- 1Department of Surgery, School of Medicine, University of Maryland, Baltimore, MD, United States
- 2Revivicor Inc., Blacksburg, VA, United States
- 3National Heart, Lung, and Blood Institute, National Institute of Health, Bethesda, MD, United States
Survival of pig cardiac xenografts in a non-human primate (NHP) model has improved significantly over the last 4 years with the introduction of costimulation blockade based immunosuppression (IS) and genetically engineered (GE) pig donors. The longest survival of a cardiac xenograft in the heterotopic (HHTx) position was almost 3 years and only rejected when IS was stopped. Recent reports of cardiac xenograft survival in a life-sustaining orthotopic (OHTx) position for 6 months is a significant step forward. Despite these achievements, there are still several barriers to the clinical success of xenotransplantation (XTx). This includes the possible transmission of porcine pathogens with pig donors and continued xenograft growth after XTx. Both these concerns, and issues with additional incompatibilities, have been addressed recently with the genetic modification of pigs. This review discusses the spectrum of issues related to cardiac xenotransplantation, recent progress in preclinical models, and its feasibility for clinical translation.
Introduction
Xenotransplantation (XTx) is an alternative source of a human organ for patients with end-stage organ failure. Many of these patients will die waiting for a human organ, as the current availability of donor organs falls short of its demand. In the past few years, substantial progress has been made in the xenotransplantation field. With the discovery and use of novel molecular biology techniques, genetically engineered (GE) porcine organ donors have been created to overcome numerous XTx barriers. The first transgenic pig for XTx was produced expressing human complement regulatory protein (hCRP) decay acceleration factor (hDAF). Organs from these pigs were transplanted in non-human primate (NHP), but hyperacute rejection (HAR) was only partially avoided (1, 2), and antibody-mediated immune response induced to terminal galactose sugar molecules (α1-3 Galactose or Gal) expressed on graft vascular endothelial cells continued to cause HAR. By using gene-editing techniques, Gal antigen was knocked out in pigs, and organs from these pigs were protected from HAR (3–5).
Other combinations of antigen knockout and human transgene expressing GE pigs were produced, and xenograft survival was extended further (6–13). We (HHTx Heart) and others (Kidney, Liver) have also reported long-term xenograft survival in NHP from genetically modified pigs (10, 12, 14–17). Recently, Langin et al. reported consistent survival in an experimental life-supporting (OHTx) in NHP (18). Strategies which have helped to achieve this success have also been summarized in Figure 1. In this review, we discuss the challenges faced in cardiac xenotransplantation and solutions that have culminated from the last several decades of work and speculate on the next steps required to make cardiac XTx a clinical reality (19, 20).
Challenges for Cardiac Xenotransplantation
Immunological
Preformed Natural and Elicited Antibodies
The presence of natural preformed antibodies (nAbs) against pig antigens in recipients is a primary and significant hurdle for the success of cardiac XTx. These antibodies trigger immune responses and causes hyperacute (HAR) and acute humoral xenograft rejection (AHXR) (21). These nAbs against donor antigens (xenoantigens) trigger the activation of complement proteins, which further cause activation and damage to endothelial cells, leading to platelet aggregation and microvascular thrombosis. This ischemic injury leads to the destruction of cardiomyocytes, interstitial hemorrhage, and eventually fibrosis. Most of nAbs are against porcine carbohydrate antigens not found in humans and NHP. The most predominant of these is Galactose-α1-3 galactose, due to the acquired mutation of α1-3 galactosyltransferase (GT), an enzyme responsible for synthesizing this carbohydrate antigen. Others include SDa, and N-glycolylneuraminic acid (Neu5Gc). While preformed antibody responses dominate Gal antigens, it has been shown that elicited Abs responses can occur in cardiac XTx also towards these other antigens (i.e., non-Gal antigens) (22, 23, 24–30). Elicited Abs also play a major role in posttransplant thrombotic microangiopathy (TM), consumptive coagulopathy (CC), and AHXR (10, 31–34).
Cellular Xenograft Rejection
Besides HAR and AHXR, acute cellular rejection of cardiac xenografts can be mediated by innate (i.e., macrophages, neutrophils, dendritic cells, and NK cells) and adaptive (i.e., T and B cells) immune responses (35–37). However, acute CXR has not been reported frequently in xenotransplantation (34, 38). Innate immune cells, like macrophages and NK cells, have been found in pig organs perfused with human blood ex vivo and in pig-to-NHP xenografts, which may trigger CXR (34). Macrophages may also be activated by xenoreactive T cells and release proinflammatory cytokines (e.g., tumor necrosis factor-alpha (TNF-α, IL-1, and IL-6), which can further stimulate T cells. Both macrophages and NK cells can also be activated by direct interaction between donor endothelial antigens and their surface receptors, which may trigger CXR by direct NK cytotoxicity or antibody-dependent cellular cytotoxicity (ADCC) (39, 40).
T cells can be activated through both direct and indirect pathways after xenotransplantation. However, the responses against xenoantigens, especially indirect responses, are more robust than seen in allotransplantation (41). T cell activation requires interaction between TCR and MHC peptide complex from the antigen-presenting cells (APC) and a costimulatory signal (e.g., CD40–CD154 and CD28–CD80/86 pathway interactions) (42, 43).
Coagulation Dysfunction
Coagulation dysregulation is also another major impediment to the success of xenotransplantation. The most extreme manifestations of it are systemic consumptive coagulopathy, characterized by thrombocytopenia and bleeding, which ultimately leads to graft loss due to ischemia from thrombotic microangiopathy (TM). Coagulation is a complex pathway that involves interactions of inflammation, vascular injury, heightened innate, humoral, and cellular immune responses. Incompatibilities between primate and pig coagulation/anti-coagulation factors can alter their function, contributing to coagulation dysfunction (44, 46). Notable proteins with cross-species incompatibilities include tissue factor pathway inhibitor (TFPI), thrombin, thrombomodulin (TBM), endothelial protein C receptor (EPCR) and CD39 (45–47).
Complement is also able to activate the clotting cascade, as it can be activated by the binding of complement fixing antibodies onto endothelium. As an example, activated product of complement C5a has been reported to induce tissue factor (TF) activity in endothelial cells (48) and has been reported to modifying the balance between pro- and anti-coagulation (49).
Preformed and elicited antibodies promote coagulation by activating porcine endothelial cells and platelets and contribute to graft loss due to TM (50–52). Systemic inflammatory responses and proinflammatory cytokines (notably IL-6) also upregulate or recruit recipient tissue factors (TF) on platelets and monocytes by interacting with porcine vascular endothelial cells which can lead to coagulation through thrombin production (54, 55).
Viral Transmission
A potential problem for cardiac xenotransplantation is a zoonotic viral transmission from swine. Most notable of which is a porcine endogenous retrovirus (PERV). There is no report yet for in-vivo pig-to-human PERVs transmission so the true risk in the context of xenotransplantation is not known (56). But, in-vitro studies have shown that PERVs could be transmitted from pig cells to human cells (57). Provirus DNAs of PERVs can be genetically transferred to offspring and cannot be eliminated by specified pathogen-free (SPF) breeding. Like other retrovirus, PERV theoretically predispose to the risks of tumors, leukemia, and neurodegeneration (58). However, studies have shown complete elimination of all copies of PERV in donor pigs (57).)
Porcine circovirus (PCV) from the Circoviridae family is also highly distributed among pigs and wild boars. Previously, two types of PCV1 and PCV2 have been characterized (59). PCV1, which is isolated from pig kidney cell culture (PK15 cells), and recently, Liu et al. have demonstrated that PCV2 can infect human cells in vitro with a reduced infection efficiency compared to pig PK-15 cells. Kruger et al. were unable to identify PCV1 and PCV2 in GE pigs. However, two other subtypes PCV3a and PCV3b, were found in the spleen, liver, lung kidney, and explanted heart of recipient baboons of GE cardiac xenografts after OHTx (60). The presence of PCV3 in the OHTx recipient baboon was higher among long-term survivors. However, the significance of PCV in causing clinical disease is unknown.
Xenograft Growth
Although there are several anatomical and physiological similarities between pigs and humans (or NHPs), their organs’ growth rate is significantly different (61). Therefore, the use of minipigs has been suggested as their mature growth rate is 1/3 that of wild type Yorkshire (domestic) pigs (62). However, mostly domestic pigs have been used even for genetic modifications, but organs from these GE pigs continue to grow too large (61). Therefore, juvenile GE pigs are being preferred, but even still, continued organ growth after transplantation has been reported (8, 62, 63). Längin et al. have also reported left ventricular hypertrophy after pig OHTx in NHPs, but it is unclear its origin and whether this is from rejection, physiologic mismatch or natural growth (i.e., intrinsic or extrinsic causes or a combination of both) (18). In contrast, others have not seen pig heart growth after HHTx until the xenograft underwent delayed xenograft rejection (14, 15). In these experiments heart graft size was maintained until the co stimulation pathway blockade was reversed by stopping the anti-CD40 antibody.
While the growth of other organs such as the kidney can be accommodated within the abdomen, the growth of a heart xenograft could be problematic due to its position in the non-compliant chest and must be addressed before clinical translation.
Overcoming the Challenges for Successful Cardiac Xenotransplantation
Generation of Genetically Modified Donors
Several genetic strategies have been developed to prevent early graft failure from preformed antibodies and coagulation dysfunction resulting in generation of GE pigs. Genome editing using zinc-finger nucleases, transcription activator-like effector nucleases, or CRISPR-Cas9 is being used to delete multiple genes with high precision to produce GE pigs. Several pig genes are knocked out (e.g., α1-3 galactosyltransferase, B4GALNT2 and CMAH) and human genes are overexpressed (e.g., hCD46, hTBM, hEPCR, hTFPI, hCD39, etc.) in these GE pigs (Table 1).
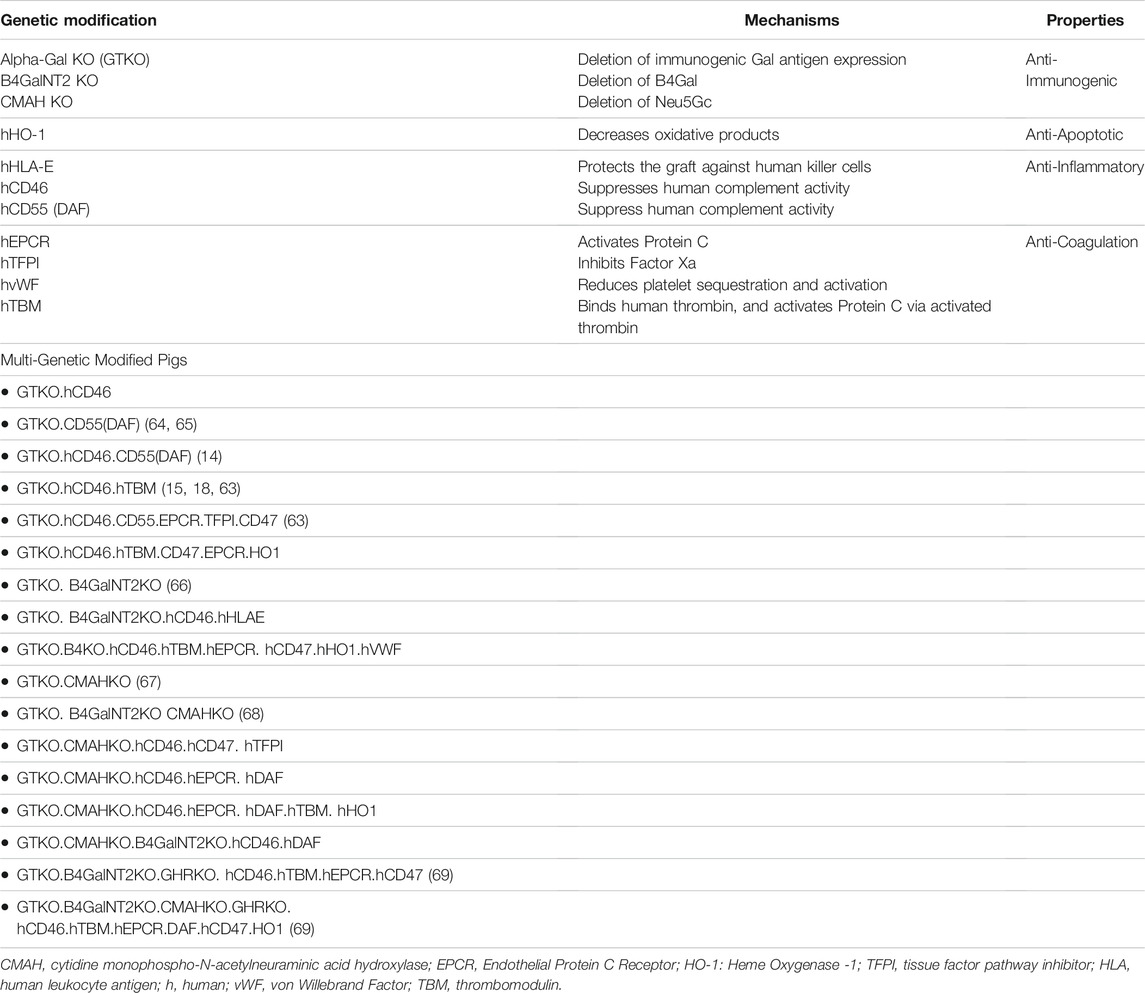
TABLE 1. The “genetic toolbox” central to our strategies to minimize or abolish hyper-acute and delayed humoral rejection.
The genetic constructs listed in Table 1, and the GE pigs produced, have been tested to various degrees. Kuwaki et al. reported the longest (179 days) heterotopic cardiac xenograft survival of GTKO hearts in NHP (6). Chen et al. also found an advantage in using GTKO pig kidneys over previously used transgenic kidneys (5). Recently, GTKO pigs along with other transgene have significantly improved the cardiac xenograft survivals in NHP to months in OHTx and years in HHTx models (14, 15, 69, 70).
CRISPR technology has now come into vogue as it affords complex genetic constructs to be employed with the highest fidelity compared to other techniques. Two carbohydrate antigen-expressing genes (e.g., GT and CMAH) have been deleted, and “double knockouts” (GTKO.CMAHKO) have been constructed (71, 72). Burlak et al. reported a reduced binding of human antibodies to cells from these GTKO. CMAH KO pigs (67). Later, Tector’s group has produced three carbohydrate antigen knockout (TKO) pigs (i.e., GTKO.CMAHKO.B4GALNT2KO), which included deletion of B4GALNT2 responsible for SDa antigen along with GT and CMAH genes (26, 31, 73). They demonstrated that the binding of human IgG and IgM antibodies to peripheral blood mononuclear cells and red blood cells from triple knockout pigs was significantly reduced. Niu et al. inactivated all known porcine endogenous retrovirus (PERVs) within pig xenograft donors (74). A combination of various genetic constructs is being developed by other groups as well, a testament to the technology’s ability to move the field forward quickly. “Multi-gene” expressing cardiac xenografts’ effect on overall graft function and survival in HHTx and OHTx is currently a topic of investigation in our lab and others.
Immunosuppression
To achieve long-term xenograft survival, various immunosuppressive (IS) drug regimens have been used along with GE pigs. Earlier conventional corticosteroids and calcineurin based (CSA) immunosuppression (IS) was used in NHP recipients, which prevented acute rejection, but failed to prolong cardiac xenograft survival (75–77). The longest reported cardiac xenograft survival using a CSA-based IS regimen was 32 days from a wild type (WT) pig (75) but was extended up to 99 days (median 26 days) using hDAF transgenic hearts (78). Various other IS regimens were used which include splenectomy or total body irradiation, non-antigenic alpha-Gal polyethylene glycol polymer (TPC) alone or in combination (9, 23, 79). Effect of these immunosuppression regimen on cardiac xenograft survival has been summarized in Table 2. Later, anti-thymocyte globulin (ATG), rituximab, mycophenolate mofetil, tacrolimus, and sirolimus were also used in various combinations as alternative regimens (10, 80–82). For complement inhibition, either cobra venom factor (CVF) or overexpression of complement regulatory protein gene expression for a donor organ or both were used (10, 15, 81). By using these IS drugs, McGregor et al. 2005 reported consistent graft survival (median 96 days; range, 15–137 days) in an HHTx model, but xenograft rejection was associated with a rise in non-Gal antibody titers. They did not observe a significant difference in graft survival when GTKO or GTKO.hCRP donors were used (35, 64).
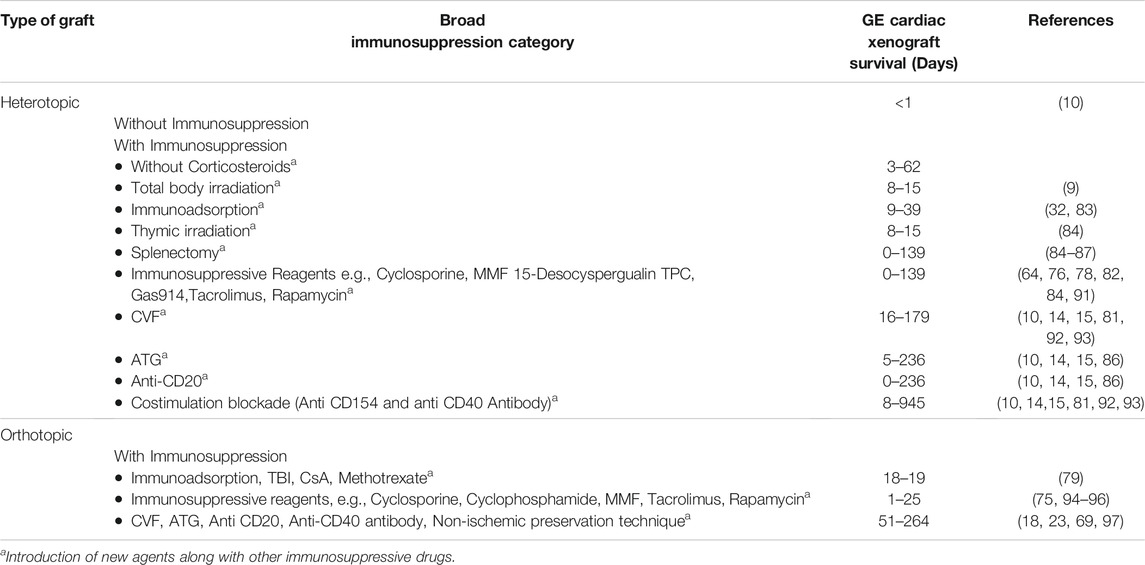
TABLE 2. Progress in Cardiac Xenograft Survival (Heterotopic and Life Supporting Orthotopic) and Immunosuppression Regimen used.
Significant progress in cardiac XTx occurred when newer agents were used that block the co-stimulation, which aids in T cell activation upon antigen exposure (10, 11, 15, 92). In 2000, Buhler et al. demonstrated that the blocking of the CD40/CD154 pathway by anti-CD154 antibody prevents an induced anti-pig humoral response (99). Kuwaki et al. also reported the longest cardiac xenograft survivals for 179 days (median 78 days) (6) in HHTx with anti-CD154 antibody treatment. We have also reported more than 8-month survival of GTKO.CD46 cardiac xenograft in HHTx with continuous co-stimulation blockade by anti-CD154 antibody (25 mg/kg; clone 5C8) and B cell depletion with Rituxan at the time of transplantation (10). Although the use of anti-CD154 antibody has improved survival, it has been reported that anti-CD154 antibody is associated with bleeding and thrombotic complications such as consumptive thrombocytopenia and venous and arterial thrombi (10, 81, 99). As a result, replacement with an anti-CD40 (25 mg/kg; clone 2C10) monoclonal antibody (mAb), which targets the same interaction, has been the focus of the active investigation. When we used this antibody, there was no significant difference found in median 70 vs. 75 days) compared to anti-CD154 blockade.
However, we demonstrated that cardiac xenograft (GTKO.CD46. TBM) survival in HHTx was significantly prolonged (median 298 days) when the anti-CD40 antibody was used at a higher dose (50 mg/kg) (15, 100). Iwase et al. also demonstrated anti-CD40 mAb combined with belatacept proved effective in preventing a T cell response (14). The anti-CD40 mAb used in these studies is a mouse/rhesus chimeric IgG4 antibody, which may not be suitable for use in humans. Still, several other humanized anti-CD40 blocking antibodies under development can be used for human use if approved as an immunosuppression adjunct in cardiac XTx (101).
Prevention of Viral Transmission
The risk of PERV transmission can be minimized by selecting PERV negative porcine donors. Thorough screening of PERV can be done by serology, western blot, ELISA, immunofluorescence, scanning electron microscopy, and PCR. Recently, Yang et al. have inactivated all PERV proviruses (62 copies of PERV’s gene pol, leading to a 1,000 times reduction in the virus’s ability to infect human cells) in the pig genome the CRISPR/Cas technique (102). The use of PERV inactivated pigs may provide tissue, organs that may address the safety issue from a porcine virus in pig-to-human xenotransplantation. However, the impact of PERV inactivation and gene editing on PERV-inactivated pigs and the necessity of these complex constructs is not known.
Prevention of Xenograft Growth
In one approach, xenograft growth is controlled by using drugs such as rapamycin (8, 14, 18). Inhibition of mTOR protein kinase has been shown to control cell growth and proliferation to treat cancers in the clinical setting (103). Längin et al have used mTOR inhibitor and anti hypertensive drugs to control the blood pressures to prevent overgrowth cardiac xenograft in OHTx (18). Recently, Hinrichs et al. have produced GHRKO pigs in order to address intrinsic organ growth. They demonstrate that GHRKO pigs have slow or reduced growth, including their organs’ growth, compared to normal wild-type pigs (61, 104–107). Recently, Goerlich, et al. have examined intrinsic and extrinsic causes of graft growth after transplantation in an OHTx model using “multi-gene” pigs growth hormone receptor knockout pigs (GHRKO) (69). Post-transplantation xenograft growth was measured by echocardiography longitudinally after transplantation between multi-gene cardiac xenografts with and without GHRKO. Extrinsic causes of graft growth, namely blood pressure and heart rate, were left without treatment. GHRKO grafts demonstrated a 50.4% increase in LV mass up to 9 months (264 days) after OHTx compared to 140.1% in xenografts with a limited survival of less than 3 months. Terminal histology demonstrated fibrosis, interstitial edema and hemorrhage as the cause of this growth and not classical hypertrophy. Moreover, blood pressures and heart rates were significantly elevated after transplantation regardless of GHRKO status, suggesting physiologic mismatch occurs after transplantation. Altogether, these data suggest that post-transplantation xenograft growth in OHTx is multifactorial; largely driven by intrinsic growth with some extrinsic component not related to physiologic mismatch. Terminal histology would suggest this extrinsic component could be rejection related.
Progress in Cardiac Xenotransplantation Toward Clinical Translation
The progress of cardiac xenotransplantation has been immense (Figure 2) but the transition from HHTx to OHTx (i.e., to the life-supporting function of xenografts) has been fraught with its own challenges as the recipient’s native heart is replaced entirely by the xenograft (108–112). Thus, any perturbations in the graft (arrhythmias, ventricular function, or rejection) can have devastating consequences to the recipient. Peri operative cardiac xenograft dysfunction (PCXD) has been observed in 40%–60% of OHTx which has also made the transition difficult (23). However, there has been a success in the OHTx with GTKO.hCD46.hTBM (3-GE) graft survival up to 6 months, despite these hurdles with the aid of non-ischemic continuous xenograft preservation (70, 112). This has been observed by others as well, but the underlying mechanism in cardiac preservation preventing primary graft dysfunction in this setting is poorly understood (113).
The advancement in donor genetic engineering capabilities has also resulted in xenografts with additional transgenes and knockouts for successful long-term OHTx survival. While multi-gene xenografts have certainly fallen into favor, there has been a recent increase in interest for “triple knock out (TKO)” xenografts, which lacks three carbohydrate antigens. In addition to Gal antigens, knockout for additional non-Gal antigens addresses other preformed antibodies that can contribute to humoral rejection. However, like our HHTx experience, we have also seen that hTBM is important in increased survival in xenografts, but specifically, we have seen that TKO grafts exhibit accelerated antibody-mediated rejection and increased incidence of thrombotic complications (16). This could be because of the lack of human transgenes in these TKO xenografts or because TKO xenografts create de novo synthesis, a novel xenoantigen on their surface due to CMAH knockout in the TKO pig that baboon recipients see as foreign (114).
However, multi-gene pigs with double and triple carbohydrate knockouts have been developed for cardiac xenotransplantation and are currently being tested in OHTx and HHTx models. Recently, we have achieved up to 264 day survival of a multi-gene cardiac xenograft with additional human transgene and knockouts (69). Notable modifications in these pigs were are carbohydrate enzyme KO (GTKO and β4GalNT2), growth hormone receptor knockout (GHRKO) and the addition of human transgenes (hCD46, hTBM, hEPCR and hCD47). We are testing cardiac xenograft survival which have over expression of other human genes (8–10 GE) in addition to these from pigs in OHTx with mixed success. These studies, along with others, will soon shed light on the advantages and disadvantages of iterative genetic modifications and pave the way for pre-clinical efficacy required for human clinical trials.
Conclusion
We are now entering an exciting time in xenotransplantation with the progression of survival in preclinical models of pig cardiac xenotransplantation. With the understanding now that a multi-pronged approach toward these recipients’ immunosuppression increases graft survival, most critical of which to date is co-stimulation blockade, attempts to reduce the burden of immunosuppression has placed genetic engineering of cardiac xenografts in the forefront. Increasing the immunocompatibility of xenografts from genetically engineered pigs are a noble approach utilizing technology that has progressed the field further. However, genetic engineering should proceed with caution, utilizing in vitro evidence for every iterative improvement in the genetically engineered cardiac xenograft. Given the field’s current progression and demonstration of success, it is our opinion that multi-gene xenografts which include iterative addition of human transgenes or knockouts of pig genes along with targeted immunosuppression will pave the wave for clinical translation a reality.
Author Contributions
AS, CG, AS, TZ, and IT wrote the paper, DA, KH, and MM reviewed, critiqued revised, and approved the paper.
Funding
Funding is generously provided by public funding-NIH U19 AI090959 “Genetically-engineered Pig Organ Transplantation into Non-Human Primates” and private funding by United Therapeutics. Additional funding was provided by public funding-NIH T32 5T32HL007698-26. The funders were not involved in the study design, collection, analysis, interpretation of data, the writing of this article, or the decision to submit it for publication.
Conflict of Interest
Author DA was employed by the company Revivicor Inc.
The remaining authors declare that the research was conducted in the absence of any commercial or financial relationships that could be construed as a potential conflict of interest.
Acknowledgments
We would like to thank all the authors whose work is cited here and sincerely apologize to significant contributors to xenotransplantation whose work is not reported due to this review's limited scope.
Abbreviations
B4GALNT2, beta-1,4-N-acetyl-galactosaminyltransferase; CC, consumptive coagulopathy; CMAH, CMP-N-acetylneuraminic acid hydroxylase; CRISPR, clustered regularly interspaced short palindromic repeats; CSA, cyclosporin; GHRKO, growth hormone receptor knockout; GTKO, 1-3 alpha Galactosyltransferase knockout; hCRP, human Complement Regulatory Protein; hDAF, human Decay Acceleration Factor; HHTx, Heterotopic Heart xenotransplantation; Neu5Gc, N-glycolylneuraminic acid; OHTx, Orthotopic Heart xenotransplantation; SPF, specified pathogen-free; TBM, thrombomodulin; TKO, triple knockout; TPC, alpha-Gal Polyethylene glycol polymer.
References
1. Rosengard, AM, Cary, N, Horsley, J, Belcher, C, Langford, G, Cozzi, E, et al. Endothelial Expression of Human Decay Accelerating Factor in Transgenic Pig Tissue: a Potential Approach for Human Complement Inactivation in Discordant Xenografts. Transpl Proc (1995) 27:326–7.
2. Cooper, DKC, Kemp, E, Platt, JL, and White, DJG. Xenotransplantation the Transplantation of Organs and Tissues between Species. Berlin: Springer Berlin (2013).
3. Dai, Y, Vaught, TD, Boone, J, Chen, S-H, Phelps, CJ, Ball, S, et al. Targeted Disruption of the α1,3-galactosyltransferase Gene in Cloned Pigs. Nat Biotechnol (2002) 20:251–5. doi:10.1038/nbt0302-251
4. Lai, L, Kolber-Simonds, D, Park, K-W, Cheong, H-T, Greenstein, JL, Im, G-S, et al. Production of α-1,3-Galactosyltransferase Knockout Pigs by Nuclear Transfer Cloning. Science (2002) 295:1089–92. doi:10.1126/science.1068228
5. Phelps, CJ, Koike, C, Vaught, TD, Boone, J, Wells, KD, Chen, S-H, et al. Production of α1,3-Galactosyltransferase-Deficient Pigs. Science (2003) 299:411–4. doi:10.1126/science.1078942
6. Kuwaki, K, Tseng, Y-L, Dor, FJMF, Shimizu, A, Houser, SL, Sanderson, TM, et al. Heart Transplantation in Baboons Using α1,3-galactosyltransferase Gene-Knockout Pigs as Donors: Initial Experience. Nat Med (2005) 11:29–31. doi:10.1038/nm1171
7. Harris, DG, Gao, Z, Sievert, EP, Benipal, P, Cheng, X, Burdorf, L, et al. Transgenic Human Thrombomodulin Expression Reduces Xenogeneic Thrombosis: a Promising Means of Reducing Pig Lung Xenograft Thrombotic Injury. J Heart Lung Transplant (2014) 33:S108-S. doi:10.1016/j.healun.2014.01.323
8. Iwase, H, Liu, H, Wijkstrom, M, Zhou, H, Singh, J, Hara, H, et al. Pig Kidney Graft Survival in a Baboon for 136 Days: Longest Life-Supporting Organ Graft Survival to Date. Xenotransplantation (2015) 22:302–9. doi:10.1111/xen.12174
9. Kozlowski, T, Shimizu, A, Lambrigts, D, Yamada, K, Fuchimoto, Y, Glaser, R, et al. Porcine Kidney and Heart Transplantation in Baboons Undergoing a Tolerance Induction Regimen and Antibody Adsorption1. Transplantation (1999) 67:18–30. doi:10.1097/00007890-199901150-00004
10. Mohiuddin, MM, Corcoran, PC, Singh, AK, Azimzadeh, A, Hoyt, RF, Thomas, ML, et al. B-cell Depletion Extends the Survival of GTKO.hCD46Tg Pig Heart Xenografts in Baboons for up to 8 Months. Am J Transpl (2012) 12:763–71. doi:10.1111/j.1600-6143.2011.03846.x
11. Mohiuddin, MM, Singh, AK, Corcoran, PC, Hoyt, RF, Thomas, ML, Ayares, D, et al. Genetically Engineered Pigs and Target-specific Immunomodulation Provide Significant Graft Survival and hope for Clinical Cardiac Xenotransplantation. J Thorac Cardiovasc Surg (2014) 148:1106–14. discussion 13-4. doi:10.1016/j.jtcvs.2014.06.002
12. Shah, JA, Patel, MS, Elias, N, Navarro-Alvarez, N, Rosales, I, Wilkinson, RA, et al. Prolonged Survival Following Pig-To-Primate Liver Xenotransplantation Utilizing Exogenous Coagulation Factors and Costimulation Blockade. Am J Transpl (2017) 17:2178–85. doi:10.1111/ajt.14341
13. Ekser, B, Tector, AJ, and Cooper, DKC Progress toward Clinical Xenotransplantation. Int J Surg (2015) 23:197–8. doi:10.1016/j.ijsu.2015.08.036
14. Iwase, H, Ekser, B, Satyananda, V, Bhama, J, Hara, H, Ezzelarab, M, et al. Pig-to-baboon Heterotopic Heart Transplantation - Exploratory Preliminary Experience with Pigs Transgenic for Human Thrombomodulin and Comparison of Three Costimulation Blockade-Based Regimens. Xenotransplantation (2015) 22:211–20. doi:10.1111/xen.12167
15. Mohiuddin, MM, Singh, AK, Corcoran, PC, Thomas III, ML, Clark, T, Lewis, BG, et al. Chimeric 2C10R4 Anti-CD40 Antibody Therapy Is Critical for Long-Term Survival of GTKO.hCD46.hTBM Pig-To-Primate Cardiac Xenograft. Nat Commun (2016) 7:11138. doi:10.1038/ncomms11138
16. Singh, AK, Chan, JL, DiChiacchio, L, Hardy, NL, Corcoran, PC, Lewis, BGT, et al. Cardiac Xenografts Show Reduced Survival in the Absence of Transgenic Human Thrombomodulin Expression in Donor Pigs. Xenotransplantation (2019) 26:e12465. doi:10.1111/xen.12465
17. Goerlich, CE, DiChiacchio, L, Zhang, T, Singh, AK, Lewis, B, Tatarov, I, et al. Heterotopic Porcine Cardiac Xenotransplantation in the Intra-abdominal Position in a Non-human Primate Model. Sci Rep (2020) 10:10709. doi:10.1038/s41598-020-66430-x
18. Längin, M, Mayr, T, Reichart, B, Michel, S, Buchholz, S, Guethoff, S, et al. Consistent success in Life-Supporting Porcine Cardiac Xenotransplantation. Nature (2018) 564:430–3. doi:10.1038/s41586-018-0765-z
19. Goerlich, CE, Chan, JL, and Mohiuddin, MM Regulatory Barriers to Xenotransplantation. Curr Opin Organ Transpl (2019) 24:522–6. doi:10.1097/mot.0000000000000678
20. Hawthorne, WJ, Cowan, PJ, Bühler, LH, Yi, S, Bottino, R, Pierson, RN, et al. ThirdWHOGlobal Consultation on Regulatory Requirements for Xenotransplantation Clinical Trials, Changsha, Hunan, China December 12-14, 2018. Xenotransplantation (2019) 26:e12513. doi:10.1111/xen.12513
21. Lexer, G, Cooper, DK, Rose, AG, Wicomb, WN, Rees, J, Keraan, M, et al. Hyperacute Rejection in a Discordant (Pig to Baboon) Cardiac Xenograft Model. J Heart Transpl (1986) 5:411–8.
22. Chen, G, Sun, H, Yang, H, Kubelik, D, Garcia, B, Luo, Y, et al. The Role of Anti-non-gal Antibodies in the Development of Acute Humoral Xenograft Rejection of hDAF Transgenic Porcine Kidneys in Baboons Receiving Anti-gal Antibody Neutralization Therapy. Transplantation (2006) 81:273–83. doi:10.1097/01.tp.0000188138.53502.de
23. Li, Q, Shaikh, S, Iwase, H, Long, C, Lee, W, Zhang, Z, et al. Carbohydrate Antigen Expression and Anti-pig Antibodies in New World Capuchin Monkeys: Relevance to Studies of Xenotransplantation. Xenotransplantation (2019) 26:e12498. doi:10.1111/xen.12498
24. Song, K-H, Kang, Y-J, Jin, U-H, Park, Y-I, Kim, S-M, Seong, H-H, et al. Cloning and Functional Characterization of Pig CMP-N-Acetylneuraminic Acid Hydroxylase for the Synthesis of N-Glycolylneuraminic Acid as the Xenoantigenic Determinant in Pig-Human Xenotransplantation. Biochem J (2010) 427:179–88. doi:10.1042/bj20090835
25. Renton, PHHP, Ikin, EW, Giles, CM, and Goldsmith, KLG. Anti-Sda, a New Blood Group Antibody. Vox Sang (1967) 13:493–501. doi:10.1159/000466498
26. Tector, AJ, Mosser, M, Tector, M, and Bach, J-M The Possible Role of Anti-Neu5Gc as an Obstacle in Xenotransplantation. Front Immunol (2020) 11:622. doi:10.3389/fimmu.2020.00622
27. Tangvoranuntakul, P, Gagneux, P, Diaz, S, Bardor, M, Varki, N, Varki, A, et al. Human Uptake and Incorporation of an Immunogenic Nonhuman Dietary Sialic Acid. Proc Natl Acad Sci (2003) 100:12045–50. doi:10.1073/pnas.2131556100
28. Scobie, L, Padler-Karavani, V, Le Bas-Bernardet, S, Crossan, C, Blaha, J, Matouskova, M, et al. Long-term IgG Response to Porcine Neu5Gc Antigens without Transmission of PERV in Burn Patients Treated with Porcine Skin Xenografts. J.I. (2013) 191:2907–15. doi:10.4049/jimmunol.1301195
29. Diswall, M, Ångström, J, Karlsson, H, Phelps, CJ, Ayares, D, Teneberg, S, et al. Structural Characterization of α1,3-galactosyltransferase Knockout Pig Heart and Kidney Glycolipids and Their Reactivity with Human and Baboon Antibodies. Xenotransplantation (2010) 17:48–60. doi:10.1111/j.1399-3089.2009.00564.x
30. Tseng, Y-L, Moran, K, Dor, FJMF, Sanderson, TM, Li, W, Lancos, CJ, et al. Elicited Antibodies in Baboons Exposed to Tissues from ??1,3-Galactosyltransferase Gene-Knockout Pigs. Transplantation (2006) 81:1058–62. doi:10.1097/01.tp.0000197555.16093.98
31. Estrada, JL, Martens, G, Li, P, Adams, A, Newell, KA, Ford, ML, et al. Evaluation of Human and Non‐human Primate Antibody Binding to Pig Cells Lacking GGTA 1/ CMAH /β4Gal NT 2 Genes. Xenotransplantation (2015) 22:194–202. doi:10.1111/xen.12161
32. Lin, SS, Weidner, BC, Byrne, GW, Diamond, LE, Lawson, JH, Hoopes, CW, et al. The Role of Antibodies in Acute Vascular Rejection of Pig-To-Baboon Cardiac Transplants. J Clin Invest (1998) 101:1745–56. doi:10.1172/jci2134
33. Sachs, DH, Sykes, M, Robson, SC, and Cooper, DKC Xenotransplantation. Adv Immunol (2001) 79:129–223. doi:10.1016/s0065-2776(01)79004-9
34. Sebille, F, Dorling, A, and Lechler, RI. The Cellular Rejection of Xenografts-Rrecent Insights. Xenotransplantation (2003) 10:4–6. doi:10.1034/j.1399-3089.2003.02082.x
35. Friedman, T, Shimizu, A, Smith, RN, Colvin, RB, Seebach, JD, Sachs, DH, et al. Human CD4+ T Cells Mediate Rejection of Porcine Xenografts. J Immunol (1999) 162:5256–62.
36. Seebach, JDWG. Natural Killer Cells in Xenotransplantation. Xenotransplantation (1997) 4. doi:10.1111/j.1399-3089.1997.tb00184.x
37. Candinas, D, Belliveau, S, Koyamada, N, Miyatake, T, Hechenleitner, P, Mark, W, et al. T Cell Independence of Macrophage and Natural Killer Cell Infiltration, Cytokine Production, and Endothelial Activation during Delayed Xenograft Rejection1,2,3. Transplantation (1996) 62:1920–7. doi:10.1097/00007890-199612270-00042
39. Itescu, S, Kwiatkowski, P, Artrip, JH, Wang, SF, Ankersmit, J, Minanov, OP, et al. Role of Natural Killer Cells, Macrophages, and Accessory Molecule Interactions in the Rejection of Pig-To-Primate Xenografts beyond the Hyperacute Period. Hum Immunol (1998) 59:275–86. doi:10.1016/s0198-8859(98)00026-3
40. Lu, T, Yang, B, Wang, R, and Qin, C. Xenotransplantation: Current Status in Preclinical Research. Front Immunol (2019) 10:3060. doi:10.3389/fimmu.2019.03060
41. Dorling, A, Lombardi, G, Binns, R, and Lechler, RI Detection of Primary Direct and Indirect Human Anti-porcine T Cell Responses Using a Porcine Dendritic Cell Population. Eur J Immunol (1996) 26:1378–87. doi:10.1002/eji.1830260630
42. Bretscher, PA A Two-step, Two-Signal Model for the Primary Activation of Precursor Helper T Cells. Proc Natl Acad Sci (1999) 96:185–90. doi:10.1073/pnas.96.1.185
43. Chen, L, and Flies, DB Molecular Mechanisms of T Cell Co-stimulation and Co-inhibition. Nat Rev Immunol (2013) 13:227–42. doi:10.1038/nri3405
44. Lin, CC, Cooper, DKC, and Dorling, A Coagulation Dysregulation as a Barrier to Xenotransplantation in the Primate. Transpl Immunol (2009) 21:75–80. doi:10.1016/j.trim.2008.10.008
45. Lwaleed, BA, and Bass, PS Tissue Factor Pathway Inhibitor: Structure, Biology and Involvement in Disease. J Pathol (2006) 208:327–39. doi:10.1002/path.1871
46. Maroney, SA, Cunningham, AC, Ferrel, J, Hu, R, Haberichter, S, Mansbach, CM, et al. A GPI-Anchored Co-receptor for Tissue Factor Pathway Inhibitor Controls its Intracellular Trafficking and Cell Surface Expression. J Thromb Haemost (2006) 4:1114–24. doi:10.1111/j.1538-7836.2006.01873.x
47. Ito, T, Kawahara, K-i., Okamoto, K, Yamada, S, Yasuda, M, Imaizumi, H, et al. Proteolytic Cleavage of High Mobility Group Box 1 Protein by Thrombin-Thrombomodulin Complexes. Atvb (2008) 28:1825–30. doi:10.1161/atvbaha.107.150631
48. Ikeda, K, Nagasawa, K, Horiuchi, T, Tsuru, T, Nishizaka, H, and Niho, Y C5a Induces Tissue Factor Activity on Endothelial Cells. Thromb Haemost (1997) 77:394–8. doi:10.1055/s-0038-1655974
49. Wojta, J, Huber, K, and Valent, P New Aspects in Thrombotic Research: Complement Induced Switch in Mast Cells from a Profibrinolytic to a Prothrombotic Phenotype. Pathophysiol Haemos Thromb (2003) 33:438–41. doi:10.1159/000083842
50. Byrne, G, Ahmad-Villiers, S, Du, Z, and McGregor, C B4GALNT2 and Xenotransplantation: A Newly Appreciated Xenogeneic Antigen. Xenotransplantation (2018) 25:e12394. doi:10.1111/xen.12394
51. Paul Morgan, B, Berg, CW, and Harris, CL ''Homologous Restriction'' in Complement Lysis: Roles of Membrane Complement Regulators. Xenotransplantation (2005) 12:258–65. doi:10.1111/j.1399-3089.2005.00237.x
52. Cozzi, E, Simioni, P, Boldrin, M, Seveso, M, Calabrese, F, Baldan, N, et al. Alterations in the Coagulation Profile in Renal Pig-To-Monkey Xenotransplantation. Am J Transpl (2004) 4:335–45. doi:10.1046/j.1600-6143.2003.00349.x
53. Cowan, PJ, and Robson, SC Progress towards Overcoming Coagulopathy and Hemostatic Dysfunction Associated with Xenotransplantation. Int J Surg (2015) 23:296–300. doi:10.1016/j.ijsu.2015.07.682
54. Ezzelarab, MB, Ekser, B, Azimzadeh, A, Lin, CC, Zhao, Y, Rodriguez, R, et al. Systemic Inflammation in Xenograft Recipients Precedes Activation of Coagulation. Xenotransplantation (2015) 22:32–47. doi:10.1111/xen.12133
55. Lin, CC, Ezzelarab, M, Shapiro, R, Ekser, B, Long, C, Hara, H, et al. Recipient Tissue Factor Expression Is Associated with Consumptive Coagulopathy in Pig-To-Primate Kidney Xenotransplantation. Am J Transpl (2010) 10:1556–68. doi:10.1111/j.1600-6143.2010.03147.x
56. Morozov, VA, Wynyard, S, Matsumoto, S, Abalovich, A, Denner, J, and Elliott, R No PERV Transmission during a Clinical Trial of Pig Islet Cell Transplantation. Virus Res (2017) 227:34–40. doi:10.1016/j.virusres.2016.08.012
57. Niu, D, Wei, H-J, Lin, L, George, H, Wang, T, Lee, I-H, et al. Inactivation of Porcine Endogenous Retrovirus in Pigs Using CRISPR-Cas9. Science (2017) 357:1303–7. doi:10.1126/science.aan4187
58. Donahue, RE, Kessler, SW, Bodine, D, McDonagh, K, Dunbar, C, Goodman, S, et al. Helper Virus Induced T Cell Lymphoma in Nonhuman Primates after Retroviral Mediated Gene Transfer. J Exp Med (1992) 176:1125–35. doi:10.1084/jem.176.4.1125
59. Allan, GM, and Ellis, JA Porcine Circoviruses: a Review. J Vet Diagn Invest (2000) 12:3–14. doi:10.1177/104063870001200102
60. Krüger, L, Längin, M, Reichart, B, Fiebig, U, Kristiansen, Y, Prinz, C, et al. Transmission of Porcine Circovirus 3 (PCV3) by Xenotransplantation of Pig Hearts into Baboons. Viruses (2019) 11. doi:10.3390/v11070650
61. Iwase, H, Ball, S, Adams, K, Eyestone, W, Walters, A, and Cooper, DKC. Growth Hormone Receptor Knockout: Relevance to Xenotransplantation. Xenotransplantation (2020) 28:e12652. doi:10.1111/xen.12652
62. Tanabe, T, Watanabe, H, Shah, JA, Sahara, H, Shimizu, A, Nomura, S, et al. Role of Intrinsic (Graft) versus Extrinsic (Host) Factors in the Growth of Transplanted Organs Following Allogeneic and Xenogeneic Transplantation. Am J Transpl (2017) 17:1778–90. doi:10.1111/ajt.14210
63. Iwase, H, Hara, H, Ezzelarab, M, Li, T, Zhang, Z, Gao, B, et al. Immunological and Physiological Observations in Baboons with Life-Supporting Genetically Engineered Pig Kidney Grafts. Xenotransplantation (2017) 24. doi:10.1111/xen.12293
64. McGregor, CGA, Ricci, D, Miyagi, N, Stalboerger, PG, Du, Z, Oehler, EA, et al. Human CD55 Expression Blocks Hyperacute Rejection and Restricts Complement Activation in Gal Knockout Cardiac Xenografts. Transplantation (2012) 93:686–92. doi:10.1097/tp.0b013e3182472850
65. Kim, SC, Mathews, DV, Breeden, CP, Higginbotham, LB, Ladowski, J, Martens, G, et al. Long‐term Survival of Pig‐to‐rhesus Macaque Renal Xenografts Is Dependent on CD4 T Cell Depletion. Am J Transpl (2019) 19:2174–85. doi:10.1111/ajt.15329
66. Adams, AB, Kim, SC, Martens, GR, Ladowski, JM, Estrada, JL, Reyes, LM, et al. Xenoantigen Deletion and Chemical Immunosuppression Can Prolong Renal Xenograft Survival. Ann Surg (2018) 268:564–73. doi:10.1097/sla.0000000000002977
67. Burlak, C, Paris, LL, Lutz, AJ, Sidner, RA, Estrada, J, Li, P, et al. Reduced Binding of Human Antibodies to Cells from GGTA1/CMAH KO Pigs. Am J Transpl (2014) 14:1895–900. doi:10.1111/ajt.12744
68. Martens, GR, Reyes, LM, Butler, JR, Ladowski, JM, Estrada, JL, Sidner, RA, et al. Humoral Reactivity of Renal Transplant-Waitlisted Patients to Cells from GGTA1/CMAH/B4GalNT2, and SLA Class I Knockout Pigs. Transplantation (2017) 101:e86–e92. doi:10.1097/tp.0000000000001646
69. Goerlich, CEGB, Hanna, P, Hong, SN, Ayares, D, Singh, AK, and Mohiuddin, MM The Growth of Xenotransplanted Hearts Can Be Reduced with Growth Hormone Receptor Knockout Pig Donors. J Thorac Cardiovasc Surg (2021) 2021:S0022-5223(21)01261-7. doi:10.1016/j.jtcvs.2021.07.051
70. Längin, M, Mayr, T, Reichart, B, Michel, S, Buchholz, S, Guethoff, S, et al. Consistent success in Life-Supporting Porcine Cardiac Xenotransplantation. Nature (2018) 564:430–3. doi:10.1038/s41586-018-0765-z
71. Lutz, AJ, Li, P, Estrada, JL, Sidner, RA, Chihara, RK, Downey, SM, et al. Double Knockout Pigs Deficient in N-Glycolylneuraminic Acid and Galactose α-1,3-Galactose Reduce the Humoral Barrier to Xenotransplantation. Xenotransplantation (2013) 20:27–35. doi:10.1111/xen.12019
72. Li, P, Estrada, JL, Burlak, C, Montgomery, J, Butler, JR, Santos, RM, et al. Efficient Generation of Genetically Distinct Pigs in a Single Pregnancy Using Multiplexed Single-Guide RNA and Carbohydrate Selection. Xenotransplantation (2015) 22:20–31. doi:10.1111/xen.12131
73. Nunes Dos Santos, RM, Carneiro D'Albuquerque, LA, Reyes, LM, Estrada, JL, Wang, Z-Y, Tector, M, et al. CRISPR/Cas and Recombinase-Based Human-To-Pig Orthotopic Gene Exchange for Xenotransplantation. J Surg Res (2018) 229:28–40. doi:10.1016/j.jss.2018.03.051
74. Niu, D, Wei, H-J, Lin, L, George, H, Wang, T, Lee, I-H, et al. Inactivation of Porcine Endogenous Retrovirus in Pigs Using CRISPR-Cas9. Science (2017) 357:1303–7. doi:10.1126/science.aan4187
75. Davis, EA, Pruitt, SK, Greene, PS, Ibrahim, S, Lam, TT, Levin, JL, et al. Inhibition of Complement, Evoked Antibody, and Cellular Response Prevents Rejection of Pig-To-Primate Cardiac Xenografts1. Transplantation (1996) 62:1018–23. doi:10.1097/00007890-199610150-00022
76. Lam, TT, Hausen, B, Squiers, E, Cozzi, E, and Morris, RE Cyclophosphamide-induced Postoperative Anemia in Cynomolgus Monkey Recipients of hDAF-Transgenic Pig Organ Xenografts. Transplant Proc (2002) 34:1451–2. doi:10.1016/s0041-1345(02)02925-1
77. Wu, G, Pfeiffer, S, Schröder, C, Zhang, T, Nguyen, BN, Kelishadi, S, et al. Coagulation cascade Activation Triggers Early Failure of Pig Hearts Expressing Human Complement Regulatory Genes. Xenotransplantation (2007) 14:34–47. doi:10.1111/j.1399-3089.2006.00362.x
78. Bhatti, FN, Schmoeckel, M, Zaidi, A, Cozzi, E, Chavez, G, Goddard, M, et al. Three-month Survival of HDAFF Transgenic Pig Hearts Transplanted into Primates. Transpl Proc (1999) 31:958. doi:10.1016/s0041-1345(98)01855-7
79. Byrne, GW, Du, Z, Sun, Z, Asmann, YW, and McGregor, CGA Changes in Cardiac Gene Expression after Pig-To-Primate Orthotopic Xenotransplantation. Xenotransplantation (2011) 18:14–27. doi:10.1111/j.1399-3089.2010.00620.x
80. Xu, H, Gundry, SR, Hancock, WW, Matsumiya, G, Zuppan, CW, Morimoto, T, et al. Prolonged Discordant Xenograft Survival and Delayed Xenograft Rejection in a Pig-To-Baboon Orthotopic Cardiac Xenograft Model. J Thorac Cardiovasc Surg (1998) 115:1342–9. doi:10.1016/s0022-5223(98)70218-1
80. McGregor, CG, Davies, WR, Oi, K, Teotia, SS, Schirmer, JM, Risdahl, JM, et al. Cardiac Xenotransplantation: Recent Preclinical Progress with 3-month Median Survival. J Thorac Cardiovasc Surg (2005) 130:844. doi:10.1016/j.jtcvs.2005.04.017
82. Kuwaki, K, Tseng, Y-L, Dor, FJMF, Shimizu, A, Houser, SL, Sanderson, TM, et al. Heart Transplantation in Baboons Using α1,3-galactosyltransferase Gene-Knockout Pigs as Donors: Initial Experience. Nat Med (2005) 11:29–31. doi:10.1038/nm1171
83. Teotia, SS, Walker, RC, Schirmer, JM, Tazelaar, HD, Michaels, MG, Risdahl, JM, et al. Prevention, Detection, and Management of Early Bacterial and Fungal Infections in a Preclinical Cardiac Xenotransplantation Model that Achieves Prolonged Survival. Xenotransplantation (2005) 12:127–33. doi:10.1111/j.1399-3089.2005.00205.x
84. Lin, SS, Hanaway, MJ, Gonzalez-Stawinski, GV, Lau, CL, Parker, W, Davis, RD, et al. The Role of Anti-gal??1-3Gal Antibodies in Acute Vascular Rejection and Accommodation of Xenografts1. Transplantation (2000) 70:1667–74. doi:10.1097/00007890-200012270-00002
85. Byrne, GW, Schirmer, JM, Fass, DN, Teotia, SS, Kremers, WK, Xu, H, et al. Warfarin or Low-Molecular-Weight Heparin Therapy Does Not Prolong Pig-To-Primate Cardiac Xenograft Function. Am J Transpl (2005) 5:1011–20. doi:10.1111/j.1600-6143.2005.00792.x
86. Byrne, GW, Davies, WR, and Oi, K Increased Immunosuppression, Not Anticoagulation, Extends Cardiac Xenograft Survival. Transplantation (2006) 82:1787–91. doi:10.1097/01.tp.0000251387.40499.0f
87. McGregor, CGA, Teotia, SS, Byrne, GW, Michaels, MG, Risdahl, JM, Schirmer, JM, et al. Cardiac Xenotransplantation: Progress toward the Clinic. Transplantation (2004) 78:1569–75. doi:10.1097/01.tp.0000147302.64947.43
88. McGregor, CG, Davies, WR, Oi, K, Teotia, SS, Schirmer, JM, Risdahl, JM, et al. Cardiac Xenotransplantation: Recent Preclinical Progress with 3-month Median Survival. J Thorac Cardiovasc Surg (2005) 130:844–51. doi:10.1016/j.jtcvs.2005.04.017
89. Doménech, N, Diaz, T, Moscoso, I, López-Peláez, E, Centeno, A, and Máñez, R. Elicited Non-anti-αgal Antibodies May Cause Acute Humoral Rejection of hDAF Pig Organs Transplanted in Baboons. Transpl Proc (2003) 35:2049–50. doi:10.1016/s0041-1345(03)00706-1
90. Chan, MCY, Stalder, M, Lam, TT, Tye, T, Borie, DC, and Morris, RE. Use of Echocardiography to Assess Function of hDAF-Transgenic Pig Cardiac Xenografts. Transpl Proc (2005) 37:1923–5. doi:10.1016/j.transproceed.2005.02.067
91. Lam, TT, Borie, D, Masek, M, Berry, G, Larson, M, and Morris, RE Graft Thrombosis in hDA-Transgenic Pig Hearts Transplanted into Rhesus Monkeys. Xenotransplantation (2003) 10:185–6. doi:10.1034/j.1399-3089.2003.02100.x
92. Schirmer, JM, Fass, DN, Byrne, GW, Tazelaar, HD, Logan, JS, and McGregor, CGA Effective Antiplatelet Therapy Does Not Prolong Transgenic Pig to Baboon Cardiac Xenograft Survival. Xenotransplantation (2004) 11:436–43. doi:10.1111/j.1399-3089.2004.00159.x
93. Kuwaki, K, Knosalla, C, Dor, FJMF, Gollackner, B, Tseng, Y-L, Houser, S, et al. Suppression of Natural and Elicited Antibodies in Pig-To-Baboon Heart Transplantation Using a Human Anti-human CD154 mAb-Based Regimen. Am J Transplant (2004) 4:363–72. doi:10.1111/j.1600-6143.2004.00353.x
94. Houser, SL, Kuwaki, K, Knosalla, C, Dor, FJMF, Gollackner, B, Cheng, J, et al. Thrombotic Microangiopathy and Graft Arteriopathy in Pig Hearts Following Transplantation into Baboons. Xenotransplantation (2004) 11:416–25. doi:10.1111/j.1399-3089.2004.00155.x
95. Brandl, U, Michel, S, Erhardt, M, Brenner, P, Bittmann, I, Rossle, M, et al. Administration of GAS914 in an Orthotopic Pig-To-Baboon Heart Transplantation Model. Xenotransplantation (2005) 12:134–41. doi:10.1111/j.1399-3089.2005.00208.x
96. Vial, C, Ostlie, DJ, and Bhatti, FNK Life Supporting Function for over One Month of a Transgenic Porcine Heart in a Baboon. J Heart Lung Transplant (2000) 19:224–9. doi:10.1016/s1053-2498(99)00099-6
97. Schmoeckel, M, Bhatti, FNK, Zaidi, A, Cozzi, E, Waterworth, PD, Tolan, MJ, et al. Orthotopic Heart Transplantation in a Transgenic Pig-To-Primate Model1. Transplantation (1998) 65:1570–7. doi:10.1097/00007890-199806270-00006
98. Brenner, PMT, Reichart, B, Guethoff, S, Buchholz, S, Dashkevich, A, Michel, S, et al. New Standards in Orthotopic Cardiac Xenotransplantation of Multitransgenic Pig Hearts Preserved with “Steens” Cold Blood Cardioplegia Perfusion in a Pig-To-Baboon Model with CD40mAb or CD40L Costimulation Blockade. Thorac Cardiovasc Surg (2017) 65(S 01). doi:10.1055/s-0037-1598771
99. Bühler, L, Awwad, M, Basker, M, Gojo, S, Watts, A, Treter, S, et al. High-dose Porcine Hematopoietic Cell Transplantation Combined with CD40 Ligand Blockade in Baboons Prevents an Induced Anti-pig Humoral Response. Transplantation (2000) 69:2296–304. doi:10.1097/00007890-200006150-00013
100. Mohiuddin, MM, Singh, AK, Corcoran, PC, Hoyt, RF, Thomas, ML, Lewis, BGT, et al. Role of Anti-CD40 Antibody-Mediated Costimulation Blockade on Non-gal Antibody Production and Heterotopic Cardiac Xenograft Survival in a GTKO.hCD46Tg Pig-To-Baboon Model. Xenotransplantation (2014) 21:35–45. doi:10.1111/xen.12066
101. McGregor, CGA, and Byrne, GW. Porcine to Human Heart Transplantation: Is Clinical Application Now Appropriate? J Immunol Res (2017) 2017:2534653. doi:10.1155/2017/2534653
102. Yang, L, Guell, M, Niu, D, George, H, Lesha, E, Grishin, D, et al. Genome-wide Inactivation of Porcine Endogenous Retroviruses (PERVs). Science (2015) 350:1101–4. doi:10.1126/science.aad1191
103. Ballou, LM, and Lin, RZ Rapamycin and mTOR Kinase Inhibitors. J Chem Biol (2008) 1:27–36. doi:10.1007/s12154-008-0003-5
104. Hinrichs, A, Riedel, EO, and Klymiuk, N Growth Hormone Receptor Knockout to Reduce the Size of Donor Pigs for Preclinical Xenotransplantation Studies. Xenotransplantation (2020) 28:e12664. doi:10.1111/xen.12664
105. Lelovas, PP, Kostomitsopoulos, NG, and Xanthos, TT. A Comparative Anatomic and Physiologic Overview of the Porcine Heart. J Am Assoc Lab Anim Sci (2014) 53:432–8.
106. Längin, M, Konrad, M, Reichart, B, Mayr, T, Vandewiele, S, Postrach, J, et al. Hemodynamic Evaluation of Anesthetized Baboons and Piglets by Transpulmonary Thermodilution: Normal Values and Interspecies Differences with Respect to Xenotransplantation. Xenotransplantation (2020) 27:e12576. doi:10.1111/xen.12576
107. Langin, M, Konrad, M, and Reichart, B Hemodynamic Evaluation of Anesthetized Baboons and Piglets by Transpulmonary Thermodilution: Normal Values and Interspecies Differences with Respect to Xenotransplantation. Xenotransplantation (2019) 2019:e12576. doi:10.1111/xen.12576
108. DiChiacchio, L, Singh, AK, Lewis, B, Zhang, T, Hardy, N, Pasrija, C, et al. Early Experience with Preclinical Perioperative Cardiac Xenograft Dysfunction in a Single Program. Ann Thorac Surg (2020) 109:1357–61. doi:10.1016/j.athoracsur.2019.08.090
109. Byrne, GW, Stalboerger, PG, Davila, E, Heppelmann, CJ, Gazi, MH, McGregor, HCJ, et al. Proteomic Identification of Non-gal Antibody Targets after Pig-To-Primate Cardiac Xenotransplantation. Xenotransplantation (2008) 15:268–76. doi:10.1111/j.1399-3089.2008.00480.x
110. Waterworth, PD, Dunning, J, Tolan, M, Cozzi, E, Langford, G, Chavez, G, et al. Life-supporting Pig-To-Baboon Heart Xenotransplantation. J Heart Lung Transpl (1998) 17:1201–7.
111. Mohiuddin, MM, Singh, AK, Corcoran, PC, Hoyt, RF, Thomas, ML, Lewis, BGT, et al. One-year Heterotopic Cardiac Xenograft Survival in a Pig to Baboon Model. Am J Transpl (2014) 14:488–9. doi:10.1111/ajt.12562
112. Brenner, P, Mayr, T, Reichart, B, Guethoff, S, Buchholz, S, Dashkevich, A, et al. 40 Days Survival after Orthotopic Cardiac Xenotransplantation of Multi-Transgenic Pig Hearts in a Pig-To-Baboon Model with CD40mAb or CD40L Costimulation Blockade and Xenograft Preservation Using "Steens" Cold Blood Cardioplegia Perfusion. Transplantation (2017) 101:S65-S. doi:10.1097/01.tp.0000520401.22958.4c
113. Goerlich, CE, Griffith, B, Singh, AK, Abdullah, M, Singireddy, S, Kolesnik, I, et al. Blood Cardioplegia Induction, Perfusion Storage and Graft Dysfunction in Cardiac Xenotransplantation. Front Immunol (2021) 12:667093. doi:10.3389/fimmu.2021.667093
Keywords: xenotransplantation, cardiac transplantation, transplantation, pre clinical model of xenotransplantation, heart transplantation
Citation: Singh AK, Goerlich CE, Shah AM, Zhang T, Tatarov I, Ayares D, Horvath KA and Mohiuddin MM (2022) Cardiac Xenotransplantation: Progress in Preclinical Models and Prospects for Clinical Translation. Transpl Int 35:10171. doi: 10.3389/ti.2022.10171
Received: 01 November 2021; Accepted: 11 January 2022;
Published: 23 March 2022.
Copyright © 2022 Singh, Goerlich, Shah, Zhang, Tatarov, Ayares, Horvath and Mohiuddin. This is an open-access article distributed under the terms of the Creative Commons Attribution License (CC BY). The use, distribution or reproduction in other forums is permitted, provided the original author(s) and the copyright owner(s) are credited and that the original publication in this journal is cited, in accordance with accepted academic practice. No use, distribution or reproduction is permitted which does not comply with these terms.
*Correspondence: Muhammad M. Mohiuddin, TU1PSElVRERJTkBzb20udW1hcnlsYW5kLmVkdQ==