- 1Laboratory of Abdominal Transplantation, Department of Microbiology, Immunology and Transplantation, Faculty of Medicine, KU Leuven, Leuven, Belgium
- 2University Hospital of Liège, Liège, Belgium
- 3University Hospitals Leuven, Leuven, Belgium
- 4General Surgery 2U–Liver Transplant Unit, A.O.U. Città della Salute e della Scienza di Torino, University of Turin, Turin, Italy
- 5Dipartimento di Chirurgia Generale e Specialistica, Azienda Ospedaliero Universitaria Città della Salute e della Scienza di Torino, Turin, Italy
- 6Department of Medical Sciences, School of Medicine, University of Turin, Turin, Italy
- 7Molecular Biotechnology Center, Department of Molecular Biotechnology and Health Sciences, School of Medicine, University of Turin, Torino, Italy
Organ preservation and assessment with machine perfusion (MP) has provided transplant physicians with the ability to evaluate and select grafts suitable for transplantation. Nevertheless, the discard of organs considered too damaged still sustains the imbalance between donor organs supply and demands. Therefore, there is the pressing clinical need for strategies to repair and/or regenerate organs before transplantation, and MP is uniquely positioned to satisfy this need. The systemic administration of mesenchymal stromal cells (MSC) was shown to reduce ischemia-reperfusion injury in pre-clinical organ transplant models but could not be reproduced in clinical transplantation, largely because of inefficient cell delivery. The administration of MSC during MP is one strategy that recently gained much attention as an alternative delivery method to target MSC directly to the donor organ. However, careful reinterpretation of preliminary results reveals that this approach is equally limited by a suboptimal delivery of short-lived MSC to the target organ. In contrast, the use of MSC secretome and/or extracellular vesicles therapy during MP seems to be more efficient in harnessing MSC properties during MP. In this mini review we speculate on the future of the novel niche of ex situ organ repair and regeneration before transplantation.
Introduction
The field of organ preservation for transplantation has undergone significant changes due to the increasing use of grafts from high-risk donors. The need for improved preservation of these organs has prompted a progressive shift from static cold storage to dynamic organ preservation strategies, also known as machine perfusion (MP). Dynamic organ preservation strategies have also moved the field at the intersection with regenerative medicine as they provide a platform for repairing and regenerating organs before transplantation [1]. However, prolonged ex situ preservation for multiple days is likely required to achieve clinically meaningful organ repair and regeneration during MP. Recent advancements in liver normothermic-MP (NMP), which allows for the preservation of the liver for up to 1 week [2], suggest that this may soon be attainable for all other transplantable organs.
To this end, several interventions during MP have been proposed, including cell therapy, pharmacological agents, gene modulation and editing, and nanoparticles [3]. Whereas most of these strategies are still in early stages of investigation, numerous pre-clinical studies have shown that the systemic administration of mesenchymal stromal cells (MSC) reduces ischemia-reperfusion injury (IRI) during organ transplantation [4]. MSC suppress the inflammatory response, downregulate innate and adaptive immunity, and promote organ regeneration, thereby interfering with the major pathophysiological events of IRI in transplantable organs [4]. However, the clinical application of MSC systemic treatment during organ transplantation has failed to replicate these results [5], one of the major putative cause being the inefficient delivery of MSC to the target organ. Therefore, MSC administration during MP has gained interest as an alternative method to deliver the cells directly to an organ and circumvent the shortcomings of systemic administration. Nevertheless, the efficiency of this approach in delivering MSC-therapy to organs for transplantation remains underinvestigated. In this narrative minireview, we summarize results and limitations of MSC-therapy during MP based on available evidence from published studies. To select these studies, we utilized a systematic literature search approach, a rigorous method for minimizing biases during evidence selection (see Supplementary Material for additional method information). Additionally, we hypothesize a path towards a cell-free future for ex situ organ repair and regeneration.
Novel Delivery Method, Same Shortcomings
Unlike preclinical transplant models of MSC-therapy, clinical studies have failed to show significant benefits from systemic MSC administration on post-transplant IRI [6, 7]. This was ascribed to inefficient and off-target delivery of cells to the graft [5]. Systemic infusion of MSC are short-lived as the cells are primarily sequestered in the lungs and eliminated by resident monocytes [5]. Administration of stem cells after organ transplantation has also been associated with a pro-inflammatory effect, which further damages the graft [8]. Lastly, calcineurin inhibitors suppress the immunomodulatory properties of MSC in vitro [9]. To overcome these hurdles, it was proposed to deliver the cells directly to an organ during MP, before the full extent of IRI events has occurred and interference with immunosuppressive agents can take place.
However, the currently available pre-clinical evidence shows that MSC delivery during MP presents shortcomings similar to those of systemic therapy, as summarized in Table 1 and depicted in Figure 1. A significant proportion of MSC injected through the vascular cannula during MP are eliminated by a “device barrier,” constituted by oxygenator(s) and filter(s), which remove the cells from the perfusate similarly to the “lung barrier” phenomenon in systemic MSC-therapy. In a porcine kidney study, Pool et al. demonstrated that 90% of infused MSC are eliminated from the perfusate in a NMP circuit operated without the organ, and that only a few MSC were retained after the first passage through the kidney [10]. In a porcine lung NMP model, Mordant et al. found MSC sequestered in the leukocyte filter [11]. Similarly, Laing et al. did not observe any cell in left hepatic segments after selectively delivering multipotent adult progenitor cells (MAPc) to the right hemi-liver during NMP of discarded human grafts [12]. These results indicate that, at best, only a (small) fraction of MSC is effectively retained in the perfused organ. Additionally, biodistribution studies have shown that MSC have an inhomogeneous distribution in otherwise well-perfused kidney [10] and liver [13] grafts. In accordance with this, histological studies have shown that <10 cells per high-power field are found outside of the vascular space during MP of the liver [12, 13], lung [11], and kidney [14, 15]. Although in the study by Pool et al. increasing the dose of MSC increased the number of cells observed at histology, a dose of MSC far exceeding the previously suggested range for MSC systemic therapy was needed to visualize the cells in the glomeruli of porcine kidneys [10]. Lastly, five other studies reported that MSC did not leave the perfusate or migrate out of the vascular lumen during MP of human and porcine kidneys [16] and rat livers [17–20] (Table 1).
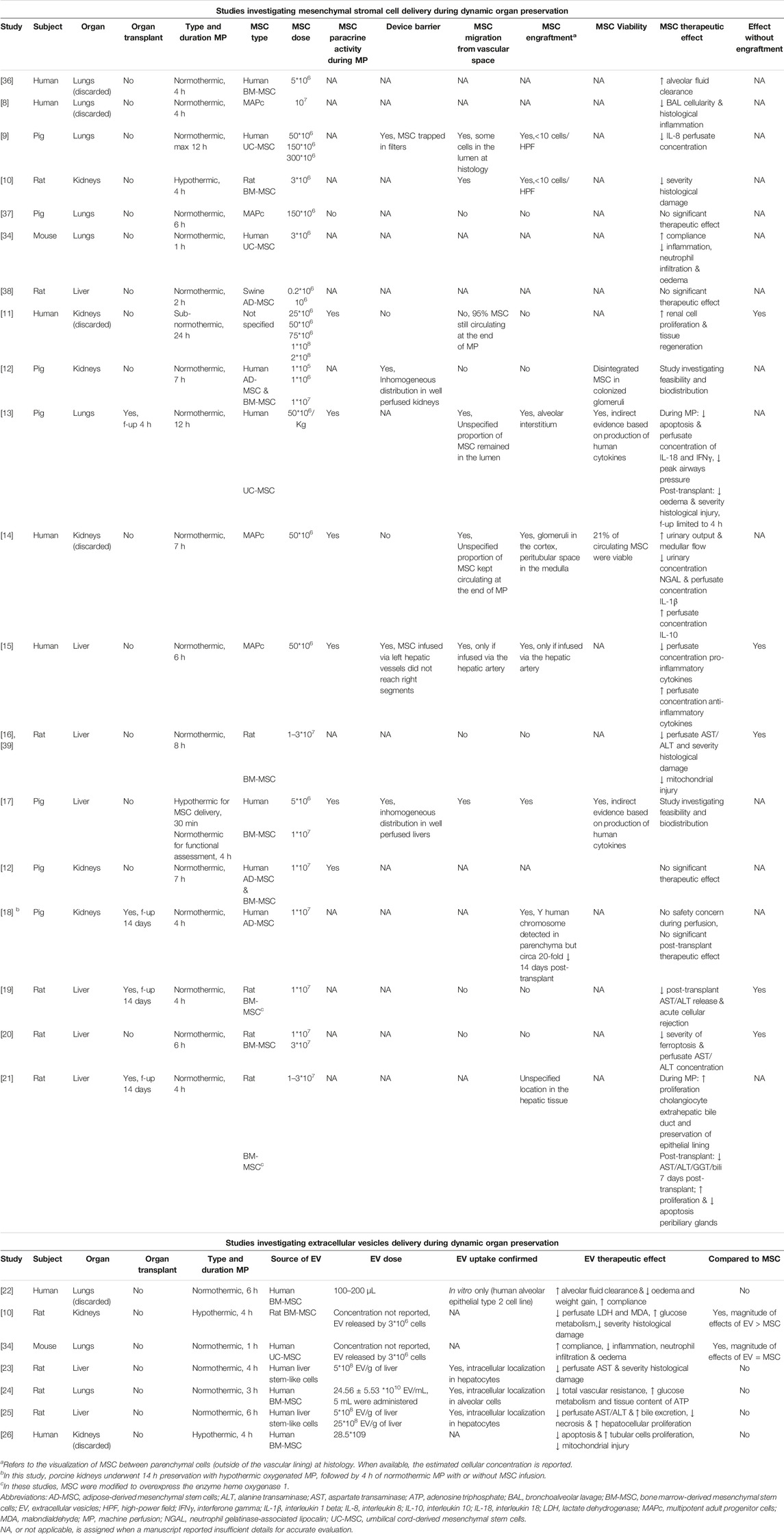
TABLE 1. Summary of findings of studies investigating stem cell therapy delivery during ex situ dynamic organ preservation identified after systematic search of the literature (details in Supplementary Material). Results from preliminary studies investigating extracellular vesicle therapy during machine perfusion of transplantable organs are also summarized.
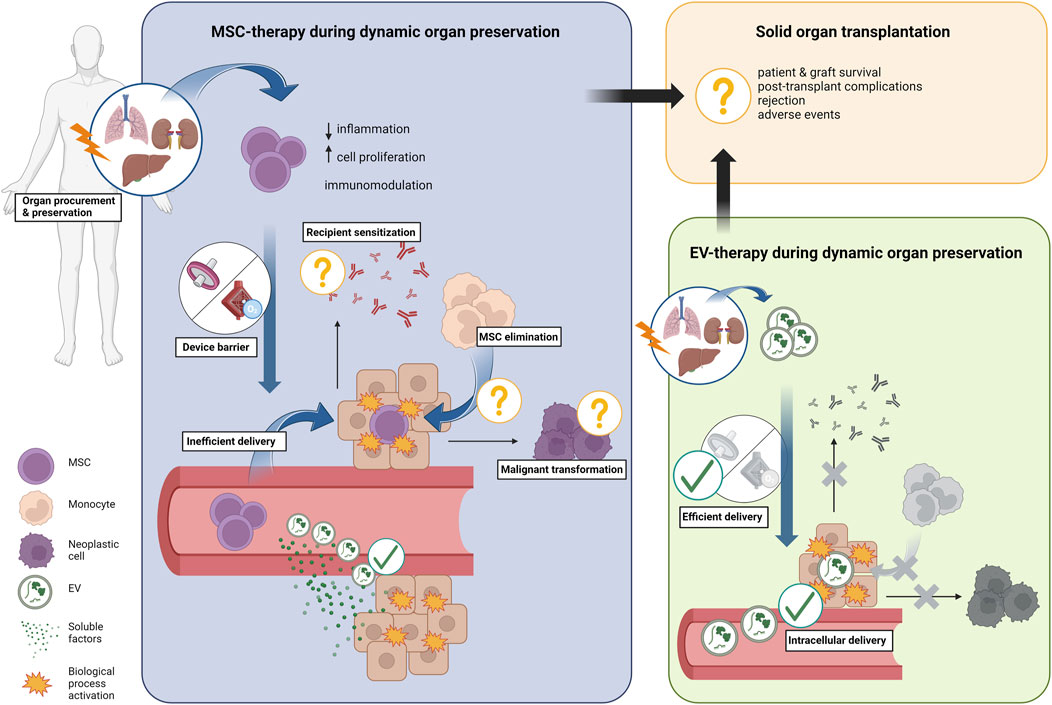
FIGURE 1. Overview of current knowledge on cell therapy delivery during ex situ dynamic preservation. Mesenchymal stromal cells (MSC) are perfect candidates for organ repair and regeneration during ex situ dynamic organ preservation due to their anti-inflammatory, regenerative, and immunomodulatory properties. However, a “device barrier” created by components of the perfusion circuit (i.e., oxygenator(s) and filter(s)) sequester the cells from the circulating perfusate, with a bottleneck effect on the number of cells that effectively reach the parenchyma during perfusion. Additionally, migration of stem cells out of the vascular space is infrequent, only few cells are usually observed in the parenchymal space at histology, and parenchymal retention rate of stem cell is rather low. Furthermore, monocytes may actively eliminate successfully engrafted stem cells, similarly to what has been previously observed in pre-clinical studies. Because secretion of soluble factors has been observed during perfusion, it is plausible that the biological effects of stem cells are mostly dependent on paracrine mediators, extracellular vesicles (EV) included. EV delivery during ex situ dynamic organ preservation has been shown to circumvent the “device barrier” while intracellular uptake of EV has been demonstrated during lung and liver perfusion specifically, resulting in significant anti-inflammatory and regenerative effects. Therefore, EV therapy may be more effective than MSC therapy in promoting organ repair and regeneration during ex situ dynamic organ preservation because of more efficient therapy delivery. Next to a more efficient therapy delivery, cell-free therapy with EV prevents resident monocytes activation and eliminates the risk of malignant transformation and recipient sensitization, which cannot be excluded when allogenic stem cells are administered.
Next to the “device barrier” and low cell retention rates, there are also indications that MSC infused during MP are short-lived, likely due to factors such as mechanical trauma, perfusate toxicity, or phagocytosis by resident monocytes. Pool et al. consistently observed disintegrated MSC in porcine glomeruli colonized by stem cells [10], whereas Thompson et al. reported that at the end of NMP of discarded human kidneys only 21% of the MAPc still circulating in the perfusate were viable (Table 1) [15]. Research has shown that, compared to standard culturing medium, suspending MSC in a standard red blood cells-based MP perfusate reduces significantly their survival and adherence to endothelial cells [21]. Additionally, because monocytes were already shown to phagocyte MSC [5], it is plausible that resident monocytes and/or passenger leukocytes will eliminate MSC during MP (Figure 1). However, to date this phenomenon has not been investigated yet.
Despite the low cell retention rates, there are indications of significant anti-inflammatory [15, 22], immunomodulatory [18] and pro-regenerative [16, 20] effects of MSC-therapy during MP (Table 1). Nevertheless, the clinical relevance and durability remain unclear as the few studies that transplanted MSC-treated grafts have only reported short-term follow-ups with contrasting results (Table 1). Rat livers were transplanted after MSC-therapy during MP, showing significant improvement of survival and reduction of the incidence of acute cellular rejection at 14 days post-transplant [18]. Porcine lungs treated with MSC were transplanted and followed up for 4 h after reperfusion, showing reduction of pulmonary oedema and severity of histological injury [23]. In contrast, porcine kidneys that were transplanted after MSC-therapy during NMP showed no relevant therapeutic effect within 14 days after transplantation [24].
Hence, while a direct comparison is lacking, available pre-clinical evidence indicates that MSC-therapy during MP presents similar shortcomings and may not be more effective than MSC systemic therapy in delivering the cells to the graft. Furthermore, allogeneic MSC-therapy during MP does not eliminate the potential for recipient allo-sensitization to cell donor antigens [6, 25] or malignant transformation of (the few) successfully engrafted cells. These are two potential complications that cannot be ruled out when allogeneic stem cells are administered to patients who will receive immunosuppressants after transplantation.
Cell-free Organ Repair and Regeneration During Ex Situ Dynamic Preservation
While MSC administration during MP does not have high efficiency in cell delivery, the anti-inflammatory [12], immunomodulatory [18], and regenerative [16, 20] effects of MSC, as well as significant reduction in the severity of graft injury [14, 17, 19, 20] have been observed during perfusion. These effects were observed even when MSC remained suspended in the perfusate, did not migrate out of blood vessels, or did not survive (Table 1). Most strikingly, Brasile et al. found that renal cell proliferation was significantly enhanced in perfused kidneys despite the fact that 95% of MSC did not migrate in the renal tissue but remained in the perfusate for 24 h [16]. This effect was attributed to the release of growth factors by MSC [16]. Several other MP studies reported that MSC actively secrete soluble and paracrine factors in the perfusate (Table 1) [12, 13, 15, 23, 26]. The frequent observation that MSC have significant detectable effects during MP even when no direct contact between MSC and parenchymal cells has taken place, and that MSC secrete paracrine mediators during MP, strongly suggest that their effects rely mostly on soluble factors and paracrine mediators, such as growth factors, cytokines, chemokines, and extracellular vesicles (EV). This implies that the biological properties of MSC beneficial against organ IRI could be harnessed during MP with a cell-free therapy consisting of MSC secretome and/or purified EV [4, 5].
EV are nano-sized particles released by every (stem) cell. As they contain genetic information, growth factors, and signal transduction molecules [4], they play an important role in (stem) cell-mediated regulation of homeostasis and orchestration of tissue regeneration [27]. Upon internalization by neighboring or distant target cells, EV release their biological active cargo and induce epigenetic modifications of target cell biology, mediating the biological effects of the parent stem cell. During ex situ dynamic organ preservation, cell-free therapy with concentrated stem cell-derived EV has already shown encouraging results (Table 1). Studies have already demonstrated that EV are taken up by alveolar cells and hepatocytes during perfusion in rodent models of NMP of freshly procured lungs [28] and livers [29], resulting in significant improvements in pulmonary metabolism and adenosine triphosphate content [28], as well as reduction in transaminases and severity of histological injury during perfusion [29]. Additionally, in the study by De Stefano et al., EV from human liver stem-like cells reduced hepatocellular injury and increased cell proliferation during NMP of rat livers that suffered 60 min warm ischemic injury [30]. Gennai et al. showed that EV-therapy during NMP of discarded human lungs significantly improved alveolar fluid clearance, reducing inflammation and pulmonary oedema [31]. Gregorini et al. delivered MSC-EV during hypothermic-MP of rat kidneys, showing a significant reduction in markers of renal injury and oxidative stress [14]. The same group reported similar observations with EV-therapy during hypothermic-MP of discarded human kidneys [32]. If replicated, these findings would indicate that there may be an additional window of opportunity to deliver cell-free therapy during hypothermic dynamic organ preservation. However, transplantation of grafts treated with EV during MP has not been attempted yet, and future studies should focus on testing the hypothesis that EV-therapy at the time of MP affects post-transplant outcomes.
Hypothetically, cell-free therapy during MP could also avail of the delivery of MSC secretome, which contains both soluble factors and EV. To our knowledge, this therapeutic option has not yet been investigated.
Discussion
The Future is Nano
Dynamic organ preservation strategies have entered the clinical arena and are expected to improve the preservation of high-risk organs. MSC-therapy during MP was proposed as an approach to repair high-risk grafts that are deemed too damaged and render them suitable for transplantation [1, 3]. However, there is sufficient accumulated evidence to conclude that MSC are short-lived during MP and poorly delivered to the target organ, similarly to systemic MSC-therapy, while the inherent risks of recipient’s sensitization [6] and malignant transformation remain. Therefore, although cell therapy may still play a role for instance in the recellularization of human organ scaffolds, alternative strategies for repairing and regenerating organs ex situ should be investigated in the future.
MSC-derived cell-free therapy during MP has several advantages and circumvent the shortcomings of MSC delivery during ex situ dynamic preservation. In a recent systematic review of preclinical studies, we examined the efficacy of EV-therapy derived from stem cells in mitigating IRI in transplantable organs. Our findings indicate that EV-therapy significantly enhances post-reperfusion outcomes, histology, and function in the heart, lung, liver, and kidney, regardless of the originating stem cell source [33]. As EV and soluble factors are unaffected by the “device barrier” phenomenon [28–30], it can be hypothesized that the EV delivery during MP will be more efficient than MSC delivery (Figure 1). Furthermore, whereas MSC suspended in the perfusate at the end of MP are flushed out of the organ before transplantation, the intracellular localization of EV during MP [30, 31] ensures that they will be readily available at the time of graft reperfusion. The EV intracellular localization also prevents their elimination by resident monocytes, and the absence of human leukocytes antigens on EV membranes minimizes the risk of allo-sensitization in the recipient. Additionally, cell-free therapy during MP eliminates the risk of malignant transformation of engrafted cells. Lastly, the use of concentrated EV may offer a selective advantage because they transfer mRNA and miRNA. This transfer has the potential to induce long-lasting biological changes in target cells, which may persist even after graft reperfusion. For these reasons, and because EV possess biological properties comparable to those of the parental stem cell population, it can be hypothesized that EV-therapy will be safer and more efficient than MSC delivery during MP in harnessing MSC properties for repairing and regenerating organs before transplantation. Treatment with EV during MP has already delivered encouraging preliminary results [29, 31]; nevertheless, this hypothesis must be tested in preclinical transplant models of high translational value, as well as in clinical studies.
To move toward clinical applications, it is crucial to determine whether the therapeutic effects of EV match those of their parent stem cells. Preliminary studies suggest that MSC and EV-therapy during MP yield similar results [14, 34]. Nonetheless, further research is required to validate this hypothesis. Additionally, the mechanisms of protection against organ IRI of soluble factors and EV released by different stem cell types should be thoroughly assessed and compared. Indeed, given the complex pathophysiology of IRI, a combined treatment with soluble factors and EV from multiple sources may deliver superior benefits. Dose-finding studies in a clinically relevant model are also necessary to identify the optimal dose of EV and/or soluble factors needed to yield relevant and durable therapeutic effects [33]. Currently, the dose of EV necessary for treating human organs can only be projected based on small animal studies, and inter-species difference may lead to overestimation of the therapeutic dose. This is a crucial point since the large scale production of purified EV is currently an unmet need and one of the major impediments to the clinical application of EV-therapy due to technological limitation. Pre-clinical studies with a larger model, phylogenetically closer to the humans may improve the estimation of the therapeutic dose. Next to organ transplantation, EV-therapy may be of benefit in several medical fields, including genetic and oncological diseases. Indeed, EV can be engineered and programmed to interact with specific cell populations to deliver a cargo enriched with gene modulating and editing agents for the treatment of genetic conditions [35], or chemotherapy and other antineoplastic agents for the treatment of malignant diseases. Thus, there seems to be ample convergence of interests for academic centres and industry to engage in research cooperations to foster technological advancements and develop procedures for scalable production and purification of EV compliant with good manufacturing practice. We strongly advocate for this type of cooperation as an essential step towards bringing EV-therapy to clinical practice, in particular to the novel field of ex situ organ repair and regeneration before transplantation.
In conclusion, MSC-therapy during MP is burdened by suboptimal delivery of short-lived MSC. However, their therapeutic benefits may be leveraged using a cell-free therapy consisting of concentrated EV and/or MSC secretome administered during MP. This approach resulted in the intracellular delivery of EV during perfusion and yielded therapeutic benefit in non-transplant models. We hypothesize that technology at nanoscale, such as EV, gene editing, and nanoparticles, have the highest likelihood of successfully translating into clinical applications and will shape the future of ex situ organ repair and regeneration before transplantation.
Author Contributions
NG and JB contributed equally to study design, systematic search planning, articles screening, data analysis, results interpretation, writing of the manuscript. JP RR, and GC participated in results interpretation, critical revision of the manuscript. DM participated in study design, data analysis, results interpretation, and critical revision of the manuscript. All authors contributed to the article and approved the submitted version.
Conflict of Interest
The authors declare that the research was conducted in the absence of any commercial or financial relationships that could be construed as a potential conflict of interest.
Acknowledgments
JP is recipient of an IGL chair for abdominal transplant surgery. DM is a senior researcher of The Research Foundation (FWO)—Flanders. The lab of Abdominal Transplantation (Department of Microbiology, Immunology and Transplantation, Faculty of Medicine, KU Leuven) has received unrestricted grants from Astellas and Roche.
Supplementary Material
The Supplementary Material for this article can be found online at: https://www.frontierspartnerships.org/articles/10.3389/ti.2023.11947/full#supplementary-material
Abbreviations
EV, extracellular vesicles; IRI, ischemia-reperfusion injury; MAPc, multipotent adult progenitor cells; MP, machine perfusion; MSC, mesenchymal stromal cells; NMP, normothermic machine perfusion.
References
1. Hoogduijn, M, Montserrat, N, van der Laan, L, Dazzi, F, Perico, N, Kastrup, J, et al. The Emergence of Regenerative Medicine in Organ Transplantation: 1st European Cell Therapy and Organ Regeneration Section Meeting. Transpl Int (2020) 33(8):833–40. doi:10.1111/tri.13608
2. Eshmuminov, D, Becker, D, Bautista Borrego, L, Hefti, M, Schuler, MJ, Hagedorn, C, et al. An Integrated Perfusion Machine Preserves Injured Human Livers for 1 Week. Nat Biotechnol (2020) 38(2):189–98. doi:10.1038/s41587-019-0374-x
3. Martins, PN, Del Turco, S, and Gilbo, N. Organ Therapeutics During Ex-Situ Dynamic Preservation. A Look Into the Future. Eur J Transplant (2022) 1(1):63–78. doi:10.57603/EJT-010
4. Rowart, P, Erpicum, P, Detry, O, Weekers, L, Grégoire, C, Lechanteur, C, et al. Mesenchymal Stromal Cell Therapy in Ischemia/Reperfusion Injury. J Immunol Res (2015) 2015:602597–8. doi:10.1155/2015/602597
5. Eggenhofer, E, Luk, F, Dahlke, MH, and Hoogduijn, MJ. The Life and Fate of Mesenchymal Stem Cells. Front Immunol (2014) 5:148. doi:10.3389/fimmu.2014.00148
6. Vandermeulen, M, Mohamed-Wais, M, Erpicum, P, Delbouille, MH, Lechanteur, C, Briquet, A, et al. Infusion of Allogeneic Mesenchymal Stromal Cells After Liver Transplantation: A 5-Year Follow-Up. Liver Transplant (2022) 28(4):636–46. doi:10.1002/lt.26323
7. Podestà, MA, Remuzzi, G, and Casiraghi, F. Mesenchymal Stromal Cell Therapy in Solid Organ Transplantation. Front Immunol (2021) 11. doi:10.3389/fimmu.2020.618243
8. Casiraghi, F, Azzollini, N, Todeschini, M, Cavinato, RA, Cassis, P, Solini, S, et al. Localization of Mesenchymal Stromal Cells Dictates Their Immune or Proinflammatory Effects in Kidney Transplantation. Am J Transplant (2012) 12(9):2373–83. doi:10.1111/j.1600-6143.2012.04115.x
9. Perico, N, Casiraghi, F, Introna, M, Gotti, E, Todeschini, M, Cavinato, RA, et al. Autologous Mesenchymal Stromal Cells and Kidney Transplantation: A Pilot Study of Safety and Clinical Feasibility. Clin J Am Soc Nephrol (2011) 6(2):412–22. doi:10.2215/CJN.04950610
10. Pool, M, Eertman, T, Sierra Parraga, J, 't Hart, N, Roemeling-van Rhijn, M, Eijken, M, et al. Infusing Mesenchymal Stromal Cells into Porcine Kidneys During Normothermic Machine Perfusion: Intact MSCs Can Be Traced and Localised to Glomeruli. Int J Mol Sci (2019) 20(14):3607. doi:10.3390/ijms20143607
11. Mordant, P, Nakajima, D, Kalaf, R, Iskender, I, Maahs, L, Behrens, P, et al. Mesenchymal Stem Cell Treatment Is Associated With Decreased Perfusate Concentration of Interleukin-8 During Ex Vivo Perfusion of Donor Lungs After 18-Hour Preservation. J Heart Lung Transplant (2016) 35(10):1245–54. doi:10.1016/j.healun.2016.04.017
12. Laing, RW, Stubblefield, S, Wallace, L, Roobrouck, VD, Bhogal, RH, Schlegel, A, et al. The Delivery of Multipotent Adult Progenitor Cells to Extended Criteria Human Donor Livers Using Normothermic Machine Perfusion. Front Immunol (2020) 11:1226. doi:10.3389/fimmu.2020.01226
13. Verstegen, MMA, Mezzanotte, L, Ridwan, RY, Wang, K, de Haan, J, Schurink, IJ, et al. First Report on Ex Vivo Delivery of Paracrine Active Human Mesenchymal Stromal Cells to Liver Grafts During Machine Perfusion. Transplantation (2020) 104(1):e5–e7. doi:10.1097/TP.0000000000002986
14. Gregorini, M, Corradetti, V, Pattonieri, EF, Rocca, C, Milanesi, S, Peloso, A, et al. Perfusion of Isolated Rat Kidney With Mesenchymal Stromal Cells/Extracellular Vesicles Prevents Ischaemic Injury. J Cell Mol Med (2017) 21(12):3381–93. doi:10.1111/jcmm.13249
15. Thompson, ER, Bates, L, Ibrahim, IK, Sewpaul, A, Stenberg, B, McNeill, A, et al. Novel Delivery of Cellular Therapy to Reduce Ischemia Reperfusion Injury in Kidney Transplantation. Am J Transplant (2021) 21(4):1402–14. doi:10.1111/ajt.16100
16. Brasile, L, Henry, N, Orlando, G, and Stubenitsky, B. Potentiating Renal Regeneration Using Mesenchymal Stem Cells. Transplantation (2019) 103(2):307–13. doi:10.1097/TP.0000000000002455
17. Yang, L, Cao, H, Sun, D, Lin, L, Zheng, WP, Shen, ZY, et al. Normothermic Machine Perfusion Combined With Bone Marrow Mesenchymal Stem Cells Improves the Oxidative Stress Response and Mitochondrial Function in Rat Donation After Circulatory Death Livers. Stem Cell Dev (2020) 29(13):835–52. doi:10.1089/scd.2019.0301
18. Cao, H, Wu, L, Tian, X, Zheng, W, Yuan, M, Li, X, et al. HO-1/BMMSC Perfusion Using a Normothermic Machine Perfusion System Reduces the Acute Rejection of DCD Liver Transplantation by Regulating NKT Cell Co-Inhibitory Receptors in Rats. Stem Cell Res Ther (2021) 12(1):587. doi:10.1186/s13287-021-02647-5
19. Sun, D, Yang, L, Zheng, W, Cao, H, Wu, L, and Song, H. Protective Effects of Bone Marrow Mesenchymal Stem Cells (BMMSCS) Combined With Normothermic Machine Perfusion on Liver Grafts Donated After Circulatory Death Via Reducing the Ferroptosis of Hepatocytes. Med Sci Monitor (2021) 27:e930258. doi:10.12659/MSM.930258
20. Tian, X, Cao, H, Wu, L, Zheng, W, Yuan, M, Li, X, et al. Heme Oxygenase-1-Modified Bone Marrow Mesenchymal Stem Cells Combined With Normothermic Machine Perfusion Repairs Bile Duct Injury in a Rat Model of DCD Liver Transplantation Via Activation of Peribiliary Glands Through the Wnt Pathway. Stem Cell Int (2021) 2021:9935370–17. doi:10.1155/2021/9935370
21. Sierra Parraga, JM, Rozenberg, K, Eijken, M, Leuvenink, HG, Hunter, J, Merino, A, et al. Effects of Normothermic Machine Perfusion Conditions on Mesenchymal Stromal Cells. Front Immunol (2019) 10:765. doi:10.3389/fimmu.2019.00765
22. La Francesca, S, Ting, AE, Sakamoto, J, Rhudy, J, Bonenfant, NR, Borg, ZD, et al. Multipotent Adult Progenitor Cells Decrease Cold Ischemic Injury in Ex Vivo Perfused Human Lungs: An Initial Pilot and Feasibility Study. Transpl Res (2014) 3(1):19. doi:10.1186/2047-1440-3-19
23. Nakajima, D, Watanabe, Y, Ohsumi, A, Pipkin, M, Chen, M, Mordant, P, et al. Mesenchymal Stromal Cell Therapy during Ex Vivo Lung Perfusion Ameliorates Ischemia-Reperfusion Injury in Lung Transplantation. J Heart Lung Transplant (2019) 38(11):1214–23. doi:10.1016/j.healun.2019.07.006
24. Lohmann, S, Pool, MBF, Rozenberg, KM, Keller, AK, Moers, C, Møldrup, U, et al. Mesenchymal Stromal Cell Treatment of Donor Kidneys During Ex Vivo Normothermic Machine Perfusion: A Porcine Renal Autotransplantation Study. Am J Transplant (2021) 21(7):2348–59. doi:10.1111/ajt.16473
25. Casiraghi, F, Perico, N, Cortinovis, M, and Remuzzi, G. Mesenchymal Stromal Cells in Renal Transplantation: Opportunities and Challenges. Nat Rev Nephrol (2016) 12(4):241–53. doi:10.1038/nrneph.2016.7
26. Pool, MBF, Vos, J, Eijken, M, van Pel, M, Reinders, MEJ, Ploeg, RJ, et al. Treating Ischemically Damaged Porcine Kidneys With Human Bone Marrow- and Adipose Tissue-Derived Mesenchymal Stromal Cells During Ex Vivo Normothermic Machine Perfusion. Stem Cell Dev (2020) 29(20):1320–30. doi:10.1089/scd.2020.0024
27. Tetta, C, Ghigo, E, Silengo, L, Deregibus, MC, and Camussi, G. Extracellular Vesicles as an Emerging Mechanism of Cell-To-Cell Communication. Endocrine (2013) 44(1):11–9. doi:10.1007/s12020-012-9839-0
28. Lonati, C, Bassani, GA, Brambilla, D, Leonardi, P, Carlin, A, Maggioni, M, et al. Mesenchymal Stem Cell–Derived Extracellular Vesicles Improve the Molecular Phenotype of Isolated Rat Lungs During Ischemia/Reperfusion Injury. J Heart Lung Transplant (2019) 38(12):1306–16. doi:10.1016/j.healun.2019.08.016
29. Rigo, F, De Stefano, N, Navarro-Tableros, V, David, E, Rizza, G, Catalano, G, et al. Extracellular Vesicles From Human Liver Stem Cells Reduce Injury in an Ex Vivo Normothermic Hypoxic Rat Liver Perfusion Model. Transplantation (2018) 102(5):e205–10. doi:10.1097/TP.0000000000002123
30. De Stefano, N, Navarro-Tableros, V, Roggio, D, Calleri, A, Rigo, F, David, E, et al. Human Liver Stem Cell-Derived Extracellular Vesicles Reduce Injury in a Model of Normothermic Machine Perfusion of Rat Livers Previously Exposed to a Prolonged Warm Ischemia. Transpl Int (2021) 34(9):1607–17. doi:10.1111/tri.13980
31. Gennai, S, Monsel, A, Hao, Q, Park, J, Matthay, MA, and Lee, JW. Microvesicles Derived From Human Mesenchymal Stem Cells Restore Alveolar Fluid Clearance in Human Lungs Rejected for Transplantation. Am J Transpl (2015) 15(9):2404–12. doi:10.1111/ajt.13271
32. Rampino, T, Gregorini, M, Germinario, G, Pattonieri, EF, Erasmi, F, Grignano, MA, et al. Extracellular Vesicles Derived From Mesenchymal Stromal Cells Delivered During Hypothermic Oxygenated Machine Perfusion Repair Ischemic/Reperfusion Damage of Kidneys From Extended Criteria Donors. Biology (Basel) (2022) 11(3):350. doi:10.3390/biology11030350
33. Blondeel, J, Gilbo, N, De Bondt, S, and Monbaliu, D. Stem Cell Derived Extracellular Vesicles to Alleviate Ischemia-Reperfusion Injury of Transplantable Organs. A Systematic Review. Stem Cell Rev Rep. (2023) 19:2225–50. doi:10.1007/s12015-023-10573-7
34. Stone, ML, Zhao, Y, Robert Smith, J, Weiss, ML, Kron, IL, Laubach, VE, et al. Mesenchymal Stromal Cell-Derived Extracellular Vesicles Attenuate Lung Ischemia-Reperfusion Injury and Enhance Reconditioning of Donor Lungs After Circulatory Death. Respir Res (2017) 18(1):212. doi:10.1186/s12931-017-0704-9
35. Herrera Sanchez, MB, Previdi, S, Bruno, S, Fonsato, V, Deregibus, MC, Kholia, S, et al. Extracellular Vesicles From Human Liver Stem Cells Restore Argininosuccinate Synthase Deficiency. Stem Cell Res Ther (2017) 8(1):176. doi:10.1186/s13287-017-0628-9
36. McAuley, DF, Curley, GF, Hamid, UI, Laffey, JG, Abbott, J, McKenna, DH, et al. Clinical Grade Allogeneic Human Mesenchymal Stem Cells Restore Alveolar Fluid Clearance in Human Lungs Rejected for Transplantation. Am J Physiology-Lung Cell Mol Physiol (2014) 306(9):L809–L815. doi:10.1152/ajplung.00358.2013
37. Martens, A, Ordies, S, Vanaudenaerde, BM, Verleden, SE, Vos, R, Van Raemdonck, DE, et al. Immunoregulatory Effects of Multipotent Adult Progenitor Cells in a Porcine Ex Vivo Lung Perfusion Model. Stem Cell Res Ther (2017) 8(1):159. doi:10.1186/s13287-017-0603-5
38. Sasajima, H, Miyagi, S, Kakizaki, Y, Kamei, T, Unno, M, Satomi, S, et al. Cytoprotective Effects of Mesenchymal Stem Cells During Liver Transplantation From Donors After Cardiac Death in Rats. Transpl Proc (2018) 50(9):2815–20. doi:10.1016/j.transproceed.2018.02.180
39. Yang, L, Cao, H, Sun, D, Hou, B, Lin, L, Shen, ZY, et al. Bone Marrow Mesenchymal Stem Cells Combine With Normothermic Machine Perfusion to Improve Rat Donor Liver Quality—The Important Role of Hepatic Microcirculation in Donation After Circulatory Death. Cell Tissue Res (2020) 381(2):239–54. doi:10.1007/s00441-020-03202-z
Keywords: organ repair, organ regeneration, machine perfusion, dynamic preservation, organ preservation, mesenchymal stromal cell, extracellular vesicles
Citation: Gilbo N, Blondeel J, Pirenne J, Romagnoli R, Camussi G and Monbaliu D (2023) Organ Repair and Regeneration During Ex Situ Dynamic Preservation: The Future is Nano. Transpl Int 36:11947. doi: 10.3389/ti.2023.11947
Received: 22 August 2023; Accepted: 24 October 2023;
Published: 10 November 2023.
Copyright © 2023 Gilbo, Blondeel, Pirenne, Romagnoli, Camussi and Monbaliu. This is an open-access article distributed under the terms of the Creative Commons Attribution License (CC BY). The use, distribution or reproduction in other forums is permitted, provided the original author(s) and the copyright owner(s) are credited and that the original publication in this journal is cited, in accordance with accepted academic practice. No use, distribution or reproduction is permitted which does not comply with these terms.
*Correspondence: Nicholas Gilbo, bmljaG9sYXMuZ2lsYm9AY2h1bGllZ2UuYmU=