitrant organic matter (ROM) was determined through the acid hydrolysis (HCl 6N) procedure (Rovira and Ramón Vallejo, 2007). SOC mineralization was measured in an incubation assay (41 days) of soil samples under optimal temperature (25°C) and moisture (75% water-holding capacity) conditions. The released CO2 was captured with soda traps (Anderson, 1983) and measured at 2-day intervals at the start of the assay. As respiration rates declined over time, the frequency of measurements was reduced accordingly. The measurement obtained was the weight of C-CO₂. The values of C-CO₂ emitted during incubation can be fitted to a simple first-order kinetic model, represented by the following formula:
Biological Characterization
The potential enzymatic activity involved in different stages of soil organic carbon cycles were determined through β-D-glucosidase analysis by using the method of Eivazi and Tabatabai (1988). According to this method, soil samples were incubated with substrate (β-D-glucopyranoside) for 1 h at 37°C in a medium buffered at pH 5. Then, the product (p-nitrophenol) was extracted by filtration after adding CaCl2 and tris (hydroxy-methyl) aminomethane buffer at a pH of 12. Finally, the concentration of p-nitrophenol was determined by colorimetry based on the yellow colour in the solution.
Carbon source utilization patterns of sol microbial communities (CLPP) were determined with Biolog EcoPlates (BIOLOG INC, Hayward, CA) as described by Liang et al. (2016). A single Biolog™ EcoPlate was used for each soil sample, resulting in three replicates per sample. Each EcoPlate was inoculated with a 130 μL aliquot of a soil dilution prepared from 1:10 (Dw/v) suspensions of fresh soil in autoclaved 0.85% sodium chloride solution, containing 3 g of glass beads to facilitate physical dispersion of the sample. The suspension was shaken on an orbital shaker at 220 rpm for 30 min. Following shaking, the soil suspension was centrifuged at 2,600 rpm for 10 min. The supernatant was carefully decanted and centrifuged again at 2,600 rpm for an additional 10 min. A 2 mL aliquot of the resulting supernatant was then pipetted and transferred into 30 mL tubes containing 18 mL of autoclaved 0.85% sodium chloride solution. This process was repeated twice to achieve a 10⁻³ dilution. The development of color in the wells indicates the potential activity of the bacterial community on a specific substrate, i.e., the intensity of microbial carbon use, which is measured by the average well color development (AWCD) (Braun et al., 2010; Fontúrbel et al., 2016; García Lucas, 2013). The diversity of the microbial community is estimated based on the carbon sources used in the EcoPlates—amines, amino acids, carbohydrates, carboxylic acids, phenols, and polymers—was determined using species richness (SR) as the number of utilized substrates, the Shannon-Wiener index (H’), Pielou’s evenness index (J’), and the average well color development (AWCD) at 96 h post incubation. Evenness represents the proportion of observed diversity in relation to the maximum expected diversity (Magurran, 2013).
Fungal activity was assessed by estimating total glomalin content (T-GRSP) and easily extractable glomalin (EE-GRSP) following the method of Wright and Upadhyaya (1996). Easily Extractable Glomalin (EEG) was used for tracing structural changes in SOM given by the vegetation cover and the occurrence of wildfires (San Emeterio et al., 2024).
Statistical Analysis
Despite being aware of the presence of pseudoreplicates — a common issue in studies examining the effects of wildfires — we acknowledge that obtaining proper replicates is challenging without introducing variability in the geomorphological and ecological characteristics of the samples, such as orientation, elevation, soil type, or moisture (Davies and Gray, 2015). To analyze the effect of management practices on soil functionality and activity, a one-way ANOVA was conducted.
Prior to the ANOVA, data normality and homoscedasticity were assessed. For non-normal data, a Box-Cox transformation was applied to meet the assumptions of the test. The significance level for the ANOVA was set at p < 0.05. In addition, a principal component analysis (PCA) was performed to identify the main associations within the measured data.
All statistical analyses were conducted using JASP version 0.19.0 for Windows (JASP Team, 2024) and PAST software (Hammer et al., 2001).
Results and Discussion
Effect on Soil Physical and Chemical Properties
A significant difference (p = 0.016) has been found in the soil pH between the control soils (pH = 5.3 ± 0.30) in relation to those subjected to clearing (CL) and burning (B), in which the pH is 6.2 in both treatments. This acidic pH indicates that cation leaching tends to drive the soil toward oligotrophic conditions, reflecting a gradual loss of basic nutrients (Table 1).
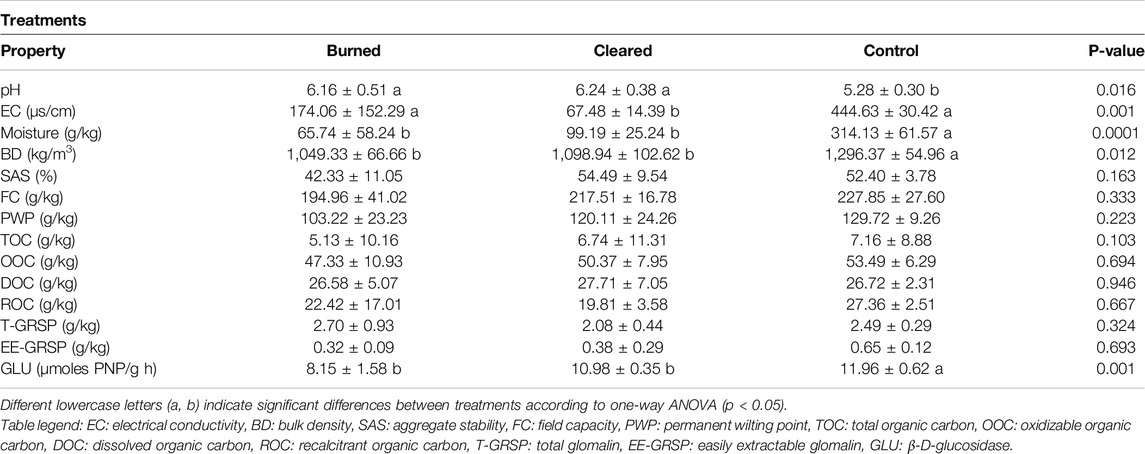
Table 1. Effects of shrub clearing and burning on soil properties for a soil depth of 0–3 cm (n = 4). Means ± standard deviations are presented for each treatment, along with significance levels (p-values).
All soil samples are hydrophilic (WDPT <5 s) with low electrical conductivity (EC values ranging from 444.63 ± 30.42 to 67.48 ± 14.39 μS/cm), showing significant differences between the control and the cleared and burned samples (p = 0.006) (Table 1). The soils exhibit moderate aggregate stability (SAS 42.33% ± 11.05% to 54.49% ± 9.54%) and contain abundant oxidizable organic carbon (OOC from 47.33 ± 10.93 to 53.49 ± 6.29 g/kg) with no significant differences observed among treatments (Table 1).
Some studies suggest that significant reductions in soil carbon may only become evident in the long-term following fire events (Girona-García et al., 2019; Girona-García et al., 2018). Additionally, any potential initial carbon loss may be offset by organic inputs from decomposing subterranean roots and the incorporation of charcoal produced during the fire. These factors act as indirect effects of fire, influencing the soil both in the short and long term. However, the impact of fire on soil organic matter remains uncertain, as it depends on the intensity and frequency of fires, the type of vegetation present and its associated fuel load, as well as the physical and chemical properties of the soil (Knicker, 2007; Hobley et al., 2017; Alcañiz et al., 2018).
Overall, the general differences between treatment groups were not pronounced. This could be attributed to the fact that the differences were subtle and did not affect the soil globally. Soil quality was primarily influenced by its long-term management history, which affected its structure, organic matter content, and biodiversity. Recent and isolated disturbances, such as fire and mechanical clearing, caused immediate changes, but their impact was often less significant than the cumulative effects of historical management practices (Blanco and Lal, 2008), suggesting that the observed differences might not be exclusively attributable to the treatments.
Effect on Soil Biological Properties
Differences in β-D-glucosidase, that represented the enzymatic activity, have been found between management treatments, specifically the burned and cleared soils present a significantly (p = 0.001) lower value than the control soil (Table 1). The activity was 8.15 ± 1.58 µmol PNP/g h for the burn treatment, 10.98 ± 0.35 µmol PNP/g h for the clearing treatment, and 11.96 ± 0.62 µmol PNP/g h for the control.
Some studies establish a direct influence of fire, as the high temperatures reached during fires can lead to the denaturation and deactivation of enzymes associated with soil colloids (Armas-Herrera et al., 2016; Lombao et al., 2021). However, 6 months post-fire, the activity of these enzymes rapidly recovers (Girona-García et al., 2019), as variations are transient. Although seasonality may affect enzyme activity, differences typically disappear within a year after treatment. Therefore, reduced activity could be attributed to the indirect effects of fire caused by changes in the soil’s physicochemical properties.
Although no significant differences were observed between the cleared and burned samples, the higher mean value observed in the cleared plot could be attributed to the transformation of surface organic residues, as these residues had nearly disappeared from the surface of the plots 2 years after treatment. Conversely, studies conducted in the central Pyrenees have not observed significant effects of fire on enzymatic activity or any modification due to the introduction of livestock (San Emeterio et al., 2016). Therefore, it is important to consider the possibility that differences observed in β-D-glucosidase activity could be influenced not only by the treatments applied but also by pre-existing natural differences between the study sites. This aspect may relate to pseudoreplication in the experimental design, as the sites selected for each treatment could have differed in their initial characteristics, such as nutrient availability or the microbiota present, potentially affecting the results independently of the treatments. Thus, while the results suggest an effect of the burning and clearing treatments, initial differences between the sites should be considered as a potential source of variability in the observed enzymatic activity.
Although fungal activity, measured through total glomalin-related soil protein (T-GRSP) and easily extractable glomalin-related soil protein (EE-GRSP), did not show significant differences in either fraction (p = 0.324 and p = 0.693, respectively), certain trends were observed in the obtained values. The average T-GRSP in the burned treatment was higher (2.70 ± 0.93 g/kg) compared to the other treatments, a common outcome following burning, though these values tend to decrease over time (Alfaro-Leranoz et al., 2022). Regarding EE-GRSP, no statistically significant differences were observed among treatments, suggesting that fungal activity was not damaged, as this fraction of glomalin represents the most recently formed portion. San Emeterio et al. (2024) considered EE-GRSP to be structurally homogeneous and likely resistant to temperatures below 200°C–250°C. Consequently, the occurrence of fires and even the type of vegetation cover can significantly influence the molecular structure of EE-GRSP. Additionally, due to mineralization, EE-GRSP eventually becomes recalcitrant, a process of significant importance since glomalin can have a soil residence time ranging from 6 to 42 years, depending on soil type and conditions (Wright and Upadhyaya, 1996; Rillig et al., 2001).
However, the lower mean value for EE-GRSP observed in the burned plot may be attributed to the impact of fire on arbuscular mycorrhizal fungi, resulting in a reduction in the number of propagules. This could potentially reduce the soil’s capacity to transform organic matter in the future (Pietikäinen and Fritze, 1995; Mataix-Solera et al., 2009). This reduction is also reflected in the inverse relationship between total glomalin and β-D-glucosidase activity, as illustrated in Figure 7. Nonetheless, it is plausible that these differences were partially influenced by the initial conditions of the sites prior to burning. Glomalin levels are linked to mycorrhizal fungal activity, which can vary naturally among sites, highlighting the possibility that the observed differences were not solely due to treatment effects but also to initial disparities associated with pseudoreplication.
The potential functional diversity of the soil microbial community was evaluated using a community-level physiological profile (CLPP) approach (García Lucas, 2013). The average color development (AWCD-DO595) values, which ranged between 0.27 ± 0.07 and 0.12 ± 0.07, (Table 2; Figure 4), did not exhibit statistically significant differences (p = 0.359). The highest average value was observed in the control sample (0.27 ± 0.07 DO595), followed by the cleared (0.19 ± 0.08 DO595) and burned (0.12 ± 0.07 DO595) samples. However, the cleared and burned samples had not yet stabilized after 96 h of incubation, suggesting that the microbial communities in the control plot had a higher and faster AWCD kinetic, while those in the burned and cleared plots were slower.

Table 2. Diversity in carbon sources consumed from Ecoplates, Species Richness, Shannon-Wiener index, Pielou’s evenness index, and Average Well Color Development (AWCD) at 96 h of incubation (n = 10). Means ± standard deviations are presented for each treatment, along with significance levels (p-values).
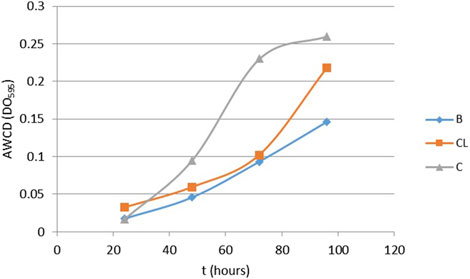
Figure 4. Average well color development (AWCD), over 96 h, using Biolog Ecoplates in burned (B), cleared (CL) and control (C) soils.
The carbon sources utilized by the microbial community showed a noticeable difference between the control sample and those subjected to clearing or burning (Figure 5). In the cleared plot, greater utilization of amino acids (0.77 ± 0.66 DO595), carbohydrates (0.619 ± 0.55 DO595), and carboxylic acids (0.57 ± 0.42 DO595) was observed. In contrast, in the burned plot, amino acids (0.60 ± 0.67 DO595) were the most utilized sources. This suggests that the microbial community in the cleared and burned plots had a higher affinity for consuming simple carbon and nitrogen sources, potentially indicating the presence of simpler organic matter forms in these soils (Koner et al., 2021). Control samples exhibited lower overall carbon source utilization but showed a preference for more complex carbon sources, such as polymers and phenolic compounds, albeit only slightly higher than in the burned and cleared samples. Amines were also utilized by the control microbial community, suggesting that the bacterial community in these plots retained the ability to degrade complex carbon sources and actively participated in the nitrogen cycle by breaking down amine-containing compounds. Bacteria capable of degrading amines can convert them into compounds such as ammonia or nitrates, potentially contributing to the mineralization of nitrogenous organic compounds and aiding in nutrient recycling.
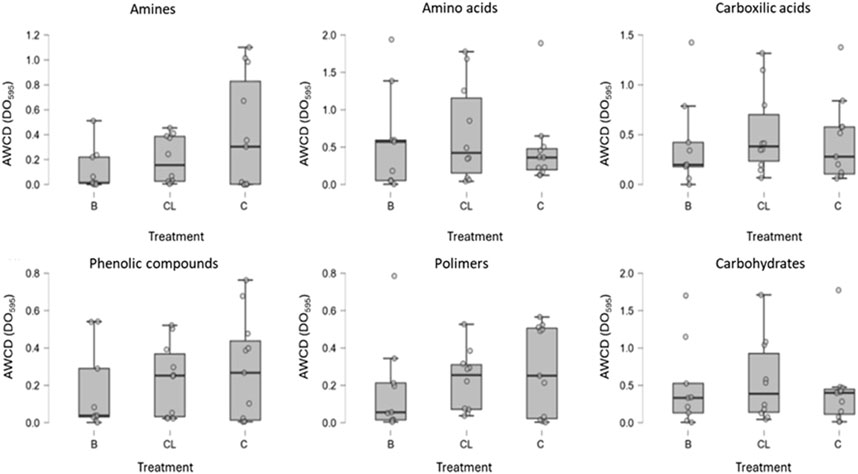
Figure 5. Catabolic activity (AWCD) of the bacterial community based on substrate families consumed and the management system (n = 9).
These findings highlight the resilience of microbial communities, recovering over the short term, reaffirming previous observations that microbial activity and carbon source utilization abilities recover approximately 6 months after fire (Lombao et al., 2020). Although no significant differences were observed between the burned and cleared samples (Table 2), the higher mean microbial activity values after clearing could be attributed to the positive effects of residual vegetation cover and the incorporation of plant residues into the soil. Additionally, microbial responses in plots subjected to prescribed burns are influenced by the fire’s severity and subsequent vegetation recovery. However, this effect may be transient, with activity levels potentially returning to their original state 4–6 years post-disturbance (Huhta, 1976). Herbivores also influence the plant-soil system through their impacts on plant composition and microbial communities. This interaction could explain why the burned and cleared plots exhibited greater carbon source utilization, as grazing occurred in these two plots (Debouk et al., 2020).
It is worth noting that the impact of each management method might be underestimated when using the CLPP technique, as microbial families exhibit functional redundancy, allowing different microbial communities to utilize the same carbon sources (Staddon et al., 1997). For this reason, it is essential to complement CLPP with an ecological diversity index analysis of carbon source utilization (Table 2). Moreover, since the carbon sources consumed depend on numerous factors, including the quantity and quality of available organic matter, it is crucial to consider that differences in carbon source utilization might result from the initial characteristics of the sites, such as the original composition of microbial communities, rather than solely from management effects.
A significant positive correlation was observed between amines and total organic carbon (r = 0.646, p = 0.021), suggesting that although total organic matter is abundant, nitrogen in readily available forms (such as ammonia or nitrates) may be limited, prompting microorganisms to utilize amines as an alternative nitrogen source. Alternatively, it could indicate that the TOM is particularly rich in nitrogen. In this case, a higher nitrogen-rich TOM could facilitate microbial access to amides as a nutrient source. Therefore, the positive relationship suggests that microorganisms are taking advantage of nitrogen-containing compounds present in the TOM, indicating a nitrogen-rich environment. This scenario is plausible in burned samples, as fires often result in increased nitrogen availability immediately following the event (Canals et al., 2024).
In the potential microbial catabolic activity richness and substrate utilization diversity (Table 2), a trend toward utilizing a greater number of carbon substrates was observed in the cleared plots compared to the others, although this difference was not statistically significant (p = 0.423). Notably, the data exhibited a lower degree of dispersion, indicating a higher level of homogeneity in substrate utilization. This suggests that bacterial communities under this condition displayed more consistent and uniform metabolic behavior. Such functional homogeneity may reflect better microbial community adaptation to environmental conditions or more efficient and uniform access to available carbon sources, underscoring the role of environmental stability or resource distribution.
Although statistically significant differences among treatments were absent, the higher mean values in cleared samples may reflect the positive impact of applying cleared material as biodegradable mulch. This practice likely enhances soil microbiota and influences soil physical and chemical properties, such as increasing water retention, maintaining appropriate soil temperatures, and improving nutrient dynamics (Aslam and Haider, 2022).
In the richness of consumed substrates, no statistically significant differences were observed. However, significantly greater heterogeneity was found in samples from plots subjected to prescribed burns, with a value of 16.83 ± 7.28. This heterogeneity could be attributed to increased nitrogen availability following fires, which can lead to short-term increases in biological productivity, as observed in the sampling conducted by Hamman et al. (2008). These findings suggest that burning impacts microbial behavior despite the treatment having occurred 2 years prior. Fire’s direct effects can persist, altering microbial biodiversity, creating or destroying niches, and affecting plant biomass and species (Mataix-Solera et al., 2009).
The same applies to diversity as represented by the Shannon index, where no significant differences (p = 0.440) were found among the treatments, with values ranging from 2.77 to 3.01. This response is consistent with other studies (Pineda and Lizarazo-Forero, 2013; Garnica and Solorio, 1995), which show that microorganisms are highly sensitive to changes in temperature, moisture, and nutrients resulting from shifts in plant cover, as often occurs after a fire. Even low-temperature fires can restrict microbial viability, and the direct and immediate effects of prescribed burns can significantly impact bacterial survival (Moya et al., 2022; Fontúrbel et al., 2024).
Mechanical clearing, while not directly altering the soil, changes environmental conditions for microbial communities, affecting their viability (Rutgers et al., 2016; Torres and Cabrera, 2005; Frazer et al., 1990). Existing differences may be more related to residues left by these treatments and changes in cover (Table 4). The presence of bare soil in burned and cleared plots can lead to greater water and wind erosion, increased evaporation and temperature, and altered nutrient cycling. These changes influence microbial communities by disrupting microbial balance and carbon source utilization during cellular growth, affecting the AWCD kinetics observed in Figure 4.
Figure 6 illustrates soil respiration as cumulative C-CO₂ emitted during the 41-day incubation assay for cleared, burned, and control soil samples. Cleared samples exhibited the highest percentage of accumulated C-CO₂ (3.56 ± 0.14 g/kg), suggesting greater microbial activity and/or higher organic matter content susceptible to decomposition compared to control (2.39 ± 0.15 g/kg) and prescribed burn (1.83 ± 0.06 g/kg) samples. Initially, all three treatments followed similar trajectories, indicating no pronounced differences in microbial activity or organic matter content. However, after the initial period, cleared samples showed a markedly higher rate of CO₂ accumulation.
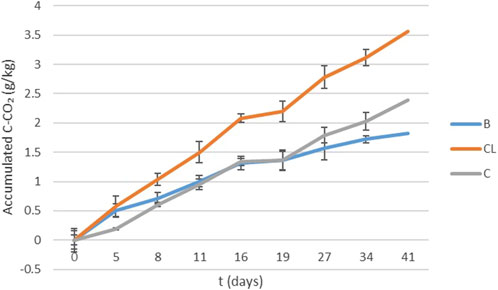
Figure 6. Soil respiration expressed as cumulative C-CO2 emitted during the incubation assays (41 days) in burned (B), cleared (CL) and control samples (C).
In burn treatments, it has been observed that the greater the fire impact, the more carbon accumulates in refractory forms, leading to a greater loss of the more labile forms of carbon. This causes the baseline respiration levels to be lower in burned soils compared to unburned ones as it was observed previously in the work of Armas-Herrera et al. (2016), who evaluated the immediate effects of prescribed burning in the Central Pyrenees on the quantity and stability of organic matter in the top few centimeters of the soil. This trend is also reflected in the values of the constant k and in the values of the mean residence time (MRT) of organic matter. As shown in Table 3, significant differences were found between treatments for the constant k (p = 0.011) and the MRT (p = 0.020).

Table 3. k constant values of the single-compartment model and average residence time (ART) of the organic matter for basal soil respiration in burned, cleared, and control samples (n = 4). Means ± standard deviations are presented for each treatment, along with significance levels (p-values).
The burned sample exhibited a higher mean residence time (MRT) of organic matter (7.25 ± 2.48 years) and a lower decomposition rate constant (k = 4.25 ± 1.9 × 10⁻⁴ days⁻1), indicating that organic matter remained in the soil for a longer period, reflecting greater recalcitrance. This suggests that organisms require more time to degrade organic matter in burned plots. A higher amount of recalcitrant organic residue (MOR) points to a shift in the quality of soil organic matter (SOM). Burning alters the composition of organic matter by increasing the proportion of recalcitrant structures, leading to a higher proportion of recalcitrant organic carbon (ROC) in the burned samples compared to the cleared plot. Microbial activity, as measured by parameters such as AWCD (Average Well Color Development), glucosidase activity, and respirometry, was lower in the burned samples. However, these differences could be related not only to the direct effect of the burning treatment but also to initial variations in the quality of organic matter or the physicochemical characteristics of the studied soils. The influence of pseudoreplication may also be a factor, limiting the ability to attribute these differences exclusively to the applied treatment. In contrast, cleared samples demonstrated higher microbial activity, as evidenced by the AWCD value, and a lower MRT of organic matter (3.44 ± 0.80 years). The control plot, with an MRT of 5.25 ± 0.45 years, showed a trend like the cleared plot.
Evolution of Vegetation Cover
Two years after the treatments, aerial biomass reached 9.88 ± 4.61 Mg/ha in the burned plot and 19.24 ± 7.22 Mg/ha in the cleared plot (p = 0.072). The presence of mulching increases soil temperature and moisture, which translates to greater microbial and enzymatic activity, as observed in Table 1. In contrast, drier sites, such as the burned plot, inhibit these positive effects. Soil enzymatic activities are indicators of soil organic matter quality, and vegetation significantly influences enzymatic activity by regulating the quality of microbial biomass (Sardans et al., 2008; Debouk et al., 2020).
The shrub removal treatment altered the composition of vegetation functional groups (Table 4). Two years after the clearing and burning treatments, E. horridum has a similar cover of 16.64% ± 4.54%, and 12.94% ± 5.39% respectively, which are significantly (p = 0.0002) lower than the control plot, with 73.69% ± 3.36%. Despite the higher percentage of E. horridum in the burned plot compared to the control plot, the results suggest that both treatments were effective in reducing shrub dominance and improving the biodiversity of the resulting pastures. The covers data obtained are very similar to those previously inventoried in other areas of the central southern Pyrenees (Mora et al., 2022).
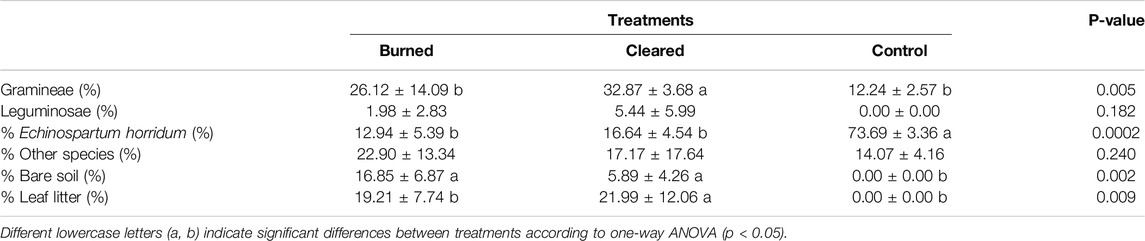
Table 4. The percentage of vegetation covers 2 years after interventions in the burned and cleared plots (n = 4). Means ± standard deviations are presented for each treatment, along with significance levels (p-values).
Although E. horridum is a legume, it was excluded from the legume functional group for quantification purposes, as the primary objective of the applied control measures was to manage its growth and establishment. Echinospartum horridum is a fire-adapted leguminous shrub that benefits from prescribed burning, as fire promotes its reproductive potential by stimulating seed bank germination. This process facilitates the establishment and recovery of the species, which can achieve full vitality and soil cover approximately 4–5 years after a fire (Mora et al., 2022; Alados et al., 2019). Since the sampling was conducted 2 years after the intervention, it is premature to assess the final state of shrub cover. However, coverage in the burned plot is expected to increase relative to the cleared plot over time. This behavior has also been observed in wetter regions of the Pyrenees, where issues of shrub encroachment are driven by Ulex gallii, another woody legume that exhibits reproductive advantages, enabling it to become invasive or dominant over other species (Múgica et al., 2024), like E. horridum.
In both treatments, grasses were the dominant functional group, with a higher coverage percentage in the cleared plot (33% ± 3.68%) compared to the burned plot (26% ± 14.09%). Significant differences (p = 0.005) were observed between the cleared plot and the control and burned plots, which did not show significant differences between each other. Although legumes were not particularly abundant in any of the plots, they were more prominent in the cleared treatment (5.44% ± 5.99%). No significant differences (p = 0.182) were observed. This functional group plays a crucial role as nitrogen fixers in the soil (Simón et al., 2005). Cleared plots exhibit a significantly higher percentage of Gramineae cover compared to both burned and control plots. These results suggest that the different treatments have distinct effects on this functional group, with clearing potentially promoting their growth more than burning or leaving the plot undisturbed. This is particularly important in mountain pastures, such as the study area, because grasses provide significant biomass, serve as a high-calorie, protein-rich food source for animals, and their extensive root systems prevent soil compaction and establish quickly, thereby reducing erosion risks (Martínez Romero and Leyva Galán, 2014).
A significant difference in bare soil was also observed (p = 0.002), with a higher percentage in the burned plot (17% ± 6.87%) compared to the cleared plot (5.89% ± 4.26%). The loss of natural cover is one of the most critical factors affecting soil fertility and natural productivity, as it leaves the soil vulnerable to various erosive agents (Muñoz-Iniestra et al., 2009).
Multivariable Analysis
The principal component analysis (PCA) of the physical, chemical, and biological properties of the analyzed soils (Figure 7) revealed that the main differences were due to fertility characteristics and biological activity. The first component (PC1) explained 52.56% of the variance, while the second component (PC2) explained 16.23%. This analysis was conducted to reduce data dimensionality and uncover underlying patterns in the variables influencing soil properties.
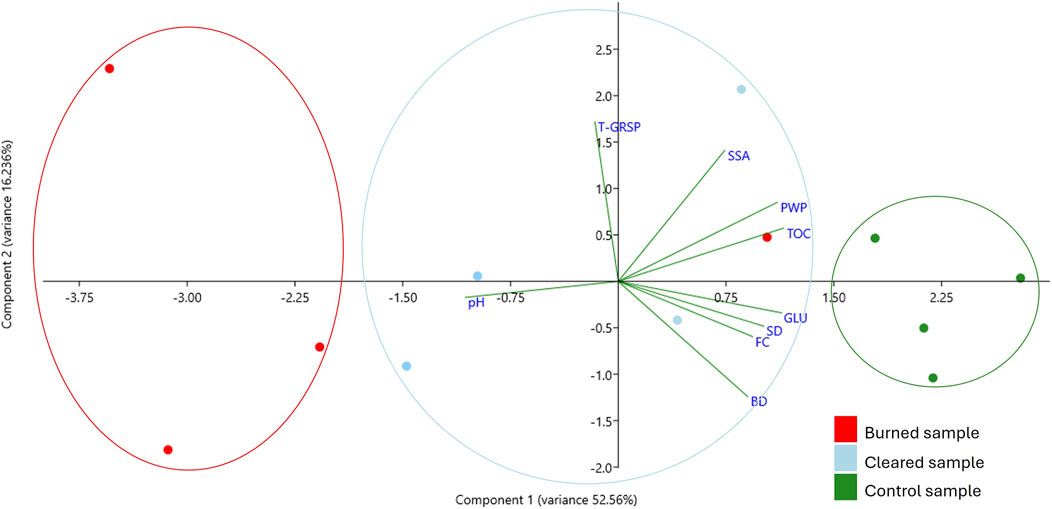
Figure 7. PCA of burned, cleared, and control samples, with principal component 1 explaining 52.56% of the variance and principal component 2 explaining 16.24%.
Control samples cluster in the positive region of Component 1 and the negative region of Component 2. This suggests that variables with strong positive loadings in Component 1, such as TOC (0.40) and GLU (0.39), are characteristic of these samples. On the other hand, variables with weaker contributions, such as pH (−0.37), show an inverse relationship with the positive loadings of this component.
Burned samples tend to occupy the negative quadrants of both components, reflecting a distinct pattern influenced by variables like BD and FC, which are moderately correlated with Component 1 (0.31 and 0.32, respectively). Cleared samples are more scattered, but they tend to align with the positive region of Component 2, influenced by variables such as T-GRSP (0.59) and SAS (0.49), which show strong positive loadings on this axis.
The relationship between variables and components highlights divergence. For example, T-GRSP shows a strong association with Component 2 but a near-zero contribution to Component 1, indicating its specific influence on certain samples. In contrast, variables like PWP and TOC contribute positively to both components, indicating a broader relationship with multiple sample types. These patterns suggest that variables positively associated with Component 1, such as TOC and GLU, are key markers for control samples, while those strongly associated with Component 2, like T-GRSP, may characterize cleared samples. Burned samples reflect unique associations with negative loadings, which may indicate altered soil properties or organic matter degradation in these samples.
The PCA determines that for the cleared and burned samples, T-GRSP and SAS in Component 2 carry greater weight, whereas for control samples, TOC and GLU in Component 1 are more influential.
Combining the results from the biological properties section with the PCA, it is observed that cleared soils exhibit higher microbial activity, which could be related to the increased presence of DOC and GLU (Debouk et al., 2020). Dissolved organic carbon is a readily accessible carbon source for soil microorganisms, serving as both an energy and nutrient source. Microorganisms use this carbon as a primary energy source, accelerating the decomposition of soil organic matter, increasing mineralization rates, and enhancing microbial respiration (Fierer and Schimel, 2002; Kalbitz et al., 2000). GLU catalyzes the hydrolysis of glycosidic bonds in complex carbohydrates, breaking them down into simple sugars that microorganisms can use as energy sources (Allison and Vitousek, 2005; Bell et al., 2008; Sinsabaugh et al., 2008).
The substantial impact of burning on soil bacteria is often emphasized, which might seem contradictory to the results obtained. This discrepancy can be explained by changes in the proportion of oxidizable organic carbon caused by fire compared to total organic carbon. When the ratio (OOC/TOC) is calculated, the burned plot has the highest proportion (OOC/TOC = 5.18), compared to the cleared and control plots, which have values of 4.11 and 3.73, respectively. This suggests that, although the lower species richness in the burned plot (Table 2), the increased availability of DOC may result in higher biological activity at the onset of post-fire recovery, compensating for the lower number of microorganisms. However, this initial increase in microbial activity may eventually be followed by the depletion of remaining carbon and nitrogen forms (Mataix-Solera et al., 2009).
On the other hand, the greater weight of SAS for both treatments may be due to its indirect alteration by fire, as heat released during the fire can directly affect aggregation. This in turn can have indirect effects on vegetation recovery, soil erosion, and degradation (Mataix-Solera et al., 2011). However, in both cases, much of this effect can be attributed to the indirect impacts of the treatments, driven by different vegetative covers. Many studies have shown that several direct effects fade over the short to medium term, leaving primarily indirect effects (Girona-García et al., 2018). A similar case applies to glomalins, where changes in vegetation cover types affect microbial communities (Lozano, 2015).
The heterogeneity of the samples in the burned and cleared plots must be considered when interpreting the results, as it introduces significant variability and high deviations in some determinations. Two subsamples from both treatments shared common soil properties and behaved similarly to the control samples, as shown in Figure 7. This may be due to variations in the shrub control methods applied, such as fire intensity, once again highlighting the importance of the temperatures reached or the amount of vegetation cleared. This heterogeneity is reflected in the total and dissolved organic matter values of these two points, with the burned and cleared plots showing 38.77% and 28.88% more dissolved organic carbon (DOC), and 50.59% and 31.45% more total organic carbon (TOC), respectively, compared to other subsamples.
It is also important to consider the spatial proximity of the plots, which may further explain this heterogeneity. The burned and control plots are adjacent to one another (Figure 2), while the cleared plot is located 0.38 km away. Notably, the two subsamples mentioned earlier are the closest to the transition between plots, which may have influenced their soil properties. Therefore, it is crucial to recognize that the observed differences could be influenced by specific initial conditions of the sites selected for each treatment. Consequently, the results should be interpreted considering both the effects of the treatments and the pre-existing natural characteristics of the sites, which may be modulating the observed responses.
Conclusion
Two years after the management treatments were implemented, both methods were effective in controlling the spread of E. horridum, reducing the presence of monospecific slopes dominated by this species, and promoting the recovery of the original vegetation, fostering grasslands with greater vegetation diversity. However, there were statistically significant differences in the vegetation cover percentages obtained, with the burned plot behaving similarly to the control plot in terms of the percentage of grasses, legumes, and litter. Although both treatments achieved greater plant diversity, this diversity was higher in the cleared plot. For a better evaluation of this diversity, incorporating the control of the target plant community would have been necessary.
Significant differences were found in the soil parameters studied, indicating that soils were primarily affected indirectly by changes in vegetation cover resulting from the implementation of both treatments. More pronounced differences were observed in some physical and chemical properties, such as pH, electrical conductivity, moisture, and bulk density. However, these remained within optimal ranges for the soil and consistently exhibited similar behavior in cleared and burned samples compared to control samples. Biological properties showed the fewest statistically significant differences, with variations observed only in β-D-glucosidase activity and in the k constant values of the single-compartment model and the average residence time of organic matter for basal soil respiration. Nevertheless, the average values obtained suggest that the mechanical treatment or clearing practice yielded the best results 2 years after implementation. This can be attributed to the fact that biological properties are more significantly impacted by burning, as fire affects organisms both directly and indirectly through exposure to flames, gases, or other heat-transferring environments, as well as through changes in soil cover during the disturbance period. Additionally, soil microorganisms are particularly sensitive to environmental changes (Verma and Jayakumar, 2012; Hart et al., 2005).
Therefore, the initial hypothesis is rejected, as statistically significant differences were observed in the physical, chemical, and biological properties of the soil 2 years after the treatments were applied. However, the expected outcome regarding vegetation cover was confirmed, as the burned plot exhibited a lower percentage of E. horridum.
However, it is crucial to recognize that the observed differences in soil and vegetation responses could be partially due to the site’s initial variability or inherent differences in environmental conditions, which may have introduced a degree of pseudoreplication in the experimental design. This variability highlights the importance of cautious interpretation of the results, as separating the effects of the treatment from the pre-existing site conditions may not always be straightforward.
Finally, it is essential to consider the land management history of these soils, the variability in how the treatments were applied, and the environmental heterogeneity of the study area, as these factors play an important role in the potential effects of control methods on soil properties. For future studies, it is crucial to conduct short-, medium-, and long-term monitoring of soil properties and vegetation cover to accurately determine the final effects of the different control methods, as well as to consider designs that minimize pseudoreplication to validate these findings.
Data Availability Statement
The raw data supporting the conclusions of this article will be made available by the authors, without undue reservation.
Author Contributions
SQ-E and DB-V performed the field sampling; SQ-E conducted the experiments; SQ-E, DB-V, and CM-D wrote the manuscript; SQ-E and CM-D performed the statistical analysis. All authors contributed to the article and approved the submitted version.
Funding
The author(s) declare that financial support was received for the research, authorship, and/or publication of this article. Financial support for this work comes from the “Investigo” program contract, funded through the Recovery and Resilience Mechanism (Next Generation EU), and the GEOFOREST research group.
Conflict of Interest
The authors declare that the research was conducted in the absence of any commercial or financial relationships that could be construed as a potential conflict of interest.
Acknowledgments
We thank Ramón and Francisco Allué, ranchers from Asín de Broto, for their collaboration in sampling and transportation.
References
Agbeshie, A. A., Abugre, S., Atta-Darkwa, T., and Awuah, R. (2022). A Review of the Effects of Forest Fire on Soil Properties. J. For. Res. 33 (5), 1419–1441. doi:10.1007/s11676-022-01475-4
Alados, C. L., Saiz, H., Nuche, P., Gartzia, M., Komac, B., Frutos, D. E., et al. (2019). Clearing vs. Burning for Restoring Pyrenean Grasslands after Shrub Encroachment. Geogr. Res. Lett. 45 (2), 441–468. doi:10.18172/cig.3589
Alcañiz, M., Outeiro, L., Francos, M., and Úbeda, X. (2018). Effects of Prescribed Fires on Soil Properties: A Review. Sci. Total Environ. 613, 944–957. doi:10.1016/j.scitotenv.2017.09.144
Alfaro-Leranoz, A., Badia-Villas, D., Marti-Dalmau, C., Emran, M., Conte-Dominguez, A. P., and Ortiz-Perpiña, O. (2023). Long-term Evolution of Shrub Prescribed Burning Effects on Topsoil Organic Matter and Biological Activity in the Central Pyrenees (NE-Spain). Sci. Total Environ. 888, 163994. doi:10.1016/j.scitotenv.2023.163994
Alfaro-Leranoz, A., Badía-Villas, D., Martí-Dalmau, C., Escuer-Arregui, M., and Quintana.Esteras, S. (2024). The Effects of Fire Intensity on the Biochemical Properties of a Soil under Scrub in the Pyrenean Subalpine Stage. Fire 7, 452. doi:10.3390/fire7120452
Alfaro-Leranoz, A., Emran, M., and Badía-Villas, D. (2022). Glomalin-related soil protein evolution after prescribed burning of scrub in mountain soils (Central Pyrenees, NE-Spain). Rev. Ciencias Agrar. 45 (4), 477–481. doi:10.19084/rca.28572
Allison, S. D., and Vitousek, P. M. (2005). Responses of Extracellular Enzymes to Simple and Complex Nutrient Inputs. Soil Biol. Biochem. 37 (5), 937–944. doi:10.1016/j.soilbio.2004.09.014
Amsten, K., Cromsigt, J. P., Kuijper, D. P., Loberg, J. M., Jung, J., Strömgren, M., et al. (2024). Pyric Herbivory in a Temperate European Wood-pasture System. J. Appl. Ecol. 61 (5), 1081–1094. doi:10.1111/1365-2664.14618
Anderson, J. P. (1983). Soil Respiration. Methods soil analysis part 2 Chem. Microbiol. Prop. 9, 831–871. doi:10.2134/agronmonogr9.2.2ed.c41
Armas-Herrera, C. M., Martí, C., Badía, D., Ortiz-Perpiñá, O., Girona-García, A., and Porta, J. (2016). Immediate Effects of Prescribed Burning in the Central Pyrenees on the Amount and Stability of Topsoil Organic Matter. Catena 147, 238–244. doi:10.1016/j.catena.2016.07.016
Aslam, A., and Haider, F. U. (2022). “Effects of Mulching on Soil Biota and Biological Indicators of Soil Quality,” in Mulching in Agroecosystems: Plants, Soil and Environment (Singapore: Springer Nature Singapore), 15–40.
Bell, C., McIntyre, N., Cox, S., Tissue, D., and Zak, J. (2008). Soil Microbial Responses to Temporal Variations of Moisture and Temperature in a Chihuahuan Desert Grassland. Microb. Ecol. 56 (1), 153–167. doi:10.1007/s00248-007-9333-z
Berninger, F., Susiluoto, S., Gianelle, D., and Balzarolo, M. (2015). Management and Site Effects on Carbon Balances of European Mountain Meadows and Rangelands. Boreal Environ. Res. 20 (6), 748–760.
Bisdom, E. B. A., Dekker, L. W., and Schoute, J. F. T. (1993). Water Repellency of Sieve Fractions from Sandy Soils and Relationships with Organic Material and Soil Structure. Geoderma 56, 105–118. doi:10.1016/0016-7061(93)90103-R
Blake, G. R., and Hartge, K. H. (1986). “Methods of Soil Analysis. Part 1,” in Physical and Mineralogical Methods. 2nd Edn. (Madison, Wisconsin: A.S.A. – S.S.S.A).
Blanco, H., and Lal, R. (2008). Principles of Soil Conservation and Management, 167169. New York: Springer.
Bodí, M. B., Martin, D. A., Balfour, V. N., Santín, C., Doerr, S. H., Pereira, P., et al. (2014). Wildland Fire Ash: Production, Composition and Eco-Hydro-Geomorphic Effects. Earth-Science Rev. 130, 103–127. doi:10.1016/j.earscirev.2013.12.007
Braun, S., Thomas, V. F. D., Quiring, R., and Flückiger, W. (2010). Does Nitrogen Deposition Increase Forest Production? The Role of Phosphorus. Environ. Pollut. 158 (6), 2043–2052. doi:10.1016/j.envpol.2009.11.030
Canals, R. M., Múgica, L., Durán, M., and San Emeterio, L. (2024). Restorative Pyric Herbivory Practices in Shrub-Encroached Grasslands Enhance Nutrient Resource Availability And Spatial Heterogeneity. Agric. Ecosyst. Environ., 109072. doi:10.1016/j.agee.2024.109072
Castillo-Garcia, M., Alados, C. L., Ramos, J., and Pueyo, Y. (2024). Effectiveness of Two Mechanical Shrub Removal Treatments for Restoring Sub-alpine Grasslands Colonized by Re-sprouting Woody Vegetation. J. Environ. Manag. 349, 119450. doi:10.1016/j.jenvman.2023.119450
Clarke, P. J., Lawes, M. J., Midgley, J. J., Lamont, B. B., Ojeda, F., Burrows, G. E., et al. (2013). Resprouting as a Key Functional Trait: How Buds, Protection and Resources Drive Persistence after Fire. New phytol. 197 (1), 19–35. doi:10.1111/nph.12001
Cortijos-López, M., Navarrete, P. S., de la Parra Muñoz, I., Martínez, T. L., and Romero, M. E. N. (2023). Secuestro de carbono en suelos de media montaña mediterránea mediante la estrategia del desbroce de matorral. Geogr. cambios, retos Adapt., 355–363. doi:10.21138/cg/2023.lc
Cottani, F., and Sabattini, R. A. (2006). Manejo y control de arbustivas en un pastizal con alta carga animal en pastoreo rotativo. Rev. Científica Agropecu. 10 (2), 109–120.
Cummings, J., and Smith, D. (2000). The Line-Intercept Method: A Tool for Introductory Plant Ecology Laboratories. Education 22.
Davies, G. M., and Gray, A. (2015). Don't Let Spurious Accusations of Pseudoreplication Limit Our Ability to Learn from Natural Experiments (And Other Messy Kinds of Ecological Monitoring). Ecol. Evol. 5 (22), 5295–5304. doi:10.1002/ece3.1782
Debouk, H., San Emeterio, L., Marí, T., Canals, R. M., and Sebastià, M. T. (2020). Plant Functional Diversity, Climate and Grazer Type Regulate Soil Activity in Natural Grasslands. Agronomy 10 (9), 1291. doi:10.3390/agronomy10091291
Doerr, S. H., Shakesby, R. A., and Walsh, R. P. D. (2000). Soil Water Repellency: Its Causes, Characteristics and Hydro-Geomorphological Significance. Earth Sci. Rev. 51 (1–4), 33–65. doi:10.1016/S0012-8252(00)00011-8
Eberhardt, L. L. (1978). Transect Methods for Population Studies. J. Wildl. Manag. 42 (1), 1. doi:10.2307/3800685
Eivazi, F., and Tabatabai, M. A. (1988). Glucosidases and Galactosidases in Soils. Soil Biol. Biochem. 20 (5), 601–606. doi:10.1016/0038-0717(88)90141-1
Fernández de Ana-Magán, F. J. (2000). El Fuego Y Los Hongos. 1 Cuad. la Soc. Española Ciencias For. (9), 101–107.
Fierer, N., and Schimel, J. P. (2002). Effects of Drying-Rewetting Frequency on Soil Carbon and Nitrogen Transformations. Soil Biol. Biochem. 34 (6), 777–787. doi:10.1016/S0038-0717(02)00007-X
Fontúrbel, M. T., Fernández, C., and Vega, J. A. (2016). Prescribed Burning versus Mechanical Treatments as Shrubland Management Options in NW Spain: Mid-term Soil Microbial Response. Appl. Soil Ecol. 107, 334–346. doi:10.1016/j.apsoil.2016.07.008
Fontúrbel, M. T., Jiménez, E., Merino, A., and Vega, J. A. (2024). Contrasting Immediate Impact of Prescribed Fires and Experimental Summer Fires on Soil Organic Matter Quality and Microbial Properties in the Forest Floor and Mineral Soil in Mediterranean Black Pine Forest. Sci. Total Environ. 907, 167669. doi:10.1016/j.scitotenv.2023.167669
Francos, M., Pereira, P., Mataix-Solera, J., Arcenegui, V., Alcañiz, M., and Úbeda, X. (2018). How Clear-Cutting Affects Fire Severity and Soil Properties in a Mediterranean Ecosystem. J. Environ. Manag. 206, 625–632. doi:10.1016/j.jenvman.2017.11.011
Frazer, D. W., McColl, J. G., and Powers, R. F. (1990). Soil Nitrogen Mineralization in a Clearcutting Chronosequence in a Northern California Conifer Forest. Soil Sci. Soc. Am. J. 54 (4), 1145–1152. doi:10.2136/sssaj1990.03615995005400040038x
García Lucas, E. (2013). Estrategias para la recuperación de suelos degradados en ambientes semiáridos: adición de dosis elevadas de residuos orgánicos de origen urbano y su implicación en la fijación de carbono. Doctoral Dissertation. Murcia, España: Universidad de Murcia, 363.
Garnica, J. G. F., and Solorio, J. D. D. B. (1995). Efecto de las quemas prescritas sobre algunas características del suelo en un rodal de pino. Rev. Mex. Ciencias For. 20 (77), 113–128.
Gelabert, P. J., Rodrigues, M., de la Riva, c, Ameztegui, A., Sebastià, M. T., and Vega-Garcia, C. (2021). LandTrendr Smoothed Spectral Profiles Enhance Woody Encroachment Monitoring. Remote Sens. Environ. 262, 112521. doi:10.1016/j.rse.2021.112521
Ghani, A., Dexter, M., and Perrott, K. W. (2003). Hot-water Extractable Carbon in Soils: A Sensitive Measurement for Determining Impacts of Fertilisation, Grazing and Cultivation. Soil Biol. Biochem. 35 (9), 1231–1243. doi:10.1016/S0038-0717(03)00186-X
Girona-García, A., Ortiz-Perpiñá, O., and Badía-Villas, D. (2019). Dynamics of Topsoil Carbon Stocks after Prescribed Burning for Pasture Restoration in Shrublands of the Central Pyrenees (NE-Spain). J. Environ. Manag. 233, 695–705. doi:10.1016/j.jenvman.2018.12.057
Girona-García, A., Ortiz-Perpiñá, O., Badía-Villas, D., and Martí-Dalmau, C. (2018). Effects of Prescribed Burning on Soil Organic C, Aggregate Stability and Water Repellency in a Subalpine Shrubland: Variations Among Sieve Fractions and Depths. Catena 166, 68–77. doi:10.1016/j.catena.2018.03.018
González-Pérez, J. A., González-Vila, F. J., Almendros, G., and Knicker, H. (2004). The Effect of Fire on Soil Organic Matter—A Review. Environ. Int. 30 (6), 855–870. doi:10.1016/j.envint.2004.02.003
Hamman, S. T., Burke, I. C., and Knapp, E. E. (2008). Soil Nutrients and Microbial Activity after Early and Late Season Prescribed Burns in a Sierra Nevada Mixed Conifer Forest. For. Ecol. Manag. 256 (3), 367–374. doi:10.1016/j.foreco.2008.04.030
Hammer, D. A. T., Ryan, P. D., Hammer, Ø., and Harper, D. A. T. (2001). Past: Paleontological Statistics Software Package for Education and Data Analysis. Available at: http://palaeo-electronica.orghttp://palaeo-electronica.org/2001_1/past/issue1_01.htm.
Hart, S. C., DeLuca, T. H., Newman, G. S., MacKenzie, M. D., and Boyle, S. I. (2005). Post-Fire Vegetative Dynamics as Drivers of Microbial Community Structure and Function in Forest Soils. For. Ecol. Manag. 220 (1–3), 166–184. doi:10.1016/j.foreco.2005.08.012
Hashimoto, S., and Suzuki, M. (2004). The Impact of Forest Clear-Cutting on Soil Temperature: A Comparison between before and after Cutting, and between Clear-Cut and Control Sites. J. For. Res. 9 (2), 125–132. doi:10.1007/s10310-003-0063-x
Heiri, O., Lotter, A. F., and Lemcke, G. (2001). Loss on Ignition as a Method for Estimating Organic and Carbonate Content in Sediments: Reproducibility and Comparability of Results. J. Paleolimnol. 25 (1), 101–110. doi:10.1023/A:1008119611481
Hobley, E. U., Brereton, A. J. L. G., and Wilson, B. (2017). Forest Burning Affects Quality and Quantity of Soil Organic Matter. Sci. Total Environ. 575, 41–49. doi:10.1016/j.scitotenv.2016.09.231
Huhta, V. (1976). Effects of Clear-Cutting on Numbers, Biomass and Community Respiration of Soil Invertebrates. Ann. Zool. Fenn. 131 (1), 63–80.
Hynes, H. M., and Germida, J. J. (2013). Impact of Clear Cutting on Soil Microbial Communities and Bioavailable Nutrients in the LFH and Ae Horizons of Boreal Plain Forest Soils. For. Ecol. Manag. 306, 88–95. doi:10.1016/j.foreco.2013.06.006
Instituto Geológico y Minero de España (IGME) (1972). Mapa geológico de España – Broto. IGME. Available at: https://info.igme.es/cartografiadigital/datos/magna50/pdfs/d1_G50/Magna50_178.pdf (Accessed June 20, 2024).
IUSS Working Group WRB (2022). “World Reference Base for Soil Resources,” in International Soil Classification System for Naming Soils and Creating Legends for Soil Maps. 4th Edn. (Vienna, Austria: International Union of Soil Sciences (IUSS)).
Kalbitz, K., Solinger, S., Park, J. H., Michalzik, B., and Matzner, E. (2000). Controls on the Dynamics Dissolved Organic Matter in Soils: A Review. Soil Sci. 165 (4), 277–304. doi:10.1097/00010694-200004000-00001
Kemper, W. D., and Koch, E. J. (1966). “Aggregate Stability of Soils from Western United States and Canada,” in Measurement Procedure, Correlation with Soil Constituents (Washington, DC: United State Department of Agriculture).
Klute, A. (1986). Methods of Soil Analysis. Part 1. Physical and Mineralogical Methods. 2on. ed. A.S.A. - S.S.S.A. Madison, Wisconsin.
Knicker, H. (2007). How Does Fire Affect the Nature and Stability of Soil Organic Nitrogen and Carbon? A Review. Biogeochemistry 85 (1), 91–118. doi:10.1007/s10533-007-9104-4
Komac, B., Alados, C., and Camarero, J. (2011). Influence of Topography on the Colonization of Subalpine Grasslands by the Thorny Cushion Dwarf Echinospartum Horridum. Arct. Antarct. Alp. Res. 43 (4), 601–611. doi:10.1657/1938-4246-43.4.601
Koner, S., Chen, J. S., Hsu, B. M., Tan, C. W., Fan, C. W., Chen, T. H., et al. (2021). Assessment of Carbon Substrate Catabolism Pattern and Functional Metabolic Pathway for Microbiota of Limestone Caves. Microorganisms 9 (8), 1789. doi:10.3390/microorganisms9081789
Lasanta, T. (2019). Gestión Activa Frente a Matorralización: Buscando el Equilibrio Entre Conservación y Explotación en Montaña. Cuad. Investig. Geográfica 45 (2), 423–440. doi:10.18172/cig.3726
Liang, G., Hui, D., Wu, X., Wu, J., Liu, J., Zhou, G., et al. (2016). Effects of Simulated Acid Rain on Soil Respiration and its Components in a Subtropical Mixed Conifer and Broadleaf Forest in Southern China. Environ. Sci. Process. Impacts 18 (2), 246–255. doi:10.1039/c5em00434a
Lombao, A., Barreiro, A., Fontúrbel, M. T., Martín, A., Carballas, T., and Díaz-Raviña, M. (2020). Key Factors Controlling Microbial Community Responses after a Fire: Importance of Severity and Recurrence. Sci. Total Environ. 741, 140363. doi:10.1016/j.scitotenv.2020.140363
Lombao, A., Barreiro, A., Fontúrbel, M. T., Martín, A., Carballas, T., and Díaz-Raviña, M. (2021). Effect of Repeated Soil Heating at Different Temperatures on Microbial Activity in Two Burned Soils. Sci. Total Environ. 799, 149440. doi:10.1016/j.scitotenv.2021.149440
Lozano, E. (2015). Sensibilidad de la Glomalina a los Efectos Provocados por el Fuego en el Suelo y su Relación con la Repelencia al Agua en Suelos Forestales Mediterráneos (Doctoral Dissertation, Universidad Miguel Hernández de Elche).
Magurran, A. E. (2013). Ecological Diversity and its Measurement. Dordrecht: Springer Science & Business Media, 177.
Martínez, O., Valenzuela, E., and Godoy, R. (2006). Poblaciones viables y grupos funcionales de hongos presentes en suelos de bosque de Araucaria-Nothofagus post-incendio. Bol. Micológico 21. doi:10.22370/bolmicol.2006.21.0.258
Martínez Romero, A., and Leyva Galán, A. (2014). La biomasa de los cultivos en el ecosistema. Sus beneficios agroecológicos -. Cultiv. Trop. 35 (1).
Mataix-Solera, J., Cerdà, A., Arcenegui, V., Jordán, A., and Zavala, L. M. (2011). Fire Effects on Soil Aggregation: A Review. Earth-Science Rev. 109 (1-2), 44–60. doi:10.1016/j.earscirev.2011.08.002
Mataix-Solera, J., Guerrero, C., García-Orenes, F., Bárcenas, G. M., and Torres, M. P. (2009). “Forest Fire Effects on Soil Microbiology,” in Fire Effects on Soils and Restoration Strategies. doi:10.1201/9781439843338-c5
Montserrat, P., Montserrat Martí, J. M., and Montserrat-Martí, G. (1984). Estudio de las comunidades de Echinospartum horridum en el Pirineo español. Acta Biol. Montserrat. 4 (IV), 249–257.
Mora, J. L., Badía–Villas, D., and Gómez, D. (2022). Fire Does Not Transform Shrublands of Echinospartum horridum (Vahl) Rothm. Into Grasslands in the Pyrenees: Development of Community Structure and Nutritive Value after Single Prescribed Burns. J. Environ. Manag. 315, 115125. doi:10.1016/j.jenvman.2022.115125
Moya, D., Fonturbel, T., Peña, E., Alfaro-Sanchez, R., Plaza-Álvarez, P. A., González-Romero, J., et al. (2022). Fire Damage to the Soil Bacterial Structure and Function Depends on Burn Severity: Experimental Burnings at a Lysimetric Facility (MedForECOtron). Forests 13 (7), 1118. doi:10.3390/f13071118
Múgica, L., Le Roux, X., San Emeterio, L., Cantarel, A., Durán, M., Gervaix, J., et al. (2024). Pyric Herbivory Decreases Soil Denitrification Despite Increased Nitrate Availability in a Temperate Grassland. J. Environ. Manag. 365, 121695. doi:10.1016/j.jenvman.2024.121695
Muñoz-Iniestra, D. J., López, G. F., Hernández, M. M., Soler, A. A., and López, G. J. (2009). Impacto de la pérdida de la vegetación sobre las propiedades de un suelo aluvial. Terra Latinoam. 27 (3), 237–246.
Nadal-Romero, E., Otal-Laín, I., Lasanta, T., Sánchez-Navarrete, P., Errea, P., and Cammeraat, E. (2018). Woody Encroachment and Soil Carbon Stocks in Subalpine Areas in the Central Spanish Pyrenees. Sci. Total Environ. 636, 727–736. doi:10.1016/j.scitotenv.2018.04.324
Nadal-Romero, E., Rubio, P., Kremyda, V., Absalah, S., Cammeraat, E., Jansen, B., et al. (2021). Effects of Agricultural Land Abandonment on Soil Organic Carbon Stocks and Composition of Soil Organic Matter in the Central Spanish Pyrenees. Catena 205, 105441. doi:10.1016/j.catena.2021.105441
Page, A. L., Miller, R. H., and Keeney, D. R. (1982). “Methods of Soil Analysis. Part 2,” in Chemical and Microbiological Methods. 2on. ed. A.S.A. - S.S.S.A. Madison, Wisconsin.
Pereira, J. S., Badía, D., Martí, C., Mora, J. L., and Donzeli, V. P. (2023). Fire Effects on Biochemical Properties of a Semiarid Pine Forest Topsoil at Cm-Scale. Pedobiologia 96, 150860. doi:10.1016/j.pedobi.2022.150860
Pietikäinen, J., and Fritze, H. (1995). Clear-Cutting and Prescribed Burning in Coniferous Forest: Comparison of Effects on Soil Fungal and Total Microbial Biomass, Respiration Activity and Nitrification. Soil Biol. Biochem. 27 (1), 101–109. doi:10.1016/0038-0717(94)00125-K
Pineda, M. E. B., and Lizarazo-Forero, L. M. (2013). Grupos funcionales de microorganismos en suelos de páramo perturbados por incendios forestales. Rev. Ciencias 17 (2), 121–136.
Rillig, M. C., Wright, S. F., Nichols, K. A., Schmidt, W. F., and Torn, M. S. (2001). Large Contribution of Arbuscular Mycorrhizal Fungi to Soil Carbon Pools in Tropical Forest Soils. Plant Soil 233 (2), 167–177. doi:10.1023/A:1010364221169
Rovira, P., and Ramón Vallejo, V. (2007). Labile, Recalcitrant, and Inert Organic Matter in Mediterranean Forest Soils. Soil Biol. Biochem. 39 (1), 202–215. doi:10.1016/j.soilbio.2006.07.021
Rutgers, M., Wouterse, M., Drost, S. M., Breure, A. M., Mulder, C., Stone, D., et al. (2016). Monitoring Soil Bacteria with Community-Level Physiological Profiles Using Biolog™ ECO-Plates in the Netherlands and Europe. Appl. Soil Ecol. 97, 23–35. doi:10.1016/j.apsoil.2015.06.007
San Emeterio, L., Múgica, L., Ugarte, M. D., Goicoa, T., and Canals, R. M. (2016). Sustainability of Traditional Pastoral Fires in Highlands under Global Change: Effects on Soil Function and Nutrient Cycling. Agric. Ecosyst. Environ. 235, 155–163. doi:10.1016/j.agee.2016.10.009
San-Emeterio, L. M., Lozano, E., Arcenegui, V., Mataix-Solera, J., Jiménez-Morillo, N. T., and González-Pérez, J. A. (2024). Soil-Easily Extractable Glomalin: An Innovative Approach to Deciphering its Molecular Composition under the Influence of Seasonality, Vegetation Cover, and Wildfire. Environ. Sci. & Technol. 58, 22624–22634. doi:10.1021/acs.est.4c10036
Santín, C., and Doerr, S. H. (2016). Fire Effects on Soils: The Human Dimension. Philosophical Trans. R. Soc. B Biol. Sci. 371 (1696), 20150171. doi:10.1098/rstb.2015.0171
Sardans, J., Peñuelas, J., and Estiarte, M. (2008). Changes in Soil Enzymes Related to C and N Cycle and in Soil C and N Content under Prolonged Warming and Drought in a Mediterranean Shrubland. Appl. Soil Ecol. 39 (2), 223–235. doi:10.1016/j.apsoil.2007.12.011
Schirpke, U., Kohler, M., Leitinger, G., Fontana, V., Tasser, E., and Tappeiner, U. (2017). Future Impacts of Changing Land-Use and Climate on Ecosystem Services of Mountain Grassland and Their Resilience. Ecosyst. Serv. 26, 79–94. doi:10.1016/j.ecoser.2017.06.008
Simón, L., Hernández, M., Reyes, F., and Sánchez, S. (2005). Efecto de las leguminosas arbóreas en el suelo y en la productividad de los cultivos acompañantes. Pastos Forrajes 28 (1), 29–45.
Sinsabaugh, R. L., Lauber, C. L., Weintraub, M. N., Ahmed, B., Allison, S. D., Crenshaw, C., et al. (2008). Stoichiometry of Soil Enzyme Activity at Global Scale. Ecol. Lett. 11 (11), 1252–1264. doi:10.1111/j.1461-0248.2008.01245.x
Staddon, W. J., Duchesne, L. C., and Trevors, J. T. (1997). Microbial Diversity and Community Structure of Postdisturbance Forest Soils as Determined by Sole-Carbon-Source Utilization Patterns. Microb. Ecol. 34, 125–130. doi:10.1007/s002489900042
Tokarczyk, N. (2017). Forest Encroachment on Temperate Mountain Meadows. Scale, Drivers and Current Research Directions. Geogr. Pol. 90 (4), 463–480. doi:10.7163/GPol.0112
Torres, L. G., and Cabrera, A. H. (2005). “La agricultura de conservación y sus beneficios,” in Protección del suelo y el desarrollo sostenible: Seminario Europeo: Soria, 15-17 de mayo de 2002 (Madrid, España: Instituto Geológico y Minero de España), 91–100.
Vadillo, J. A., Flaño, P. R., Monreal, N. L. R., Romero, M. E. N., Martínez, T. L., and Cortijos-López, M. (2023). “Comportamiento de la Infiltración del Suelo en Diferentes Cubiertas Vegetales y Usos del Suelo en la Media Montaña Mediterránea,” in Geografía: Cambios, Retos y Adaptación: Libro de Actas. XVIII Congreso de la Asociación Española de Geografía, Logroño, 12 al 14 de Septiembre de 2023 (Madrid, España: Asociación Española de Geografía), 291–300.
Verma, S., and Jayakumar, S. (2012). Impact of Forest Fire on Physical, Chemical and Biological Properties of Soil: A Review. Proc. Int. Acad. Ecol. Environ. Sci. 2 (3), 168.
Wright, S. F., and Upadhyaya, A. (1996). Extraction of an Abundant and Unusual Protein from Soil and Comparison with Hyphal Protein of Arbuscular Mycorrhizal Fungi. Soil Sci. 161 (9), 575–586. doi:10.1097/00010694-199609000-00003
Keywords: basal soil respiration, CLPP, glomalin, biodiversity, shrub management
Citation: Quintana-Esteras S, Badía-Villas D and Martí-Dalmau C (2025) Shrub Control by Burning and Clearing in the Southern Pyrenees: Effects on Soils After Two Years of Treatment. Span. J. Soil Sci. 15:13749. doi: 10.3389/sjss.2025.13749
Received: 05 September 2024; Accepted: 29 January 2025;
Published: 19 February 2025.
Edited by:
Iñigo Virto, Universidad Pública de Navarra - ISFOOD, SpainCopyright © 2025 Quintana-Esteras, Badía-Villas and Martí-Dalmau. This is an open-access article distributed under the terms of the Creative Commons Attribution License (CC BY). The use, distribution or reproduction in other forums is permitted, provided the original author(s) and the copyright owner(s) are credited and that the original publication in this journal is cited, in accordance with accepted academic practice. No use, distribution or reproduction is permitted which does not comply with these terms.
*Correspondence: Silvia Quintana-Esteras, c3F1aW50YW5hQHVuaXphci5lcw==