- 1CIRI, INSERM U1111, Université Claude Bernard Lyon I, CNRS UMR5308, Ecole Normale Supérieure de Lyon, University of Lyon, Lyon, France
- 2Hospices Civils de Lyon, Edouard Herriot Hospital, Department of Transplantation, Nephrology and Clinical Immunology, Lyon, France
- 3Lyon-Est Medical Faculty, Claude Bernard University (Lyon 1), Lyon, France
Chronic graft rejection represents a significant threat to long-term graft survival. Early diagnosis, understanding of the immunological mechanisms and appropriate therapeutic management are essential to improve graft survival and quality of life for transplant patients. Knowing which immune cells are responsible for chronic vascular rejection would allow us to provide effective and appropriate treatment for these patients. It is now widely accepted that natural killer (NK) cells play an important role in chronic vascular rejection. They can either initiate chronic vascular rejection by recognizing missing self on the graft or be recruited by donor-specific antibodies to destroy the graft during antibody-mediated rejection. Whatever the mechanisms of activation of NK cells, they need to be primed to become fully activated and damaging to the graft. A better understanding of the signaling pathways involved in NK cell priming and activation would pave the way for the development of new therapeutic strategies to cure chronic vascular rejection. This review examines the critical role of NK cells in the complex context of chronic vascular rejection.
Introduction
Solid organ transplantation represents the optimal treatment option for patients with end-stage organ failure [1]. Nevertheless, the survival of allografts is constrained by the occurrence of rejection, which is initiated when the recipient’s immune system identifies donor determinants [in particular, mismatched Human Leucocyte Antigen (HLA) molecules] and conducts the destruction of the graft. Over the past few decades, the development of immunosuppressants has enabled significant improvements in short-term graft survival, largely by preventing early rejections, which are mainly driven by T cells. However, the percentage of grafts that do experience attrition beyond the first transplant year remains unchanged [2]. Long-term graft survival represents an unmet medical need in solid organ transplantation. In the majority of cases, long-term graft loss is of immunological origin and is referred to as chronic rejection. Although there are discrepancies in the lesions found in cases of chronic rejection in the different organs, it is now clear that vascular lesions are a common feature [3]. It is widely accepted that these lesions may be the result of mechanisms involving donor specific antibodies (DSA) [3]. However, in some cases, antibodies are not present, suggesting that other mechanisms may also be involved in chronic vascular rejection. Recent research has challenged traditional views on chronic vascular rejection, revealing a prominent role for Natural Killer (NK) cells in chronic vascular rejection, regardless of the presence or absence of DSA. This challenges the prevailing view that innate immune cells only play a role in rejection if they are recruited by the adaptive immune system. The objective of this review is to examine the role of NK cells in chronic vascular rejection, with a particular focus on the mechanisms that can trigger their activation. This will provide insights into the pathways involved and identify potential therapeutic avenues for preventing their activation and, consequently, reducing the occurrence of chronic vascular rejection.
General Overview of NK Cell Activation
NK cells are a crucial component of the innate immune system, responsible for detecting and eliminating infected, stressed, or transformed cells, such as cancerous cells. Unlike T cells and B cells, which are part of the adaptive immune system and require prior exposure to a specific pathogen for an effective response, NK cells can respond immediately to threats without prior sensitization. To achieve this, NK cells express several germline-encoded activating and inhibitory receptors on their surface (Figure 1). The balance between these signals determines whether the NK cell will attack the target cell. NK cells can be activated through three main mechanisms. First, missing-self recognition occurs when a target cell loses or downregulates its HLA class I molecules, often due to viral infection or cancer (Figure 1). The lack of inhibitory signals allows NK cells to destroy the target cell. Second, NK cells can be activated by induced-self ligands. Cells under stress or transformation may express specific ligands, such as MICA/B or ULBPs, which are recognized by activating receptors on NK cells, triggering NK cell activation (Figure 1). Finally, NK cells can be activated through antibody-dependent cellular cytotoxicity (ADCC). In this process, NK cells recognize antibodies bound to the surface of target cells via their Fcγ receptor (FcγRIIIA) (Figure 1). This antibody-mediated recognition leads to NK cell activation and targeted killing of the antibody-coated cell.
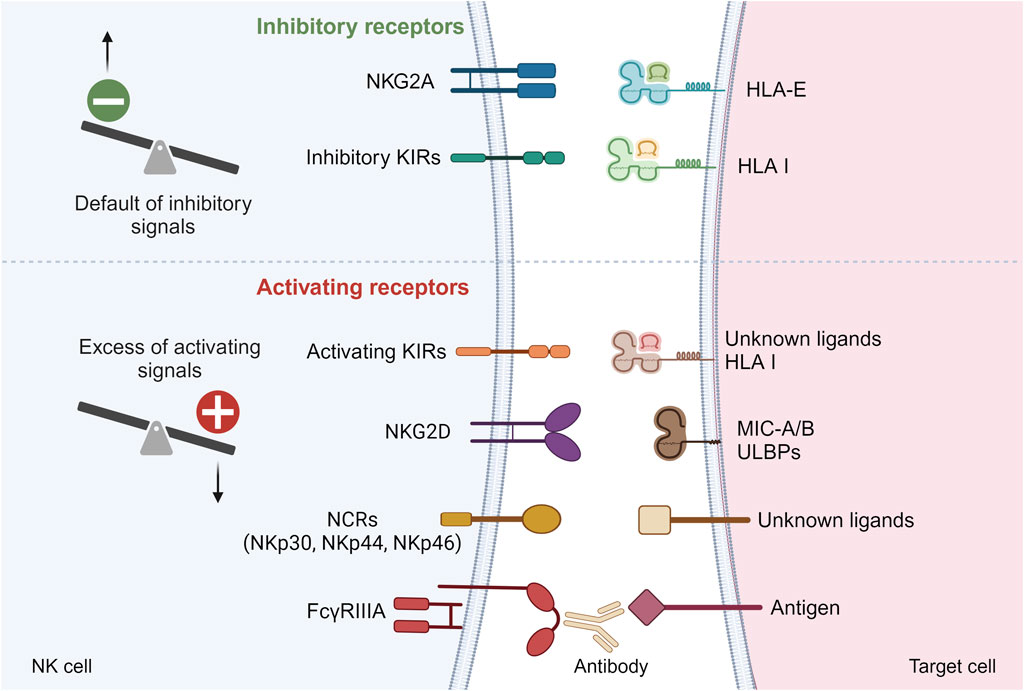
Figure 1. Main inhibitory and activating receptors on NK cell surface. Default of inhibitory signals or excess of activating signals can lead to NK cell activation. KIRs, Killer Immunoglobin-like Receptors; NCRs, Natural Cytotoxicity Receptors; HLA, Human Leucocyte Antigen; MIC-A/B, MHC class I-related chain A/B; ULBPs, UL16-binding proteins.
In the following sections of the review, we will describe how these mechanisms of NK cell activation might be involved in chronic vascular rejection.
Missing-Self Induced NK Cell Activation and “Innate” Rejections
Inhibitory Killer Cell Immunoglobulin-Like Receptors (KIR) Bind to HLA Class I
Inhibitory KIRs are highly polymorphic at the genetic level, heterogeneously expressed by NK cells. Inhibitory KIRs possess two (2D) or three (3D) extracellular immunoglobulin domains, a transmembrane domain and a long (L) cytoplasmic tail containing two immunoreceptor tyrosine-based inhibitory motifs (ITIMs) [4]. Each inhibitory KIR has for ligands a subgroup of HLA class I allotypes. Inhibitory KIRs with 2 extracellular domains recognize HLA-C molecules based on two dimorphisms at position 77 and 80 of the α1 domain of the α chain [4]. KIR2DL1 associates with HLA C2 allotypes [4]. In contrast, KIR2DL2 and three show specificity for HLA C1 allotypes [4]. KIR3DL1 recognizes HLA-A and HLA-B molecules that display the Bw4 motif [5]. Finally, KIR3DL2 solely interacts with HLA-A3 and A11 molecules [4].
NK Cell Education and Activation by Missing-Self
The complex interplay between inhibitory KIRs and HLA class I molecules is of paramount importance in the education and activation of NK cells. As the genes for inhibitory KIRs and HLA class I molecules are on different chromosomes and that NK cells express germline-encoded receptors that do not undergo somatic rearrangement during development, NK cells need to undergo an educational process where they learn to distinguish “self” from “non-self” through interactions between inhibitory KIRs and self HLA class I molecules [6]. NK cells that encounter self-HLA class I molecules during their maturation process receive inhibitory signals, ensuring that they do not attack healthy cells displaying self HLA class I molecules (Figure 2). In absence of any interaction between inhibitory KIRs and HLA class I molecules during their development, NK cells become hypo responsive (Figure 2). This process helps to refine NK cell self-tolerance and future reactivity as the number of interactions between inhibitory KIRs on NK cells and HLA class I molecules determines the degree of responsiveness of mature NK cells. Conversely, when these educated NK cells encounter virally infected or transformed cells that lack or display altered HLA class I molecules, the lack of inhibitory signals transmitted by inhibitory KIRs allows for the activation of NK cells by the recognition of missing self (Figure 2).
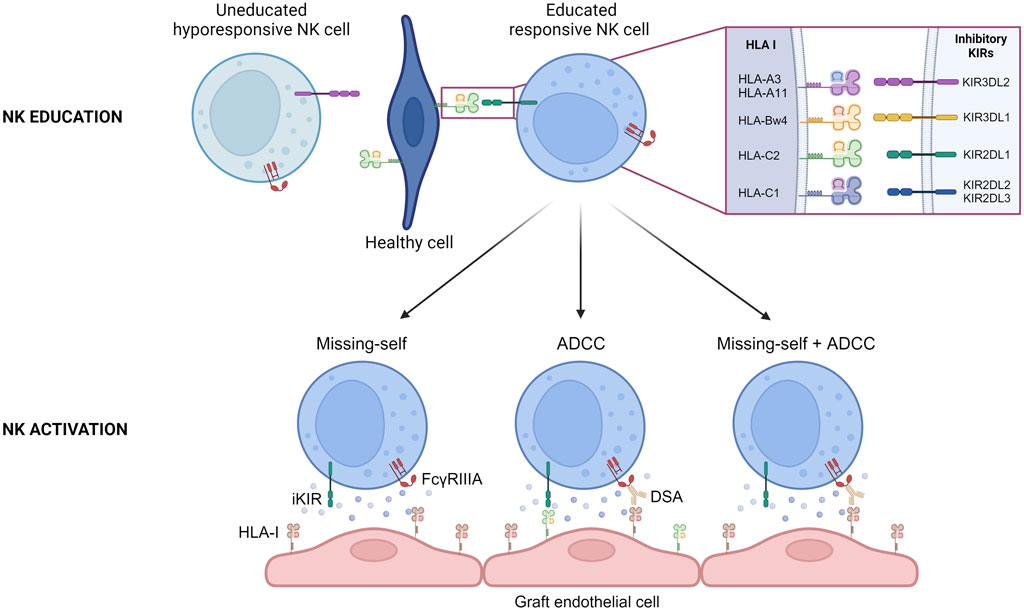
Figure 2. NK cell education and activation during chronic vascular rejection. Schematic representation of the education process of NK cells during their maturation. The interaction between specific inhibitory KIRs on NK cell surface and HLA I on healthy cells allows NK cell to become responsive. Schematic representation of the three situations that can conduct to recipient NK cell activation during chronic vascular rejection. ADCC, Antibody Dependent Cell Cytotoxicity; DSA, Donor Specific Antibody; HLA-I, Human Leucocyte Antigen class I.
Missing-Self Induced NK Cell Activation Triggers Rejection After Organ Transplantation
For a considerable period, the implication of the “missing self” in organ transplantation was overlooked. New advances were made when Colvin and his team observed that when a heart transplant from a parental strain was transplanted to an F1 hybrid strain (between the parental line and a second strain), the graft presented chronic vascular rejection [7]. It is notable that this model demonstrates that NK cells can initiate chronic vascular rejection, but that they are insufficient to trigger chronic vascular lesions when acting alone. For this to occur, they must recruit T cells that are not specific for donor major histocompatibility complex (MHC) and macrophages in particular, through the secretion of interferon gamma (IFN-γ) [7, 8]. In humans, many studies have looked at this issue for a long time without results, probably because of a lack of data to analyze the presence of missing-self correctly [9–13], or because the chosen read out was not the good one [10], or because of confounding factors [14, 15]. A decade ago, in a cohort of 137 kidney transplant patients compatible for HLA-A, HLA-B and HLA-DR (i.e., in whom minimal T- and B cell alloreactivity was expected), Van Bergen et al. observed that missing self was associated with a worse allograft survival [14]. This was one of the first clinical studies to suggest the involvement of missing self after kidney transplantation, but to go deeper into the demonstration, we have to wait for a study published by our group a few years ago [15]. In a cohort of deeply phenotyped kidney transplant patients, we demonstrated that a greater proportion of patients with microvascular inflammation lesions in the absence of DSA (either HLA or non-HLA DSA) exhibited a missing self on their graft susceptible to being sensed by the recipient’s NK cells and this resulted in reduced allograft survival compared to a control cohort (patients with no vascular lesions and no DSA) [15]. This clinical correlation was confirmed in an in vitro model in which we demonstrated that the lack of self HLA class I molecules on endothelial cells can activate NK cells, which in turn induce endothelial cell damage (Figure 2) [15]. An in vivo mouse model of heart transplantation was then developed. Heart transplants were performed from β2 micro KO mice (i.e., mice that do not express MHC class I molecules) to mice of the same genetic background [15]. The grafts developed microvascular inflammation lesions only in the presence of NK cells and a missing self, thereby confirming the existence of missing-self induced NK-mediated rejection [15]. Our results were confirmed in a population-based study which showed that missing self increased microvascular inflammation occurrence after kidney transplantation, independently of DSA [16]. Microvascular inflammation severity increased with the number of missing self [16].
NK Cell-Mediated ADCC During B Cell-Mediated Rejections
Key Role of NK Cells During Chronic Antibody-Mediated Rejection (AMR)
It is widely accepted that NK cells play a pivotal role in the development of chronic vascular rejection in the presence of DSA. Binding of circulating DSA to directly accessible donor HLA molecules expressed by graft endothelial cells can sometimes trigger the classical complement pathway, which accelerates the rejection process [17, 18]. However, this is not a mandatory requirement for the development of chronic AMR [19]. It has been demonstrated that the recruitment of innate immune cells by DSA is sufficient to trigger endothelial cell damage through an ADCC mechanism (Figure 2). In 2012, Colvin and his team have shown that when RAG−/−C3−/− mice were transplanted with an allogeneic heart and injected regularly with DSA, they developed chronic vascular lesions in their graft and that these lesions were completely abrogated in the absence of NK cells [20]. In the clinical setting, the key role of NK cells in AMR is supported by transcriptomic analyses of renal graft biopsies [21–23]. Recent data suggesting that afucosylation of HLA-specific IgG1 is directly related to antibody pathogenicity in kidney transplantation also provides indirect evidence for the key role of NK cells in AMR, as fucosylation is known to strongly influence the affinity of IgG for FcγRIIIA, which is expressed by NK cells [24, 25]. Furthermore, the intensity of NK cell infiltration within the graft correlates with graft survival after kidney transplantation [26].
NK cell activation during AMR is thought to be triggered by the interaction between NK’s unique Fcγ receptor, FcγRIIIA and DSA (Figure 2). In the murine heart transplantation model of AMR described above, mice injected with F (ab′)2 DSA fragments failed to develop chronic vascular lesions in comparison to mice injected with intact DSA, suggesting that the interaction of NK cells with the Fc fragment of DSA is necessary [20]. Transcriptomic analyses conducted on sets of renal allograft biopsies from patients with AMR identified NK cell signaling, including evidence for the FcγRIIIA signaling elements [27, 28] suggestive of an NK cell activation through FcγRIIIA in AMR. In a recent study, thanks to single cell RNA sequencing analyses and multiplexed immunofluorescence, Lamarthée and colleagues have shown an association between FcγRIIIA+ NK cells and the severity of intragraft inflammation in the context of AMR [22]. Like in missing-self induced NK-mediated rejections, NK cells do not act alone and seem to interact in particular with FcγRIIIA+ non-classical monocytes via LGALS9-HAVCR2 to trigger allograft destruction [22]. They also interact to a lesser extent with T cells expressing CD74CXCR4 via the secretion of macrophage migration inhibitory factor (MIF) [22].
Factors Modulating NK Cell Activation During AMR
A single nucleotide polymorphism exists in the FcGR3A gene, resulting in the expression of two co-dominant alleles coding for either a phenylalanine (F) or a valine (V) at amino acid position 158 in the extracellular domain of the receptor [29]. This single nucleotide polymorphism modulates the binding capacity of FcγRIIIA to the Fc fragment of IgG, which may in turn modulate the severity of lesions and the prognosis of patients with AMR. In kidney transplantation, Arnold et al. observed that patients with DSA and presenting at least one high binding allele (V/V or V/F) exhibited more frequent and severe peritubular capillaritis lesions [30]. Furthermore, the researchers hypothesize that this heightened microvascular inflammation may be due to the secretion of IFN-γ, which facilitates the recruitment of other immune cells in the graft [30]. These findings were corroborated in a cohort of kidney transplant recipients with chronic humoral rejection [31]. The patients with two V/V alleles exhibited a higher glomerulitis score and a reduced graft survival compared to patients of other genotypes [31]. The authors propose that the V-allele is associated with an increased expression of FcγRIIIA by NK cells, which translates into a greater propensity for NK cells to degranulate when they interact with their targets [31].
In addition, a recent study has investigated the impact of different genetic variations present in the genes of different NK cells in a cohort of patients with de novo DSA [32]. In particular, they got interested in a polymorphism in KLRC2 gene which encodes NKG2C, an activating NK cell receptor, which binds to HLA-E and is known to be present on memory like NK cells with more potent effector functions [29]. Heterozygous or homozygous KLRC2 deletion (KLRC2del) is associated with a significantly lower or absent NKG2C expression level. Here, they showed that patients with a KLRC2wt/wt genotype presented more microvascular lesions than KLRC2wt/del patients but failed to show any impact on allograft survival [32].
Finally, our group has recently shown that missing self and DSA can combine to induce chronic vascular rejection in a cohort of kidney transplant patients with AMR (Figure 2) [33]. Notably, the additive effect of missing self on allograft survival was only observed in patients with ADCC-dependent AMR [33]. This may be explained by the fact that allograft loss is too rapid in patients with complement-dependent AMR.
Whether the NK cell initiates rejection in missing self-induced rejection or is recruited by the adaptive immune system in AMR, it seems that a final common pathway involving NK cell is triggered during chronic vascular rejection. This was suggested in particular by a study which showed that the transcriptomic signatures were similar in biopsies of patients with DSA-positive and DSA-negative microvascular inflammation lesions [34]. These patients showed similar upregulation of pathways such as IFNγ-induced pathways and NK cell activation, and similar enrichment of infiltrating leukocytes [34]. A better understanding of the pathways leading to NK activation may therefore be useful in the development of treatments targeting NK cells in chronic vascular rejection, whether or not DSA are present.
Signaling Pathways Involved in NK Cell Priming and Activation
To become fully activated, NK cells need to receive a priming signal from cytokines and a second signal that results from the balance of inhibitory and activating signals transmitted by their many inhibitory and activating receptors (Figure 1).
NK Cell Priming
Among the various cytokines that may play a role in NK cell priming (IL-2, IL-12, IL-15, IL-18 and IL-21), IL-15 is of particular importance in regulating NK cell homeostasis and activation [35–37]. IL-15 binds to IL-15Rα expressed on antigen presenting cells and is then presented in trans to the IL-2/IL-15Rβγ heterodimer on NK cells. IL-15 induces the phosphorylation of JAK1/JAK3, allowing the recruitment and activation of the transcription factor STAT5 (Figure 3), which then translocates to the nucleus to support NK cell survival [38]. In parallel, it induces the activation of the PI3K-AKT-mTOR signaling pathway which promotes the acquisition of cytolytic potential by NK cells when they will encounter abnormal cells (Figure 3) [38].
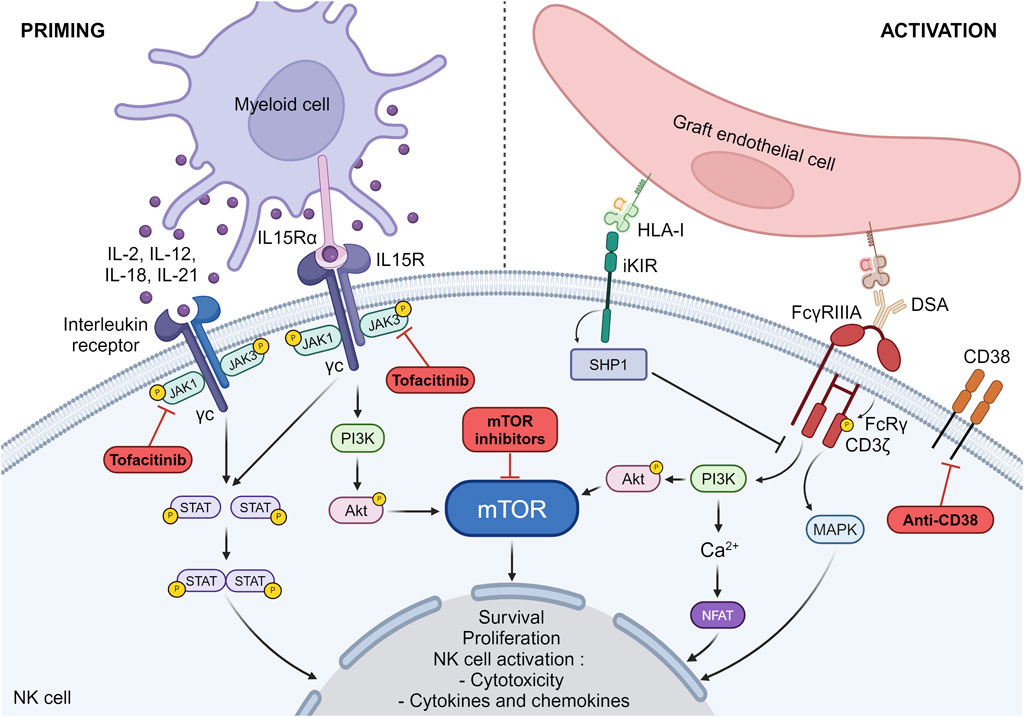
Figure 3. NK cell priming and activation pathways. The pathways involved in NK cell priming and activation as well as the drugs susceptible to block these pathways are depicted. IL15Rα, IL-15 receptor alpha subunit; IL15R, IL-15 receptor; DSA, Donor specific antibody; iKIR, inhibitory killer cell immunoglobulin-like receptors; MAPK, mitogen-activated protein kinase; HLA-I, Human Leucocyte Antigen class I; NFAT, nuclear factor of activated T-cells.
In the context of transplantation, we and others have shown that NK cells need to undergo priming to get activated against the graft [15, 39, 40]. In particular, we have shown that prolonged cold ischemia (suggesting significant ischemia-reperfusion injury) and viral infections such as cytomegalovirus can lead to NK cell priming after transplantation, a prerequisite for NK cell activation and the development of chronic vascular rejection in the presence of a missing self [15]. These two types of events favor the induction of IL-15 and its trans presentation by dendritic cells, thereby promoting NK cell cycle entry, proliferation, survival and cytotoxicity [35, 41–44]. In addition, cytomegalovirus also induces a remodeling of the NK phenotype with an increase in NK cell populations expressing educated inhibitory KIRs and expression of markers such as NKG2C and CD57 [45, 46]. This subpopulation of NK cells is considered to be a memory like population and is known to be better at exhibiting cytotoxicity and secreting cytokines such as IFN-γ [46].
Finally, a recent study also showed that IL-21 may help NK cells to differentiate into polyfunctional type 1 activated cytotoxic effectors with a potentially deleterious ability to infiltrate the kidney allograft during AMR and damage the vascular endothelium [47].
NK Cell Activation
Activating NK cell receptors are associated with different adaptor molecules which contain immunoreceptor tyrosine-based activation motifs (ITAMs) on their cytoplasmic domains. FcγRIIIa receptor which is a low affinity receptor for IgG forms a complex with 2 adaptor molecules FcRγ and CD3ζ. Upon ligation of the Fc fragment of IgG by FcγRIIIa, the ITAMs are phosphorylated. This triggers a cascade of downstream events involving the PI3K pathway, the mitogen-activated protein kinase (MAPK) pathway and calcium release conducting to the activation of nuclear factor of activated T-cells (NFAT) (Figure 1) [48–50]. Together, these pathways contribute to the cellular response leading to NK cell activation [51–53]. Unlike activating receptors, inhibitory receptors and in particular inhibitory KIRs have 2 ITIMs in their cytoplasmic tails. When inhibitory KIRs interact with their self-MHC class I ligands, the ITIMs are phosphorylated and recruit the tyrosine phosphatases such as SHP-1 (Src homology containing tyrosine phosphatase-1), which remove phosphate groups from several proteins downstream of activating receptors, thereby preventing NK cell activation (Figure 1) [54]. In the absence of ligation of their self-MHC class I ligands, inhibitory KIRs do not interfere with activating signals, allowing NK cell activation. We have shown that missing cell induced-NK cell activation triggers the mTOR pathway [15]. Whether this is also the case for FcγRIIIa-induced NK cell activation is not clear, but experimental data investigating NK cell activation by other activating receptors suggest that this is certainly the case [55].
Activation of NK cells can lead to killing of the target cell. NK cells exert their cytotoxic activity through two main pathways: the exocytosis of lytic granules containing perforin and granzymes A and B; and the expression of ligands (FAS ligand, TRAIL) of death receptors (FAS, DR4 and DR5) expressed on target cells. In addition, NK cells can secrete pro-inflammatory cytokines (TNF-α and INF-γ) that favor the activation of dendritic cells, T cells and monocytes [56]. They also secrete chemokines such as CCL-2 (MCP-1), CCL3 (MIP-1α), CCL4 (MIP-1β), CCL5 (RANTES), which attract effector lymphocytes and myeloid cells to inflamed tissues [57, 58]. In a murine heart transplantation model of AMR, Lin and colleagues showed that both IFN-γ and contact-dependent cytotoxicity are necessary for NK cells to induce chronic vascular lesions [59]. The same observations have been made in the hybrid resistance model regarding the need for IFN-γ to help NK cells recruit T cells and monocytes to induce the vascular lesions [7, 8]. Several clinical studies have shown that the transcripts involved in IFN-γ pathway and NK cell-mediated cytotoxicity are upregulated in biopsies of patients with HLA positive or negative microvascular inflammation lesions [28, 34].
Targeting NK Cells: An Interesting Lead to Prevent and Treat Chronic Vascular Rejection?
Prevention of NK-Driven Chronic Rejection
Transplant patients classically received a two- or three-drug regimen consisting of a calcineurin inhibitor, an antiproliferative agent, and corticosteroids. The aim of these treatments is to inhibit T-cell activation. Theoretically, calcineurin inhibitors could have an effect on NK cell activation because NFAT is expressed by NK cells [50]. In a first in vitro study, human NK cells were treated with cyclosporine and different cytokines (IL-2 or IL-15) for 7 days. This study confirmed that the NFAT pathway was blocked, especially in CD56dimCD16+inhKIR+ NK cells, and that this resulted in a decrease in NK cell proliferation but an increase in cytotoxicity against different target cells and an increased ability to secrete IFN-γ after a new stimulation with IL-12 and IL-18 [60]. Two more recent studies confirm the conserved cytotoxicity of NK cells exposed to cyclosporine or tacrolimus and activated by the two mechanisms involved in chronic rejection (MS and ADCC) [61, 62]. In vivo, we confirm that cyclosporine does not prevent missing-self-induced NK-mediated rejection in our murine heart transplant model, suggesting that this immunosuppressant may have a limited effect in preventing chronic vascular rejection in patients [15]. The effect of antiproliferative drugs such as azathioprine and mycophenolate mofetil and low-dose corticosteroids on NK cells has been less studied. Some in vitro studies suggest that these drugs may prevent NK cell activation, but these data should be treated with caution due to the lack of in vivo data [61, 62].
Alternatively, transplant recipients may receive mTOR inhibitors (mammalian target of rapamycin) as part of their maintenance regimen. As previously depicted, mTOR pathway plays an important role in NK cell education, priming and activation [38, 55]. In vitro studies have shown that mTOR inhibitors can prevent NK cell cytotoxicity [55, 61]. In vivo, we confirm that rapamycin can efficiently prevent missing-self induced NK-mediated rejection in our murine heart transplantation model [33]. Finally, a recent clinical study in a cohort of lung transplant recipients treated with rapamycin showed that their NK cells had reduced mTOR activity, which was associated with decreased cell proliferation and lower levels of FcRγ, the adaptor molecule of FcγRIIIa, suggesting that they may be less efficient at performing ADCC [63]. A pilot clinical trial (NCT03955172) is currently underway to assess the efficacy of mTOR inhibitors in preventing the formation of chronic lesions in kidney transplant patients presenting a missing-self induced NK-mediated rejection. Although promising, the universality of mTOR inhibitors could be questioned, as this treatment is associated with many side effects, leading to its discontinuation in 30%–50% of patients, and cannot be used late in the course of chronic rejection, as mTOR inhibitors are poorly tolerated in patients with chronic glomerular lesions, as they prevent the adaptation of podocytes to stress [64].
As the cytokine priming process is necessary for all NK cell mechanisms involved in chronic vascular rejection, blocking it offers a unique opportunity to prevent the deleterious effects of NK cells during chronic vascular rejection. As NK cell priming may be the result of multiple cytokines, rather than trying to block one cytokine, blocking the pathways which result from the binding of cytokines on their receptors which share the common γc chain may be more appropriate. Tofacitinib is an oral JAK inhibitor that selectively inhibit intracellular cytokine signaling mediated by JAK3 and/or JAK1. A recent study in a rat model of mixed cellular and antibody-mediated rejection indicates that tofacitinib effectively reduces the infiltration of T cells and NK cells into the graft, thereby limiting the progression of lesions and improving graft and recipient survival [65]. Its use in renal transplant patients has been tested in a clinical randomized phase 2b trial [66]. Patients received either tofacitinib (low or high dose) or cyclosporine in combination with mycophenolic acid and corticosteroids [66]. Tofacitinib was equivalent to cyclosporine in preventing acute rejection and was associated with an improved renal function and less tubular atrophy and interstitial fibrosis [66]. However, it was also responsible for more cancerous and infectious complications in the high dose tofacitinib regimen [66]. This side effect may be the reflect of the efficient blocking of NK cells which are well known for their role in anti-viral and anti-tumoral immunity.
Treatment of NK-Driven Chronic Rejection
The current consensus for the treatment of AMR associates rapid depletion of circulating DSA with plasmapheresis with a combination of corticosteroids and high-dose intravenous immunoglobulins (IVIg) [67]. This costly and prolonged therapeutic approach has a reported 3-year graft survival of <50% [67]. By preventing complement activation, these treatments slow down the course of rejection and convert it from acute to chronic rejection in which ADCC is the main mechanism of graft destruction.
Few studies have investigated the effect of high-dose corticosteroids on NK cells. In one in vitro study, human purified NK cells and T cells were incubated with elevated doses of hydrocortisone, comparable to drug levels achieved in patients receiving 1 mg/kg to 1 g of methylprednisolone. In contrast to T cells, NK cells were resistant to steroid-induced apoptosis and their cytolytic capacity against MHC class I deficient tumoral cell was not affected [68]. In the same line, although methylprednisolone seems to affect NK cell activation through some activating receptors such as NKp46, NKG2D or 2B4, it does not seem to prevent FcγRIIIA-mediated NK cell cytotoxicity [69]. High dose IVIg may also prevent NK cell activation by saturating all FcγRIIIa receptors present on NK cells, rendering them blind to DSA. However, their impact on NK cells is still debated [67].
To achieve a sustained therapeutic effect in AMR, attempts have been made to target DSA-producing cells with either anti-CD20 monoclonal depleting antibody (rituximab) or a proteasome inhibitor (bortezomib) without efficacy [70, 71]. Recently, an antibody targeting CD38 (felzartamab), a receptor present on plasma cells and NK cells, has been tested in a randomized phase 2 trial study for the treatment of renal transplant recipients diagnosed with late active or chronic-active antibody-mediated rejection after kidney transplantation [72]. This treatment efficiently reduced rejection lesions. This improvement seems to be due to NK cell depletion rather than plasma cells depletion as DSA MFI barely decreased with the treatment. These data need to be confirmed in a larger cohort of patients. A further clinical trial is currently underway to test an alternative anti-CD38 (daratumumab) in the same indication (NCT05913596). If effective, its use can be extended to missing self-induced NK-mediated rejection.
Conclusion
In conclusion, this review has explored the role of NK cells in chronic vascular rejection. They appear to be the masters of chronic vascular rejection, regardless of whether DSA are present or not. This makes them a prime target for attempts to prevent and/or treat chronic vascular rejection, which is currently the leading cause of allograft loss. Inhibition of NK cell priming and activation represent interesting avenues for preventing NK-mediated chronic vascular rejection, while drugs that deplete NK cells may be of interest for the treatment of chronic vascular rejection. By reducing chronic vascular rejection, these strategies may lead to prolonged allograft survival.
Author Contributions
All authors listed have made a substantial, direct, and intellectual contribution to the work and approved it for publication.
Funding
The authors declare that no financial support was received for the research, authorship, and/or publication of this article. MC is funded by ANR (ANR-21-CE17-0057) and AK is supported by ANR (ANR-21-CE17-0057) and HORIZON EUROPE grant n°101057651.
Conflict of Interest
The authors declare that the research was conducted in the absence of any commercial or financial relationships that could be construed as a potential conflict of interest.
Generative AI Statement
The author(s) declare that no Generative AI was used in the creation of this manuscript.
References
1. Tonelli, M, Wiebe, N, Knoll, G, Bello, A, Browne, S, Jadhav, D, et al. Systematic Review: Kidney Transplantation Compared With Dialysis in Clinically Relevant Outcomes. Am J Transpl (2011) 11(10):2093–109. doi:10.1111/j.1600-6143.2011.03686.x
2. Lamb, KE, Lodhi, S, and Meier-Kriesche, H-U. Long-Term Renal Allograft Survival in the United States: A Critical Reappraisal. Am J Transpl (2011) 11(3):450–62. doi:10.1111/j.1600-6143.2010.03283.x
3. Loupy, A, and Lefaucheur, C. Antibody-Mediated Rejection of Solid-Organ Allografts. N Engl J Med (2018) 379(12):1150–60. doi:10.1056/NEJMra1802677
4. Thielens, A, Vivier, E, and Romagné, F. NK Cell MHC Class I Specific Receptors (KIR): From Biology to Clinical Intervention. Curr Opin Immunol (2012) 24(2):239–45. avr. doi:10.1016/j.coi.2012.01.001
5. Uhrberg, M, Valiante, NM, Shum, BP, Shilling, HG, Lienert-Weidenbach, K, Corliss, B, et al. Human Diversity in Killer Cell Inhibitory Receptor Genes. Immunity (1997) 7(6):753–63. doi:10.1016/S1074-7613(00)80394-5
6. Höglund, P, and Brodin, P. Current Perspectives of Natural Killer Cell Education by MHC Class I Molecules. Nat Rev Immunol (2010) 10(10):724–34. doi:10.1038/nri2835
7. Uehara, S, Chase, CM, Kitchens, WH, Rose, HS, Colvin, RB, Russell, PS, et al. NK Cells Can Trigger Allograft Vasculopathy: The Role of Hybrid Resistance in Solid Organ Allografts. J Immunol (2005) 175(5):3424–30. doi:10.4049/jimmunol.175.5.3424
8. Kitchens, WH, Chase, CM, Uehara, S, Cornell, LD, Colvin, RB, Russell, PS, et al. Macrophage Depletion Suppresses Cardiac Allograft Vasculopathy in Mice. Am J Transpl (2007) 7(12):2675–82. doi:10.1111/j.1600-6143.2007.01997.x
9. Kreijveld, E, Van Der Meer, A, Tijssen, HJ, Hilbrands, LB, and Joosten, I. KIR Gene and KIR Ligand Analysis to Predict Graft Rejection After Renal Transplantation. Transplantation (2007) 84(8):1045–51. doi:10.1097/01.tp.0000286097.11173.70
10. Kunert, K, Seiler, M, Mashreghi, MF, Klippert, K, Schönemann, C, Neumann, K, et al. KIR/HLA Ligand Incompatibility in Kidney Transplantation. Transplantation (2007) 84(11):1527–33. doi:10.1097/01.tp.0000290681.41859.41
11. Tran, TH, Mytilineos, J, Scherer, S, Laux, G, Middleton, D, and Opelz, G. Analysis of KIR Ligand Incompatibility in Human Renal Transplantation. Transplantation (2005) 80(8):1121–3. doi:10.1097/01.tp.0000179110.15304.90
12. Hanvesakul, R, Kubal, C, Moore, J, Neil, D, Cook, M, Ball, S, et al. KIR and HLA-C Interactions Promote Differential Dendritic Cell Maturation and Is a Major Determinant of Graft Failure Following Kidney Transplantation. PLoS ONE (2011) 6(8):e23631. août. doi:10.1371/journal.pone.0023631
13. Tran, TH, Middleton, D, Döhler, B, Scherer, S, Meenagh, A, Sleator, C, et al. Reassessing the Impact of Donor HLA-C Genotype on Long-Term Liver Transplant Survival. Am J Transpl (2009) 9(7):1674–8. doi:10.1111/j.1600-6143.2009.02609.x
14. Van Bergen, J, Thompson, A, Haasnoot, GW, Roodnat, JI, de Fijter, JW, Claas, FHJ, et al. KIR-ligand Mismatches Are Associated With Reduced Long-Term Graft Survival in HLA-Compatible Kidney Transplantation. Am J Transpl (2011) 11(9):1959–64. doi:10.1111/j.1600-6143.2011.03621.x
15. Koenig, A, Chen, CC, Marçais, A, Barba, T, Mathias, V, Sicard, A, et al. Missing Self Triggers NK Cell-Mediated Chronic Vascular Rejection of Solid Organ Transplants. Nat Commun (2019) 10(1):5350. doi:10.1038/s41467-019-13113-5
16. Callemeyn, J, Senev, A, Coemans, M, Lerut, E, Sprangers, B, Kuypers, D, et al. Missing Self–Induced Microvascular Rejection of Kidney Allografts: A Population-Based Study. J Am Soc Nephrol (2021) 32(8):2070–82. doi:10.1681/ASN.2020111558
17. Loupy, A, Lefaucheur, C, Vernerey, D, Prugger, C, Duong van Huyen, JP, Mooney, N, et al. Complement-Binding Anti-HLA Antibodies and Kidney-Allograft Survival. N Engl J Med (2013) 369(13):1215–26. doi:10.1056/NEJMoa1302506
18. Sicard, A, Ducreux, S, Rabeyrin, M, Couzi, L, McGregor, B, Badet, L, et al. Detection of C3d-Binding Donor-Specific Anti-HLA Antibodies at Diagnosis of Humoral Rejection Predicts Renal Graft Loss. J Am Soc Nephrol (2015) 26(2):457–67. doi:10.1681/ASN.2013101144
19. Guidicelli, G, Guerville, F, Lepreux, S, Wiebe, C, Thaunat, O, Dubois, V, et al. Non-Complement–Binding De Novo Donor-Specific Anti-HLA Antibodies and Kidney Allograft Survival. J Am Soc Nephrol (2016) 27(2):615–25. doi:10.1681/ASN.2014040326
20. Hirohashi, T, Chase, CM, Della Pelle, P, Sebastian, D, Alessandrini, A, Madsen, JC, et al. A Novel Pathway of Chronic Allograft Rejection Mediated by NK Cells and Alloantibody. Am J Transpl (2012) 12(2):313–21. doi:10.1111/j.1600-6143.2011.03836.x
21. Hidalgo, LG, Sis, B, Sellares, J, Campbell, PM, Mengel, M, Einecke, G, et al. NK Cell Transcripts and NK Cells in Kidney Biopsies From Patients With Donor-Specific Antibodies: Evidence for NK Cell Involvement in Antibody-Mediated Rejection. Am J Transpl (2010) 10(8):1812–22. doi:10.1111/j.1600-6143.2010.03201.x
22. Lamarthée, B, Callemeyn, J, Van Herck, Y, Antoranz, A, Anglicheau, D, Boada, P, et al. Transcriptional and Spatial Profiling of the Kidney Allograft Unravels a Central Role for FcyRIII+ Innate Immune Cells in Rejection. Nat Commun (2023) 14(1):4359. doi:10.1038/s41467-023-39859-7
23. Shah, Y, Yang, H, Mueller, FB, Li, C, Gul Rahim, SE, Varma, E, et al. Transcriptomic Signatures of Chronic Active Antibody-Mediated Rejection Deciphered by RNA Sequencing of Human Kidney Allografts. Kidney Int (2024) 105(2):347–63. doi:10.1016/j.kint.2023.11.012
24. Bharadwaj, P, Shrestha, S, Pongracz, T, Concetta, C, Sharma, S, Le Moine, A, et al. Afucosylation of HLA-Specific IgG1 as a Potential Predictor of Antibody Pathogenicity in Kidney Transplantation. Cell Rep. Med (2022) 3(11):100818. doi:10.1016/j.xcrm.2022.100818
25. Beyze, A, Larroque, C, and Le Quintrec, M. The Role of Antibody Glycosylation in Autoimmune and Alloimmune Kidney Diseases. Nat Rev Nephrol (2024) 20(10):672–89. doi:10.1038/s41581-024-00850-0
26. Yazdani, S, Callemeyn, J, Gazut, S, Lerut, E, de Loor, H, Wevers, M, et al. Natural Killer Cell Infiltration Is Discriminative for Antibody-Mediated Rejection and Predicts Outcome After Kidney Transplantation. Kidney Int (2019) 95(1):188–98. doi:10.1016/j.kint.2018.08.027
27. Venner, JM, Hidalgo, LG, Famulski, KS, Chang, J, and Halloran, PF. The Molecular Landscape of Antibody-Mediated Kidney Transplant Rejection: Evidence for NK Involvement Through CD16a Fc Receptors. Am J Transpl (2015) 15(5):1336–48. doi:10.1111/ajt.13115
28. Parkes, MD, Halloran, PF, and Hidalgo, LG. Evidence for CD16a-Mediated NK Cell Stimulation in Antibody-Mediated Kidney Transplant Rejection. Transplantation (2017) 101(4):e102–11. doi:10.1097/TP.0000000000001586
29. Mahaweni, NM, Olieslagers, TI, Rivas, IO, Molenbroeck, SJJ, Groeneweg, M, Bos, GMJ, et al. A Comprehensive Overview of FCGR3A Gene Variability by Full-Length Gene Sequencing Including the Identification of V158F Polymorphism. Sci Rep (2018) 8(1):15983. doi:10.1038/s41598-018-34258-1
30. Arnold, ML, Kainz, A, Hidalgo, LG, Eskandary, F, Kozakowski, N, Wahrmann, M, et al. Functional Fc Gamma Receptor Gene Polymorphisms and Donor-Specific Antibody-Triggered Microcirculation Inflammation. Am J Transpl (2018) 18(9):2261–73. doi:10.1111/ajt.14710
31. Litjens, N, Peeters, A, Gestel, JK, Klepper, M, Betjes, M, et al. The FCGR3A 158 V/V-Genotype Is Associated With Decreased Survival of Renal Allografts With Chronic Active Antibody-Mediated Rejection. Sci Rep (2021) 11(1):7903. doi:10.1038/s41598-021-86943-3
32. Diebold, M, Vietzen, H, Heinzel, A, Haindl, S, Herz, CT, Mayer, K, et al. Natural Killer Cell Functional Genetics and Donor-Specific Antibody-Triggered Microvascular Inflammation. Am J Transpl (2024) 24(5):743–54. doi:10.1016/j.ajt.2023.12.005
33. Koenig, A, Mezaache, S, Callemeyn, J, Barba, T, Mathias, V, Sicard, A, et al. Missing Self-Induced Activation of NK Cells Combines With Non-Complement-Fixing Donor-Specific Antibodies to Accelerate Kidney Transplant Loss in Chronic Antibody-Mediated Rejection. J Am Soc Nephrol (2021) 32(2):479–94. doi:10.1681/ASN.2020040433
34. Callemeyn, J, Lerut, E, de Loor, H, Arijs, I, Thaunat, O, Koenig, A, et al. Transcriptional Changes in Kidney Allografts With Histology of Antibody-Mediated Rejection Without Anti-HLA Donor-Specific Antibodies. J Am Soc Nephrol (2020) 31(9):2168–83. doi:10.1681/ASN.2020030306
35. Lucas, M, Schachterle, W, Oberle, K, Aichele, P, and Diefenbach, A. Dendritic Cells Prime Natural Killer Cells by Trans-Presenting Interleukin 15. Immunity (2007) 26(4):503–17. doi:10.1016/j.immuni.2007.03.006
36. Guia, S, Cognet, C, de Beaucoudrey, L, Tessmer, MS, Jouanguy, E, Berger, C, et al. A Role for Interleukin-12/23 in the Maturation of Human Natural Killer and CD56+ T Cells In Vivo. Blood (2008) 111(10):5008–16. doi:10.1182/blood-2007-11-122259
37. Chaix, J, Tessmer, MS, Hoebe, K, Fuséri, N, Ryffel, B, Dalod, M, et al. Cutting Edge: Priming of NK Cells by IL-18. J Immunol (2008) 181(3):1627–31. doi:10.4049/jimmunol.181.3.1627
38. Marçais, A, Cherfils-Vicini, J, Viant, C, Degouve, S, Viel, S, Fenis, A, et al. The Metabolic Checkpoint Kinase mTOR Is Essential for IL-15 Signaling During the Development and Activation of NK Cells. Nat Immunol (2014) 15(8):749–57. doi:10.1038/ni.2936
39. Kroemer, A, Xiao, X, Degauque, N, Edtinger, K, Wei, H, Demirci, G, et al. The Innate NK Cells, Allograft Rejection, and a Key Role for IL-15. J Immunol (2008) 180(12):7818–26. doi:10.4049/jimmunol.180.12.7818
40. Graham, JA, Wilkinson, RA, Hirohashi, T, Chase, CM, Colvin, RB, Madsen, JC, et al. Viral Infection Induces De Novo Lesions of Coronary Allograft Vasculopathy Through a Natural Killer Cell-Dependent Pathway. Am J Transpl (2009) 9(11):2479–84. doi:10.1111/j.1600-6143.2009.02801.x
41. Silvis, MJM, Kaffka Genaamd Dengler, SE, Odille, CA, Mishra, M, van der Kaaij, NP, Doevendans, PA, et al. Damage-Associated Molecular Patterns in Myocardial Infarction and Heart Transplantation: The Road to Translational Success. Front Immunol (2020) 11:599511. doi:10.3389/fimmu.2020.599511
42. Mattei, F, Schiavoni, G, Belardelli, F, and Tough, DF. IL-15 Is Expressed by Dendritic Cells in Response to Type I IFN, Double-Stranded RNA, or Lipopolysaccharide and Promotes Dendritic Cell Activation. J Immunol (2001) 167(3):1179–87. doi:10.4049/jimmunol.167.3.1179
43. Baranek, T, Manh, TPV, Alexandre, Y, Maqbool, MA, Cabeza, JZ, Tomasello, E, et al. Differential Responses of Immune Cells to Type I Interferon Contribute to Host Resistance to Viral Infection. Cell Host Microbe (2012) 12(4):571–84. doi:10.1016/j.chom.2012.09.002
44. Nguyen, KB, Salazar-Mather, TP, Dalod, MY, Van Deusen, JB, Wei, X-q, Liew, FY, et al. Coordinated and Distinct Roles for IFN-Αβ, IL-12, and IL-15 Regulation of NK Cell Responses to Viral Infection. J Immunol (2002) 169(8):4279–87. doi:10.4049/jimmunol.169.8.4279
45. Lopez-Vergès, S, Milush, JM, Schwartz, BS, Pando, MJ, Jarjoura, J, York, VA, et al. Expansion of a Unique CD57 + NKG2Chi Natural Killer Cell Subset During Acute Human Cytomegalovirus Infection. Proc Natl Acad Sci (2011) 108(36):14725–32. doi:10.1073/pnas.1110900108
46. Béziat, V, Dalgard, O, Asselah, T, Halfon, P, Bedossa, P, Boudifa, A, et al. CMV Drives Clonal Expansion of NKG2C + NK Cells Expressing Self-specific KIRs in Chronic Hepatitis Patients. Eur J Immunol (2012) 42(2):447–57. doi:10.1002/eji.201141826
47. Bailly, E, Macedo, C, Ossart, J, Louis, K, Gu, X, Ramaswami, B, et al. Interleukin-21 Promotes Type-1 Activation and Cytotoxicity of CD56dimCD16bright Natural Killer Cells During Kidney Allograft Antibody–Mediated Rejection Showing a New Link Between Adaptive and Innate Humoral Allo-Immunity. Kidney Int (2023) 104(4):707–23. doi:10.1016/j.kint.2023.04.024
48. Ståhls, A, Liwszyc, GE, Couture, C, Mustelin, T, and Andersson, LC. Triggering of Human Natural Killer Cells Through CD16 Induces Tyrosine Phosphorylation of the P72syk Kinase. Eur J Immunol (1994) 24(10):2491–6. doi:10.1002/eji.1830241035
49. Ting, AT, Dick, CJ, Schoon, RA, Karnitz, LM, Abraham, RT, Leibson, PJ, et al. Interaction Between Lck and Syk Family Tyrosine Kinases in Fcγ Receptor-Initiated Activation of Natural Killer Cells. J Biol Chem (1995) 270(27):16415–21. doi:10.1074/jbc.270.27.16415
50. Aramburu, J, Azzoni, L, Rao, A, and Perussia, B. Activation and Expression of the Nuclear Factors of Activated T Cells, NFATp and NFATc, in Human Natural Killer Cells: Regulation Upon CD16 Ligand Binding. J Exp Med (1995) 182(3):801–10. doi:10.1084/jem.182.3.801
51. Brumbaugh, KM, Binstadt, BA, Billadeau, DD, Schoon, RA, Dick, CJ, Ten, RM, et al. Functional Role for Syk Tyrosine Kinase in Natural Killer Cell–Mediated Natural Cytotoxicity. J Exp Med (1997) 186(12):1965–74. doi:10.1084/jem.186.12.1965
52. Ben Mkaddem, S, Benhamou, M, and Monteiro, RC. Understanding Fc Receptor Involvement in Inflammatory Diseases: From Mechanisms to New Therapeutic Tools. Front Immunol (2019) 10:811, avr. doi:10.3389/fimmu.2019.00811
53. Daëron, M, Malbec, O, Uénard, H, Bruhns, P, and Fridman, WH. Immunoreceptor Tyrosine-Based Inhibition Motif-Dependent Negative Regulation of Mast Cell Activation and Proliferation. In: Mast Cells and Basophils. Elsevier (2000). p. 185–93. doi:10.1016/B978-012473335-0/50014-3
54. MacFarlane, AW, and Campbell, KS, Signal Transduction in Natural Killer Cells. In: Current Topics in Microbiology and Immunology, vol. 298, RW Compans, MD Cooper, T Honjo, H Koprowski, F Melchers, MBA Oldstoneet al. Éd., Berlin, Heidelberg: Springer, 2006, p. 23–57. doi:10.1007/3-540-27743-9_2
55. Marçais, A, Marotel, M, Degouve, S, Koenig, A, Fauteux-Daniel, S, Drouillard, A, et al. High mTOR Activity Is a Hallmark of Reactive Natural Killer Cells and Amplifies Early Signaling Through Activating Receptors. eLife (2017) 6:e26423. doi:10.7554/eLife.26423
56. Vivier, E, Tomasello, E, Baratin, M, Walzer, T, and Ugolini, S. Functions of Natural Killer Cells. Nat Immunol (2008) 9(5):503–10. doi:10.1038/ni1582
57. Fauriat, C, Long, EO, Ljunggren, H-G, Bryceson, YT, et al. Regulation of Human NK-Cell Cytokine and Chemokine Production by Target Cell Recognition. Blood (2010) 115(11):2167–76. doi:10.1182/blood-2009-08-238469
58. Bluman, EM, Bartynski, KJ, Avalos, BR, and Caligiuri, MA. Human Natural Killer Cells Produce Abundant Macrophage Inflammatory Protein-1 Alpha in Response to Monocyte-Derived Cytokines. J Clin Invest (1996) 97(12):2722–7. juin. doi:10.1172/JCI118726
59. Lin, CM, Plenter, RJ, Coulombe, M, and Gill, RG. Interferon Gamma and Contact-Dependent Cytotoxicity Are Each Rate Limiting for Natural Killer Cell–Mediated Antibody-Dependent Chronic Rejection. Am J Transpl (2016) 16(11):3121–30. doi:10.1111/ajt.13865
60. Wang, S-Y, Racila, E, Taylor, RP, and Weiner, et GJ. NK-Cell Activation and Antibody-Dependent Cellular Cytotoxicity Induced by Rituximab-Coated Target Cells Is Inhibited by the C3b Component of Complement. Blood (2008) 111(3):1456–63. doi:10.1182/blood-2007-02-074716
61. Qin, R, Qin, J, Li, X, Xu, Z, Yuan, X, et al. Influence of Immunosuppressive Drugs on Natural Killer Cells in Therapeutic Drug Exposure in Liver Transplantation. Hepatobiliary Surg Nutr (2023) 12(6):835–53. doi:10.21037/hbsn-22-438
62. Jalali, S, Stankovic, S, Westall, GP, Reading, PC, Sullivan, LC, and Brooks, et AG. Examining the Impact of Immunosuppressive Drugs on Antibody-Dependent Cellular Cytotoxicity (ADCC) of Human Peripheral Blood Natural Killer (NK) Cells and Gamma Delta (γδ) T Cells. Transpl Immunol (2024) 82:101962. doi:10.1016/j.trim.2023.101962
63. Shemesh, A, Su, Y, Calabrese, DR, Chen, D, Arakawa-Hoyt, J, Roybal, KT, et al. Diminished Cell Proliferation Promotes Natural Killer Cell Adaptive-Like Phenotype by Limiting FcεRIγ Expression. J Exp Med (2022) 219(11):e20220551. doi:10.1084/jem.20220551
64. Canaud, G, Bienaimé, F, Viau, A, Treins, C, Baron, W, Nguyen, C, et al. AKT2 Is Essential to Maintain Podocyte Viability and Function During Chronic Kidney Disease. Nat Med (2013) 19(10):1288–96. doi:10.1038/nm.3313
65. Rovira, J, Ramírez-Bajo, MJ, Banon-Maneus, E, Lazo-Rodríguez, M, Moya-Rull, D, Hierro-Garcia, N, et al. Tofacitinib Halts Progression of Graft Dysfunction in a Rat Model of Mixed Cellular and Humoral Rejection. Transplantation (2018) 102(7):1075–84. doi:10.1097/TP.0000000000002204
66. Vincenti, F, Tedesco Silva, H, Busque, S, O'Connell, P, Friedewald, J, Cibrik, D, et al. Randomized Phase 2b Trial of Tofacitinib (CP-690,550) in De Novo Kidney Transplant Patients: Efficacy, Renal Function and Safety at 1 Year. Am J Transpl (2012) 12(9):2446–56. doi:10.1111/j.1600-6143.2012.04127.x
67. Lefaucheur, C, Nochy, D, Andrade, J, Verine, J, Gautreau, C, Charron, D, et al. Comparison of Combination Plasmapheresis/IVIg/Anti-CD20 Versus High-Dose IVIg in the Treatment of Antibody-Mediated Rejection. Am J Transpl (2009) 9(5):1099–107. doi:10.1111/j.1600-6143.2009.02591.x
68. Ramanathan, M, Lundqvist, A, Yokoyama, H, Smith, A, and Childs, R. Natural Killer (NK) Cells Are Resistant to the Apoptotic Effects of Corticosteroids Compared to T Cells: Implications for Adoptive NK Cell Therapy Following Allogeneic HCT. Biol Blood Marrow Transpl (2009) 15(2):9–10. doi:10.1016/j.bbmt.2008.12.026
69. Chiossone, L, Vitale, C, Cottalasso, F, Moretti, S, Azzarone, B, Moretta, L, et al. Molecular Analysis of the Methylprednisolone-Mediated Inhibition of NK-Cell Function: Evidence for Different Susceptibility of IL-2– Versus IL-15–Activated NK Cells. Blood (2007) 109(9):3767–75. doi:10.1182/blood-2006-07-037846
70. Sautenet, B, Blancho, G, Büchler, M, Morelon, E, Toupance, O, Barrou, B, et al. One-Year Results of the Effects of Rituximab on Acute Antibody-Mediated Rejection in Renal Transplantation: RITUX ERAH, a Multicenter Double-Blind Randomized Placebo-Controlled Trial. Transplantation (2016) 100(2):391–9. doi:10.1097/TP.0000000000000958
71. Eskandary, F, Regele, H, Baumann, L, Bond, G, Kozakowski, N, Wahrmann, M, et al. A Randomized Trial of Bortezomib in Late Antibody-Mediated Kidney Transplant Rejection. J Am Soc Nephrol (2018) 29(2):591–605. doi:10.1681/ASN.2017070818
Keywords: antibody mediated rejection, chronic rejection, natural killer cells, missing self, antibody dependent cellular cytotoxicity
Citation: Chambon M and Koenig A (2024) NK Cells: Not Just Followers But Also Initiators of Chronic Vascular Rejection. Transpl Int 37:13318. doi: 10.3389/ti.2024.13318
Received: 30 May 2024; Accepted: 23 September 2024;
Published: 16 October 2024.
Copyright © 2024 Chambon and Koenig. This is an open-access article distributed under the terms of the Creative Commons Attribution License (CC BY). The use, distribution or reproduction in other forums is permitted, provided the original author(s) and the copyright owner(s) are credited and that the original publication in this journal is cited, in accordance with accepted academic practice. No use, distribution or reproduction is permitted which does not comply with these terms.
*Correspondence: Alice Koenig, YWxpY2Uua29lbmlnQGluc2VybS5mcg==