- 1Department of Forest and Soil Sciences, Institute of Soil Research, BOKU University, Vienna, Austria
- 2Department of Chemistry Physics and Environmental and Soil Sciences, Universitat de Lleida, Lleida, Spain
- 3Department of Material Sciences and Process Engineering, Institute of Physics and Materials Science, BOKU University, Vienna, Austria
- 4Galápagos National Park Directorate, Galápagos, Ecuador
Galápagos has very diverse soil resources. In a recent study, we found vastly different pedogenic development on lava vs. scoria along a hydroclimatic gradient on Santa Cruz Island (800 m elevation difference; precipitation ranging from 100 to 1,600 mm a−1). On the more porous scoria deposits, soils consistently developed towards deeper and more weathered profiles with increasing humidity, while on the lavas, soil development did not follow a consistent elevational pattern. The micromorphological investigations show an increasing degree of primary mineral weathering with increasing altitude and humidity, which was more pronounced in the profiles on scoria than on lava. The greater weathering on scoria is likely due to higher inter-particle porosity and therefore larger surface area exposed to weathering. In the arid climate zone, the soil on scoria was very shallow and the primary minerals hardly weathered, whereas the soil on lava showed a greater profile thickness and a noticeable weathering of primary minerals. Unlike the relatively homogeneous scoria deposits, the lavas show irregular flow patterns with large rock outcrop sections and small depression patches, in which soils with higher available water capacity have formed. In a previous study, we also found strongly elevated phosphorus levels in these soil patches in the arid zone. Bolstered by electron microscopy and microanalyses we show evidence that these possibly originate from tortoise nesting activities. Our results point to a complex interplay of parent material, geomorphology, climate and also the activities of the Galápagos giant tortoises in shaping the soil-scape of this unique ecosystem.
Introduction
Climate change, land-use change and intensive land use are undoubtedly modifying soil properties and functions. In order to assess possible changes, a mechanistic understanding of the influence of the five important soil forming factors, parent material, climate, relief, organisms (fauna and flora) and time as defined by (Dokuchaev, 1886) and (Jenny, 1941), is needed. In this paper, we focus on the factors soil parent material and climate in a two-factorial approach, but also address the influence of organisms. We use an appropriate model region, the Galápagos Islands. Their volcanic origin, the different ages of the islands and the greatly varying humidity on the islands has resulted in an enormous diversity of soil resources, which has been documented by several authors in the recent past, some, but not all of them focusing on Santa Cruz Island (Candra et al., 2021; Candra et al., 2023; Stoops, 2013; Stoops et al., 2023; Taboada et al., 2016; Taboada et al., 2019).
The reported diversity of soil resources partly is due to the eastward migration of the Nazca Plate, on which the islands are located. This resulted in a separation of the older islands from the geostationary volcanic hotspot, which lies west of Isabela Island, thereby creating a chain of differently aged islands. Timing has been demonstrated to be a determining factor in soil formation and functions (Wardle et al., 2004). In order to better understand this important factor in soil formation, a chronosequence of soils has been established in the humid climate zone of the four inhabited islands of the Galápagos Archipelago, with soils ranging in age from 1.5 to 1,070 ka (Zehetner et al., 2020). The basis for this was the different ages of the islands and the determination of rock age at the study sites. This chronosequence has since been used to study the development of soil mineralogy, geochemistry, organic carbon and fine particle stocks, as well as the retention properties of soils for heavy metals and phosphorus (Candra et al., 2021; Gerzabek et al., 2022; Mahmoodi et al., 2016; Zehetner et al., 2024). For example, Candra et al. (2021) found a trend in the type of clays, where allophanes and ferrihydrite dominate in the young soils (age of less than 4.3 ka), illite and vermiculite become dominant after 26 ka and kaolinite, gibbsite and hematite prevail in the oldest soils. A recent micromorphological study supported these findings and added interesting information, e.g., the simultaneous presence of kaolinite aggregates formed in situ and aligned kaolinite transported in pores in the second oldest soil (Gerzabek et al., 2023). The soil microstructure evolved to increasing complexity from the young to the intermediate-aged soils but reverted back to less complexity in the very old soils.
A second very important factor in soil formation are the climatic conditions. In particular, the temperature and precipitation are very important parameters influencing soil development, soil properties and functions [e.g., (Sion et al., 2022)]. In fact, soil properties can even be used to infer historical climatic conditions (Guillod et al., 2013). The third important factor in soil formation and development is the parent material type, its weatherability playing a central role [e.g., (Mahmoodi et al., 2016)]. In recent years, some studies using elevation gradients have been carried out in the Galápagos Islands to characterize the climatic influence on soil development. Due to the large differences in precipitation with altitude, the focus is primarily on the effect of humidity rather than temperature, as mean temperature does not vary as strongly with altitude (Lasso et al., 2018). Candra et al. (2019) investigated a transect on Alcedo volcano, Isabela Island, and showed that soil organic carbon (SOC) stocks and phosphate retention decreased sharply with decreasing precipitation and that allophane and ferrihydrite were only found under humid climate, while halloysite was formed in drier locations.
Although early publications about soil formation on Santa Cruz Island date back to the 1970ies of the last century (Eswaran et al., 1973; Morrás, 1976), the first comprehensive micromorphological study about soils of Santa Cruz Island, based on sampling during the first soil scientific expedition to the Galápagos archipelago in the year 1962, was published only in 2013 (Stoops, 2013). In that study, 60 profiles on the windward side of the island were analyzed. Lower weathering degree of soils in the arid, coastal zone and enhanced weathering under more humid conditions at higher elevations were clearly documented by clay mineral types and their distribution, the c/f related distribution patterns and the occurrence of gibbsite (Stoops, 2013). In Candra et al. (2023), we showed that, in addition to the climatic differences along the slopes of Santa Cruz Island, differences in parent material type, i.e., lava vs. scoria, despite geochemical similarity, exerted a significant influence on pedogenic development. On the highly porous and less crystalline scoria deposits, the soils showed consistent development towards deeper and more weathered profiles with increasing humidity. On the denser and comparatively more crystalline lavas, pedogenesis did not follow a consistent altitudinal pattern. On scoria, the soil types from arid to very humid climatic conditions changed from Regosol to Umbrisol to Andosol, while on lava they changed from Cambisol to Phaeozem to Andosol (Table 1) (Candra et al., 2023; Rechberger et al., 2021). In the present work, we use micromorphological and microanalysis methods to test the hypothesis that interactions between the factors parent material and climate drive the observed differential pedogenic development on lava vs. scoria. An additional research question concerns an anomaly in the mobile soil phosphorus content previously observed for the arid lava site of the aforementioned (Candra et al., 2023) study. For that site, Rechberger et al. (2021) had reported particularly high Mehlich-3-extractable P contents (>1,000 mg kg−1; remaining sites ranging between 3.8 and 50 mg kg−1). Here, our intention was to elucidate this phenomenon using micromorphological and microanalysis approaches.
Materials and Methods
Study Area
The Galápagos archipelago comprises 15 larger islands, most of which are uninhabited. It is situated almost exactly on the equator, about 1,100 km west of the Ecuadorian coast, thus being geographically quite isolated. A detailed description of the geological and climatic conditions as well as the vegetation cover and parent materials can be found in (Candra et al., 2021; Zehetner et al., 2020; Sauzet et al., 2017). The islands consist mainly of basaltic lava (White et al., 1993). The sites investigated in this study are located on basaltic scoria deposits or lava flows. The climate of the islands is cooler and drier than its equatorial location would suggest. Rainfall varies greatly, being higher on the windward side of the islands than on the leeward side, which is almost entirely dry. The amount of precipitation increases with altitude. Unfortunately, there are only few climate records. For the studied island Santa Cruz some information is available. According to isohyets developed by Trueman and d’Ozouville (2010), mean annual precipitation in the dry lowlands and humid highlands can be estimated at 100–500 mm and up to 1,600 mm, respectively. Average annual temperatures are between 23°C and 25°C in the lowlands and 18°C–21°C in the highlands (Lasso et al., 2018). The climatic zones, which range from arid to very humid, cause a high diversity of vegetation. While xerophytes dominate in the arid lowlands, the more humid areas are home to forest vegetation (Bowman, 1963; Hamann, 1981). In the humid zones agricultural land use is also common (Adapted with permission from “2. Material and methods” by Candra et al., licensed under CC BY 4.0).
Study Sites and Sampling
This study builds on a previously described elevation sequence on Santa Cruz Island (Candra et al., 2023), which includes sites in the arid (coastal area), the humid (approx. 400–500 m asl) and the very humid zone (>800 m asl) (Figure 1). In each of these climate zones, soils developed on two parent material types, i.e., lava (sites E1, E3, E5) and scoria (sites E2, E4, E6) were compared (Table 1; Figure 1; Supplementary Figure S1). Hence, the study follows a two-factorial approach. Recent 40Ar/39Ar dating shows an age span of the parent materials at the study sites of approximately 45–165 ka (Paque et al., 2024). The bulk density of the lavas ranges from 2.0 to 2.3 g cm−3 whereas that of the scorias ranges from 1.2 to 1.6 g cm−3 (Candra et al., 2023), which is caused by pronounced differences in the materials’ particle vesicularity and inter-particle porosity. Furthermore, the scorias contain a larger amorphous fraction compared to the more crystalline lavas. The lava sites are located on a’a flows, and the scorias are composed of loosely packed larger lapilli and pyroclastic blocks (mainly between 50 and 100 mm) with irregular shapes and sharp edges. The six sites show little to no influence of human land use. The classification of the investigated soils according to the World Reference Base for Soil Resources (WRB), 2022 IUSS Working Group WRB (2022), is described in detail in Candra et al. (2023) and can be found in Table 1.
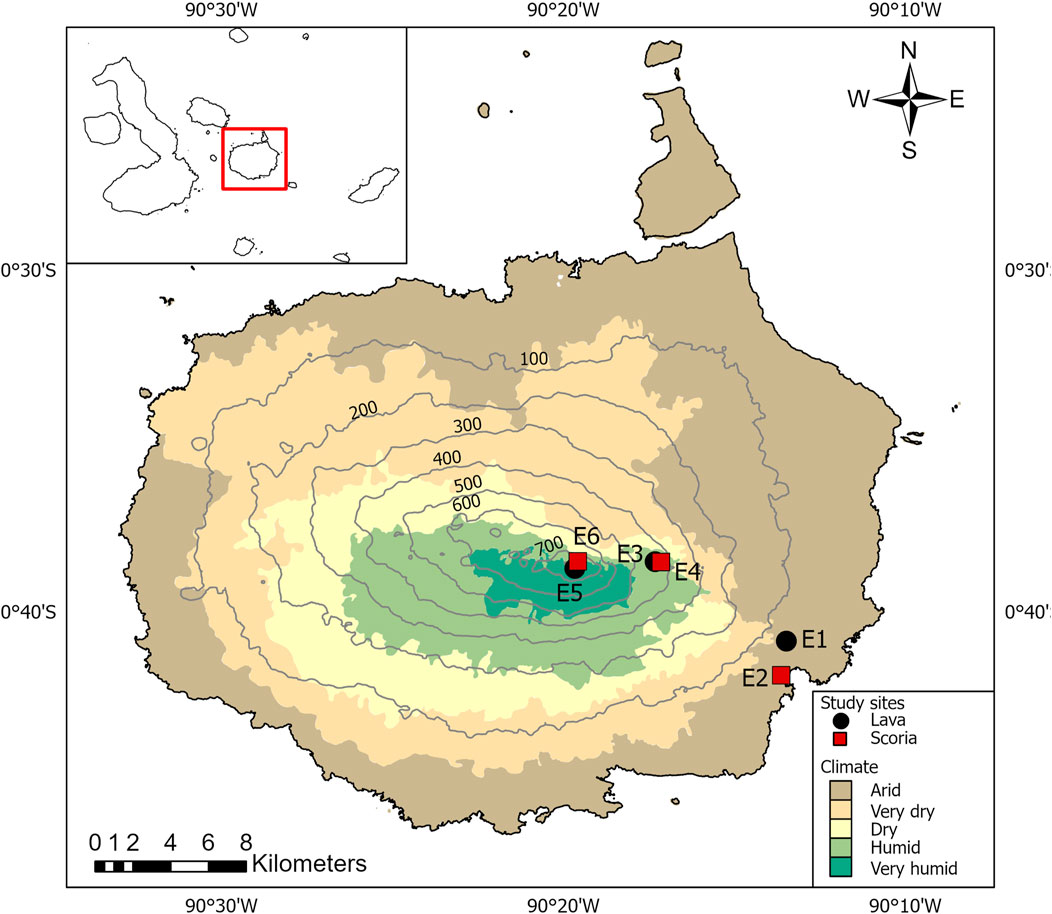
Figure 1. Study sites and climatic zonation on Santa Cruz Island, Galápagos. Climate zones according to PRONAREG – ORSTOM – INGALA (1987): arid = 12 dry months; very dry = 10 to 12 dry months; dry = 8 to 10 dry months; humid = 4 to 8 dry months; very humid = less than 4 dry months. Reproduced with permission from “Study sites and climatic zonation on Santa Cruz Island, Galápagos” by Candra et al., licensed under CC BY 4.0.
At each study site, three replicate soil profiles were separately sampled and analyzed for fundamental soil characteristics. Bulk soil samples were taken according to genetic horizons, air-dried and passed through a 2-mm sieve. Undisturbed samples (Kubiëna boxes) were taken in the field from selected soil horizons of one profile per site and impregnated with polyester after air drying. Uncovered thin sections of 55 by 130 mm were prepared following the methods described by (Stoops, 2013). The thin sections were polished to a thickness of 20 µm. Table 1 provides information about the sites and soil horizons sampled with Kubiëna boxes.
Soil Analyses
Standard soil analyses were performed as already described in detail in (Candra et al., 2021). Tukey’s HSD test with p < 0.05 was used in the program SPSS 16.0 (SPSS Inc. Released 2007) for analyzing significant differences in soil parameter values between studied samples (Candra et al., 2023). Detailed results of statistical analyses of soil parameters presented in Table 1 can be found in (Candra et al., 2023). Descriptions of thin sections were made according to the concepts and terminology proposed by G. Stoops (Stoops, 2003; Stoops, 2021). Micrographs without polarization were recorded with a digital microscope (Keyence VHX-5000), whereas standard polarization microscopes [Bresser Science MPO 401 and an Olympus petrographic microscope (BX51)] were used for mineralogical analyses. For soil mineralogy please see (Candra et al., 2023) and Table 1. The soils’ macro-porosity (50–100 μm in diameter) was determined from the thin sections. For this purpose, the sections were scanned under parallel polarized light (PPL), cross polarized light (XPL) and circular polarized light (CPL) following the methodology of (Torres-Guerrero et al., 2023). Also, a classification was made according to the origin of the macro-porosity following the methodology proposed by (Sauzet et al., 2017). A FEI Quanta 250 FEG (Thermo Fisher Scientific, Waltham, Massachusetts, USA) was used under high vacuum condition and a high tension of 20 kV. Selected features in thin sections were visualized. For this, they were mounted with conductive double sided carbon tape on an aluminum stub. The micrographs were recorded with the Everhart-Thornley-Detector in secondary electron (SE) mode. For elemental analysis, energy dispersive X-ray analysis (EDX) was utilized, employing an Octane Elect 30 mm2 detector with APEX 2.0 Standard software (EDAX, Inc., Mahwah, New Jersey, USA). Elemental distributions were assessed through line scans across transects, allowing for detailed mapping of elemental variation across microstructural features.
Results
General Characteristics of Investigated Soils
The general soil characteristics along the studied elevation sequence are presented in detail in (Candra et al., 2023). Here, the main trends are briefly summarized. Soil pH decreased with increasing humidity on both parent material types (Table 1). The profile thickness of the soils on scoria increased considerably from the arid (E2; 3 cm) to the humid (E4; >100 cm) and very humid climate zones (E6; >150 cm). On lava, a thicker solum has developed in the arid zone (E1; approx. 20–35 cm), which showed comparatively little change in the humid (E3; 50 cm) and very humid zones (E5; 30 cm). The soil at the lava site E1 showed several traits indicative of more advanced pedogenesis compared to the scoria site E2: higher silt, clay and organic carbon (Corg) contents, as well as higher cation exchange capacity (Table 1). On the other hand, further soil development on the lava sites in the humid and very humid zones seems to have proceeded more slowly compared to the scoria sites. Besides the pronounced differences in solum thickness, this is also indicated by a rather constant share (15%–30%) of coarse fragments in the A horizons at the three lava sites E1, E3, and E5, compared to a marked decrease with increasing humidity in the topsoils formed on scoria (from 20% at site E2 to 0% at E4 and E6) (Table 1).
Soil Micromorphological Features
Figures 2, 3 show micrographs of the three lava (E1, E3, E5) and scoria (E2, E4, E6) sites, respectively. Supplementary Table S1 provides a detailed micromorphological description of all investigated soils. At the arid lava site (E1), the microstructure in the A/Bw horizon is weakly subangular blocky juxtaposed with granular due to faunal activity. Coarse material consists of basalt fragments (Figure 2A) with plagioclases (partly phenocrysts) and olivines, as well as quartz fragments, which were found in all profiles in small traces. The b-fabric is mainly undifferentiated. Observed pedofeatures are fecal pellets, orthic and disorthic Fe and Mn oxides and oxalate crystals in root sections. Several shell fragments of tortoise eggs and snail shells are present, as well as microlaminated (illuvial) clay coatings and infillings (Figure 2B).
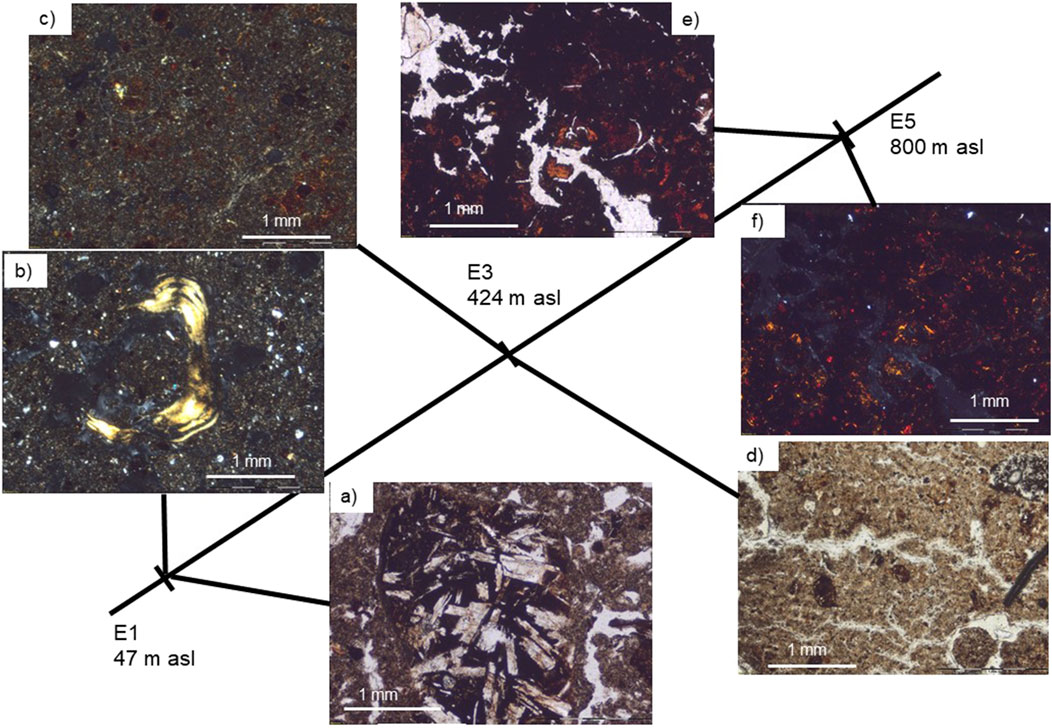
Figure 2. Elevation sequence – profiles on lava: (A) E1, A-horizon, weathered basalt fragment, plagioclases, ppl, (B) E1, A-horizon, illuvial clay, xpl, (C) E3, A-horizon, Fe-oxide nodules with clays, xpl, (D) E3, A-horizon, with characteristic cracks, ppl, (E) E5, A-horizon, Fe-oxide groundmass with newly formed clays, ppl, (F) like (E) but xpl.
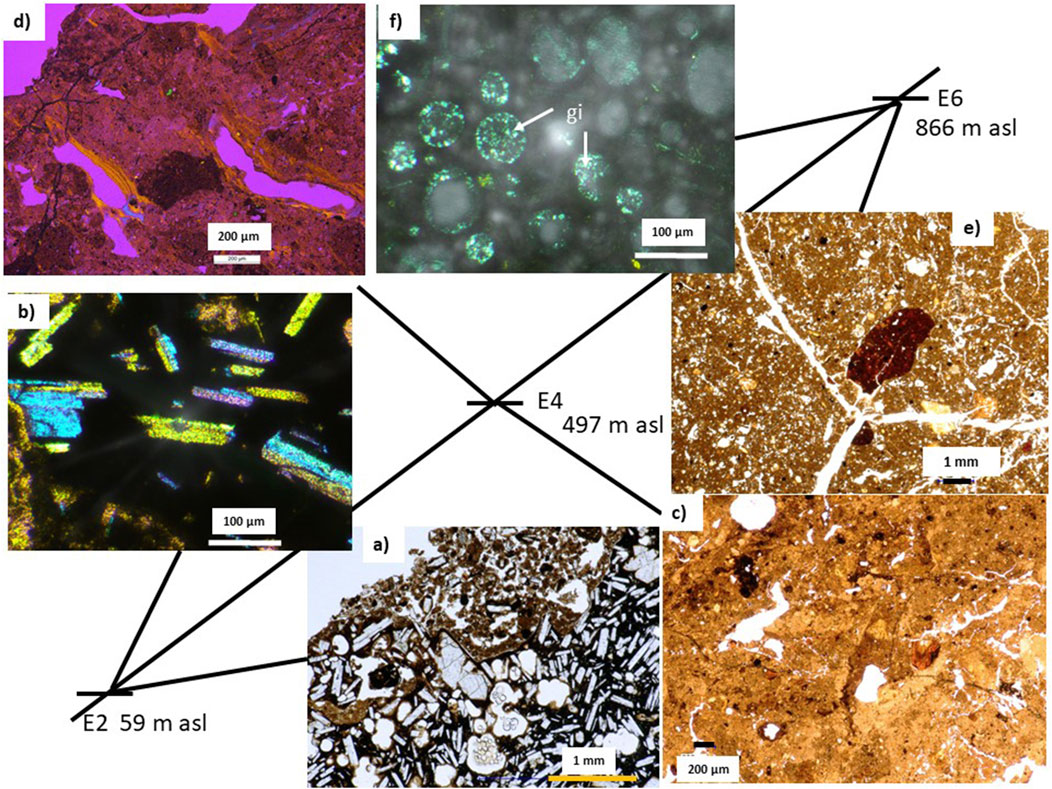
Figure 3. Elevation sequence–profiles on scoria: (A) E2, AC horizon, tachylitic scoria, (B) E2, AC horizon, plagioclase, xpl, lamda (C) E4, Bto horizon, vugh- or channel-intrapedal microstructure, iron oxide nodules, ppl, (D) E4, Bto horizon, clay coatings and infillings (yellow and blue), xpl, lambda, (E) E6, A/E1 horizon, blocky microstructure, iron oxide nodule (hematite dominated), ppl (F) E6, A/E1 horizon, gibbsite (gi) infillings in heavily weathered scoria, xpl.
For the arid scoria site (E2), the thin section (AC horizon) shows very weak signs of weathering. It has an intergrain microaggregate to granular microstructure due to faunal activity. The coarse material is dominated by basaltic fragments and the minerals present are tachylyte, plagioclase and olivine grains (Supplementary Table S1; Figures 3A, B). The plagioclases are only slightly weathered.
The humid lava site (E3) has a strongly subangular blocky microstructure, coarse fragments are mainly basalt with plagioclases and olivines (partially weathered). Additional minerals include few quartz fragments and opaques. The fine material consists of clay minerals, fine silt and organic matter. The b-fabric is undifferentiated to granostriated. Numerous Fe-oxide nodules, partly aggregated and concentric, can be observed in horizon A1 (Table 1; Figures 2C, D).
At the humid scoria site (E4), the microstructure is subangular blocky with intrapedal channels, the coarse material consists of a mixture of yellow-brownish and dark brown highly weathered minerals (not directly recognizable) and iddingsite dominates, although coarse olivines with an iddingsite rim also occur (Supplementary Table S1). Noteworthy pedofeatures are limpid yellow clay coatings and infillings in the Bto horizon, also as.fragments mixed with the groundmass (Figures 3C, D).
The lava profile in the very humid zone (E5) has a primary fine granular, secondary very strong coarse granular microstructure in the topsoil (Figure 2E). The fine material consists of a mixture of clay minerals, fine silt and organic material. The b-fabric is undifferentiated and in the lower part of the thin section there are coatings and infillings of amorphous and oriented clay (Figure 2F). An internal undulating intercalation of clay and Fe-oxides was observed.
The scoria profile in the very humid zone (E6) has an equal intergrain to angular blocky microstructure (Figure 3E) and contains weathered scoria, palagonite fragments and iddingsite as coarse material. Minerals present are palagonite (mixture of minerals) and hematite. Pedofeatures include gibbsite infillings in the weathered scoria fragments (Figure 3F) as well as few clay infillings (mainly kaolinite, Table 1) and Fe-oxide nodules. The yellowish-brown micromass has an undifferentiated b-fabric and the A horizon contains some fresh roots.
The macro-porosity of the thin sections apparently changes with the elevation of the soil profiles (Supplementary Figure S2). On lava, there seems to be a strong increase in porosity from the arid to the very humid location (E1: 8%, E3: 15%, E5: 44%), while on scoria porosity does not change much (E2: 11%, E4: 9%, E6: 8%; Supplementary Figure S1). At E5, however, disruption during sampling cannot be completely ruled out due to the high soil skeleton content of the soil. Thus, the extraction of undisturbed soil samples in Kubiena boxes at site E5 was difficult. This could partly explain the very high porosity value. The average bulk densities of the horizons investigated here are approx. 0.66 g cm−3 for the sites on lava and 0.99 g cm−3 for the sites on scoria (Candra et al., 2023). In both cases, the very humid sites have the lowest bulk densities (E5: 0.49 g cm−3, E6: 0.62 g cm−3). In relative terms, more bio-pores (channels and chambers) can be observed in profiles E1 and E2 and more pores due to physical weathering in the more strongly weathered profiles E5 and E6 (Supplementary Figure S1).
Phosphorus Anomaly at Site E1
At the arid lava site (E1), an anomaly with regard to the mobile soil phosphorus content has been detected by (Rechberger et al., 2021). In the A horizon of that soil, Mehlich-3-extractable P was found at a concentration at least 20-fold higher than in the other soils [Mehlich-3-extractable-P in mg kg−1: E1: 1,007 ± 266 (a), E2: 50.4 ± 18.4 (b), E3: 14.8 ± 1.92 (b), E4: 3.76 ± 1.08 (b), E5: 11.3 ± 3.75 (b), E6: 6.85 ± 0.91 (b); different letters in parentheses indicate significant difference; Tukey HSD (Rechberger et al., 2021)]. The phosphate sorption capacity in E1 was the lowest of all sites in the elevation sequence, and the degree of P saturation reached 344% ± 87% at this site (Rechberger et al., 2021). In the thin sections of E1 we found large quantities of tortoise egg shells and also some snail shells, which were identified in the field. In both cases, we recorded element transects using EDX - line scans (Figure 4). The dominant elements of the soil matrix were Si and Al, with smaller amounts of Ca, Na, K, Fe, Mg and P. Ca was the dominant element in both investigated shell regions. Phosphorus was detectable in both transects (Figure 4) but showed no increase in the respective shells. The presence of phosphate (apatite) in the shells, therefore, seems unlikely. In the snail shell transect, EDX detected a sharp Fe peak indicating the presence of an Fe nodule.
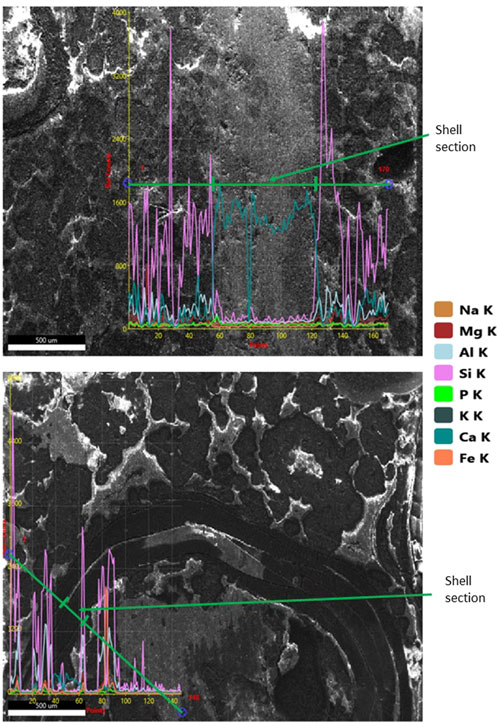
Figure 4. Electron micrograph (magnification: 999x) with EDX line-scans across a tortoise eggshell (top) and a snail shell (bottom) in uncovered thin sections of the topsoil at site E1 (lava). Straight green line indicates the location of the analyzed cross-sections.
Discussion
Micromorphology and Soil Development
Comparing the investigated profiles with the micromorphological units defined by (Stoops, 2013) for the soils on the windward side of Santa Cruz Island, there seems to be quite good agreement regarding their characteristics and location (see Supplementary Table S1). According to (Stoops, 2013), both units (E1 and E2) tend to be influenced by colluvial material due to the absence of weathered coarse fraction. We observed slight to no weathering of primary minerals in these profiles (Table 3). Yet, for site E2, the deposition of colluvial material is unlikely due to its geomorphic position on the backslope of a cinder cone and the high permeability of the scoriaceous parent material. The situation is very different for site E1, which is located in a soil patch amidst outcropping lava from an a’a flow. This represents more of a receiving geomorphic position and the underlying lava a greater barrier for water seepage. Hence, water and material may episodically accumulate at this site. In the humid zone, the weathering status of plagioclases and olivines at site E3 is similar to that of E1 (Table 3). However, in contrast to the respective unit defined by (Stoops, 2013) (Supplementary Table S1), we found vermiculite, but no gibbsite in this profile. In E4, we found gibbsite in line with the description of (Stoops, 2013) albeit only in traces. This unit should be derived from total weathering of hard mesocrystalline scoria (Stoops, 2013). In the very humid zone, the profile E5, according to (Stoops, 2013), could have been influenced by volcanic ash input as indicated by the low coarse fraction.
Table 2 summarizes the main differences between the soils formed on scoria vs. those formed on lava in the three climatic zones. The proportion of coarse components (>2 mm) is consistently greater in the soils on lava than on scoria, with the differences being particularly pronounced under humid and very humid climatic conditions. Conversely, the coarse/fine ratio differs less between sites on different parent materials. The soil organic matter content is consistently higher in the soils on lava than on scoria, with the difference being particularly pronounced in the arid zone. It is remarkable that in this dry climate, the primary minerals in the topsoil on lava show greater weathering than on scoria (Figure 5; Table 3). In the latter, we found almost unaltered plagioclase and olivine. By contrast, in the humid climate zone, plagioclase is no longer found in the soils on scoria, but only more strongly weathered olivine, palagonite and iddingsite, a mixture of clay minerals and iron oxides that emerge from olivine through weathering (Smith et al., 1987). The observed mineral assemblage and their weathering status suggest that in the humid climate zone, the soils on scoria are overall more strongly weathered than those on lava. In the very humid climate zone, the differences in weathering of minerals are smaller between the lava and scoria sites (Table 3). However, the previous clay mineralogical findings (Candra et al., 2023) underscore a higher degree of weathering, notably the substantial gibbsite content in E6 (scoria, very humid) compared to E5 (lava, very humid, Table 2). Also, the 2:1 clay minerals in the lava profile of the humid zone (E3) indicate less weathering than the dominant 1:1 clay minerals of the soil on scoria (E4, Tables 1, 2). Already (Morrás, 1976) described the new formation of gibbsite in more strongly weathered soils of Santa Cruz Island. Bhattacharyya et al. (1993) investigated two red-soil pedons and one black-soil pedon from the Bhimashankar plateau (India) at an elevation of 1,000 m asl, with a humid (>5,000 mm rainfall) tropical climate. Three additional pedons of black soils were from the semi-arid zone (500–1,000 mm rainfall), at an elevation of 800–900 m asl. The study showed differences in the clay mineralogy of soils formed on basalt under humid and more arid conditions. In our case, it is not the different levels of precipitation that makes the difference for profiles in the same climatic zone, but the porosity, especially the inter-particle porosity, and the crystallinity of the parent material. The higher inter-particle porosity of the scorias leads to a larger area of exposed surfaces [by a factor of approx. 7; (Paque et al., 2024)] and a faster removal of weathering products as compared to the denser lavas. Sowards et al. (2018) demonstrated the influence of permeability on water movement in volcanic materials and showed increased weathering in more permeable lavas. Additionally, the higher amorphous content of the scorias makes them more weatherable compared to the more crystalline lavas. Volcanic glass is thermodynamically unstable and decomposes more easily than almost all associated mineral phases (Fisher et al., 1984).
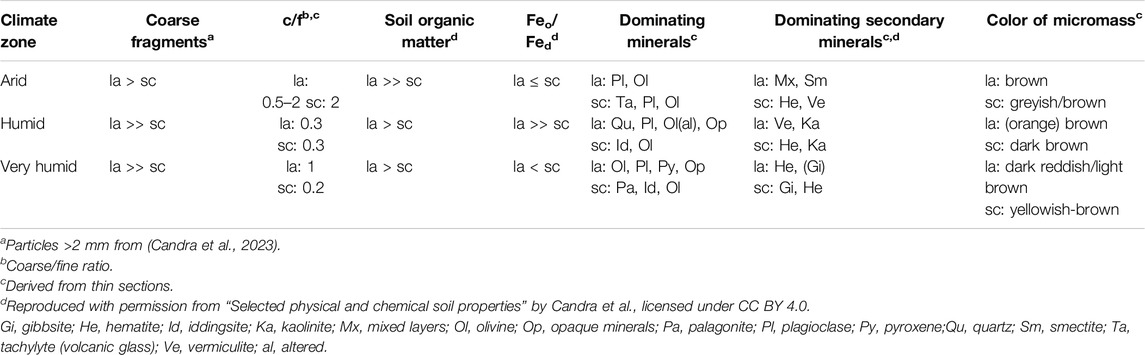
Table 2. Overview of major differences between soils of the elevation sequence on Santa Cruz developed on lava (la) vs. scoria (sc) in the same climate zone.
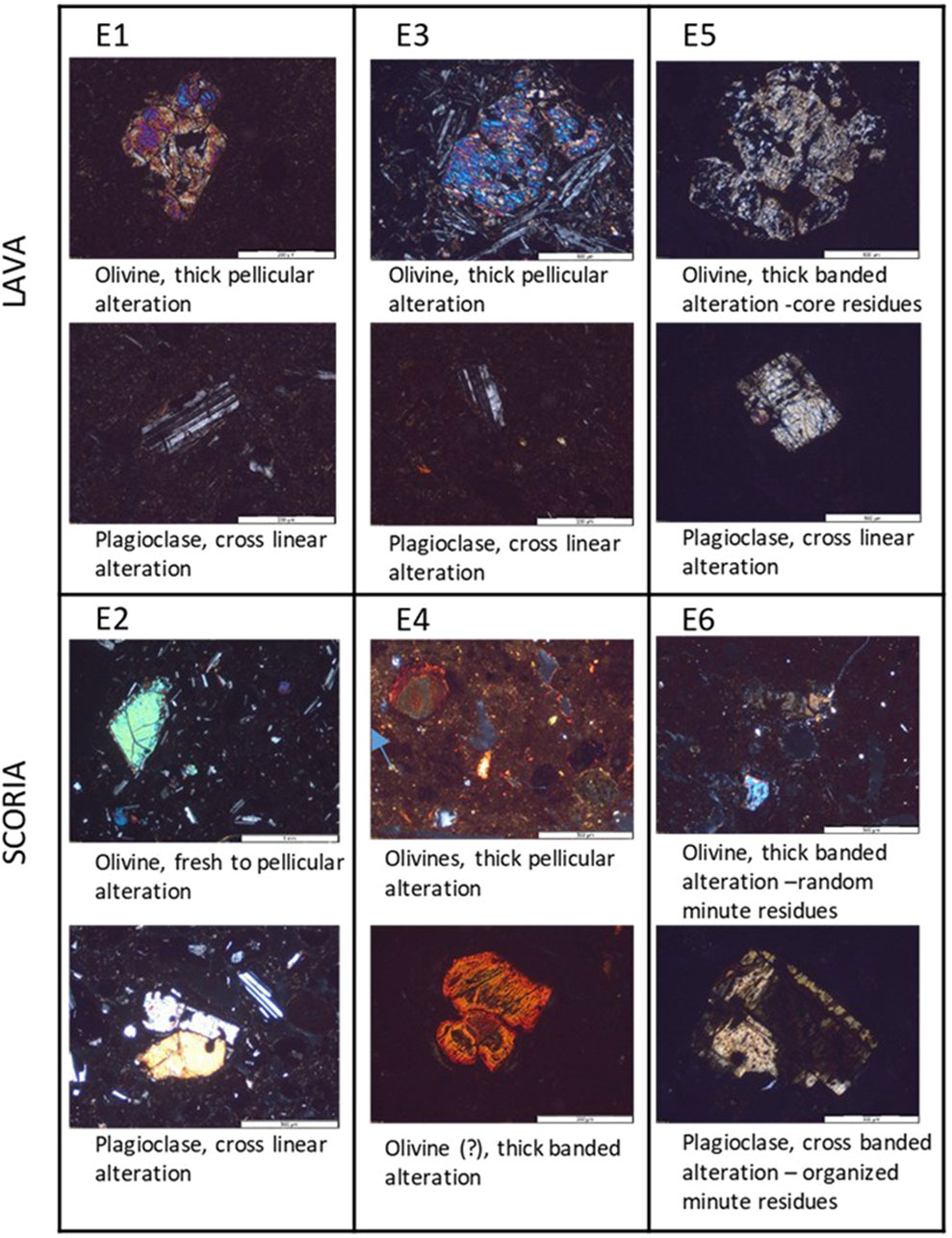
Figure 5. Examples of mineral grains in soils on lava (upper rows) and scoria (lower rows) for the 6 profiles on lava and scoria. The description of the weathering degree was performed according to the method published by [stoops, 2021].

Table 3. Comparison of the weathering degree of dominant primary minerals derived from the analysis of thin sections. 0: not altered, 4: heavily weathered. The figures presented do not refer to abundances. Visual examples are shown in Figure 5.
A peculiarity we are observing is that in the arid climate zone, the soil on lava (E1) seems considerably more developed - this was confirmed by three replicate profiles - than that on scoria (E2). The plagioclase and olivine minerals are more weathered in the A-horizon on the lava site (E1, Table 3), clay content is higher and the profile deeper with a B-horizon formed. The lava sites differ from the scoria sites not only in rock porosity but also in their geomorphology and micro-topography. While the scoria deposits form a continuous mantle over cinder cones and their surroundings, the lavas show irregular flow patterns with large rock outcrop sections and small depression patches, in which soils have formed. It is conceivable that due to this specific geomorphic location, site E1 received inputs of more weathered colluvial material, as suggested by (Stoops, 2013) for similar sites. Another possible reason for the more pronounced soil development at E1 could be the soil water dynamics. While scoria drains away rainwater immediately due to its enormous macropore volume, the lavas, exhibiting much fewer and finer pores (Paque et al., 2024), lower permeability and higher water holding capacity, could retain more rainwater and thus provide the vegetation with more plant-available water. This could play an important role, especially at the beginning of soil formation, promoting faster colonization and establishment of a plant cover in the arid zone. Paque et al. (2024) reports a porosity of 45.0% ± 10.6% for the scorias and 23.0% ± 5.6% for the lavas of the studied elevation sequence. This and the presence of (highly conductive) inter-particle pores in the scoria deposits likely results in rapid and excessive drainage of the scoria soils. Additionally, the organic matter content in the A horizon of the profile on lava, which is about a factor 5 higher than that on scoria (Table 1), is a strong indication of a considerably higher net primary production at the lava site (Noe et al., 2019) and resulting in a larger soil volume. Nevertheless, the observed greater profile thickness at E1 might only partly be explained by faster in situ soil development compared to E2. We deem it likely that a combination of soil formation and aggradation is the cause. Soil formation at site E1 is very patchy (see Supplementary Figure S1). This means that the soil fine material is concentrated in smaller areas between lava boulders. The influence of wind and water likely led to the deposition of fine material in such patches (Stoops, 2013).
In the humid and very humid zones, the soils formed on lava are considerably less developed (evidenced by less weathering of primary minerals; Table 3), but also much shallower than those on scoria (Candra et al., 2023). Besides the above-mentioned differences in inter-particle porosity and crystallinity and the resulting weatherability of the two parent material types, the observed trend could, in part, be also caused by soil erosion processes due to less permeable underlying rock strata and potentially amplified by the patchy nature of the lava sites. Indeed, the discontinuity observed at the top horizon (3–11 cm) of this profile, shown by a clay intercalation, points to a sedimentation crust after an erosion episode (Supplementary Table S1; Supplementary Figure S3). The solum thickness at the lava sites also varies inconsistently with elevation/humidity (and soil age). The older high-elevation profile on lava, E5 (145 ± 52 ka), is shallower than the younger mid-elevation lava profile, E3 (44.6 ± 24.5 ka; Table 1). In case of site E5, the input of volcanic ash, assumed by (Stoops, 2013) into the Brown clayey isotropic basaltic granular Unit, may have temporarily damaged the vegetation and promoted erosion. In fact, in a profile located very close to E5 (819 m asl), we found a buried soil with an overlying ash layer (Supplementary Figure S4). Numerous pieces of charcoal were visible in the thin sections of that soil, witnessing intensive wildfires.
The lower proportion of pores in the very humid locations compared to the arid locations are probably due to higher clay content of the more weathered (very) humid soils and their lower pH values (Table 1). The latter reduce the biological activity in the soils, the former promote physical processes of aggregation. Alvarez et al. (2021) were able to establish a close inverse connection between the proportion of biopores and the bulk density of Luvic Phaeozems in Argentina. However, this seems not to be the case with our soils developed on volcanic materials. The lowest bulk densities along the studied elevation-sequence were found in profiles E5 and E6, which is likely due to the preferential formation of short-range order minerals in these soils (Candra et al., 2023). Additionally, the very high proportions of Fe- and Al-oxides (Candra et al., 2023) could have led to very strong micro-aggregation, the so-called pseudo-sand formation, which we detected earlier also in the oldest chronosequence soils (Gerzabek et al., 2022) and is triggered by mineral-mineral interactions of Fe and Al oxides as well as kaolinite (Martinez and Souza, 2020). The pseudo-sand formation could reduce the accessibility of organic matter for soil organisms by occlusion in microaggregates (Martinez and Souza, 2020), or even the occlusion of microaggregates in very stable macroaggregates (Huber et al., 2023). A subsequent reduction of the biological activity is likely.
Phosphorus Anomaly at Site E1 (Soil Patch Amidst a’a Lava)
The extremely high Mehlich-3-extractable phosphorus content in the topsoil of E1, which is 20–270 times that found in the other soils of the elevation sequence (Rechberger et al., 2021), is most likely of biological origin. Based on the micromorphology and mineral analyses, no P-containing minerals could be detected. The tortoise eggshells, which are abundant in several places at site E1, suggest a possible influence of the Galápagos giant tortoises on the P status of the soil. However, we did not find increased P contents in the eggshells themselves compared to the immediately surrounding soil matrix (see transects in Figure 4). Tortoises are known to release excrements into the nesting cavity while laying their eggs, and this could represent a substantial inflow of P into nesting areas. Liesegang et al. (2001) reported P contents in the feces of juvenile Galápagos tortoises of 0.07%–0.28% (in fresh matter). Subaluski and Ellies-Soto (2024) found significantly elevated nutrient concentrations in ponds used by Galápagos tortoises. In a comprehensive review article, (Ellis-Soto et al., 2021) emphasize the importance of giant tortoises’ migrations for the transport and distribution of nutrients on Santa Cruz Island. Site E1 is located in the national park area and may not be entered by residents or tourists. Besides, it is very difficult to access through a tangle of thorn bushes. Direct human influence can therefore be ruled out. It is also unlikely that the site has experienced exceptionally high P inputs from seabirds, because it is and has been relatively far inland and is unsuitable for colony-forming seabirds. It therefore seems possible that the observed phosphorus anomaly at site E1 is due to tortoise nesting activities.
Conclusions
In Candra et al. (2023), we had reported strongly diverging pedogenic development on lava vs. scoria along an elevation-sequence on Santa Cruz Island, Galápagos, and in (Rechberger et al., 2021), we detected unusually high soil phosphorus levels at the lava site in the low-elevation arid zone of that sequence. Here, micromorphological methods combined with electron microscopy and microanalyses enabled us to unravel the formerly observed discrepancies and anomalies.
The differences in soil formation on lava vs. scoria appear to be caused by a combination of (1) different rates of weathering and soil development due mainly to differences in the parent materials’ porosity and (2) erosion and aggradation processes. The former processes render scoria deposits more weatherable than lava flows given sufficient water supply, i.e., under humid conditions, but strongly limit soil development under arid conditions. The latter processes modulate pedogenesis especially at lava sites, whose topography is inherently more heterogeneous (patchy) and conducive to lateral relocation of water and soil material. We saw that this may result in enhanced soil build-up under arid conditions but strongly reduced soil formation under very humid conditions on lava compared to scoria.
Our analyses suggest that the phosphorus anomaly at the arid lava site was possibly linked to tortoise nesting activities, as evidenced by eggshell fragments encountered in soil thin sections and found at several locations of E1. This shows that the migration of giant tortoises and the associated transport of nutrients between different tortoise habitats may have significant implications on nutrient cycling at the landscape level. The influence of giant tortoises, and more generally of large herbivores, on soil development appears to be an interesting (but largely neglected) research topic that needs to be tackled for a more holistic understanding of ecosystems and their changes in the future. Overall, the closer examinations of this study highlight the complex interplay of several factors (in our case: parent material, geomorphology, climate and also the activities of large animal species) in soil formation and ecosystem evolution.
Data Availability Statement
The original contributions presented in the study are included in the article/Supplementary Material, further inquiries can be directed to the corresponding author.
Author Contributions
Authors MG and FZ designed the experiments and took the samples; authors RP, DA, and MG contributed to the description of thin-sections. LP performed the electron microscopical analysis. MG wrote the first draft of the manuscript. All authors contributed to the article and approved the submitted version.
Funding
The author(s) declare that financial support was received for the research and/or publication of this article. The study was supported by the Prometeo Project of Ecuador’s Secretariat of Higher Education, Science, Technology and Innovation (SENESCYT). FZ was supported through this grant.
Conflict of Interest
The authors declare that the research was conducted in the absence of any commercial or financial relationships that could be construed as a potential conflict of interest.
Generative AI Statement
The author(s) declare that no Generative AI was used in the creation of this manuscript.
Acknowledgments
This study was conducted under research permit no. PC-60-16 of the Galápagos National Park Directorate (GNPD). We wish to thank the GNPD for their help, especially Jorge Carrión, the former director, for supporting and facilitating our research in Galápagos. A big thank you to the National Park rangers and the technicians of the Ecuadorian Ministry of Agriculture and Livestock (MAG) for their assistance in the field, as well as the Charles Darwin Foundation for logistic support in Galápagos.
Supplementary Material
The Supplementary Material for this article can be found online at: https://www.frontierspartnerships.org/articles/10.3389/sjss.2025.14219/full#supplementary-material
References
Alvarez, M. F., Poch, R. M., and Osterrieth, M. (2021). Bioporosity in Thin Sections of Luvic Phaeozems of Southeast of Buenos Aires, Argentina, and its Possible Role as an Indicator of Soil Quality. An Micromorphological Approach. Eurasian Soil Sci. 54, 918–926. doi:10.1134/s1064229321060028
Bhattacharyya, T., Pal, D. K., and Deshpande, S. B. (1993). Genesis and Transformation of Minerals in the Formation of Red (Alfisols) and Black (Inceptisols and Vertisols) Soils on Deccan Basalt in the Western Ghats, India. Soil Sci. 44, 159–171. doi:10.1111/j.1365-2389.1993.tb00442.x
Bowman, B. (1963). Evolutionary Patterns in Darwin Finches, Galápagos Islands. A Unique Area for Scientific Investigations. Occas. Pap. Calif. Acad. Sci. 44, 107–140.
Candra, I. N., Gerzabek, M. H., Ottner, F., Tintner, J., Wriessnig, K., and Zehetner, F. (2019). Weathering and Soil Formation in Rhyolitic Tephra along a Moisture Gradient on Alcedo Volcano, Galápagos. Geoderma 343, 215–225. doi:10.1016/j.geoderma.2019.01.051
Candra, I. N., Gerzabek, M. H., Ottner, F., Wriessnig, K., Tintner, J., Schmidt, G., et al. (2023). Soil Formation and Mineralogical Changes on Basaltic Lava vs Scoria along a Hydroclimatic Gradient on Santa Cruz Island, Galápagos. Catena 220, 106696. doi:10.1016/j.catena.2022.106696
Candra, I. N., Gerzabek, M. H., Ottner, F., Wriessnig, K., Tintner, J., Schmidt, G., et al. (2021). Soil Development and Mineral Transformations along a One-million-year Chronosequence on the Galápagos Islands. Soil Sci. Soc. Am. J. 85, 2077–2099. doi:10.1002/saj2.20317
Dokuchaev, V. V. (1886). “Materials on Land Evaluation of the Nizhni Novgorod Governorate. Natural and Historical Part: Report to the Nizhni Novgorod Governorate Zemstvo,” in Key Points in the History of Land Evaluation in the European Russia, with Classification of Russian Soils (Russia: Tipogr. Evdokimova, St. Petersburg), 1. [in Russian].
Ellis-Soto, D., Ferraro, K. M., Rizzuto, M., Briggs, E., Monk, J. D., and Schmitz, O. (2021). A Methodological Roadmap to Quantify Animal-Vectored Spatial Ecosystem Subsidies. J. Anim. Ecol. 90, 1605–1622. doi:10.1111/1365-2656.13538
Eswaran, H., Stoops, G., and De Paepe, P. (1973). A Contribution to the Study of Soil Formation on Isla Santa Cruz, Galápagos. Pedologie 23, 100–122.
Fisher, R. V., and Schmincke, H.-U. (1984). Alteration of Volcanic Glass. In: R. V. Fisher, and H.-U. Schmincke, editor. Pyroclastic Rocks. (Berlin, Heidelberg, Germany: Springer), 312–345.
Gerzabek, M. H., Rechberger, M. V., Schmidt, G., Wriessnig, K., and Zehetner, F. (2022). Soil Organic Carbon and Fine Particle Stocks along a Volcanic Chrono- and Elevation-Sequence on the Galápagos Archipelago/Ecuador. Geoderma Reg. 29, e00508. doi:10.1016/j.geodrs.2022.e00508
Gerzabek, M. H., Stoops, G., Ottner, F., Wang, S.-L., Huang, L.-S., and Zehetner, F. (2023). The Evolution of Soil Microstructure and Micromineralogy along a Soil Age Gradient on the Galápagos Islands (Ecuador). Geoderma Reg. 32, e00609. doi:10.1016/j.geodrs.2023.e00609
Guillod, B. P., Davin, E. L., Kündig, C., Smiatek, G., and Seneviratne, S. I. (2013). Impact of Soil Map Specifications for European Climate Simulations. Clim. Dyn. 40 (1-2), 123–141. doi:10.1007/s00382-012-1395-z
Huber, M. E., Yavitt, J. B., and Wright, S. J. (2023). Mineralogical Control on Physically Protected Soil Organic Matter in a Neotropical Moist Forest. Soil Res. 61, 569–581. doi:10.1071/sr22265
IUSS Working Group WRB (2022). World Reference Base for Soil Resources. International Soil Classification System for Naming Soils and Creating Legends for Soil Maps. 4th ed. Vienna, Austria: International Union of Soil Sciences IUSS. Available online at: https://wrb.isric.org/files/WRB_fourth_edition_2022-12-18.pdf.
Lasso, L., and Espinosa, J. (2018). Soils From the Galapagos Islands, the Soils of Ecuador. In: J. Espinosa, J. Moreno, and G. Bernal, editor. World Soils Book Series, (Cham, Switzerland: Springer International Publishing AG). 139–150.
Liesegang, A., Hatt, J.-M., Nijboer, J., Forrer, R., Wanner, M., and Isenbügel, E. (2001). Influence of Different Dietary Calcium Levels on the Digestibility of Ca, Mg, and P in Captive-born Juvenile Galapagos Giant Tortoises (Geochelone nigra). Zoo. Biol. 20, 367–374. doi:10.1002/zoo.1035
Mahmoodi, M., Khormali, F., Amini, A., and Ayoubi, S. (2016). Weathering and Soils Formation on Different Parent Materials in Golestan Province, Northern Iran. J Mt. Sci. 13/5, 870–881. doi:10.1007/s11629-015-3567-x
Martinez, P., and Souza, I. F. (2020). Genesis of Pseudo-Sand Structure in Oxisols From Brazil – A Review. Geoderma Reg. 22, e00292. doi:10.1016/j.geodrs.2020.e00292
Morrás, H. J. M. (1976). Gibbsite Glaebules of a Soil Profile from Santa Cruz Island, Galápagos (Ecuador). Pedologie 26, 91–96.
Noe, J. L., Billen, G., Mary, B., and Garnier, J. (2019). Drivers of Long-Term Carbon Dynamics in Cropland: A Bio-Political History (France, 1852–2014). Environ. Sci. & Policy 93, 53–65. doi:10.1016/j.envsci.2018.12.027
Paque, R., Alomia Herrera, I., Dixon, J., Molina, A., Zehetner, F., and Vanacker, V. (2024). Constraining the Effect of Climate and Rock Porosity on Weathering Extent in the Volcanic Island of Santa Cruz (Galápagos, Ecuador). J. Geophys. Res. Earth Surf. 129, e2024JF007651. doi:10.1029/2024JF007651
Rechberger, M. V., Zehetner, F., and Gerzabek, M. H. (2021). Phosphate Sorption-desorption Properties in Volcanic Topsoils along a Chronosequence and a Climatic Gradient on the Galápagos Islands. J. Plant Nutr. Soil Sci. 184, 479–491. doi:10.1002/jpln.202000488
Sauzet, O., Cammas, C., Gilliot, J., Bajard, M., and Montagne, D. (2017). Development of a Novel Image Analysis Procedure to Quantify Biological Porosity and Illuvial Clay in Large Soil Thin Sections. Geoderma 292, 135–148. doi:10.1016/j.geoderma.2017.01.004
Sion, B. D., Harrison, B. J., McDonald, E. V., Phillips, F. M., and Axen, G. J. (2022). Chronofunctions for New Mexico, USA Soils Show Relationships Among Climate, Dust Input, and Soil Development. Quat. Int. 618, 35–51. doi:10.1016/j.quaint.2021.06.024
Smith, K. L., Milnes, A. R., and Eggleton, R. A. (1987). Weathering of Basalt – Formation of Iddingsite. Clays clay minerals 35, 418–428. doi:10.1346/ccmn.1987.0350602
Sowards, K. F., Nelson, S. T., McBride, J. H., Bickmore, B. R., Heizler, M. T., Tingey, D. D., et al. (2018). A Conceptual Model for the Rapid Weathering of Tropical Ocean Islands: A Synthesis of Geochemistry and Geophysics, Kohala Peninsula, Hawaii, USA. Geosphere 14, 1324–1342. doi:10.1130/GES01642.1
Stoops, G. (2003). Guidelines for Analysis and Description of Soil and Regolith Thin Sections. Madison, Wisconsin: Soil Science Society of America, 184.
Stoops, G. (2013). A Micromorphological Evaluation of Pedogenesis on Isla Santa Cruz (Galápagos). Span. J. Soil Sci. 3, 14–37. doi:10.3232/sjss.2013.v3.n2.02
Stoops, G. (2021). Guidelines for the Analysis and Description of Soil and Regolith Thin Sections. 2nd ed. Madison, WI: Wiley and SSSA, 256.
Stoops, G., Mees, F., Dumon, M., Adhikari, R., and Van, R. (2023). Soils of the Coastal and Lower Belt of Galápagos Islands – The Case of Isla Santa Fé and Isla Santa Cruz. Geoderma Reg. 29, e00520. doi:10.1016/j.geodrs.2022.e00520
Taboada, T., Ferro-Vázquez, C., Stoops, G., Martínez-Cortizas, A., Rodríguez-Flores, R., and Rodríguez-Lado, L. (2019). Secondary Aluminium, Iron and Silica Phases across a Volcanic Soil Climosequence, Galápagos Islands. Eur. J. Soil Sci. 70, 540–549. doi:10.1111/ejss.12788
Taboada, T., Rodríguez Lado, L., Ferro Vázquez, C., Stoops, G., and Martínez-Cortízas, A. (2016). Chemical Weathering in the Volcanic Soils of Isla Santa Cruz (Galápagos Islands, Ecuador). Geoderma 261, 160–168. doi:10.1016/j.geoderma.2015.07.019
Torres-Guerrero, C. A., Álvarez, D., Preusser, F., Olarieta, J. R., and Poch, R. M. (2023). Evolution of Soil Porosity in Loess-Palaeosol Sequences of the Ebro Valley, NE Iberia. Catena 230, 107244. doi:10.1016/j.catena.2023.107244
Trueman, M., and d'Ozouville, N. (2010). Characterizing the Galapagos Terrestrial Climate in the Face of Global Climate Change. Gal. Res. 67, 26–37.
Wardle, D. A., Walker, L. R., and Bardgett, R. D. (2004). Ecosystem Properties and Forest Decline in Contrasting Long-Term Chronosequences. Science 305, 509–513. doi:10.1126/science.1098778
White, W. M., McBirney, A. R., and Duncan, R. A. (1993). Petrology and Geochemistry of the Galápagos Islands: Portrait of a Pathological Mantle Plume. J. Geophys. Res. 98, 19533–19563. doi:10.1029/93jb02018
Zehetner, F., Gerzabek, M. H., Shellnutt, J. G., Chen, P.-H., Candra, I. N., Huang, K.-F., et al. (2024). Chemical Weathering along a One-Million-Year Soil Age Gradient on the Galápagos Islands. Geochimica Cosmochimica Acta 371, 95–110. doi:10.1016/j.gca.2024.02.021
Keywords: andosol, cambisol, phaeozem, precipitation gradient, regosol
Citation: Gerzabek MH, Poch RM, Álvarez D, Ploszczanski L and Zehetner F (2025) Strong Differences in Pedogenesis on Lava Vs. Scoria Along a Hydroclimatic Gradient on Santa Cruz Island/Galápagos Archipelago (Ecuador) – Insights From Micromorphology. Span. J. Soil Sci. 15:14219. doi: 10.3389/sjss.2025.14219
Received: 16 December 2024; Accepted: 14 March 2025;
Published: 07 April 2025.
Edited by:
Avelino Núñez-Delgado, University of Santiago de Compostela, SpainCopyright © 2025 Gerzabek, Poch, Álvarez, Ploszczanski and Zehetner. This is an open-access article distributed under the terms of the Creative Commons Attribution License (CC BY). The use, distribution or reproduction in other forums is permitted, provided the original author(s) and the copyright owner(s) are credited and that the original publication in this journal is cited, in accordance with accepted academic practice. No use, distribution or reproduction is permitted which does not comply with these terms.
*Correspondence: Rosa M. Poch, cm9zYS5wb2NoQHVkbC5jYXQ=
†ORCID: Martin H. Gerzabek, orcid.org/0000-0002-3307-8416; Rosa M. Poch, orcid.org/0000-0001-8639-4204; Leon Ploszczanski, orcid.org/0000-0002-6052-4610; Franz Zehetner, orcid.org/0000-0002-8848-9650