- 1Institute of Transfusion Medicine and Transplant Engineering, Hannover Medical School, Hannover, Germany
- 2German Center for Infection Research (DZIF), Braunschweig, Germany
- 3Clinic for Hepatopancreaticobiliary Surgery and Transplantation, First Affiliated Hospital, University of Science and Technology of China, Hefei, China
- 4Department of Gastroenterology, Hepatology, Infectious Diseases and Endocrinology, Hannover Medical School, Hannover, Germany
- 5TWINCORE, Centre for Experimental and Clinical Infection Research, A Joint Venture Between the Helmholtz Centre for Infection Research and the Hannover Medical School, Hannover, Germany
- 6Department of Pediatric Hematology and Oncology, Hannover Medical School, Hannover, Germany
Infectious complications, including widespread human cytomegalovirus (CMV) disease, frequently occur after hematopoietic stem cell and solid organ transplantation due to immunosuppressive treatment causing impairment of T-cell immunity. Therefore, in-depth analysis of the impact of immunosuppressants on antiviral T cells is needed. We analyzed the impact of mTOR inhibitors sirolimus (SIR/S) and everolimus (EVR/E), calcineurin inhibitor tacrolimus (TAC/T), purine synthesis inhibitor mycophenolic acid (MPA/M), glucocorticoid prednisolone (PRE/P) and common double (T+S/E/M/P) and triple (T+S/E/M+P) combinations on antiviral T-cell functionality. T-cell activation and effector molecule production upon antigenic stimulation was impaired in presence of T+P and triple combinations. SIR, EVR and MPA exclusively inhibited T-cell proliferation, TAC inhibited activation and cytokine production and PRE inhibited various aspects of T-cell functionality including cytotoxicity. This was reflected in an in vitro infection model, where elimination of CMV-infected human fibroblasts by CMV-specific T cells was reduced in presence of PRE and all triple combinations. CMV-specific memory T cells were inhibited by TAC and PRE, which was also reflected with double (T+P) and triple combinations. EBV- and SARS-CoV-2-specific T cells were similarly affected. These results highlight the need to optimize immune monitoring to identify patients who may benefit from individually tailored immunosuppression.
Introduction
Infectious complications following hematopoietic stem cell and solid organ transplantation (HSCT, SOT) are common due to immunosuppressive treatment for prevention of graft-versus-host disease (GvHD) and allograft rejection. Persistent herpesviruses, such as human cytomegalovirus (CMV), are particularly frequent pathogens. An association between CMV infection/reactivation, the development and severity of GvHD and graft injury has been described in several clinical studies of HSCT and SOT [1–3]. Risk factors include in vivo or in vitro T-cell depletion, HLA-mismatched HSCT, the intensity of immunosuppression, and - in the setting of SOT - the type of transplanted organ [4, 5]. Moreover, CMV-seronegative (CMV-) SOT recipients of a graft from a CMV-seropositive (CMV+) donor (D+/R-) are at high-risk, with incidences of CMV disease up to 50% [6, 7].
The two main strategies to prevent CMV infection or disease in transplant patients are antiviral prophylaxis and preemptive therapy. Especially in high-risk SOT recipients, the most common strategy is antiviral prophylaxis, which is applied for up to 12 months after transplantation. Despite effectiveness of antiviral prophylaxis, side-effects such as nephrotoxicity or bone marrow suppression can result in discontinuation of prophylaxis and late-onset CMV disease after end of prophylaxis [8]. In addition, drug resistances can limit the efficacy of antiviral drugs [9–11]. In 2017/2018, letermovir was approved for prophylaxis after HSCT. In a recent phase III clinical trial comparing valganciclovir and letermovir prophylaxis in kidney transplant recipients (D+/R-), similar incidences of CMV disease were observed in both groups, with fewer side effects in patients receiving letermovir [12]. Preemptive treatment comprises of regular monitoring of viral load, allowing rapid therapy initiation upon detection of an increase. By this, progression to CMV disease can be prevented at an early stage of virus replication while at the same time, myelotoxicity associated with antiviral drugs is reduced [4, 13].
Mechanistically, a relationship between the magnitude of T-cell responses, especially by CD8+ T cells, CMV clearance and restoration of antiviral immunity was found [14]. In line, late-onset CMV disease and mortality have been correlated with the absence of CMV-specific T cells [7, 15, 16]. In recent studies, lower incidence of late-onset CMV disease was observed in liver transplant patients receiving preemptive therapy compared to prophylaxis and this was hypothesized to be due to enhanced CMV-specific T-cell immunity [17, 18]. Assuming that preemptive treatment potentially allows early immune reconstitution and the establishment of cellular antiviral immunity due to controlled low-level CMV replication, the restoration of endogenous antiviral immunity may be sensitively disrupted or delayed by immunosuppressive therapy.
Appropriate T-cell function relies on a variety of aspects and these are targeted via different mechanisms by post-transplant immunosuppressants. Reduction of immunosuppression as tolerated is an alternative option to restore a functional antiviral immune response. CMV disease after SOT typically occurs after 30–90 days [19–22]. At this point, patients are mostly treated by maintenance therapy, e.g., triple combinations usually consisting of a calcineurin inhibitor (CNI, e.g., tacrolimus) and a corticosteroid (e.g., prednisolone), supplemented with a purine synthesis inhibitor (e.g., mycophenolate mofetil, MMF) or a mechanistic target of rapamycin inhibitor (mTORi, e.g., sirolimus, everolimus). Of note, different clinical studies including the ATHENA study showed that the use of an mTORi was associated with lower CMV infection incidences compared to MMF-based regimens [23–29].
To support the restoration of antiviral immunity in SOT recipients and thereby reduce the risk of viral infection or reactivation, in-depth analysis of the effects of immunosuppressive drugs and combination regimens on antiviral T cells is required. In this study, we analyzed the impact of mTORi sirolimus (S/SIR) and everolimus (E/EVR), the CNI tacrolimus (T/TAC), the active metabolite of the purine synthesis inhibitor MMF - mycophenolic acid (M/MPA) - and the glucocorticoid prednisolone (P/PRE) [30] on CMV-specific T cells. As combination regimens are often used due to synergistic effects and lower single doses thereby minimizing toxicities, we included double (T+S/E/M/P) and triple combinations (T+S/E/M+P) in our study. Detailed assessment of CMV-specific T-cell responses in vitro revealed that SIR, EVR and MPA selectively inhibited T-cell proliferation, TAC slightly inhibited different aspects of CMV-specific T-cell functionality and PRE had broad inhibitory effects. Severe impairment was observed with triple combinations, and this could not be compensated by mTORi harboring partial beneficial effects on CMV-specific T cells. In line with that, T+P impaired antiviral T-cell functionality more strongly than T+S/E/M. These results, including evidence of a similar effect on T cells against EBV and SARS-CoV-2, highlight the need to optimize monitoring of immunocompromised patients or patients with viral infection/reactivation by determining antigen-specific T-cell functionality to further individualize immunosuppressive therapy.
Materials and Methods
For description of methods please see Supplementary Material.
Results
PRE and Triple Combinations Reduce Antiviral T-cell Activation and Effector Molecule Production
To analyze the impact of the different immunosuppressants on the reactivity of CMV-specific memory T cells, PBMCs were isolated from CMV+ healthy donors and subjected to IFN-γ ELISpot assay using CMV_pp65 overlapping peptide pool for restimulation in absence or presence of immunosuppressants (Figure 1A; Supplementary Figure S1A). To account for inter-individual differences (Supplementary Figure S1A), the data were normalized to values obtained from untreated (UT; stimulated but not treated with immunosuppressants) controls (Figure 1A). The frequencies of reactive CMV-specific T cells were significantly decreased upon treatment with PRE and T+S/E/M+P. SIR and TAC slightly reduced detectable CMV-specific T-cell response. In addition to the number of spots, correlating to the number of reactive CMV-specific memory T cells, average spot intensities and sizes were significantly reduced in presence of triple combinations. Since all triple combinations severely impaired memory T-cell reactivity, we analyzed the impact of double combination of immunosuppressants (T+S/E/P) on the reactivity of CMV-specific T cells in a small donor cohort, revealing significantly reduced number of spots in the presence of T+P (Supplementary Figure S1B). Of note, EBV- and SARS-CoV-2-specific T-cell responses were similarly affected by immunosuppressive treatment (Supplementary Figures S1C, S1D), with SARS-CoV-2-specific T cells being more susceptible.
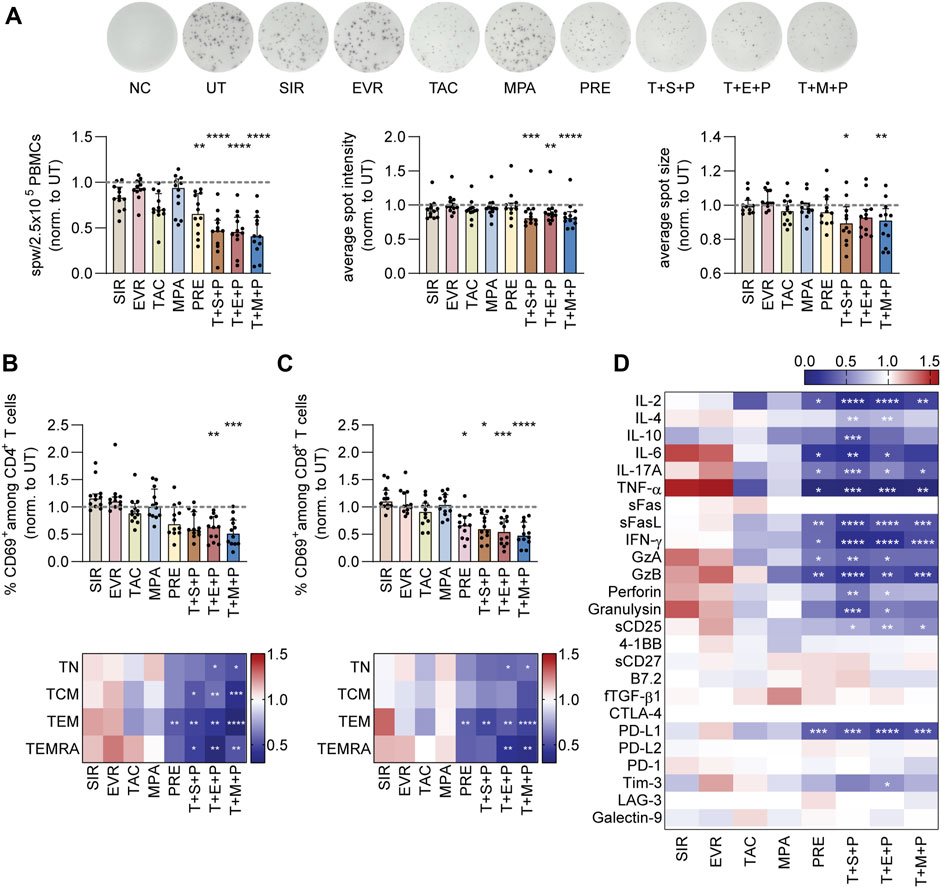
Figure 1. IFN-γ ELISpot, activation and cytokine secretion of CMV_pp65-stimulated PBMCs under immunosuppression. (A) PBMCs were isolated from CMV+ donors, rested overnight and stimulated with CMV_pp65 on day 1 in presence and absence of indicated immunosuppressants on IFN-γ ELISpot plates. After 24h, secreted IFN-γ was detected. Representative and summarized IFN-γ ELISpot results shown as spots per well (spw)/2.5 × 105 PBMCs, spot intensity and spot size, normalized to untreated control (UT). (B–D) PBMCs were isolated from CMV+ donors, rested overnight and stimulated with CMV_pp65 on day 1 in presence and absence of indicated immunosuppressants. After 24 h cells were harvested for flow cytometric analysis and cell culture supernatants were collected for multiplex analysis. (B) Frequencies of CD69+ cells among CD4+ T cells (bar graph) and memory CD4+ T-cell subsets (heat map), normalized to UT. (C) Summarized frequencies of CD69+ cells among CD8+ T cells and memory CD8+ T-cell subsets (heat map), normalized to UT. (B–C) Bar graphs show median and interquartile range Q1-Q3, each symbol represents data from one donor (n = 12). (B–D) Heat maps show median values, normalized to UT (n = 12). Statistical significance (in comparison to UT) was calculated using (A–C) Friedman test followed by Dunn’s multiple comparison and (D) 2way ANOVA followed by Dunnett’s multiple comparison. *p < 0.05, **p < 0.01, ***p < 0.001, ****p < 0.0001. NC negative control (unstimulated), UT untreated, SIR/S sirolimus, EVR/E everolimus, TAC/T tacrolimus, MPA/M mycophenolic acid, PRE/P prednisolone, TN naïve T cells (CD45RA+/CD62L+), TCM central memory T cells (CD45RA−/CD62L+), TEM effector memory T cell (CD45RA−/CD62L−), TEMRA effector memory T cell re-expressing CD45RA (CD45RA+/CD62L−).
To gain more insights into the affected T-cell populations, PBMCs from CMV+ donors were stimulated with CMV_pp65 for 24 h in absence or presence of immunosuppressants, followed by analysis of CD69 expression as indicator of activation (Figures 1B, C; Supplementary Figures S2A, S2B). Frequencies of CD69+ T cells after antigenic stimulation varied between donors and T-cell subsets (Fig. S2b) and were normalized to values obtained from UT controls (Figures 1B, C). Activation of CD4+ T cells by CMV_pp65 was significantly reduced in presence of T+E+P and T+M+P (Figure 1B). Of note, within the different CD4+ memory T-cell subsets, activation was significantly reduced in presence of all triple combinations. Moreover, in presence of PRE, CD4+ effector memory T cells (TEM, CD45RA−CD62L−) were significantly less activated. Slightly reduced CD69 expression on CD4+ central memory T cells (TCM, CD45RA−CD62L+) and TEM was detected in presence of TAC and MPA. Similarly, activation of CD8+ T cells by CMV_pp65 was significantly reduced in presence of triple combinations and PRE (Figure 1C). The main affected CD8+ memory T-cell subsets were TEM and effector memory T cells re-expressing CD45RA (TEMRA, CD45RA+CD62L−). In line with the effect of PRE on CD4+ T cells, significant reduction of CD69 expression among CD8+ TEM was observed in presence of PRE. Of note, slightly increased activation of CD4+ and CD8+ TEM and TEMRA were observed in presence of SIR and EVR. In a small donor cohort, T-cell activation was analyzed after antigenic restimulation in presence of double combinations of immunosuppressive drugs (T+S/E/M/P) and found to be slightly reduced in presence of T+P (Supplementary Figure S2C). Similar tendencies were observed for EBV- and SARS-CoV-2-specific T-cell responses (Supplementary Figures S2D, S2E). The activation of CD4+ and CD8+ SARS-CoV-2-specific T cells was significantly reduced in presence of T+P (Supplementary Figure S2E).
For a more comprehensive overview on the impact of immunosuppression on the production of cytotoxic mediators, multiplex cytokine assays were performed with supernatants of CMV_pp65-stimulated PBMCs (Figure 1D; Supplementary Figure S3). The raw values (Supplementary Figure S3) were normalized to the values obtained from UT controls (Figure 1D). While SIR and EVR induced slightly higher concentrations of, e.g., IL-6 and TNF-α, the secretion of pro-inflammatory effector molecules was slightly reduced in presence of TAC, MPA and significantly reduced in presence of PRE and T+S/E/M+P. To confirm antiviral T cells as source of the measured effector molecules, we analyzed the culture supernatants of T-cell-depleted PBMCs (Supplementary Figure S4A) stimulated with CMV_pp65 (Supplementary Figure S4B). Effector molecules such as, e.g., IL-2, TNF-α and IFN-γ were upregulated in PBMCs but not T-cell-depleted PBMCs after restimulation. Analysis of the effects of dual immunosuppression (T+S/E/M/P) on the secretion of effector molecules (Supplementary Figure S5) revealed significantly reduced secretion of different effector molecules by PBMCs after stimulation with CMV_pp65 in presence of T+P (Supplementary Figure S5B). Overall, similar patterns were observed after stimulation under the influence of immunosuppression for EBV- and SARS-CoV-2-specific T cells (Supplementary Figure S5B).
Taken together, PRE and triple combinations significantly reduced activation and effector molecule secretion of CMV-specific T cells. While all CD4+ memory T-cell subsets were affected by triple combinations, effects on CD8+ T cells were mainly attributed to TEM. Among the double combinations, T+P had the most pronounced impact on antiviral T cells. Moreover, immunosuppressive treatment resulted in impaired T-cell responses towards EBV and SARS-CoV-2.
TAC, MPA, PRE and Triple Combinations Inhibit Cytokine Production by CD4+ and CD8+ T-cell Subsets Upon Antigenic Stimulation
To further discriminate between CD4+ and CD8+ T cells, we performed intracellular cytokine staining of PBMCs stimulated with CMV_pp65 in absence or presence of immunosuppressants and triple combinations thereof (Figure 2; Supplementary Figure S6). The data were normalized to values obtained from UT controls (Figure 2; Supplementary Figure S6B, S6C). Frequencies of IFN-γ+, TNF-α+, and IL-2+ cells within CD4+ T cells were significantly reduced by triple combinations (Figure 2A). Moreover, IFN-γ+ cells within CD4+ T cells were significantly reduced by TAC and the frequencies of IL-2+ cells within CD4+ T cells were significantly reduced by TAC and PRE. In contrast, frequencies of IFN-γ+ cells within CD8+ T cells were reduced by TAC, whereas triple combinations had no impact (Figure 2B). TNF-α production by CD8+ T cells was slightly reduced in presence of triple combinations, while IL-2 production was significantly reduced by TAC, PRE and triple combinations. Inhibitory effects on CD4+ T cells were primarily focused on TEM (IFN-γ, TNF-α, IL-2) and TEMRA (TNF-α) (Figure 2C). Moreover, significantly reduced IFN-γ and IL-2 production by CD4+ TEM was observed in presence of TAC. Among CD8+ memory T-cell subsets, reduction of TNF-α and IL-2 production was comparable to CD4+ T-cell subsets.
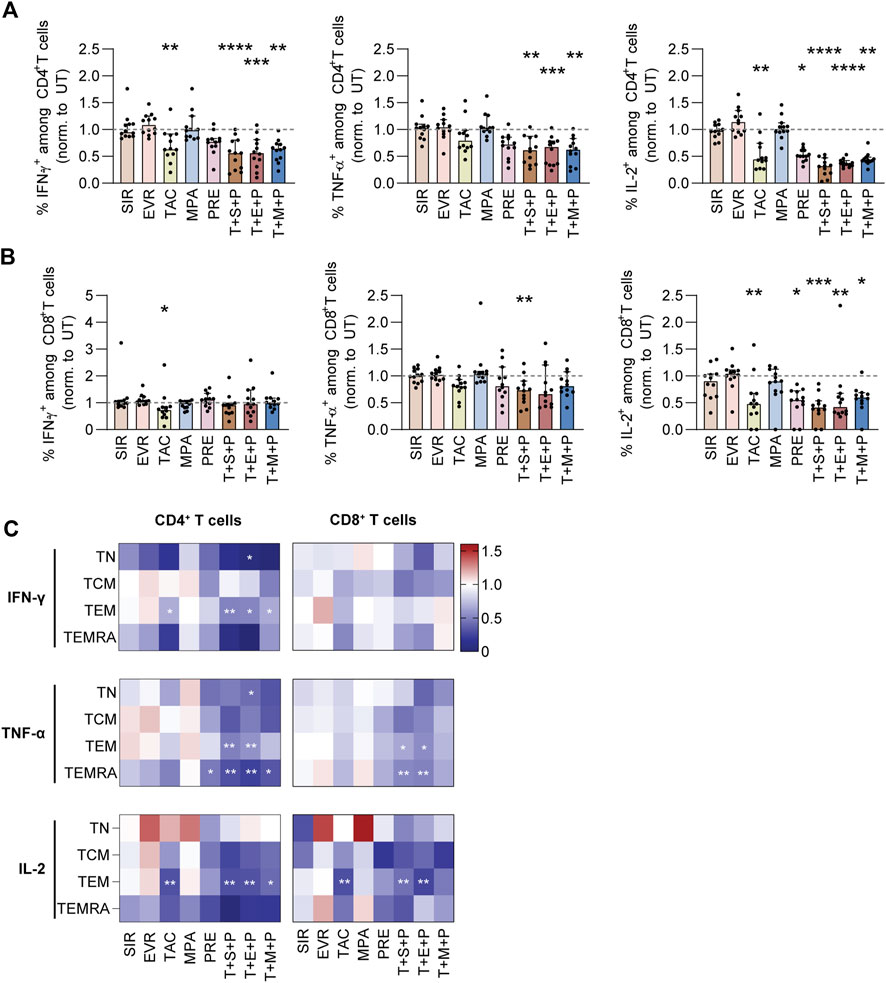
Figure 2. Cytokine profiling of CMV_pp65 stimulated PBMCs under immunosuppression. PBMCs were isolated from CMV+ donors, rested overnight and stimulated with CMV_pp65 on day 1 in presence and absence of indicated immunosuppressants. After 24h, intracellular cytokine production was detected using multicolor flow cytometry and secreted cytotoxic mediators were measured using a flow cytometry-based multiplex assay (LEGENDplex). (A,B) Bar graphs summarize frequencies of IFN-γ+, TNF-α+ and IL-2+ cells among (A) CD4+ and (B) CD8+ T cells. The data are shown as median and interquartile range Q1-Q3 (n = 12). (C) Heat maps summarize frequencies of IFN-γ+, TNF-α+ and IL-2+ cells among CD4+ (left) and CD8+ (right) memory T-cell subsets, normalized to untreated control (UT). Data are shown as median (n = 12). Statistical significance (in comparison to UT) was calculated using Friedman test followed by Dunn’s multiple comparison. *p < 0.05, **p < 0.01, ***p < 0.001, ****p < 0.0001. NC negative control (unstimulated), UT untreated, SIR/S sirolimus, EVR/E everolimus, TAC/T tacrolimus, MPA/M mycophenolic acid, PRE/P prednisolone, TN naïve T cells (CD45RA+/CD62L+), TCM central memory T cells (CD45RA−/CD62L+), TEM effector memory T cell (CD45RA−/CD62L−), TEMRA effector memory T cell re-expressing CD45RA (CD45RA+/CD62L−).
Taken together, SIR and EVR mostly preserved the release of pro-inflammatory cytokines by CMV-specific memory T cells, which is in contrast to TAC, PRE and triple combinations. Moreover, impairment of IFN-γ production by immunosuppressive treatment was mostly restricted to CD4+ T cells, while IL-2 production was strongly reduced in CD4+ and CD8+ T cells.
MPA and Triple Combinations Inhibit CMV-specific T-cell Proliferation
To analyze the impact of immunosuppression on proliferation of CMV-specific memory T cells, we isolated CMV_pp65-specific T cells by IFN-γ cytokine secretion assay (CSA). The cells were labeled with CellTrace Violet (CTV) proliferation dye and expanded on irradiated autologous PBMCs (feeder cells) in presence or absence of immunosuppressants and combinations thereof for 4 days (Figure 3; Supplementary Figure S7). The data were normalized to values obtained from untreated controls (Figure 3; Supplementary Figures S7B, S7C). Presence of MPA, T+S+P and T+E+P resulted in significantly reduced proliferation of T cells (Figure 3A). Among CD4+ T-cell subsets, treatment with T+E+P and T+M+P resulted in significantly reduced proliferation of TEM (Figure 3B). Proliferation of CD8+ TEM was significantly reduced in presence of all triple combinations and CD8+ TCM and TEMRA proliferation was significantly reduced in presence of T+E+P.
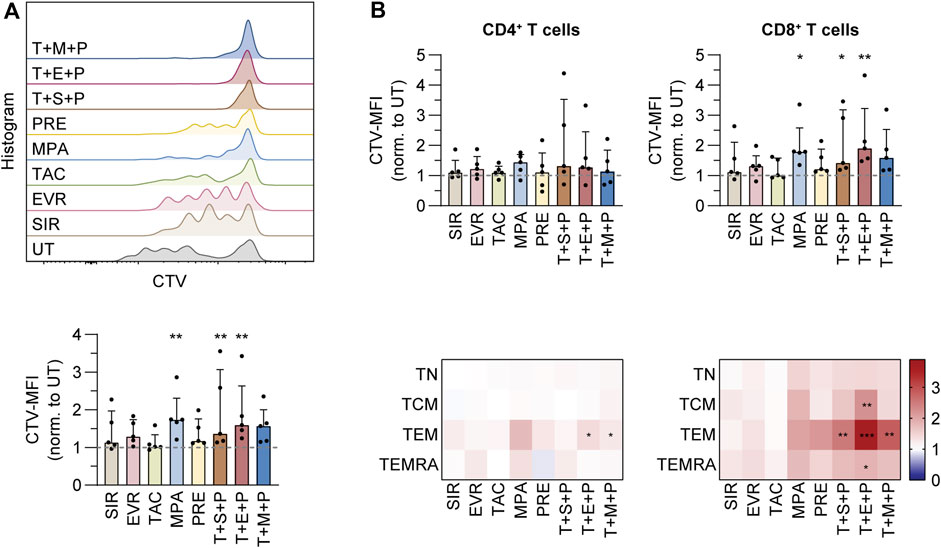
Figure 3. Proliferation analysis of purified CMV-specific T cells under immunosuppression. PBMCs were isolated from CMV+ donors, labeled with CellTrace™ Violet (CTV) and rested overnight, followed by magnetic enrichment of CMV-specific T cells using Cytokine Secretion Assay and CMV_pp65 stimulation. Afterwards, the T cells were expanded on irradiated autologous PBMCs in presence or absence of indicated immunosuppressants, followed by flow cytometric analysis. (A) Histograms showing CTV signals of CMV-specific T cells from a representative donor after 5 days of expansion (upper graph). Bar graph shows summarized mean fluorescent intensities (MFIs) of CTV from proliferating T cells on day 5, normalized to untreated control (UT) (lower graph). (B) Bar graphs show summarized MFIs of CTV from proliferating CD4+ (left) and CD8+ (right) T cells on day 5, normalized to untreated control (UT) (upper). Heat maps show summarized MFIs of CTV from proliferating CD4+ (left) and CD8+ (right) memory T-cell subsets on day 5, normalized to untreated control (UT) (lower). (A,B) Bar graphs show median and interquartile range Q1-Q3, each symbol represents data from one donor (n = 5). Heat maps show data as median values (n = 5). Statistical significance (in comparison to UT) was calculated for each T-cell subset using Friedman test followed by Dunn’s multiple comparison. *p < 0.05, **p < 0.01, ***p < 0.001. UT untreated, SIR/S sirolimus, EVR/E everolimus, TAC/T tacrolimus, MPA/M mycophenolic acid, PRE/P prednisolone, TN naïve T cells (CD45RA+/CD62L+), TCM central memory T cells (CD45RA−/CD62L+), TEM effector memory T cell (CD45RA−/CD62L−), TEMRA effector memory T cell re-expressing CD45RA (CD45RA+/CD62L−).
Taken together, treatment with MPA and triple combinations resulted in significantly impaired proliferation of CMV-specific T cells.
PRE and Triple Combinations Impair CMV-specific T-cell Activation and Cytotoxicity
For measurement of the cytotoxic capacity of CMV-specific T cells under immunosuppression, CMV_pp65-specific memory T cells were isolated as described before and expanded on feeder cells for 12 days, followed by co-culture with CTV-labeled autologous CMV_pp65-loaded PBMCs in presence or absence of immunosuppressants. Unloaded PBMCs served as negative control. After 4 h, the cells were harvested for flow cytometric analysis of target cell death and T-cell activation (Figure 4; Supplementary Figure S8). The data were normalized to values obtained from UT controls (Figure 4; Supplementary Figure S8). While no unspecific cytotoxicity of T cells co-cultured with unloaded PBMCs was observed (Supplementary Figures S8A, S8B), frequencies of dead (7-AAD+) PBMCs were increased when peptide pool-loaded and co-cultured with T cells, and this effect was dose-dependent (Figure 4A; Supplementary Figure S8B). At both ratios, T+M+P resulted in reduced cytotoxicity of T cells towards loaded PBMCs. Moreover, at the 5:1 ratio, treatment with T+E+P significantly reduced cytotoxicity. Slightly reduced cytotoxicity was observed in presence of MPA, PRE and triple combinations at both ratios. In line, frequencies of CD69-expressing CD8+ T cells and memory subsets were significantly reduced under treatment with PRE (5:1), T+S+P (1:1 and 5:1) and T+E+P (1:1 and 5:1) (Figure 4B; Supplementary Figures S8C, S8D).
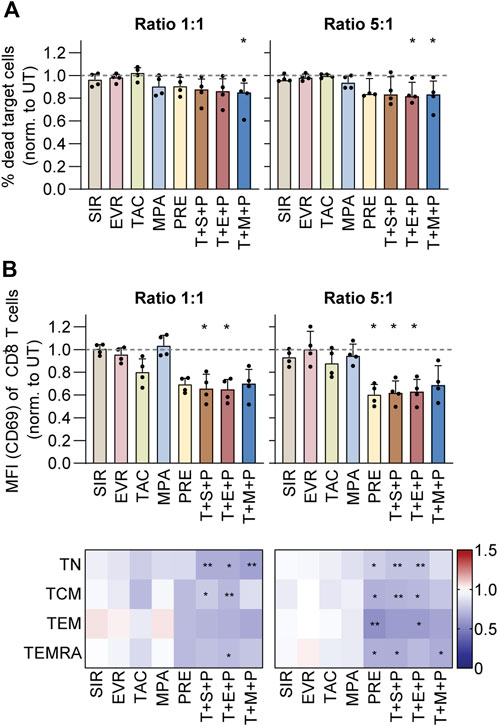
Figure 4. Cytotoxic capacity and activation of CMV-specific T cells under immunosuppression. PBMCs were isolated from CMV+ donors and rested overnight, followed by magnetic enrichment of CMV-specific T cells using Cytokine Secretion Assay and CMV_pp65 stimulation. The T cells were expanded on irradiated autologous PBMCs for 11 days and subsequently co-cultured with CTV-labeled autologous CMV_pp65-loaded PBMCs in different effector-to-target ratios and in presence or absence of indicated immunosuppressants. After 4 h their cytotoxic capacity was analyzed using flow cytometry. Unloaded PBMCs served as negative control. (A) Bar graphs show the frequencies of dead (7-AAD+) target cells, normalized to untreated control (UT). (B) Bar graphs show the CD69 expression (MFI) among CD8+ T cells, normalized to untreated control (UT) (upper). Heat maps show the CD69 expression (MFI) among CD8+ memory T-cell subsets, normalized to untreated control (UT) (lower). (A,B) Bar graphs show median and interquartile range Q1-Q3, each symbol represents data from one donor (n = 4). Heat maps show data as median values (n = 5). Statistical significance (in comparison to UT) was calculated using Friedman test followed by Dunn’s multiple comparison. *p < 0.05, **p < 0.01, ***p < 0.001. UT untreated, SIR/S sirolimus, EVR/E everolimus, TAC/T tacrolimus, MPA/M mycophenolic acid, PRE/P prednisolone, TN naïve T cells (CD45RA+/CD62L+), TCM central memory T cells (CD45RA−/CD62L+), TEM effector memory T cell (CD45RA−/CD62L−), TEMRA effector memory T cell re-expressing CD45RA (CD45RA+/CD62L−).
Taken together, PRE and triple combinations resulted in comparable inhibition of cytotoxicity and activation after co-culture with autologous CMV_pp65-loaded PBMCs.
PRE and Triple Combinations Inhibit Real-Time Cytotoxicity Towards CMV-Infected Fibroblasts
To evaluate long-term effects of immunosuppressive treatment, we measured real-time cytotoxicity of CMV-specific T cells towards partially HLA-matched CMV-infected or CMV_pp65-loaded human foreskin fibroblasts (HFF) using xCelligence Real Time Cell Analyzer (RTCA) (Figure 5). Fluorescence microscopy confirmed the successful infection, indicated by expression of a green fluorescent protein (GFP) signal in the CMV-infected cells (Figure 5A). Direct comparison of growth curves for HFF cells only and HFF cells plus T cells showed reduced cell indices in presence of T cells for all three target cell conditions (Figure 5B). PRE and all triple combinations markedly inhibited cytotoxicity as indicated by higher cell indices. Area under the curve (AUC) values (Supplementary Figure S9A) were normalized to the AUC values obtained from the respective UT control (Figure 5C). While slightly higher normalized AUC values were measured in co-cultures treated with PRE or triple combinations, these effects were markedly stronger in co-cultures with CMV-infected HFF cells compared to the other two conditions.
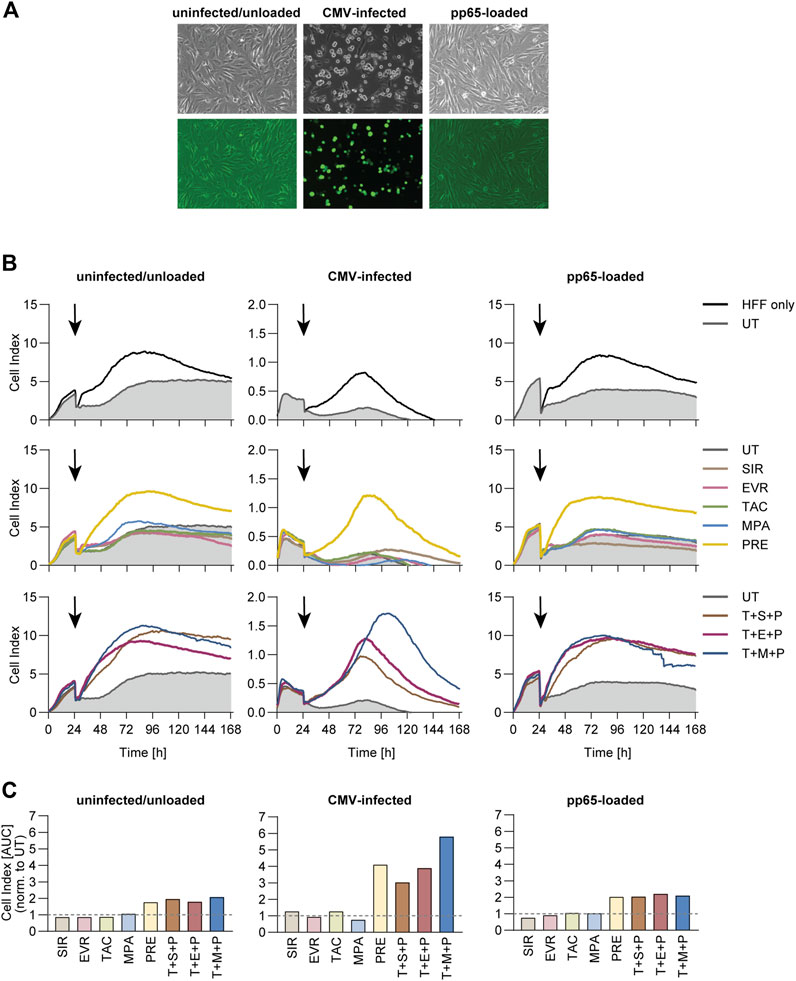
Figure 5. Cytotoxic capacity of CMV-specific T cells towards CMV-infected fibroblasts under immunosuppression. PBMCs were isolated from CMV+ donors and rested overnight, followed by magnetic enrichment of CMV-specific T cells using Cytokine Secretion Assay and CMV_pp65 stimulation. The T cells were expanded on irradiated autologous PBMCs for 11 days and subsequently co-cultured with uninfected, CMV-infected or CMV_pp65-loaded Human Foreskin Fibroblasts (HFF) in an effector-to-target ratio of 1:1 and in presence or absence of indicated immunosuppressants for 7 days using an xCELLigence RTCA S16 Real Time Cell Analyzer. (A) Microscopic image of the different target cells prior to co-culture. (B) Realtime impedance-based growth curves of HFF cells cultured alone (HFF cells only) or together with CMV-specific T cells in presence or absence of indicated immunosuppressants. Black arrows indicate time of T-cell addition. (C) Bar graphs display the AUC of growth curves shown in (B), normalized to untreated control (UT). UT untreated, SIR/S sirolimus, EVR/E everolimus, TAC/T tacrolimus, MPA/M mycophenolic acid, PRE/P prednisolone.
Supernatants of these co-cultures were analyzed with respect to secreted cytotoxic mediators (Supplementary Figure S9B). Specific upregulation of IL-6, sFasL and IFN-γ was observed in co-cultures with CMV-infected HFF cells and this was slightly reduced in presence of PRE and triple combinations.
Taken together, CMV-specific T cells were unable to eliminate CMV-infected fibroblasts under immunosuppression with PRE or triple combinations, and this was accompanied by decreased effector molecule production.
Summary
Spider web graphs including all assay read-outs were created for each immunosuppressant in comparison to UT controls (Figure 6). While all triple combinations conferred homogenously and broadly attenuated CMV-specific memory T cells, divergent effects of single immunosuppressants were observed. SIR and EVR slightly inhibited T-cell proliferation while mostly sparing activation and cytokine secretion. MPA selectively inhibited T-cell proliferation more profoundly. In contrast, TAC slightly inhibited different aspects of CMV-specific T-cell functionality and PRE had broad inhibitory effects on CMV-specific T cells.
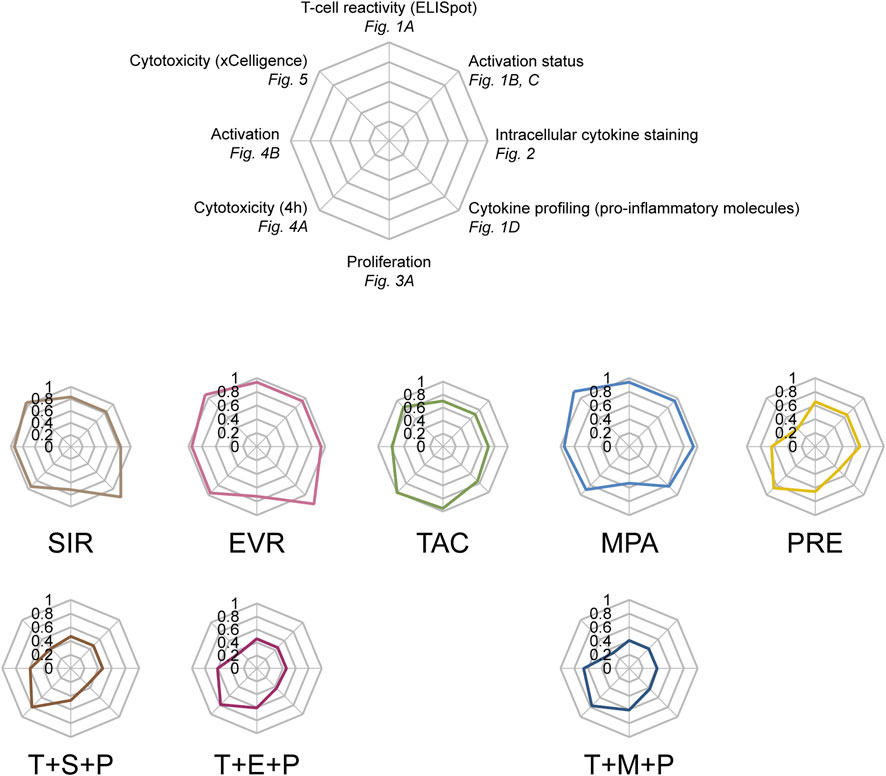
Figure 6. In vitro profiles of commonly used post-transplant immunosuppressants in context of antiviral T-cell immunity. Spider web graphs summarizing the impact of the respective immunosuppressants on CMV-specific T cells as measured by the indicated assays and in comparison to untreated controls. Values used for the diagrams are (clockwise starting from the top): IFN-γ ELISpot (spot numbers), activation status (frequencies of CD69+ CD4+ and CD8+ T cells), intracellular cytokine staining (cumulative frequencies of IFN-γ+/TNF-α+/IL-2+ CD4+ and CD8+ T cells), multiplex cytokine profiling (concentrations of pro-inflammatory molecules), proliferation (CD3+ T cells), cytotoxicity (4 h) (frequencies of dead target cells), activation (4 h) (CD69-MFIs of CD8+ T cells), realtime cytotoxicity (area under curve). UT untreated, SIR/S sirolimus, EVR/E everolimus, TAC/T tacrolimus, MPA/M mycophenolic acid, PRE/P prednisolone.
Discussion
The influence of post-transplant immunosuppressants on CMV susceptibility and on antiviral T cells is of high importance for choosing preventive and therapeutic measures, since T cells are required for the final control of CMV replication [31]. Appropriate T-cell function relies on different aspects such as proliferation, cytokine secretion and cytotoxicity [32] and these aspects are targeted via different mechanisms by post-transplant immunosuppressants. Usually, for early prevention of allograft rejection and perioperative lowering of maintenance immunosuppressants following SOT, an induction therapy is applied. In this phase, different T cell-depleting agents are used. However, most CMV diseases following SOT typically occur after 30–90 days [19–22]. At this point, mostly a switch to maintenance therapy has been made by using triple combinations [33, 34]. Of note, immunosuppressive regimens differ regarding choice of immunosuppressants and dosages between the transplanted organs and centers. Of note, in case of resistant/refractory CMV disease, treatment options include secondary antiviral drugs and individual change of immunosuppression [35]. In case of insufficient antiviral T-cell immunity, adoptive transfer of virus-specific T cells can restore a long-lasting endogenous antiviral immune defense [36, 37]. In this study, we screened commonly used immunosuppressive drugs and combinations thereof with respect to different aspects of T-cell functionality in vitro.
We observed that PRE and combinations containing PRE attenuate IFN-γ secretion, which is in harmony with earlier findings [38]. PRE, the active metabolite of prednisone, is a glucocorticoid with broad immunomodulatory effects including interference with different pro-inflammatory genes and non-genomic cytosolic molecule interferences [39, 40]. IFN-γ is crucially involved in the defense against CMV and it may foreshadow the outcome prior and post transplantation [41, 42] and determines the prognosis of critically ill patients as well [43]. It was recently demonstrated that addition of methylprednisolone to regimens featuring TAC and MMF worsened the T-cell response in liver transplant recipients [44]. We did not observe significant decreases of IFN-γ secretion by the other tested immunosuppressive drugs, which is in concordance especially for SIR and EVR [45]. Of note, an additive effect was revealed for triple combinations, exceeding the inhibitory potential of PRE. Additionally, PRE and triple combinations led to decreased expression of CD69, which is regulating T-cell differentiation and metabolism [46].
SIR and EVR are mTORi and interfere with a variety of cascades, including pathways essential for T-cell proliferation [47–50]. Despite their chemical difference, distinct pharmacokinetic characteristics and mTOR complex affinities have been summarized, creating the interest of detailed side-by-side comparisons [51]. Interestingly, clinical studies showed that mTORi-based regimens are associated with lower CMV infection incidences compared to MMF-based combinations [23–29, 52].
We extended the range of surveyed molecules using intracellular cytokine staining to measure IL-2 and TNF-α production, which are both known to play an important role in the anti-viral response [53, 54]. For the CNI TAC, which leads to a decreased activation of the nuclear factor of activated T cells (NFAT) and a lower production of pro-inflammatory stimuli [55–57], one of its main effects - the depletion of IL-2 - was reflected in our study. Furthermore, we found an inhibition pattern of TAC, PRE and triple combinations that was focused on TEM and TEMRA, which are known for secreting high amounts of cytokines [58].
Together with the production of pro-inflammatory molecules, recruitment and proliferation is required for T-cell mediated organ rejection [59] and therefore targeted by immunosuppressants. Here, MPA, the active metabolite of MMF, stood out in our study. As a purine synthesis inhibitor targeting the inosine-5′-monophosphate dehydrogenase (IMDPH), it is relatively lymphocyte specific, due to the compromised de novo pathway of guanosine nucleotides (lymphocytes cannot use salvage pathway of purine synthesis) and a high affinity to their IMDPH isoform. This leads to inhibited human T- and B-cell proliferation [60]. MPA has a high growth-arresting profile [61], which we conferred to be as effective as from the investigated triple combinations. Other groups described that its function extends beyond the antimetabolite pathway inhibition [62, 63], which was partly supported by our experiments, where it showed accompanying decreased cytokine release. For this, PRE and triple combinations showed severe T-cell impairment. Moreover, under triple combinations, slightly decreased cytotoxic capacity was observed, alongside reduction of T-cell activation.
Notably, the mTORi SIR and EVR showed a selective and compared to MPA less profound inhibition of CMV-specific T-cell proliferation. Our group showed earlier that SIR can augment CMV-specific effector memory T cells while inhibiting naive T cells [64], supporting the assumption that it does not only have an isolated immunosuppressive effect. Deciphering more mechanisms is a current topic, e.g., it was recently found that for kidney transplants, mTORi prevented CMV infection via αβ and γδ T-cell preservation [65]. Moreover, CMV seems to utilize mTOR for its replication, e.g., in macrophages [66]. Furthermore, for adoptive T-cell therapy, advanced strategies are being developed to overcome limitations due to immunosuppression, like the utilization of gene knockouts for creating T cell drug resistance [67, 68]. This displays an interesting approach besides providing evidence for individual changes to more favorable drugs regimens.
To evaluate functional effects of CMV-specific T cells in context of CMV infection, we established a real-time cytotoxicity model using CMV-infected human fibroblasts in which pp65 protein expression was reported as early as 1 h and up to 24 h post infection [69]. Here, we observed that PRE and triple combinations inhibited T cell-mediated elimination of CMV-infected fibroblasts, confirming our previous results. In a study by Jackson et al., CD8+ T cells recognizing peptides derived from different CMV proteins (pp65, IE-1) were effective in an in vitro virus dissemination assay independent of their peptide specificity [70], therefore indicating that the assay developed here can be utilized to investigate T-cell responses against different viral antigens. Such assays are of broad interest, e.g., for the investigation of chimeric antigen receptor (CAR) T cells [71] and may be beneficial for future projects studying virus-specific T cells as well.
Therapeutic drug monitoring is routinely applied for CNI/mTORi and occasionally for MMF/MPA to prevent rejection and toxicities. Hence, drug concentrations investigated in this study were derived from known plasma levels to mimic a clinical situation [72–75]. Immunosuppressive protocols vary between different institutions and patients, desired ranges of combinatory sustaining therapies may lie between 5–8 ng/mL of TAC, 3–8 ng/mL EVR and 1–3.5 μg/mL MPA, for example, following liver transplantation, which was represented in our study. In a recent publication, 7.5–20 mg/d administered PRE led to a median peak plasma concentration of 0.271–0.921 μg/mL [76]. While the concentration of PRE investigated in our study was above those concentrations applied during maintenance therapy, it rather correlates to early post-transplant oral dosage. Titration studies should be conducted in the future to allow for further conclusions on dose-dependent effects. However, the results of our screening study may be useful for these further studies, including clinical trials. Further experiments comparing alloreactivity and antiviral responses side-by-side may be helpful as well. In addition, a more detailed investigation of drug interferences is of great interest, since both, TAC and SIR/EVR, bind to the FK506 binding protein at first and thus may inhibit each other [77]. Moreover, only recall responses of memory T cells but not the activation of naïve T cells was analyzed, hence future studies are needed to investigate the dose-dependent effects on memory and naïve T cells. In this study, we aimed at systematic analysis of the impact of different immunosuppressive drugs on different aspects of antiviral T-cell functionality. The impact of different immunosuppressive treatment regimens in patients with different transplantation history needs to be addressed in future studies. Especially for SOT recipients at high risk, studies on the impact of immunosuppressive drugs on the initiation of an anti-CMV immune response via activation of naïve T cells are of great interest.
To conclude, we showed that immunosuppressants administered after SOT or HSCT differentially affect CMV-specific T-cell functionality. CMV-specific T-cell responses were strongly impaired by triple combinations, while SIR, EVR and MPA selectively affected T-cell proliferation. TAC slightly inhibited activation and cytokine production. Further, PRE strongly impaired CMV-specific memory T cells, which was also reflected in the investigated triple combinations. While the focus of this study was on the impact of immunosuppressive treatment on CMV-specific T-cell immunity, our data suggest that T-cell responses towards other clinically relevant viruses such as EBV and SARS-CoV-2 might be similarly–and in case of SARS-CoV-2 even more profoundly–affected by post-transplant immunosuppressive treatment. Based on our results on double combinations (T+S/E/M/P), it can be assumed that the discontinuation of PRE in patients receiving combinatory regimens such as T+S/E/M+P would be beneficial to restore antiviral T-cell immunity. Taken together, our data suggest potential beneficial effects of treatment with mTORi whilst, if possible, TAC, MPA, PRE and triple combinations should be used cautiously for patients at high risk or suffering from CMV disease.
Data Availability Statement
The original contributions presented in the study are included in the article/Supplementary Material, further inquiries can be directed to the corresponding author.
Ethics Statement
The studies involving humans were approved by the Ethics Committee of Hannover Medical School. The studies were conducted in accordance with the local legislation and institutional requirements. The participants provided their written informed consent to participate in this study.
Author Contributions
Research design: MK, AB, BM-K, and BE-V. Writing of the paper: MK, AB, and BE-V. Performance of the research: MK, AB, AD, and MS. New reagents or analytic tools: UK, RB, and BE-V. Data analysis: MK, AB, AD, and MS. Funding acquisition: AB and BE-V. Supervision: AB, BN, RT, and BE-V. All authors contributed to the article and approved the submitted version.
Funding
The author(s) declare that financial support was received for the research, authorship, and/or publication of this article. This research was supported in part by grants from the German Research Foundation (DFG; Research Unit 2830, grant no 398367752), the German Centre for Infection Research (DZIF, TI 07.003_007) and internal project funding (HiLF) of the Hannover Medical School (MHH).
Conflict of Interest
The authors declare that the research was conducted in the absence of any commercial or financial relationships that could be construed as a potential conflict of interest.
Acknowledgments
The authors wish to thank Sarina Lukis and Elvira Schulde for excellent technical support and all donors for their participation.
Supplementary Material
The Supplementary Material for this article can be found online at: https://www.frontierspartnerships.org/articles/10.3389/ti.2024.12720/full#supplementary-material
References
1. Teira, P, Battiwalla, M, Ramanathan, M, Barrett, AJ, Ahn, KW, Chen, M, et al. Early Cytomegalovirus Reactivation Remains Associated with Increased Transplant-Related Mortality in the Current Era: A CIBMTR Analysis. Blood (2016) 127(20):2427–38. doi:10.1182/blood-2015-11-679639
2. Potena, L, and Valantine, HA. Cytomegalovirus-Associated Allograft Rejection in Heart Transplant Patients. Curr Opin Infect Dis (2007) 20(4):425–31. doi:10.1097/QCO.0b013e328259c33b
3. Stern, M, Hirsch, H, Cusini, A, van Delden, C, Manuel, O, Meylan, P, et al. Cytomegalovirus Serology and Replication Remain Associated with Solid Organ Graft Rejection and Graft Loss in the Era of Prophylactic Treatment. Transplantation (2014) 98(9):1013–8. doi:10.1097/TP.0000000000000160
4. Jakharia, N, Howard, D, and Riedel, DJ. CMV Infection in Hematopoietic Stem Cell Transplantation: Prevention and Treatment Strategies. Curr Treat Options Infect Dis (2021) 13(3):123–40. doi:10.1007/s40506-021-00253-w
5. Ramanan, P, and Razonable, RR. Cytomegalovirus Infections in Solid Organ Transplantation: A Review. Infect Chemother (2013) 45(3):260–71. doi:10.3947/ic.2013.45.3.260
6. Limaye, AP, Babu, TM, and Boeckh, M. Progress and Challenges in the Prevention, Diagnosis, and Management of Cytomegalovirus Infection in Transplantation. Clin Microbiol Rev (2020) 34(1):e00043. doi:10.1128/CMR.00043-19
7. Veit, T, Pan, M, Munker, D, Arnold, P, Dick, A, Kunze, S, et al. Association of CMV-Specific T-Cell Immunity and Risk of CMV Infection in Lung Transplant Recipients. Clin Transpl (2021) 35(6):e14294. doi:10.1111/ctr.14294
8. Grossi, PA, Kamar, N, Saliba, F, Baldanti, F, Aguado, JM, Gottlieb, J, et al. Cytomegalovirus Management in Solid Organ Transplant Recipients: A Pre-COVID-19 Survey from the Working Group of the European Society for Organ Transplantation. Transpl Int (2022) 35:10332. doi:10.3389/ti.2022.10332
9. Haidar, G, Boeckh, M, and Singh, N. Cytomegalovirus Infection in Solid Organ and Hematopoietic Cell Transplantation: State of the Evidence. J Infect Dis (2020) 221(Suppl. 1):S23–S31. doi:10.1093/infdis/jiz454
10. Tamzali, Y, Pourcher, V, Azoyan, L, Ouali, N, Barrou, B, Conti, F, et al. Factors Associated with Genotypic Resistance and Outcome Among Solid Organ Transplant Recipients with Refractory Cytomegalovirus Infection. Transpl Int (2023) 36:11295. doi:10.3389/ti.2023.11295
11. Avery, RK, Arav-Boger, R, Marr, KA, Kraus, E, Shoham, S, Lees, L, et al. Outcomes in Transplant Recipients Treated with Foscarnet for Ganciclovir-Resistant or Refractory Cytomegalovirus Infection. Transplantation (2016) 100(10):e74–80. doi:10.1097/TP.0000000000001418
12. Limaye, AP, Budde, K, Humar, A, Vincenti, F, Kuypers, DRJ, Carroll, RP, et al. Letermovir vs Valganciclovir for Prophylaxis of Cytomegalovirus in High-Risk Kidney Transplant Recipients: A Randomized Clinical Trial. JAMA (2023) 330:33–42. doi:10.1001/jama.2023.9106
13. Kotton, CN, Kumar, D, Caliendo, AM, Huprikar, S, Chou, S, Danziger-Isakov, L, et al. The Third International Consensus Guidelines on the Management of Cytomegalovirus in Solid-Organ Transplantation. Transplantation (2018) 102(6):900–31. doi:10.1097/TP.0000000000002191
14. Ozdemir, E, St John, LS, Gillespie, G, Rowland-Jones, S, Champlin, RE, Molldrem, JJ, et al. Cytomegalovirus Reactivation Following Allogeneic Stem Cell Transplantation Is Associated with the Presence of Dysfunctional Antigen-Specific CD8+ T Cells. Blood (2002) 100(10):3690–7. doi:10.1182/blood-2002-05-1387
15. Boeckh, M, Leisenring, W, Riddell, SR, Bowden, RA, Huang, ML, Myerson, D, et al. Late Cytomegalovirus Disease and Mortality in Recipients of Allogeneic Hematopoietic Stem Cell Transplants: Importance of Viral Load and T-Cell Immunity. Blood (2003) 101(2):407–14. doi:10.1182/blood-2002-03-0993
16. Donadeu, L, Revilla-Lopez, E, Jarque, M, Crespo, E, Torija, A, Bravo, C, et al. CMV-Specific Cell-Mediated Immunity Predicts a High Level of CMV Replication after Prophylaxis Withdrawal in Lung Transplant Recipients. J Infect Dis (2021) 224(3):526–31. doi:10.1093/infdis/jiaa727
17. Limaye, AP, Green, ML, Edmison, BC, Stevens-Ayers, T, Chatterton-Kirchmeier, S, Geballe, AP, et al. Prospective Assessment of Cytomegalovirus Immunity in High-Risk Donor-Seropositive/Recipient-Seronegative Liver Transplant Recipients Receiving Either Preemptive Therapy or Antiviral Prophylaxis. J Infect Dis (2019) 220(5):752–60. doi:10.1093/infdis/jiz181
18. Singh, N, Winston, DJ, Razonable, RR, Lyon, GM, Silveira, FP, Wagener, MM, et al. Effect of Preemptive Therapy vs Antiviral Prophylaxis on Cytomegalovirus Disease in Seronegative Liver Transplant Recipients with Seropositive Donors: A Randomized Clinical Trial. JAMA (2020) 323(14):1378–87. doi:10.1001/jama.2020.3138
19. Azevedo, LS, Pierrotti, LC, Abdala, E, Costa, SF, Strabelli, TM, Campos, SV, et al. Cytomegalovirus Infection in Transplant Recipients. Clinics (Sao Paulo) (2015) 70(7):515–23. doi:10.6061/clinics/2015(07)09
20. Humar, A, Limaye, AP, Blumberg, EA, Hauser, IA, Vincenti, F, Jardine, AG, et al. Extended Valganciclovir Prophylaxis in D+/R-Kidney Transplant Recipients Is Associated with Long-Term Reduction in Cytomegalovirus Disease: Two-Year Results of the IMPACT Study. Transplantation (2010) 90(12):1427–31. doi:10.1097/tp.0b013e3181ff1493
21. Legendre, C, and Pascual, M. Improving Outcomes for Solid-Organ Transplant Recipients at Risk from Cytomegalovirus Infection: Late-Onset Disease and Indirect Consequences. Clin Infect Dis (2008) 46(5):732–40. doi:10.1086/527397
22. Blyth, D, Lee, I, Sims, KD, Gasink, LB, Barton, TD, Van Deerlin, VM, et al. Risk Factors and Clinical Outcomes of Cytomegalovirus Disease Occurring More Than One Year post Solid Organ Transplantation. Transpl Infect Dis (2012) 14(2):149–55. doi:10.1111/j.1399-3062.2011.00705.x
23. Vigano, M, Dengler, T, Mattei, MF, Poncelet, A, Vanhaecke, J, Vermes, E, et al. Lower Incidence of Cytomegalovirus Infection with Everolimus Versus Mycophenolate Mofetil in De Novo Cardiac Transplant Recipients: A Randomized, Multicenter Study. Transpl Infect Dis (2010) 12(1):23–30. doi:10.1111/j.1399-3062.2009.00448.x
24. Hauser, IA, Marx, S, Sommerer, C, Suwelack, B, Dragun, D, Witzke, O, et al. Effect of Everolimus-Based Drug Regimens on CMV-Specific T-Cell Functionality After Renal Transplantation: 12-Month ATHENA Subcohort-Study Results. Eur J Immunol (2021) 51(4):943–55. doi:10.1002/eji.202048855
25. Tedesco-Silva, H, Pascual, J, Viklicky, O, Basic-Jukic, N, Cassuto, E, Kim, DY, et al. Safety of Everolimus with Reduced Calcineurin Inhibitor Exposure in De Novo Kidney Transplants: An Analysis from the Randomized TRANSFORM Study. Transplantation (2019) 103(9):1953–63. doi:10.1097/TP.0000000000002626
26. Demopoulos, L, Polinsky, M, Steele, G, Mines, D, Blum, M, Caulfield, M, et al. Reduced Risk of Cytomegalovirus Infection in Solid Organ Transplant Recipients Treated with Sirolimus: A Pooled Analysis of Clinical Trials. Transpl Proc (2008) 40(5):1407–10. doi:10.1016/j.transproceed.2008.03.084
27. Kobashigawa, J, Ross, H, Bara, C, Delgado, JF, Dengler, T, Lehmkuhl, HB, et al. Everolimus Is Associated with a Reduced Incidence of Cytomegalovirus Infection Following De Novo Cardiac Transplantation. Transpl Infect Dis (2013) 15(2):150–62. doi:10.1111/tid.12007
28. Mallat, SG, Tanios, BY, Itani, HS, Lotfi, T, McMullan, C, Gabardi, S, et al. CMV and BKPyV Infections in Renal Transplant Recipients Receiving an mTOR Inhibitor-Based Regimen Versus a CNI-Based Regimen: A Systematic Review and Meta-Analysis of Randomized, Controlled Trials. Clin J Am Soc Nephrol (2017) 12(8):1321–36. doi:10.2215/CJN.13221216
29. Sommerer, C, Suwelack, B, Dragun, D, Schenker, P, Hauser, IA, Witzke, O, et al. An Open-Label, Randomized Trial Indicates that Everolimus with Tacrolimus or Cyclosporine Is Comparable to Standard Immunosuppression in De Novo Kidney Transplant Patients. Kidney Int (2019) 96(1):231–44. doi:10.1016/j.kint.2019.01.041
30. Tonshoff, B. Immunosuppressants in Organ Transplantation. Handb Exp Pharmacol (2020) 261:441–69. doi:10.1007/164_2019_331
31. Loewendorf, A, and Benedict, CA. Modulation of Host Innate and Adaptive Immune Defenses by Cytomegalovirus: Timing Is Everything. J Intern Med (2010) 267(5):483–501. doi:10.1111/j.1365-2796.2010.02220.x
32. Klenerman, P, and Oxenius, A. T Cell Responses to Cytomegalovirus. Nat Rev Immunol (2016) 16(6):367–77. doi:10.1038/nri.2016.38
33. Kidney Disease: Improving Global Outcomes Transplant Work G. KDIGO Clinical Practice Guideline for the Care of Kidney Transplant Recipients. Am J Transpl (2009) 9(Suppl. 3):S1–155. doi:10.1111/j.1600-6143.2009.02834.x
34. Arnol, M, Naumovic, R, Dimitrov, EP, Racki, S, Bucsa, CA, Covic, A, et al. Immunosuppressive Regimens Following Kidney Transplantation in Five European Countries: The Observational RECORD Study. Transplant Rep (2020) 5(3):100061. doi:10.1016/j.tpr.2020.100061
35. Asberg, A, Jardine, AG, Bignamini, AA, Rollag, H, Pescovitz, MD, Gahlemann, CC, et al. Effects of the Intensity of Immunosuppressive Therapy on Outcome of Treatment for CMV Disease in Organ Transplant Recipients. Am J Transpl (2010) 10(8):1881–8. doi:10.1111/j.1600-6143.2010.03114.x
36. Eiz-Vesper, B, Maecker-Kolhoff, B, and Blasczyk, R. Adoptive T-Cell Immunotherapy from Third-Party Donors: Characterization of Donors and Set up of a T-Cell Donor Registry. Front Immunol (2012) 3:410. doi:10.3389/fimmu.2012.00410
37. Tzannou, I, Papadopoulou, A, Naik, S, Leung, K, Martinez, CA, Ramos, CA, et al. Off-The-Shelf Virus-Specific T Cells to Treat BK Virus, Human Herpesvirus 6, Cytomegalovirus, Epstein-Barr Virus, and Adenovirus Infections After Allogeneic Hematopoietic Stem-Cell Transplantation. J Clin Oncol (2017) 35(31):3547–57. doi:10.1200/JCO.2017.73.0655
38. Gardiner, BJ, Lee, SJ, Cristiano, Y, Levvey, BJ, Sullivan, LC, Snell, GI, et al. Evaluation of Quantiferon®-Monitor as a Biomarker of Immunosuppression and Predictor of Infection in Lung Transplant Recipients. Transpl Infect Dis (2021) 23(3):e13550. doi:10.1111/tid.13550
39. Herold, MJ, McPherson, KG, and Reichardt, HM. Glucocorticoids in T Cell Apoptosis and Function. Cell Mol Life Sci (2006) 63(1):60–72. doi:10.1007/s00018-005-5390-y
40. Liberman, AC, Budzinski, ML, Sokn, C, Gobbini, RP, Steininger, A, and Arzt, E. Regulatory and Mechanistic Actions of Glucocorticoids on T and Inflammatory Cells. Front Endocrinol (Lausanne) (2018) 9:235. doi:10.3389/fendo.2018.00235
41. Cantisan, S, Lara, R, Montejo, M, Redel, J, Rodriguez-Benot, A, Gutierrez-Aroca, J, et al. Pretransplant Interferon-Gamma Secretion by CMV-Specific CD8+ T Cells Informs the Risk of CMV Replication after Transplantation. Am J Transpl (2013) 13(3):738–45. doi:10.1111/ajt.12049
42. De Gracia-Guindo, MDC, Ruiz-Fuentes, MDC, Galindo-Sacristan, P, Osorio-Moratalla, JM, Ruiz-Fuentes, N, Rodriguez Granger, J, et al. Cytomegalovirus Infection Monitoring Based on Interferon Gamma Release Assay in Kidney Transplantation. Transpl Proc (2018) 50(2):578–80. doi:10.1016/j.transproceed.2017.09.052
43. Caston, JJ, Cantisan, S, Gonzalez-Gasca, F, Paez-Vega, A, Abdel-Hadi, H, Illescas, S, et al. Interferon-Gamma Production by CMV-Specific CD8+ T Lymphocytes Provides protection Against Cytomegalovirus Reactivation in Critically Ill Patients. Intensive Care Med (2016) 42(1):46–53. doi:10.1007/s00134-015-4077-6
44. Uemoto, S, Ozawa, K, Kaido, T, Mori, A, and Fujimoto, Y. Advantage of Tacrolimus/Mycophenolate Mofetil Regimen for Cytotoxic T Cell Mediated Defence and its Inhibition by Additive Steroid Administration in High-Risk Liver Transplant Recipients. Clin Exp Immunol (2016) 184(1):126–36. doi:10.1111/cei.12740
45. Jin, N, Malcherek, G, Mani, J, Zurleit, R, Schmitt, A, Chen, B, et al. Suppression of Cytomegalovirus-Specific CD8(+)T Cells by Everolimus. Leuk Lymphoma (2014) 55(5):1144–50. doi:10.3109/10428194.2013.822496
46. Cibrian, D, and Sanchez-Madrid, F. CD69: From Activation Marker to Metabolic Gatekeeper. Eur J Immunol (2017) 47(6):946–53. doi:10.1002/eji.201646837
47. Schuurman, HJ, Cottens, S, Fuchs, S, Joergensen, J, Meerloo, T, Sedrani, R, et al. SDZ RAD, a New Rapamycin Derivative: Synergism with Cyclosporine. Transplantation (1997) 64(1):32–5. doi:10.1097/00007890-199707150-00007
48. Schuler, W, Sedrani, R, Cottens, S, Haberlin, B, Schulz, M, Schuurman, HJ, et al. SDZ RAD, a New Rapamycin Derivative: Pharmacological Properties In Vitro and In Vivo. Transplantation (1997) 64(1):36–42. doi:10.1097/00007890-199707150-00008
49. Dumont, FJ, and Su, Q. Mechanism of Action of the Immunosuppressant Rapamycin. Life Sci (1996) 58(5):373–95. doi:10.1016/0024-3205(95)02233-3
50. Laplante, M, and Sabatini, DM. mTOR Signaling in Growth Control and Disease. Cell (2012) 149(2):274–93. doi:10.1016/j.cell.2012.03.017
51. Klawitter, J, Nashan, B, and Christians, U. Everolimus and Sirolimus in Transplantation-Related but Different. Expert Opin Drug Saf (2015) 14(7):1055–70. doi:10.1517/14740338.2015.1040388
52. Nashan, B, Gaston, R, Emery, V, Saemann, MD, Mueller, NJ, Couzi, L, et al. Review of Cytomegalovirus Infection Findings with Mammalian Target of Rapamycin Inhibitor-Based Immunosuppressive Therapy in De Novo Renal Transplant Recipients. Transplantation (2012) 93(11):1075–85. doi:10.1097/TP.0b013e31824810e6
53. Boyman, O, and Sprent, J. The Role of Interleukin-2 During Homeostasis and Activation of the Immune System. Nat Rev Immunol (2012) 12(3):180–90. doi:10.1038/nri3156
54. Fiers, W. Tumor Necrosis Factor. Characterization at the Molecular, Cellular and In Vivo Level. FEBS Lett (1991) 285(2):199–212. doi:10.1016/0014-5793(91)80803-b
55. Thomson, AW, Bonham, CA, and Zeevi, A. Mode of Action of Tacrolimus (FK506): Molecular and Cellular Mechanisms. Ther Drug Monit (1995) 17(6):584–91. doi:10.1097/00007691-199512000-00007
56. Rusnak, F, and Mertz, P. Calcineurin: Form and Function. Physiol Rev (2000) 80(4):1483–521. doi:10.1152/physrev.2000.80.4.1483
57. Ho, S, Clipstone, N, Timmermann, L, Northrop, J, Graef, I, Fiorentino, D, et al. The Mechanism of Action of Cyclosporin A and FK506. Clin Immunol Immunopathol (1996) 80(3 Pt 2):S40–5. doi:10.1006/clin.1996.0140
58. Raphael, I, Joern, RR, and Forsthuber, TG. Memory CD4(+) T Cells in Immunity and Autoimmune Diseases. Cells (2020) 9(3):531. doi:10.3390/cells9030531
59. Ronca, V, Wootton, G, Milani, C, and Cain, O. The Immunological Basis of Liver Allograft Rejection. Front Immunol (2020) 11:2155. doi:10.3389/fimmu.2020.02155
60. Allison, AC. Mechanisms of Action of Mycophenolate Mofetil. Lupus. (2005) 14(Suppl. 1):s2–8. doi:10.1191/0961203305lu2109oa
61. Staatz, CE, and Tett, SE. Clinical Pharmacokinetics and Pharmacodynamics of Mycophenolate in Solid Organ Transplant Recipients. Clin Pharmacokinet (2007) 46(1):13–58. doi:10.2165/00003088-200746010-00002
62. He, X, Smeets, RL, Koenen, HJ, Vink, PM, Wagenaars, J, Boots, AM, et al. Mycophenolic Acid-Mediated Suppression of Human CD4+ T Cells: More Than Mere Guanine Nucleotide Deprivation. Am J Transpl (2011) 11(3):439–49. doi:10.1111/j.1600-6143.2010.03413.x
63. Lemoine, R, Velge-Roussel, F, Herr, F, Felix, R, Nivet, H, Lebranchu, Y, et al. Interferon Gamma Licensing of Human Dendritic Cells in T-helper-Independent CD8+ Alloimmunity. Blood (2010) 116(16):3089–98. doi:10.1182/blood-2010-02-268623
64. Bak, S, Tischer, S, Dragon, A, Ravens, S, Pape, L, Koenecke, C, et al. Selective Effects of mTOR Inhibitor Sirolimus on Naive and CMV-Specific T Cells Extending its Applicable Range beyond Immunosuppression. Front Immunol (2018) 9:2953. doi:10.3389/fimmu.2018.02953
65. Kaminski, H, Marseres, G, Yared, N, Nokin, MJ, Pitard, V, Zouine, A, et al. mTOR Inhibitors Prevent CMV Infection Through the Restoration of Functional αβ and γδ T Cells in Kidney Transplantation. J Am Soc Nephrol (2022) 33(1):121–37. doi:10.1681/ASN.2020121753
66. Poglitsch, M, Weichhart, T, Hecking, M, Werzowa, J, Katholnig, K, Antlanger, M, et al. CMV Late Phase-Induced mTOR Activation Is Essential for Efficient Virus Replication in Polarized Human Macrophages. Am J Transpl (2012) 12(6):1458–68. doi:10.1111/j.1600-6143.2012.04002.x
67. Amini, L, Wagner, DL, Rossler, U, Zarrinrad, G, Wagner, LF, Vollmer, T, et al. CRISPR-Cas9-Edited Tacrolimus-Resistant Antiviral T Cells for Advanced Adoptive Immunotherapy in Transplant Recipients. Mol Ther (2021) 29(1):32–46. doi:10.1016/j.ymthe.2020.09.011
68. Kaeuferle, T, Deisenberger, L, Jablonowski, L, Stief, TA, Blaeschke, F, Willier, S, et al. CRISPR-Cas9-Mediated Glucocorticoid Resistance in Virus-specific T Cells for Adoptive T Cell Therapy Posttransplantation. Mol Ther (2020) 28(9):1965–73. doi:10.1016/j.ymthe.2020.06.002
69. Biolatti, M, Dell'Oste, V, Pautasso, S, Gugliesi, F, von Einem, J, Krapp, C, et al. Human Cytomegalovirus Tegument Protein Pp65 (pUL83) Dampens Type I Interferon Production by Inactivating the DNA Sensor cGAS Without Affecting STING. J Virol (2018) 92(6):e01774. doi:10.1128/JVI.01774-17
70. Jackson, SE, Mason, GM, Okecha, G, Sissons, JG, and Wills, MR. Diverse Specificities, Phenotypes, and Antiviral Activities of Cytomegalovirus-Specific CD8+ T Cells. J Virol (2014) 88(18):10894–908. doi:10.1128/JVI.01477-14
71. Lisby, AN, Carlson, RD, Baybutt, TR, Weindorfer, M, and Snook, AE. Evaluation of CAR-T Cell Cytotoxicity: Real-Time Impedance-Based Analysis. Methods Cel Biol (2022) 167:81–98. doi:10.1016/bs.mcb.2021.08.002
72. Bergan, S, Brunet, M, Hesselink, DA, Johnson-Davis, KL, Kunicki, PK, Lemaitre, F, et al. Personalized Therapy for Mycophenolate: Consensus Report by the International Association of Therapeutic Drug Monitoring and Clinical Toxicology. Ther Drug Monit (2021) 43(2):150–200. doi:10.1097/FTD.0000000000000871
73. Brunet, M, van Gelder, T, Asberg, A, Haufroid, V, Hesselink, DA, Langman, L, et al. Therapeutic Drug Monitoring of Tacrolimus-Personalized Therapy: Second Consensus Report. Ther Drug Monit (2019) 41(3):261–307. doi:10.1097/FTD.0000000000000640
74. Shipkova, M, Hesselink, DA, Holt, DW, Billaud, EM, van Gelder, T, Kunicki, PK, et al. Therapeutic Drug Monitoring of Everolimus: A Consensus Report. Ther Drug Monit (2016) 38(2):143–69. doi:10.1097/FTD.0000000000000260
75. MacDonald, A, Scarola, J, Burke, JT, and Zimmerman, JJ. Clinical Pharmacokinetics and Therapeutic Drug Monitoring of Sirolimus. Clin Ther (2000) 22(Suppl. B):B101–21. doi:10.1016/s0149-2918(00)89027-x
76. Skauby, RH, Gustavsen, MT, Andersen, AM, Bjerre, A, Asberg, A, Midtvedt, K, et al. Prednisolone and Prednisone Pharmacokinetics in Adult Renal Transplant Recipients. Ther Drug Monit (2021) 43(2):247–55. doi:10.1097/FTD.0000000000000835
Keywords: CMV-specific T cells, immunosuppression, adoptive T-cell therapy, solid organ transplantation, hematopoietic stem cell transplantation
Citation: Krueger MB, Bonifacius A, Dragon AC, Santamorena MM, Nashan B, Taubert R, Kalinke U, Maecker-Kolhoff B, Blasczyk R and Eiz-Vesper B (2024) In Vitro Profiling of Commonly Used Post-transplant Immunosuppressants Reveals Distinct Impact on Antiviral T-cell Immunity Towards CMV. Transpl Int 37:12720. doi: 10.3389/ti.2024.12720
Received: 22 January 2024; Accepted: 27 March 2024;
Published: 09 April 2024.
Copyright © 2024 Krueger, Bonifacius, Dragon, Santamorena, Nashan, Taubert, Kalinke, Maecker-Kolhoff, Blasczyk and Eiz-Vesper. This is an open-access article distributed under the terms of the Creative Commons Attribution License (CC BY). The use, distribution or reproduction in other forums is permitted, provided the original author(s) and the copyright owner(s) are credited and that the original publication in this journal is cited, in accordance with accepted academic practice. No use, distribution or reproduction is permitted which does not comply with these terms.
*Correspondence: Britta Eiz-Vesper, ZWl6LXZlc3Blci5icml0dGFAbWgtaGFubm92ZXIuZGU=
†These authors have contributed equally to this work