- 1Prague Lung Transplant Program, 3rd Department of Surgery, First Faculty of Medicine, Charles University and Motol University Hospital, Prague, Czechia
- 2Laboratory of Respiratory Diseases and Thoracic Surgery (BREATHE), Department of Chronic Diseases and Metabolism, KU Leuven, Leuven, Belgium
- 3Department of Pathology and Molecular Medicine, Second Faculty of Medicine, Charles University and Motol University Hospital, Prague, Czechia
- 4Department of Immunogenetics, Institute for Clinical and Experimental Medicine, Prague, Czechia
- 5Department of Immunology, Second Faculty of Medicine, Charles University and Motol University Hospital, Prague, Czechia
- 6Department of Public Health and Primary Care, Leuven Biostatistics and Statistical Bioinformatics Center (L-BioStat), KU Leuven, Leuven, Belgium
- 7Department of Anesthesiology, Resuscitation and Intensive Care Medicine, Second Faculty of Medicine, Charles University and Motol University Hospital, Prague, Czechia
- 8Department of Obstetrics and Gynecology, First Faculty of Medicine, Charles University, General University Hospital, Prague, Czechia
- 9Department of Thoracic Surgery, University Hospitals Leuven, Leuven, Belgium
Acute cellular rejection (ACR) frequently occurs following lung transplantation (LuTx) and represents a risk factor for the development of chronic lung allograft dysfunction (CLAD) as well as long-term survival. The histopathological diagnosis of ACR carries a burden of interobserver variability. The widespread utilization and cost-effectiveness of immunohistochemistry (IHC) was proven beneficial in diagnosing rejection in human kidney transplantations and LuTx rat models. However, its potential for ACR detection in patients remains unexplored. We analyzed surface markers (CD3, CD4, CD8, CD20, CD68, CD47, PD-1, PD-L1, and CD31/PECAM-1) on lung tissue cryobiopsy samples collected within 6 months post-LuTx from 60 LuTx recipients, 48 of whom were diagnosed with ACR. Additionally, serum samples from 51 patients were analyzed using a multiplex bead-based Luminex assay. The cytokines and markers included PD-L1, IL2, TNFα, IFNγ, and Granzyme B. We observed a significant increase in PD-L1 tissue expression within the rejection group, suggesting a concerted effort to suppress immune responses, especially those mediated by T-cells. Furthermore, we noted significant differences in PECAM-1 levels between ACR/non-ACR. Additionally, peripheral blood C-reactive-protein levels tended to be higher in the ACR group, while Luminex serum analyses did not reveal any significant differences between groups. In conclusion, our findings suggest the potential value of PECAM-1 and PD-L1 markers in diagnosing ACR.
Introduction
Long-term allograft survival has always been significantly challenged by the persistent risk of transplantation rejection [1–4]. During transplantation, both ischemia-reperfusion and mechanical injury as well as inadequate organ storage conditions prompt immune system reactions through the local release of cytokines, chemokines, adhesion molecules, damage-associated molecular patterns, and other signaling molecules [5–7]. These events trigger an influx of innate immune cells to the graft, which is followed by the presentation of allogeneic antigens by antigen-presenting cells (APCs) to adaptive immune cells [8].
Acute organ rejection involves acute cellular rejection (ACR) orchestrated by T-cells and acute humoral rejection (AMR) driven by antibody-producing plasma cells [9].
Antibody-mediated rejection (AMR) is a profoundly studied phenomenon particularly in kidney transplants, leading to standardized nomenclature and diagnostic criteria. However, its applicability in lung transplants is limited, emphasizing the significance of T-cell-mediated rejection in lung allografts [10].
T-cell-mediated ACR in lung transplants, impacting small airways and vasculature, represents a significant clinical challenge [11–13]. The incidence of ACR is highest early post-lung transplantation, with 27% of adult patients experiencing at least one treated episode within the first year. ACR is associated with bronchiolitis obliterans syndrome (BOS), a main phenotype of chronic lung allograft dysfunction (CLAD), with late ACR episodes (after 180 days post-transplant) linked to an elevated risk of BOS [1, 14–18].
Diagnostic assessment of ACR faces interobserver variability, particularly in lower-grade rejection, and understanding of the specific traits and phenotypic patterns of infiltrating T-cells during ACR remains limited [11]. Therefore, ACR demands attention from researchers to pinpoint potential biomarkers that could help to understand immune responses and strengthen the diagnostic process and early detection of rejection.
Immune checkpoint molecules have been extensively studied in the oncological context [19]. However, their role and potential use in solid organ transplantation is far from being understood. Several studies have shown that the interaction between Programmed Death-Receptor 1 (PD-1) and Programmed Death-Ligand 1 (PD-L1) is essential for both initiating and sustaining tolerance to the graft [20].
PD-1 is a key inhibitory receptor involved in both adaptive and innate immune responses. It is expressed on various immune cells, including activated T cells, natural killer cells, B lymphocytes, macrophages, dendritic cells, and monocytes. PD-1 plays a crucial role in dampening autoimmune reactions and thus, preserving immune tolerance [21, 22]. As a PD-1 ligand, PD-L1 is typically found on macrophages, activated T and B cells, dendritic cells, and various epithelial cells, with its expression being elevated under inflammatory conditions. PD-L1 is often found in immune environments characterized by high loads of CD8+ T cells and the production of Th1 cytokines and interferons [21].
In contrast to other costimulatory molecules, PD-L1 expression extends beyond hematopoietic cells, as it can also be detected on endothelial cells, placental trophoblasts, and even pancreatic islet cells [23].
In the context of transplantation, the PD-1/PD-L1 pathway has been primarily investigated in animal models, with limited research was conducted in humans, particularly among lung transplant recipients [24, 25].
PD-L1 expression was shown to be significantly upregulated following transplantation on endothelial cells within heart allografts [26].
This increased expression within the vasculature indicates that PD-L1 may play a crucial role at the interface between immune cells and the transplanted organ, highlighting its potential importance in regulating the alloimmune response. In this regard, molecules involved in endothelial-immune cell interactions warrant particular attention.
Platelet/endothelial cell adhesion molecule-1 (PECAM-1 or CD31) is a key regulator of leukocyte transmigration across the endothelium and has been shown to be essential for transendothelial migration. PECAM-1-mediated leukocyte migration can be effectively inhibited by PECAM-1-specific blocking antibodies or by downregulating PECAM-1 expression [27].
Thus, examining PD-1/PD-L1 coinhibitory signals could provide valuable insights into the regulation of the alloimmune response, while clarifying the involvement of PECAM-1 in transplant rejection could highlight its potential as a novel therapeutic target in transplantation.
Immunohistochemistry (IHC), a cost-effective technique, has proven useful in diagnosing ACR in human kidney transplants [28–30]. Although in rat models, IHC aimed specifically at CD4⁺ and CD8⁺ T-cell proportions and distribution, improved the sensitivity and specificity of lung rejection diagnosis and grading, the same approach in human lung ACR is insufficient [31].
To address these gaps, and to better understand the role of immune checkpoint molecules in transplantation, our study explores multiple IHC biomarkers, including CD3, CD4, CD8, CD20, CD68, CD47, PD-1, PD-L1, and CD31/PECAM-1 within a large cohort. We aimed to identify T-cell subtype proportions and phenotypes, assess immune exhaustion levels, understand immune system dynamics, examine leukocyte transendothelial migration patterns, evaluate “don’t eat me” signals expression, and determine macrophage and B-cell proportions within lung allograft specimens.
Additionally, to obtain a detailed understanding of the immune landscape in LuTx recipients, we have extended our analyses by measuring T cell functionality via a multiplex assay. To provide a comprehensive profile of the immune status and functionality of T cells, which are critical in the context of transplantation, PD-L1, IL-2, Granzyme B, Tumor Necrosis Factor alpha (TNFα) and interferon gamma (IFNγ), were evaluated.
PD-L1 was included due to its role in immune regulation, whereas IL-2 provided insights into the activation status and responsiveness of T cells. Granzyme B, TNFα, and IFNγ are integral to the effector functions of T cells. Together, they provide comprehensive insights into T cells’ cytotoxic potential, inflammatory responses, and the regulatory balance of immune activation, all of which are crucial for graft survival and effective immune defense.
Understanding not just the phenotype but also the function of T cells is vital for developing strategies to enhance graft survival and reduce the risk of rejection.
This comprehensive analysis aimed to provide crucial insights into immune events within lung allografts.
Materials and Methods
Study Design
This retrospective study includes 171 adult patients (≥18 years) who underwent bilateral lung transplantation (LuTx) at Motol University Hospital in Prague between 1 January 2018, and 31 December 2021. Excluded were single, lobar, and multiorgan LuTx, as well as re-transplants. Patients without cryobiopsy within the initial 6 months post-transplant, lacking cryopreserved samples, or tissue samples for research were also excluded.
Routine and on-demand cryobiopsies were collectively analyzed, with routine samples taken after one, three, or six post-transplant months. Demographics and clinical data were obtained from patient files, and only laboratory results before ACR treatment initiation were considered. Serum samples taken prior to the initiation of potential rejection treatment were analyzed for selected cytokines using a customized Luminex Human Magnetic Assay. Tissue samples were evaluated using IHC. The study, approved by the Ethics Committee of Motol University Hospital (EK-530/21), received written informed consent from all patients at transplantation listing. Follow-up was censored on 24 September 2023.
Study Population
Donor and preservation variables included: age, gender, weight, height, BMI, donor type [donation after brain death (DBD) vs. donation after circulatory death], cytomegalovirus (CMV) status and times of ischemia for both lungs.
Recipient variables included: age, gender, weight, height, BMI, CMV status, underlying comorbidities, indication for transplant, immunosuppression regimen used, date of the first post-transplant lung tissue cryobiopsy, acute cellular rejection (grades A and B), infection status, peripheral blood levels of C-reactive protein (mg/L), peripheral blood levels of white blood cells (×109/L) and percentage and count (×109/L) of its subtypes, namely, lymphocytes, monocytes, neutrophils, eosinophils, basophils and immature granulocytes.
Immunohistochemistry
Sixty formalin-fixed paraffin-embedded tissue samples were retrospectively analyzed, evaluating the expression of CD3, CD8, CD20, CD4, CD68, CD47, PECAM-1 (CD31), PD1, and PD-L1. Histologic sections (3 µm thick) underwent staining with specific antibodies, including Anti-CD3, Anti-CD8, Anti-CD20, Anti-CD4, Anti-CD68, Anti-CD47, Anti-CD31, Anti-PD1, and Anti-PD-L1. Staining protocols involved various pre-treatments and dilutions (Table 1), with detection using a micropolymeric non-biotin system, except for PD-L1. Manual scoring by an experienced pathologist assessed the absolute count of immune cells positive for selected markers per 1 mm2, starting from “hot spots” within each sample. Our study focused on immune cells and evaluated cytoplasmic and membranous staining. Specifically, PECAM-1 showed membranous staining and PECAM-1-positive endothelial cells were excluded from scoring. Differentiation of PECAM-positive immune cells (specifically intraalveolar macrophages) from endothelial cells was enabled by distinct characteristics of the macrophages, such as their morphology, intra-alveolar location, and lower staining intensity, as shown in Figure 4. The nuclei were counterstained with hematoxylin.
Luminex Assay
Our sample preparation procedures were followed in accordance with the manufacturer’s guidelines to ensure accuracy and reproducibility. Specifically, we focused on blood sera derived from 51 patients and analyzed a panel of cytokines and markers, including IFNγ, Granzyme B, IL-2, PD-L1 and TNFα. A customized Luminex Human Magnetic Assay, sourced from Biotechne, R&D Systems s.r.o. in Prague, was used. The assay enabled precise detection of cytokines and chemokines in serum from lung transplant samples. Data were acquired using the Bio-Plex 200 system.
Cryobiopsies
Transbronchial cryobiopsy was the method of lung tissue sample collection, facilitated through flexible bronchoscopy targeting primarily the left lower lobe when possible. This procedure, conducted under total anesthesia, adhered to standard medical protocols. Cryobiopsies were evaluated according to ISHLT guidelines and scored for acute cellular rejection (ACR) (Grade A) and lymphocytic bronchiolitis (Grade B) [32]. Both tissue samples and peripheral blood were meticulously preserved at a stable temperature of −80°C until the analysis was performed.
Statistical Analyses
Continuous variables were standardly reported as median (interquartile range) and categorical variables as number (percentage). Data were grouped into two main groups – control group (only grade A0 = non-ACR) and rejection group (ACR grade A1-3). Fisher’s exact test was used to compare categorical variables between groups. Spearman correlations (ρ) and Mann–Whitney U tests were used to evaluate relations between clinical, IHC and Luminex variables and ACR. Kruskal-Wallis test was used to evaluate relations between all A0-A3 groups (Supplementary Table S1). Values falling below the lower limit of quantification were subjected to a halving procedure in the analytical process.
To evaluate the predictive capacity of IHC markers for graft acceptance or rejection (non-ACR vs. ACR), individual Receiver Operating Characteristic (ROC) curves were constructed for each marker, and the corresponding Area Under the Curve (AUC) was calculated. The Youden’s Index and Euclidian distance were computed to find the ideal cut-off values. This part of the analysis was performed by an experienced biostatistician (A.B.).
Results
Study Population and Baseline Characteristics
Immunohistochemical analyses were performed on a cohort of 60 tissue samples obtained from 60 bilateral lung transplant (LuTx) recipients. However, for subsequent Luminex analyses, samples from 9 patients were unavailable. Recipient age was 53 (42–60) years. Among recipients, 40 (67%) were male and 20 (33%) female. Indications for transplantation included chronic obstructive pulmonary disease (COPD) in 19 (32%) patients, interstitial lung disease (ILD) in 26 (43%), cystic fibrosis in 8 (13%) and pulmonary arterial hypertension (PAH) in 7 (12%) patients. Only DBD donors were reported in this cohort. Donor age was 44 (31–53) years. Two (3%) donors were older than 70 and one (2%) was younger than 18. Among donors, 32 (53%) were male, 28 (47%) female. Tables 2, 3 summarize the baseline characteristics of the study cohort. No differences in baseline characteristics were observed between control and rejection group. Supplementary Table S1 presents the distribution of acute cellular rejection grades in the study cohort. Standard induction immunosuppression at our center consists of basiliximab, tacrolimus, mycophenolate, and corticosteroids. For selected patients, an alternative strategy to basiliximab is employed. Supplementary Table S2 presents the percentage of patients in whom basiliximab and each alternative modality to it was used, either alone or in combination. For maintenance immunosuppression tacrolimus, mycophenolate, and corticosteroids are used. No significant difference was observed when comparing induction immunosuppressive regimens (p = 0.3341) and infection status (p = 0.7191) between the groups (Supplementary Tables S2, S3). A description of underlying immunological conditions is provided in Supplementary Table S4.
The Levels of C-Reactive Protein Were Associated With Acute Cellular Rejection
Our study cohort was initially stratified into two subgroups based on the presence or absence of ACR. These subgroups were subsequently compared in relation to differential white blood cell counts (WBC) and acute-phase proteins, specifically C-reactive protein (CRP). Figure 1 shows scatter plots with median and interquartile range of the measured values. Interestingly, no difference was observed in total WBC count, and percentages and counts of neutrophils, monocytes, lymphocytes, and eosinophils (Table 4), suggesting the limited efficacy of basic leukocyte parameters in predicting ACR within this context.
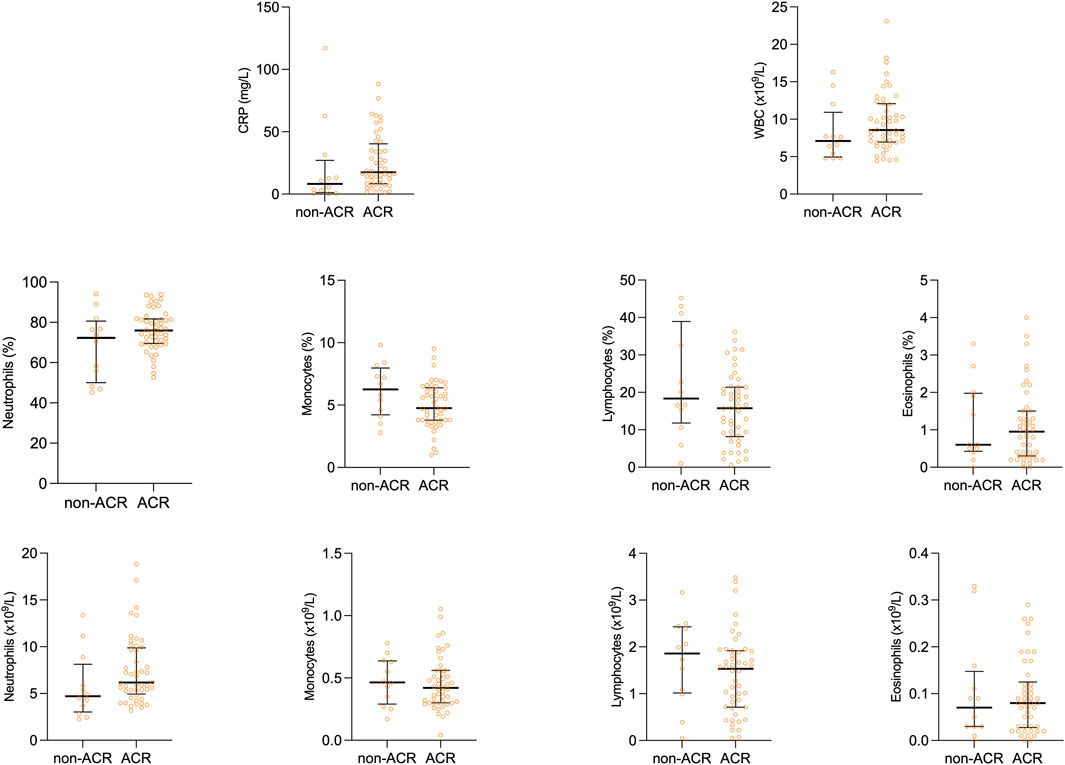
Figure 1. Scatter plots of peripheral blood cell profile comparing rejection group to control group. Median and interquartile range is visualized.
On the other hand, CRP, an acute-phase protein synthesized in the liver due to interleukin-6 secretion by macrophages and T-cells, displayed variations between the observed groups. As shown in Figure 1, peripheral blood levels of CRP tended to be lower in the non-ACR group [8.15 (2.4–17.8) as compared to the ACR group 17.5 (8.45–36.83) p = 0.055].
Neither T-Cell Subsets Nor B-Cells and Macrophages Exhibited Significant Elevation in Patients With Acute Cellular Rejection
In the course of our investigations, our primary objective revolved around elucidating the potential impact of ACR on the proportions of critical immune cell types (T-cells, B-cells, and macrophages) and demonstrating whether ACR elicits substantial changes in the abundance or distribution of these cell populations. Table 5 outlines the counts of positive immune cells per 1 mm2 for selected IHC markers. We hypothesized that examining the specific surface markers, such as CD3, CD4, CD8, CD20, and CD68, might offer a viable means of detecting the initial stages of ACR. Unfortunately, these markers did not show any differences between the ACR and non-ACR groups. As depicted in Figure 2, neither T-cell subsets nor B-cells and macrophages exhibited significant elevation in patients with ACR.
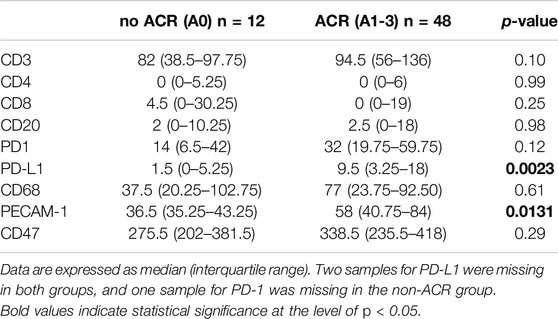
Table 5. Positive immune cell counts per 1 mm2 in lung tissue were determined for specific IHC markers in the study groups, excluding endothelial cells in PECAM-1 from the scoring system.
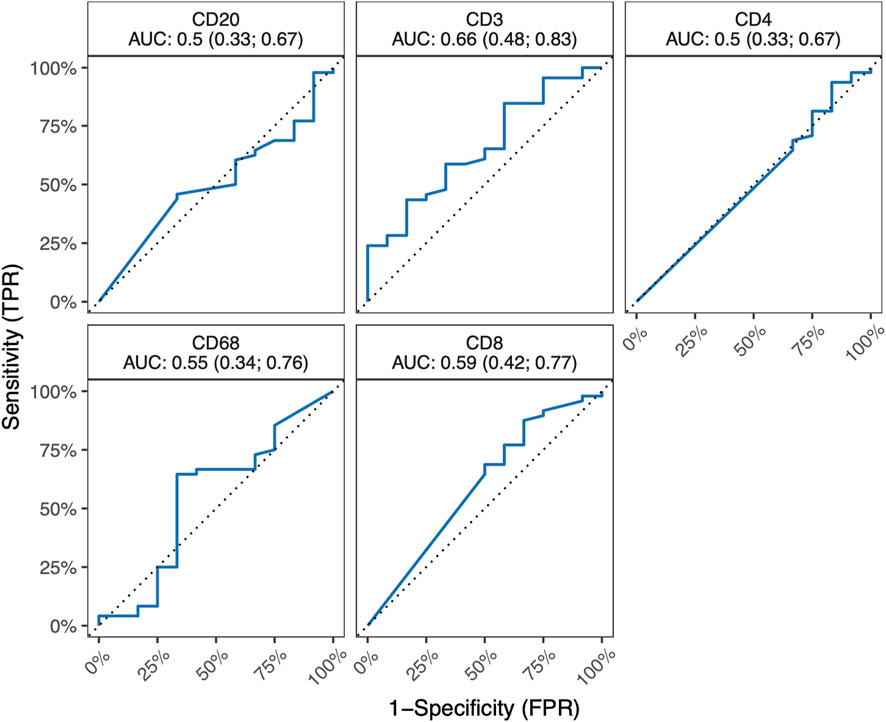
Figure 2. ROC curves for IHC markers CD3, CD4, CD8, CD20 a CD68, along with corresponding AUC values and their 95% confidence interval. All 95% confidence intervals include 0.5, which shows that none of the markers are good predictors.
PD-L1 Is Significantly Increased in Lung Transplant Recipients Exhibiting Acute Cellular Rejection
In the subsequent array of analyses, we examined immune checkpoints in lung tissue samples to understand the balance of activation/inhibition signals transmitted through immune receptors. Our primary focus was on the most prominent immune checkpoint pathway, predominantly occurring in T-cells, which involves the interaction between PD-1 and PD-L1 [33]. Following this, our attention shifted to exploring the novel potent macrophage checkpoint CD47, known as the “don’t eat me” signal [34]. While CD47 displayed no significant variations between the ACR and non-ACR group, striking differences were observed when analyzing the PD-L1 expression within lung allografts.
As shown in Figure 3A, PD-L1 exhibited significant increase in the rejection group (PD-L1 p = 0.0023), indicating an ongoing attempt to inhibit immune responses, particularly those involving T-cells. On the other hand, while the increase in PD-L1 levels might imply an effort to foster peripheral immune tolerance through its interaction with the PD-1 receptor, there was no observed increase in PD-1 receptor within the ACR cohort when compared to the non-ACR group. Figure 3B shows areas under ROC curves, and associated 95% confidence intervals, based on marked values for PD-L1 (0.80 confidence interval [0.65; 0.94]) and PD-1 (0.65 confidence interval [0.44; 0.86]). PD-L1 does not include 0.5 in its 95% confidence interval, therefore it can be a good ACR predictor. Supplementary Figure S1 shows Youden’s Index and Euclidian distance for PD-L1. Table 6, 7 show confusion matrices for PD-L1. PD-L1 remained significant (p = 0.0112) when analyzed across all ACR A grades collectively, as shown in Supplementary Table S5.
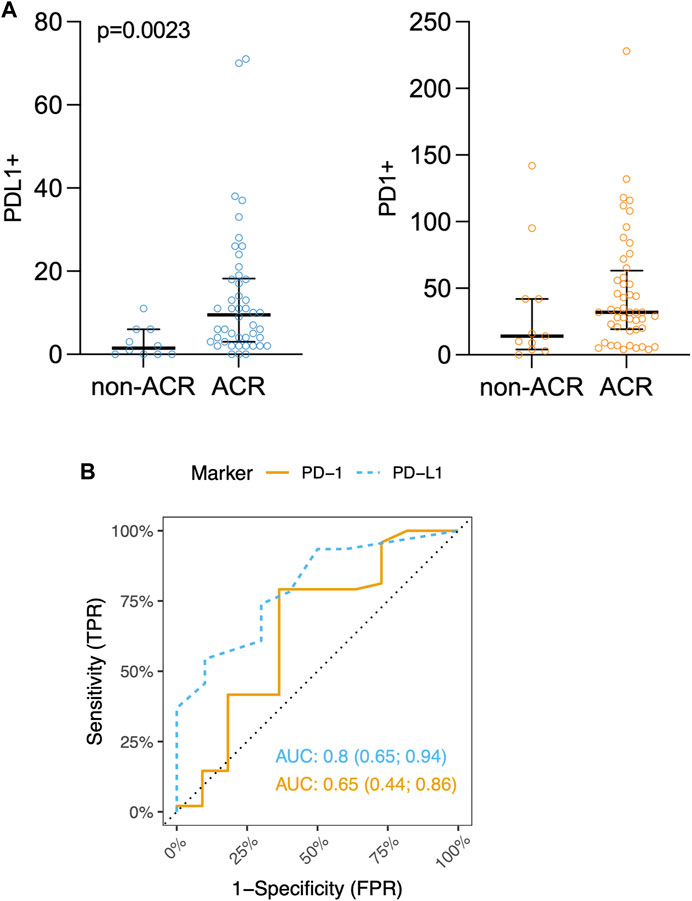
Figure 3. (A) Scatter plots of PD-L1+ and PD-1+ immune cells counts (positive immune cells per 1 mm2) in lung tissue biopsy. Median and interquartile range is visualized. (B) ROC curves for IHC markers PD-L1 and PD-1. Area under ROC-curves (AUC) (and associated 95% confidence interval) based on marked values for PD-L1 is 0.80 (0.65; 0.94) and for PD-1 is 0.65 (0.44; 0.86).
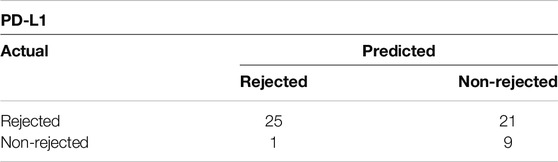
Table 6. Confusion matrix showing Youden’s Index for PD-L1 with cut-off value of 7 positive cells/mm2.
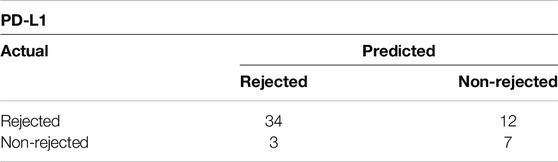
Table 7. Confusion matrix showing Euclidian distance for PD-L1 with cut-off value of 4 positive cells/mm2.
In Human Lung Allografts, Leukocytes Exhibit Dynamic Transendothelial Migration
PECAM-1, also known as CD31, plays a crucial role in facilitating the movement of leukocytes across the intercellular junctions of vascular endothelial cells during the process of leukocyte transmigration [35]. Given the increased scientifical interest in anti-PECAM-1 therapies blocking transendothelial migration of leukocytes, our aim was to investigate the potential involvement of PECAM-1 in ACR of lung allografts. Figures 4A–C show the PECAM-1 IHC staining of the samples.
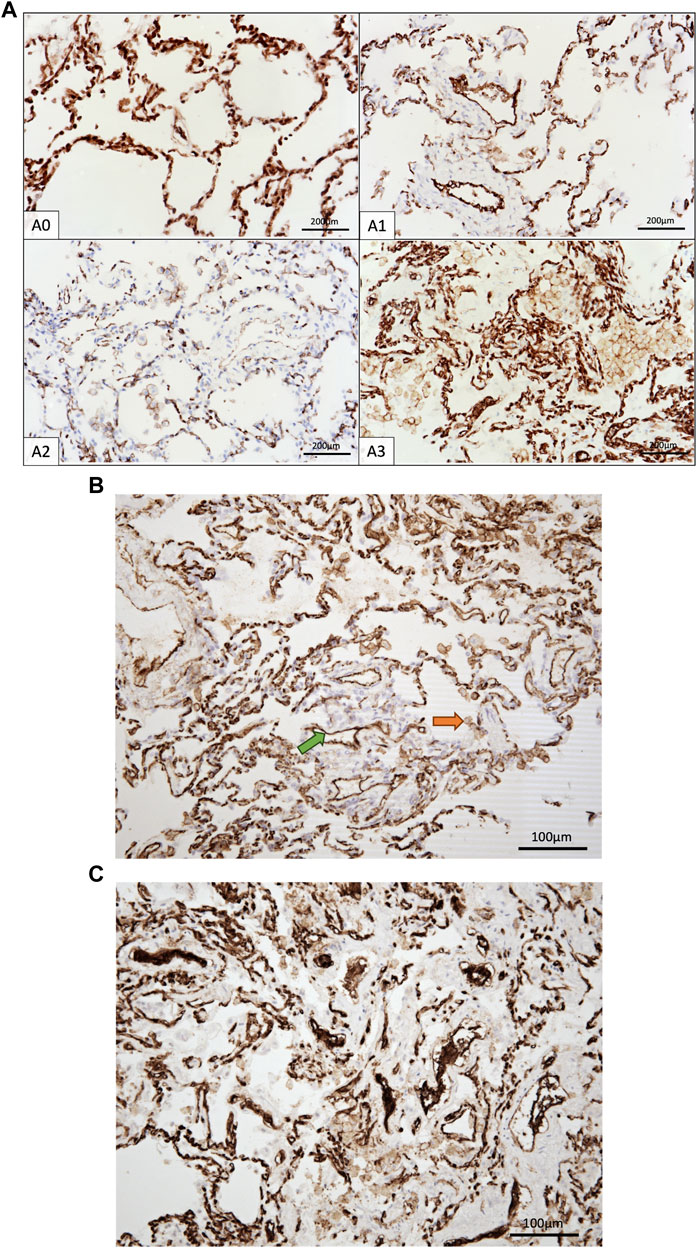
Figure 4. (A) IHC staining of CD31+ cells in control group and A1-A3 rejection groups. (B) CD31+ immune cells (orange arrow) and endothelia cells (green arrow). Only immune cells were counted. (C) Endothelium exhibiting CD31 positivity, presumably indicative of endothelial swelling associated with endothelitis, a characteristic frequently observed in A3.
Interestingly, PECAM-1 expression, assessed via IHC, demonstrated a trend towards significance (p = 0.0874) when analyzed across all ACR A grades collectively (Supplementary Table S5). PECAM-1 was significantly elevated in LuTx patients diagnosed with ACR (p = 0.0131) compared to those without ACR (Figure 5A). This finding suggests that PECAM-1 may have promising potential as a biomarker for ACR detection.Figure 5B shows area under ROC curve based on marked value for PECAM-1 (0.73).
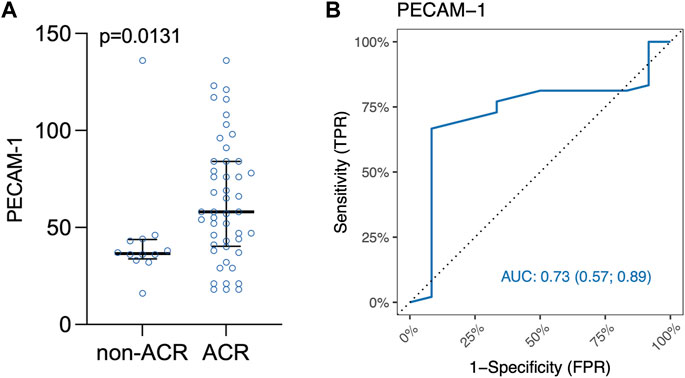
Figure 5. (A) Scatter plot of PECAM-1/CD31 immune cells in lung tissue biopsy. Median and interquartile range is visualized. (B) ROC curve for IHC marker PECAM-1. Area under ROC curve based on marked values for PECAM-1 is 0.73.
Both Youden’s Index and Euclidian distance cut-off point value based on the ranked values for PECAM-1 was 47 positive immune cells/mm2 (Supplementary Figure S2) indicating a threshold for distinguishing between samples that are positive or negative for PECAM-1 expression. Table 8 shows confusion matrix for PECAM-1.
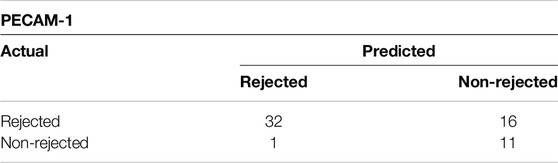
Table 8. Confusion matrix showing Youden’s Index and Euclidian distance for PECAM-1/CD31 with cut-off value of 47 positive cells/mm2.
T Cell Functional Capacities Were Unaffected in the Rejection Group
To gain a deeper understanding of T cell functionality in LuTx, we conducted a multiplex bead-based immunoassay using Luminex technology on serum samples to evaluate key molecules reflecting T cytotoxic and proliferative function. These molecules included PD-L1, IL-2, Granzyme B, TNFα, and IFNγ.
This multi-faceted approach allowed us to assess the cytotoxic capacities of T cells, and the inflammatory environment in the context of graft survival.
In the rejection group, our analysis revealed an increase in serum levels of IL-2, a cytokine that plays a critical role in T cell proliferation and immune regulation, alongside a decrease in PD-L1 levels. However, these changes did not reach statistical significance, with p-values of 0.8046 for IL-2 and 0.1224 for PD-L1, respectively. This suggests that while there may be a trend in these biomarkers, the observed variations are not strong enough to draw definitive conclusions about their roles in rejection processes.
Furthermore, no significant differences were detected in the levels of granzyme B, TNFα, and IFNγ, indicating that these immune markers may not be associated with rejection in this study population. Figure 6 shows areas under ROC curves, and associated 95% confidence intervals, based on marked values for analyzed molecules. All 95% confidence intervals include 0.5, which shows that none of the markers are good predictors.
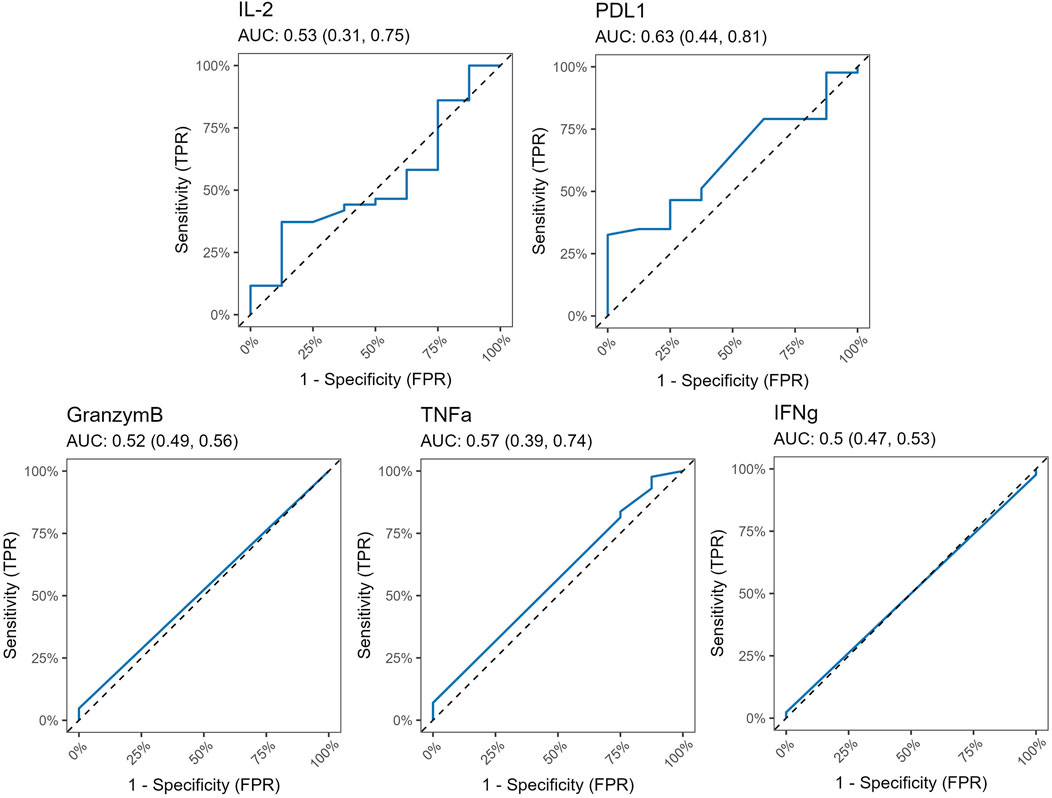
Figure 6. ROC curves for Luminex markers PD-L1, IL-2, Granzym B, TNFα and IFNγ, along with corresponding AUC values and their 95% confidence interval. All 95% confidence intervals include 0.5, which shows that none of the markers are good predictors.
Discussion
LuTx patients frequently face ACR complications, impacting lung function and contributing to CLAD. Our study analyzed ACR and non-ACR groups, focusing on variations in WBC counts and acute-phase proteins. Since these variables may be affected by immunosuppressive treatment, our study included only patients treated at our center, where the standard maintenance therapy consists of tacrolimus, mycophenolate, and corticosteroids. The induction immunosuppressive regimens varied among individual patients; however, there were no statistically significant differences between the ACR and non-ACR groups. Similar investigations by Vos et al. linked systemic inflammation, CRP levels, and graft failure, aligning with elevated CRP during acute heart rejection observed by Eisenberg et al [36–38]. In our study, despite no significant differences in total WBC count or cell percentages, CRP levels tended to be higher in the ACR group, indicating a potential association. Although the infection status of our patients did not statistically differ between ACR and non-ACR groups, changes in CRP levels should always be interpreted with caution, as not only accompanying infections, but also stress, inflammatory conditions, and other factors, may influence CRP [39, 40].
Next, we employed IHC to assess specific surface markers, aiming to uncover how ACR might influence the ratios of crucial immune cell (T-cells, B-cells, macrophages). Unfortunately, no significant differences were found between ACR and non-ACR groups.
Upon examining checkpoint molecules, it became apparent that CD47 did not seem a feasible marker for ACR. However, our focus shifted towards exploring the PD-1 and PD-L1 inhibitory pathway. To date, several studies have explored the functions of PD-1 and PD-L1 in transplantation. Wang et al. underscored their vital role in establishing cardiac allograft tolerance in mouse models [41]. Tanaka et al. highlighted PD-L1’s pivotal role in both inducing and maintaining peripheral tolerance following heart transplantation by modulating the equilibrium among T-cell subsets [42]. Additionally, Choudhary et al. observed an upregulation of PD-L1 within cardiomyocytes, demonstrating a correlation with the severity of ACR after transplantation [43].
Righi et al., focusing on 24 LuTx patients, revealed the importance of PD-1 in acute rejection and its progression into CLAD. They proposed evaluating PD-1-expressing lymphocytes in transbronchial biopsies for prognostic monitoring [44]. Subsequently, Kaiho et al. investigated PD-1/PD-L1 in acute rejection using a mouse tracheal transplantation model, finding a PD-L1-mediated immune checkpoint association with rejection, suggesting a potential immunotherapy target in LuTx [45].
Our study contributes to the understanding of the involvement of the PD-1/PD-L1 axis in LuTx by demonstrating a significant increase of tissue PD-L1 levels within the ACR group. While it remains uncertain which cells produce PD-L1 in lung allografts in vivo, this increase indicates an active effort to suppress immune responses, particularly those associated with T-cells. However, we did not detect a concurrent rise in the PD-1 receptor among the ACR cohort when compared to the non-ACR group.
We hypothesize that this phenomenon may result from PD-L1 production by various non-immune cells within the lung tissue, such as epithelial and endothelial cells, representing a localized immune suppression effort within the graft, primarily mediated by tissue-specific responses rather than T-cell-mediated modulation [46]. Furthermore, animal transplant models have shown that blocking PD-L1 leads to rejection, while blocking PD-1 and PD-L2 has no effect on graft survival. This indicates that PD-L1 and PD-L2 may play distinct roles in promoting tolerance, with PD-L1 expression, rather than PD-1 expression, emerging as the more reliable marker of immune regulation in transplantation [20].
Thus, the role of the PD-1/PD-L1 mechanism in acute rejection after lung transplantation has not yet been elucidated. These data, in accordance with previous studies, may imply the impairment of peripheral tolerance in LuTx recipients experiencing ACR.
In contemporary oncology, checkpoint molecules have emerged as pivotal targets in the therapeutic landscape, particularly within the realm of cancer treatment. This prominence arises from their capacity to modulate immune responses, a feature notably exploited to counteract the immunosuppressive microenvironment characteristics of malignancies [47]. Conversely, in the context of transplantation, the immune system often experiences heightened activation, resulting in the potential rejection of the transplanted organ. Hence, the contrasting immunological dynamics observed between cancer and transplantation underscore the likelihood of checkpoint molecules assuming a significant role in the latter scenario as well.
Khan et al. showed that the CTLA4, combined with the Fc portion of human immunoglobulin G1 (CTLA4-Ig) used as monotherapy immunosuppressant in mouse airway transplants promoted a favorable phase of immunotolerance, which facilitated microvascular and tissue repair [48].
The TIM family, notably TIM-1 and TIM-3, are pivotal regulators of the immune response and have been investigated in experimental transplant models. Murine studies reveal that inhibiting TIM-1 and boosting TIM-3 signaling enhances allograft outcomes [49]. The consistent findings across acute and chronic rejection models underscore the potential of TIM-3 interaction in mitigating detrimental immune responses [49]. The administration of stable galectin-9 in murine skin and cardiac transplants prolongs allograft survival by decreasing Th1 and Th17 cytokines and fostering Tregs [50–52]. To date, studies evaluating the role of LAG-3 in lung transplantation are lacking.
While there is speculation regarding the therapeutic utility of checkpoint molecules in modulating immune responses to prevent rejection, it is imperative to consider the potential adverse effects associated with such therapies. Of particular concern is the development of autoimmunity, a consequence that is undesirable across various clinical context. Moreover, according to Cui et al., immune checkpoint inhibitors were significantly associated with rejection in solid organ transplant recipients [53]. Therefore, any exploration of checkpoint inhibitor therapy in transplantation must carefully weigh the benefits of immune modulation against the risk of inducing autoimmune phenomena.
PECAM-1 plays a pivotal role in facilitating the migration of leukocytes across intercellular junctions within vascular endothelial cells during the transmigration process [35, 54]. The protective role of PECAM-1 in acute rejection has been demonstrated in various studies, yet its expression has not been previously analyzed by IHC in lung tissue during rejection episodes [55]. In 2022, Tran-Dinh et al. introduced an AI model evaluating CD31 cleavage for early ACR detection post LuTx [55]. We assessed the immunohistochemical surface expression of PECAM-1 in leukocytes from two distinct groups of LuTx recipients: individuals experiencing ACR and those without such complications. To our surprise, LuTx patients diagnosed with ACR showed a significant increase in PECAM-1 expression (IHC), prompting us to hypothesize that inhibiting transendothelial migration might represent a therapeutic approach for ACR.
In oncology, endothelial-immune cell interactions within the tumor microenvironment influence immune infiltration and function, highlighting the critical role of endothelial cells in immune response [56]. There is no reason to believe this would be any different in transplantation. Notably, endothelial cells in the donor lung are among the first to encounter the recipient’s immune system.
PECAM-1 is involved in a wide array of processes related to inflammation, vascular biology, and various immune functions [57]. It has several splice variants, each capable of exhibiting distinct adhesive properties, which may subsequently impact its ligand-binding characteristics and functional role in leukocyte transmigration [58, 59]. The functional role of PECAM-1 is influenced by multiple factors, including the nature and tissue localization of the inflammatory response, as well as genetic determinants [57].
PECAM-1 possess both pro- and anti-inflammatory roles. Besides facilitating transendothelial leukocyte migration, it also plays a role in dampening leukocyte activation and reducing pro-inflammatory cytokine production [60]. In the context of ACR, macrophages expressing PECAM-1 may polarize into M2 subset which exhibit anti-inflammatory and graft-protective effects [61]. Thus, high PECAM-1 expression in the graft may reflect immune cell infiltration as well as active repair processes and endothelial resilience.
This study shows the potential of IHC in ACR diagnosis. Despite the additional cost, time, and effort required to perform IHC, its application could be advantageous in borderline cases as a supplementary technique to traditional histopathology. To improve assessments, the ISHLT recommends obtaining at least five adequate samples, reducing variability [32]. This is particularly crucial in cases of ACR, especially when confronting lower-grade rejection. This variability not only presents challenges in individual patient management but also hinders efforts to achieve standardization in multicenter trials [11, 62]. Identifying appropriate IHC markers could help tackle these issues and our data suggests that both PD-L1 and PECAM-1 need further exploration in ACR.
In order to better understand T cell functionality in LuTx, we also employed a multiplex bead-based immunoassay using Luminex technology to assess key molecules that reflect T cell cytotoxicity and proliferation. The molecules analyzed were PD-L1, IL-2, Granzyme B, TNFα, and IFNγ. This approach aimed to provide insights into the cytotoxic potential of T cells and the surrounding inflammatory environment, which are crucial for improving graft survival, formulating targeted therapies, and enhancing outcomes for transplant patients.
The role of IL-2 in acute lung rejection has been previously reported [63–65]. Luminex analysis in our study cohort did not yield significant results. However, elevated levels of IL-2 in patients with ACR is in line with the older work of Jordan et al. [66] Our Luminex analyses were constrained by the small size of the study cohort. To this we also attribute the inconclusive results of other biomarkers investigated by the Luminex method. Future studies with larger sample sizes and the inclusion of more relevant biomarkers, such as PECAM-1, could provide more insightful findings.
In our center, cryobiopsies are the standard of care. The Zurich group has demonstrated that cryobiopsies offer a superior diagnostic yield for ACR compared to forceps biopsies, leading to reclassification and treatment strategy changes in 28.6% of cases [67]. Our findings show that nearly half of the samples in this cohort exhibit an A1 rejection grade. Notably, identifying A1 rejection in a clinically stable patient through biopsy may not necessitate therapeutic intervention.
This study adopts a retrospective design, encompassing solely double lung transplant patients with samples available in archives in a single high-volume transplant center. Observational design, limited cohort size and group size imbalances are notable. However, the cohorts, aside from size differences, exhibit consistent characteristics. Our selection might indeed introduce bias and therefore, a prospective study would be imperative to also ascertain an accurate representation of the prevalence within our patient cohort. Despite widespread use of IHC, interpretational variability also remains a challenge.
Conducting larger studies is essential for evaluating immunohistochemistry (IHC), with a specific focus on PD-L1/PECAM-1 markers, in the diagnosis of ACR.
Conclusion
IHC investigations of PECAM-1 and PD-L1 markers might be valuable for diagnosing ACR. Further research is required to enhance our understanding of the role of immune checkpoint inhibitors in lung transplantation.
Data Availability Statement
The raw data supporting the conclusions of this article will be made available by the authors, without undue reservation.
Ethics Statement
The studies involving humans were approved by the Ethics Committee of Motol University Hospital (EK-530/21). The studies were conducted in accordance with the local legislation and institutional requirements. The participants provided their written informed consent to participate in this study.
Author Contributions
Conceptualization: RN and ZOS; Methodology: RN, ZOS, JB, JT, VT, JS, AB, and MB; Formal analysis and investigation: RN, JB, JT, and ZOS; Data acquisition: RN, JB, VT, AS, KV, JS, and ZOS; Statistical analysis: AB, MB, and RN; Writing - original draft preparation: RN and ZOS; Writing - review and editing: RN, JB, JT, VT, AS, KV, JS, AB, JaV, MB, BV, RL, JiV, LC, and ZOS; Funding acquisition: RL, ZOS, and LC; Resources: RL, ZOS, and LC; Supervision: ZOS and LC. All authors contributed to the article and approved the submitted version.
Funding
The author(s) declare that financial support was received for the research, authorship, and/or publication of this article. This study was supported by the Ministry of Health, Czech Republic – Conceptual Development of Research Organization, University Hospital Motol, Prague, Czech Republic (No. 6028), and by the Cooperatio Program, Research Area SURG.
Conflict of Interest
LC declares being a senior Clinical Research Fellow of the Research Foundation-Flanders (FWO) (#18E2B24N) and supported by a KU Leuven University Chair funded by Medtronic, unrelated to the study.
The remaining authors declare that the research was conducted in the absence of any commercial or financial relationships that could be construed as a potential conflict of interest.
Acknowledgments
The authors would like to thank all surgeons, transplant coordinators, anesthesiologists, pulmonologists, intensive care physicians, nursing staff, physiotherapists, and lung transplant scientists involved in the Prague Lung Transplant Program for their contribution. During the preparation of this work the authors used ChatGPT (OpenAI) in order to improve readability and language. After using this tool, the authors reviewed and edited the content as needed and take full responsibility for the content of the publication.
Supplementary Material
The Supplementary Material for this article can be found online at: https://www.frontierspartnerships.org/articles/10.3389/ti.2024.13796/full#supplementary-material
Abbreviations
ACR, acute cellular rejection; AMR, acute humoral rejection; APCs, antigen presenting cells; BOS, bronchiolitis obliterans syndrome; CLAD, chronic lung allograft dysfunction; CMV, cytomegalovirus; COPD, chronic obstructive pulmonary disease; CRP, C-reactive protein; DBD, donation after brain death; IHC, immunohistochemistry; IFNγ, interferon gamma; ILD, interstitial lung disease; ISHLT, the International Society for Heart and Lung Transplantation; PAH, pulmonary arterial hypertension; PD-1, programmed death-receptor 1; PD-L1, programmed death-ligand 1; LuTx, lung transplantation; RAS, restrictive allograft syndrome; ROC, receiver operating characteristics; TNFα, tumor necrosis factor alpha; WBC, white blood cells.
References
1. Burton, CM, Iversen, M, Carlsen, J, Mortensen, J, Andersen, CB, Steinbruchel, D, et al. Acute Cellular Rejection Is a Risk Factor for Bronchiolitis Obliterans Syndrome Independent of Post-Transplant Baseline FEV1. J Heart Lung Transpl (2009) 28(9):888–93. doi:10.1016/j.healun.2009.04.022
2. Clayton, PA, McDonald, SP, Russ, GR, and Chadban, SJ. Long-Term Outcomes After Acute Rejection in Kidney Transplant Recipients: An ANZDATA Analysis. J Am Soc Nephrol (2019) 30(9):1697–707. doi:10.1681/ASN.2018111101
3. Reddy, KS, Davies, D, Ormond, D, Tuteja, S, Lucas, BA, Johnston, TD, et al. Impact of Acute Rejection Episodes on Long-Term Graft Survival Following Simultaneous Kidney-Pancreas Transplantation. Am J Transpl (2003) 3(4):439–44. doi:10.1034/j.1600-6143.2003.00059.x
4. Levy, L, Huszti, E, Tikkanen, J, Ghany, R, Klement, W, Ahmed, M, et al. The Impact of First Untreated Subclinical Minimal Acute Rejection on Risk for Chronic Lung Allograft Dysfunction or Death After Lung Transplantation. Am J Transpl (2020) 20(1):241–9. doi:10.1111/ajt.15561
5. Wu, MY, Yiang, GT, Liao, WT, Tsai, AP, Cheng, YL, Cheng, PW, et al. Current Mechanistic Concepts in Ischemia and Reperfusion Injury. Cell Physiol Biochem (2018) 46(4):1650–67. doi:10.1159/000489241
6. Gracia-Sancho, J, Villarreal, G, Zhang, Y, Yu, JX, Liu, Y, Tullius, SG, et al. Flow Cessation Triggers Endothelial Dysfunction During Organ Cold Storage Conditions: Strategies for Pharmacologic Intervention. Transplantation (2010) 90(2):142–9. doi:10.1097/TP.0b013e3181e228db
7. Chen-Yoshikawa, TF. Ischemia-Reperfusion Injury in Lung Transplantation. Cells (2021) 10(6):1333. doi:10.3390/cells10061333
8. Aiello, S, Podesta, MA, Rodriguez-Ordonez, PY, Pezzuto, F, Azzollini, N, Solini, S, et al. Transplantation-Induced Ischemia-Reperfusion Injury Modulates Antigen Presentation by Donor Renal CD11c(+)F4/80(+) Macrophages Through IL-1R8 Regulation. J Am Soc Nephrol (2020) 31(3):517–31. doi:10.1681/ASN.2019080778
9. Martinu, T, Pavlisko, EN, Chen, DF, and Palmer, SM. Acute Allograft Rejection: Cellular and Humoral Processes. Clin Chest Med (2011) 32(2):295–310. doi:10.1016/j.ccm.2011.02.008
10. Loupy, A, and Lefaucheur, C. Antibody-Mediated Rejection of Solid-Organ Allografts. N Engl J Med (2018) 379(12):1150–60. doi:10.1056/NEJMra1802677
11. Levine, DJ, and Hachem, RR. Lung Allograft Rejection. Thorac Surg Clin (2022) 32(2):221–9. doi:10.1016/j.thorsurg.2021.12.003
12. Koutsokera, A, Levy, L, Pal, P, Orchanian-Cheff, A, and Martinu, T. Acute Cellular Rejection: Is It Still Relevant? Semin Respir Crit Care Med (2018) 39(2):181–98. doi:10.1055/s-0037-1617424
13. Martinu, T, Howell, DN, and Palmer, SM. Acute Cellular Rejection and Humoral Sensitization in Lung Transplant Recipients. Semin Respir Crit Care Med (2010) 31(2):179–88. doi:10.1055/s-0030-1249113
14. Martinu, T, Chen, DF, and Palmer, SM. Acute Rejection and Humoral Sensitization in Lung Transplant Recipients. Proc Am Thorac Soc (2009) 6(1):54–65. doi:10.1513/pats.200808-080GO
15. Chambers, DC, Yusen, RD, Cherikh, WS, Goldfarb, SB, Kucheryavaya, AY, Khusch, K, et al. The Registry of the International Society for Heart and Lung Transplantation: Thirty-Fourth Adult Lung and Heart-Lung Transplantation Report-2017; Focus Theme: Allograft Ischemic Time. J Heart Lung Transpl (2017) 36(10):1047–59. doi:10.1016/j.healun.2017.07.016
16. Verleden, SE, Ruttens, D, Vandermeulen, E, Vaneylen, A, Dupont, LJ, Van Raemdonck, DE, et al. Bronchiolitis Obliterans Syndrome and Restrictive Allograft Syndrome: Do Risk Factors Differ? Transplantation (2013) 95(9):1167–72. doi:10.1097/TP.0b013e318286e076
17. Glanville, AR, Aboyoun, CL, Havryk, A, Plit, M, Rainer, S, and Malouf, MA. Severity of Lymphocytic Bronchiolitis Predicts Long-Term Outcome After Lung Transplantation. Am J Respir Crit Care Med (2008) 177(9):1033–40. doi:10.1164/rccm.200706-951OC
18. Girgis, RE, Tu, I, Berry, GJ, Reichenspurner, H, Valentine, VG, Conte, JV, et al. Risk Factors for the Development of Obliterative Bronchiolitis After Lung Transplantation. J Heart Lung Transpl (1996) 15(12):1200–8.
19. He, X, and Xu, C. Immune Checkpoint Signaling and Cancer Immunotherapy. Cell Res (2020) 30(8):660–9. doi:10.1038/s41422-020-0343-4
20. Riella, LV, Paterson, AM, Sharpe, AH, and Chandraker, A. Role of the PD-1 Pathway in the Immune Response. Am J Transpl (2012) 12(10):2575–87. doi:10.1111/j.1600-6143.2012.04224.x
21. Han, Y, Liu, D, and Li, L. PD-1/PD-L1 Pathway: Current Researches in Cancer. Am J Cancer Res (2020) 10(3):727–42.
22. Keir, ME, Butte, MJ, Freeman, GJ, and Sharpe, AH. PD-1 and Its Ligands in Tolerance and Immunity. Annu Rev Immunol (2008) 26:677–704. doi:10.1146/annurev.immunol.26.021607.090331
23. Francisco, LM, Sage, PT, and Sharpe, AH. The PD-1 Pathway in Tolerance and Autoimmunity. Immunol Rev (2010) 236:219–42. doi:10.1111/j.1600-065X.2010.00923.x
24. Ozkaynak, E, Wang, L, Goodearl, A, McDonald, K, Qin, S, O’Keefe, T, et al. Programmed Death-1 Targeting Can Promote Allograft Survival. J Immunol (2002) 169(11):6546–53. doi:10.4049/jimmunol.169.11.6546
25. Morita, M, Fujino, M, Jiang, G, Kitazawa, Y, Xie, L, Azuma, M, et al. PD-1/B7-H1 Interaction Contribute to the Spontaneous Acceptance of Mouse Liver Allograft. Am J Transpl (2010) 10(1):40–6. doi:10.1111/j.1600-6143.2009.02859.x
26. Riella, LV, Watanabe, T, Sage, PT, Yang, J, Yeung, M, Azzi, J, et al. Essential Role of PDL1 Expression on Nonhematopoietic Donor Cells in Acquired Tolerance to Vascularized Cardiac Allografts. Am J Transpl (2011) 11(4):832–40. doi:10.1111/j.1600-6143.2011.03451.x
27. Strizova, Z, Bartunkova, J, and Smrz, D. The Challenges of Adoptive Cell Transfer in the Treatment of Human Renal Cell Carcinoma. Cancer Immunol Immunother (2019) 68(11):1831–8. doi:10.1007/s00262-019-02359-z
28. Park, J, Byun, SW, Yu, E, Park, SK, Han, DJ, and Cho, YM. Immunohistochemical Profile of Acute Cellular Rejection in Renal Allograft. J Pathol Translational Med (2007) 41(1):15–20.
29. Coons, AH, Creech, HJ, and Jones, RN. Immunological Properties of an Antibody Containing a Fluorescent Group. Exp Biol Med (1941) 47(2):200–2. doi:10.3181/00379727-47-13084p
30. Kohler, G, and Milstein, C. Continuous Cultures of Fused Cells Secreting Antibody of Predefined Specificity. Nature (1975) 256(5517):495–7. doi:10.1038/256495a0
31. Cheng, L, Guo, H, Qiao, X, Liu, Q, Nie, J, Li, J, et al. T Cell Immunohistochemistry Refines Lung Transplant Acute Rejection Diagnosis and Grading. Diagn Pathol (2013) 8:168. doi:10.1186/1746-1596-8-168
32. Stewart, S, Fishbein, MC, Snell, GI, Berry, GJ, Boehler, A, Burke, MM, et al. Revision of the 1996 Working Formulation for the Standardization of Nomenclature in the Diagnosis of Lung Rejection. J Heart Lung Transpl (2007) 26(12):1229–42. doi:10.1016/j.healun.2007.10.017
33. Zhang, P, Wang, Y, Miao, Q, and Chen, Y. The Therapeutic Potential of PD-1/pd-L1 Pathway on Immune-Related Diseases: Based on the Innate and Adaptive Immune Components. Biomed Pharmacother (2023) 167:115569. doi:10.1016/j.biopha.2023.115569
34. Wang, C, Feng, Y, Patel, D, Xie, H, Lv, Y, and Zhao, H. The Role of CD47 in Non-Neoplastic Diseases. Heliyon (2023) 9(12):e22905. doi:10.1016/j.heliyon.2023.e22905
35. Muller, WA, Weigl, SA, Deng, X, and Phillips, DM. PECAM-1 Is Required for Transendothelial Migration of Leukocytes. J Exp Med (1993) 178(2):449–60. doi:10.1084/jem.178.2.449
36. Vos, R, Vanaudenaerde, BM, De Vleeschauwer, SI, Van Raemdonck, DE, Dupont, LJ, and Verleden, GM. Plasma C-Reactive Protein Levels Correlate With Markers of Airway Inflammation After Lung Transplantation: A Role for Systemic Inflammation in Bronchiolitis Obliterans Syndrome? Transpl Proc (2009) 41(2):595–8. doi:10.1016/j.transproceed.2008.12.024
37. Vos, R, Vanaudenaerde, BM, De Vleeschauwer, SI, Willems-Widyastuti, A, Scheers, H, Van Raemdonck, DE, et al. Circulating and Intrapulmonary C-Reactive Protein: A Predictor of Bronchiolitis Obliterans Syndrome and Pulmonary Allograft Outcome. J Heart Lung Transpl (2009) 28(8):799–807. doi:10.1016/j.healun.2009.05.011
38. van Gelder, T, Balk, AH, Zondervan, PE, Maat, AW, Mochtar, B, van der Meer, P, et al. C-Reactive Protein in the Monitoring of Acute Rejection After Heart Transplantation. Transpl Int (1998) 11(5):361–4. doi:10.1007/s001470050158
39. Gouin, JP, Glaser, R, Malarkey, WB, Beversdorf, D, and Kiecolt-Glaser, J. Chronic Stress, Daily Stressors, and Circulating Inflammatory Markers. Health Psychol (2012) 31(2):264–8. doi:10.1037/a0025536
40. Landry, A, Docherty, P, Ouellette, S, and Cartier, LJ. Causes and Outcomes of Markedly Elevated C-Reactive Protein Levels. Can Fam Physician (2017) 63(6):e316–23.
41. Wang, L, Han, R, and Hancock, WW. Programmed Cell Death 1 (PD-1) and Its Ligand PD-L1 Are Required for Allograft Tolerance. Eur J Immunol (2007) 37(10):2983–90. doi:10.1002/eji.200737583
42. Tanaka, K, Albin, MJ, Yuan, X, Yamaura, K, Habicht, A, Murayama, T, et al. PDL1 Is Required for Peripheral Transplantation Tolerance and Protection From Chronic Allograft Rejection. J Immunol (2007) 179(8):5204–10. doi:10.4049/jimmunol.179.8.5204
43. Choudhary, A, Brinkley, DM, Besharati, S, Meijers, WC, Atkinson, JB, Amancherla, K, et al. PD-L1 (Programmed Death Ligand 1) as a Marker of Acute Cellular Rejection After Heart Transplantation. Circ Heart Fail (2021) 14(10):e008563. doi:10.1161/CIRCHEARTFAILURE.121.008563
44. Righi, I, Vaira, V, Morlacchi, LC, Croci, GA, Rossetti, V, Blasi, F, et al. PD-1 Expression in Transbronchial Biopsies of Lung Transplant Recipients Is a Possible Early Predictor of Rejection. Front Immunol (2022) 13:1024021. doi:10.3389/fimmu.2022.1024021
45. Kaiho, T, Suzuki, H, Hata, A, Matsumoto, H, Tanaka, K, Sakairi, Y, et al. Targeting PD-1/PD-L1 Inhibits Rejection in a Heterotopic Tracheal Allograft Model of Lung Transplantation. Front Pharmacol (2023) 14:1298085. doi:10.3389/fphar.2023.1298085
46. Hudson, K, Cross, N, Jordan-Mahy, N, and Leyland, R. The Extrinsic and Intrinsic Roles of PD-L1 and Its Receptor PD-1: Implications for Immunotherapy Treatment. Front Immunol (2020) 11:568931. doi:10.3389/fimmu.2020.568931
47. Xiao, Y, Li, ZZ, Zhong, NN, Cao, LM, Liu, B, and Bu, LL. Charting New Frontiers: Co-Inhibitory Immune Checkpoint Proteins in Therapeutics, Biomarkers, and Drug Delivery Systems in Cancer Care. Transl Oncol (2023) 38:101794. doi:10.1016/j.tranon.2023.101794
48. Khan, MA, Shamma, T, Altuhami, A, Ahmed, HA, Assiri, AM, and Broering, DC. CTLA4-Ig Mediated Immunosuppression Favors Immunotolerance and Restores Graft in Mouse Airway Transplants. Pharmacol Res (2022) 178:106147. doi:10.1016/j.phrs.2022.106147
49. Yeung, MY, McGrath, M, and Najafian, N. The Emerging Role of the TIM Molecules in Transplantation. Am J Transpl (2011) 11(10):2012–9. doi:10.1111/j.1600-6143.2011.03727.x
50. He, W, Fang, Z, Wang, F, Wu, K, Xu, Y, Zhou, H, et al. Galectin-9 Significantly Prolongs the Survival of Fully Mismatched Cardiac Allografts in Mice. Transplantation (2009) 88(6):782–90. doi:10.1097/TP.0b013e3181b47f25
51. Wang, F, He, W, Yuan, J, Wu, K, Zhou, H, Zhang, W, et al. Activation of Tim-3-Galectin-9 Pathway Improves Survival of Fully Allogeneic Skin Grafts. Transpl Immunol (2008) 19(1):12–9. doi:10.1016/j.trim.2008.01.008
52. Seki, M, Oomizu, S, Sakata, KM, Sakata, A, Arikawa, T, Watanabe, K, et al. Galectin-9 Suppresses the Generation of Th17, Promotes the Induction of Regulatory T Cells, and Regulates Experimental Autoimmune Arthritis. Clin Immunol (2008) 127(1):78–88. doi:10.1016/j.clim.2008.01.006
53. Cui, X, Yan, C, Xu, Y, Li, D, Guo, M, Sun, L, et al. Allograft Rejection Following Immune Checkpoint Inhibitors in Solid Organ Transplant Recipients: A Safety Analysis From a Literature Review and a Pharmacovigilance System. Cancer Med (2023) 12(5):5181–94. doi:10.1002/cam4.5394
54. Berman, ME, Xie, Y, and Muller, WA. Roles of Platelet/endothelial Cell Adhesion Molecule-1 (PECAM-1, CD31) in Natural Killer Cell Transendothelial Migration and Beta 2 Integrin Activation. J Immunol (1996) 156(4):1515–24. doi:10.4049/jimmunol.156.4.1515
55. Tran-Dinh, A, Laurent, Q, Even, G, Tanaka, S, Lortat-Jacob, B, Castier, Y, et al. Personalized Risk Predictor for Acute Cellular Rejection in Lung Transplant Using Soluble CD31. Sci Rep (2022) 12(1):17628. doi:10.1038/s41598-022-21070-1
56. Fang, J, Lu, Y, Zheng, J, Jiang, X, Shen, H, Shang, X, et al. Exploring the Crosstalk Between Endothelial Cells, Immune Cells, and Immune Checkpoints in the Tumor Microenvironment: New Insights and Therapeutic Implications. Cell Death Dis (2023) 14(9):586. doi:10.1038/s41419-023-06119-x
57. Woodfin, A, Voisin, MB, and Nourshargh, S. PECAM-1: A Multi-Functional Molecule in Inflammation and Vascular Biology. Arterioscler Thromb Vasc Biol (2007) 27(12):2514–23. doi:10.1161/ATVBAHA.107.151456
58. Yan, HC, Baldwin, HS, Sun, J, Buck, CA, Albelda, SM, and DeLisser, HM. Alternative Splicing of a Specific Cytoplasmic Exon Alters the Binding Characteristics of Murine Platelet/Endothelial Cell Adhesion Molecule-1 (PECAM-1). J Biol Chem (1995) 270(40):23672–80. doi:10.1074/jbc.270.40.23672
59. Baldwin, HS, Shen, HM, Yan, HC, DeLisser, HM, Chung, A, Mickanin, C, et al. Platelet Endothelial Cell Adhesion Molecule-1 (PECAM-1/CD31): Alternatively Spliced, Functionally Distinct Isoforms Expressed During Mammalian Cardiovascular Development. Development (1994) 120(9):2539–53. doi:10.1242/dev.120.9.2539
60. Privratsky, JR, Newman, DK, and Newman, PJ. PECAM-1: Conflicts of Interest in Inflammation. Life Sci (2010) 87(3-4):69–82. doi:10.1016/j.lfs.2010.06.001
61. Strizova, Z, Benesova, I, Bartolini, R, Novysedlak, R, Cecrdlova, E, Foley, LK, et al. M1/M2 Macrophages and Their Overlaps - Myth or Reality? Clin Sci (Lond) (2023) 137(15):1067–93. doi:10.1042/CS20220531
62. Arcasoy, SM, Berry, G, Marboe, CC, Tazelaar, HD, Zamora, MR, Wolters, HJ, et al. Pathologic Interpretation of Transbronchial Biopsy for Acute Rejection of Lung Allograft Is Highly Variable. Am J Transpl (2011) 11(2):320–8. doi:10.1111/j.1600-6143.2010.03382.x
63. Yamada, Y, Nguyen, TT, Impellizzieri, D, Mineura, K, Shibuya, R, Gomariz, A, et al. Biased IL-2 Signals Induce Foxp3-Rich Pulmonary Lymphoid Structures and Facilitate Long-Term Lung Allograft Acceptance in Mice. Nat Commun (2023) 14(1):1383. doi:10.1038/s41467-023-36924-z
64. Yamada, Y, Impellizzieri, D, Jang, J, Nguyen, T, Karakus, U, Inci, I, et al. Regulatory T Cells Induce Persistent Acceptance by IL-2 Complexes After Mouse Lung Transplantation. J Heart Lung Transplant (2018) 37(4):S124–5. doi:10.1016/j.healun.2018.01.297
65. Sweet, SC, De La Morena, MT, Shapiro, SD, Mendeloff, EN, and Huddleston, CB. Interleukin-2-Receptor Blockade With Daclizumab Decreases the Incidence of Acute Rejection in Pediatric Lung Transplant Recipients. J Heart Lung Transplant (2001) 20(2):221–2. doi:10.1016/s1053-2498(00)00486-1
66. Jordan, SC, Marchevski, A, Ross, D, Toyoda, M, and Waters, PF. Serum Interleukin-2 Levels in Lung Transplant Recipients: Correlation With Findings on Transbronchial Biopsy. J Heart Lung Transpl (1992) 11(5):1001–4.
Keywords: lung transplantation, acute cellular rejection, immunohistochemistry, luminex, checkpoint inhibitors
Citation: Novysedlak R, Balko J, Tavandzis J, Tovazhnianska V, Slavcev A, Vychytilova K, Smetanova J, Bohyn A, Vajter J, Borcinova M, Vanaudenaerde BM, Lischke R, Vachtenheim J Jr., Ceulemans LJ and Ozaniak Strizova Z (2024) Elevated PD-L1 and PECAM-1 as Diagnostic Biomarkers of Acute Rejection in Lung Transplantation. Transpl Int 37:13796. doi: 10.3389/ti.2024.13796
Received: 12 September 2024; Accepted: 08 November 2024;
Published: 21 November 2024.
Copyright © 2024 Novysedlak, Balko, Tavandzis, Tovazhnianska, Slavcev, Vychytilova, Smetanova, Bohyn, Vajter, Borcinova, Vanaudenaerde, Lischke, Vachtenheim, Ceulemans and Ozaniak Strizova. This is an open-access article distributed under the terms of the Creative Commons Attribution License (CC BY). The use, distribution or reproduction in other forums is permitted, provided the original author(s) and the copyright owner(s) are credited and that the original publication in this journal is cited, in accordance with accepted academic practice. No use, distribution or reproduction is permitted which does not comply with these terms.
*Correspondence: Zuzana Ozaniak Strizova, enV6YW5hLnN0cml6b3ZhQGZubW90b2wuY3o=
†ORCID: Rene Novysedlak, orcid.org/0000-0002-2660-6815; Jan Balko, orcid.org/0000-0003-0451-2786; Janis Tavandzis, orcid.org/0009-0005-7901-8610; Vira Tovazhnianska, orcid.org/0000-0002-4340-9186; Antonij Slavcev, orcid.org/0000-0002-6154-9131; Katerina Vychytilova, orcid.org/0000-0001-6097-7700; Jitka Smetanova, orcid.org/0000-0001-7744-8119; Alexandre Bohyn, orcid.org/0000-0001-9776-7467; Jaromir Vajter, orcid.org/0000-0001-6277-2151; Martina Borcinova, orcid.org/0000-0002-5016-149X; Bart M. Vanaudenaerde, orcid.org/0000-0001-6435-6901; Robert Lischke, orcid.org/0000-0002-0578-1833; Jiri Vachtenheim Jr., orcid.org/0000-0002-1468-4242; Laurens J. Ceulemans, orcid.org/0000-0002-4261-7100; Zuzana Ozaniak Strizova, orcid.org/0000-0003-4976-9534