- Department of Preclinical Sciences, Institute of Veterinary Medicine, Warsaw University of Life Sciences, Warsaw, Poland
The global pandemic of COVID-19 caused by SARS-CoV-2 has had a devastating impact. Although many survived the acute effects of the pandemic, a significant number of survivors, including those with only mild symptoms, are now experiencing a prolonged and debilitating post-viral syndrome known as LC/PASC (long COVID/post-acute sequelae of SARS-CoV-2). Typical symptoms of LC/PASC include fatigue, breathlessness, chest pain, impaired cognition, difficulty sleeping, fever and gastrointestinal symptoms. Anxiety and depression can also last for weeks to months and range from mild to disabling. The association between neuropsychiatric symptoms and SARS-CoV-2 infection raises questions about the possible routes of SARS-CoV-2 entry to the central nervous system (CNS) and long-term effects of the virus on the CNS, their molecular basis, and the potential risk of neuronal damage associated with the subsequent development of neurodegenerative diseases.
Introduction
As of 31 December 2023, there are more than 773 million reported cases and 7 million deaths worldwide caused by severe acute respiratory syndrome coronavirus 2 (SARS-CoV-2) and coronavirus disease 2019 (COVID-19) (World Health Organization, 2024). While the symptoms of infection are mainly respiratory complications, the neurological manifestations of COVID-19 have been increasingly recognized. Many patients present with mild neurological symptoms such as dizziness, headache, and smell or taste impairment, however a small percentage of patients may develop severe neurological disease, including myopathy, cerebrovascular disease, seizures, movement disorders, encephalitis, Guillain-Barré syndrome, optic neuritis, meningitis, acute transverse myelitis and coma, as well as altered mental status (Mao et al., 2020; Ray et al., 2021; Varatharaj et al., 2020; Whittaker et al., 2020). Moreover, imaging data shows reduction in grey matter thickness and global brain size after SARS-CoV-2 infection (Douaud et al., 2022). In this mini review article, we discussed the possible routes of SARS-CoV-2 entry to the central nervous system (CNS) and the consequences of neuroinvasion based on the emerging evidence.
Neurotropism and neuropathologies caused by SARS-CoV-2
SARS-CoV-2’s neurotropism is a controversial topic. In vitro study using cells and organoids derived from human pluripotent stem cells (hPSC) showed SARS-CoV-2’s tropism for choroid plexus epithelial cells, limited neuronal infection and the inability of axonal trafficking of the virus (Jacob et al., 2020; Luczo et al., 2024). However, hPSC-derived dopaminergic neurons, but not cortical neurons, were shown to be susceptible and permissive to the virus (Yang et al., 2020; 2024). In contrast, in a study done by Kettunen et al. (2023), hPSC-derived cortical neurons were infected by SARS-CoV-2. Moreover, hPSC-derived astrocytes were not infected or rarely showed signs of infection (Jacob et al., 2020; Kettunen et al., 2023). Contradicting results were published by Crunfli et al. (2022), where both hPSC-derived astrocytes and astrocytes in brain samples of COVID-19 patients were infected by the virus. Post mortem studies showed the presence of SARS-CoV-2, i.e., in the dorsal medulla, substantia nigra, frontal lobe, cortical neurons, cranial nerves (Emmi et al., 2023; Matschke et al., 2020; Song et al., 2021).
Possible ways of SARS-CoV-2 entry to the CNS are intensively researched. One of the suspected routes is the olfactory system. As the virus can infect sustentacular cells, there is a question of whether it can gain access to olfactory neurons, i.e., through exosomes, to stem cells that generate olfactory neurons, or to cerebrospinal fluid (Butowt and Bilinska, 2020; Butowt and von Bartheld, 2021). SARS-CoV-2 WA1 and Delta infected hamster model showed the transport of the virus to the brain through olfactory neuron axons, especially in younger animals (Chen et al., 2024). In a study on non-human primates, viral RNA was detected both in the olfactory bulb and brain, with SARS-CoV-2 N protein detected in the axons of olfactory neurons (Shimizu et al., 2024). However, SARS-CoV-2 infection in human olfactory neurons is rare (de Melo et al., 2021; Meinhardt et al., 2021), or not detected (Khan et al., 2021).
Blood-brain barrier disruption is one of the possible ways of viral entry to the CNS. In vitro and in vivo research on brain vascular endothelial cells (BCECs) suggests viral replication and transcellular transport resulting in neuronal damage (Krasemann et al., 2022; Zhang et al., 2021). Although SARS-CoV-2 did replicate in the human in vitro blood-brain barrier (BBB) model, it was limited and did not induce strong inflammatory response or BBB disruption. Moreover, although peripheral inflammation may cause BBB disruption (Huang et al., 2021; Yang et al., 2022), COVID-19 patients’ serum with high concentrations of proinflammatory cytokines also did not disrupt the integrity of BBB in vitro (Constant et al., 2021). However, a study using a 3D microfluidic model of the human BBB showed that SARS-CoV-2 S protein promotes loss of barrier integrity and proinflammatory response (Buzhdygan et al., 2020). Infection of human brain microvascular endothelial cells (HBMEC) also showed proinflammatory activation, possibly by NF-κB non-canonical pathway, and remodelling of mitochondrial network and tight junctions, even without active replication (Motta et al., 2023). Post mortem studies do not give a definitive answer to whether BBB epithelium can or cannot be infected. ACE2, the entry receptor of SARS-CoV-2, is expressed in brain epithelium (Hamming et al., 2004; Zhou et al., 2020). Viral particles were detected in the frontal lobe in neural and capillary endothelial cells (Paniz-Mondolfi et al., 2020), however, RNA sequencing did not detect SARS-CoV-2 presence in brain tissues, including choroid plexus epithelium (Fullard et al., 2021; Yang et al., 2021). However, multifocal microvascular injury was observed in brain tissue and olfactory bulbs of patients who died of COVID-19 (Lee et al., 2021).
Enhanced expression of proinflammatory cytokines and chemokines is associated with ageing and age-related diseases, i.e., Parkinson’s Disease (PD) and Alzheimer’s Disease (AD) (Rea et al., 2018). SARS-CoV-2 and its proteins activate toll-like receptors (TLRs) – TLR2 and TLR4, leading to proinflammatory cytokine expression (Asaba et al., 2024; Fontes-Dantas et al., 2023; Sariol and Perlman, 2021; Szabo et al., 2022). Infected HBMEC showed upregulation of genes encoding factors related to endothelial activation pathways – CXCL1, -2, -8, CCL20, TNF, IL-6, IL-8, and that can lead to a BBB disruption and contribute to neuroinflammation (Motta et al., 2023). Infection of a non-human primate model resulted in neuroinflammation and neuronal damage, with pathology being more pronounced in aged and diabetic macaques (Beckman et al., 2022). Viral infection of microglia and astrocytes is suspected to be an important factor in neurological disorders development. The infection of microglia leads to M1-like proinflammatory response, production of cytokines and chemokines, i.e., IL-1β, IL-6, TNF-α, IFN-γ, CCL11, and NLRP3 inflammasome activation (Albornoz et al., 2023; Fernández-Castañeda et al., 2022; Jeong et al., 2022; Krasemann et al., 2022). SARS-CoV-2 has been found to infect astrocytes, leading to cell activation, elevated expression of inflammatory genes, cytokine and growth factor signalling in both infected and bystander astrocytes (Andrews et al., 2022). Infected astrocytes had changes in energy metabolism, and that could indirectly result in the reduction of neuronal viability (Crunfli et al., 2022). Brains of patients who died of COVID-19 studied post mortem showed neuropathological changes with astrogliosis, microgliosis and cytotoxic T lymphocytes infiltration, hallmarks of neuroinflammation (Matschke et al., 2020). What is more, SARS-CoV-2 infection outside of CNS can lead to cytokine storm (Hu et al., 2021), and as a result, cytokines and chemokines in the blood may cause BBB disruption and consequently lead to microglia and astrocyte activation (Meinhardt et al., 2023) (Figure 1).
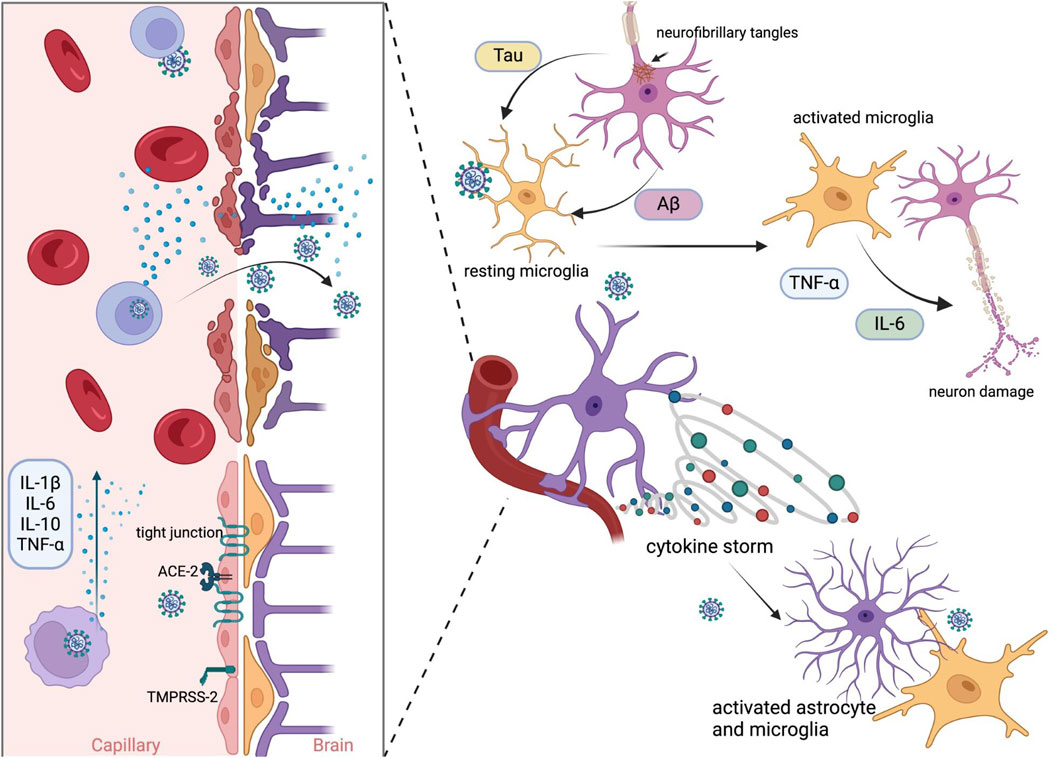
Figure 1. Possible mechanism of SARS-CoV-2 neuroinvasion by BBB disruption. Infection begins with proteolytic activation of the S protein by furin protease-TMPRSS-2 and binding to the ACE2 receptor in epithelial cells. Viral replication promotes the activation of inflammatory mechanisms. The release of primary proinflammatory cytokines, such as IFN-γ and TNF-α, leads to the activation of immune cells, astrocytes, and microglia. Activated microglial cells induce the release of cytokines such as IL-1, IL-6, and TNF-α, which further activate astrocytes. Activated astrocytes release mediators, which lead to neuroinflammation. These events and viral particles evade the host immune system, resulting in chronic infection and the subsequent deposition of Aβ (amyloid-beta) and phosphorylated tau in the brain. Created in BioRender. Bartak, M. (2023) BioRender.com/q85y420.
Moreover, ORF6 and ORF10 fragments and S protein fragments form amyloid assemblies causing neuronal death (Charnley et al., 2022; Nyström and Hammarström, 2022). PD’s onset and progression are tightly connected to α-synuclein (α-syn) aggregation, which was observed to be promoted by both S and N proteins of SARS-CoV-2 (Semerdzhiev et al., 2023; Wang et al., 2023; Zilio et al., 2023). SARS-CoV-2 infection can also lead to tau phosphorylation, a key factor in tauopathies such as AD (Di Primio et al., 2023; Eberle et al., 2023). Moreover, AD patients seem to be more prone to severe course of infection, which could possibly exacerbate already existing neuropathology (Ciaccio et al., 2021; Meinhardt et al., 2023).
Discussion
Several mechanisms have been suggested to cause neurological symptoms and exacerbation of pre-existing neurological conditions during SARS-CoV-2 infection. These include direct effects of the virus on the CNS, e.g., by nasal entry into the brain and infection of neuronal populations (Meinhardt et al., 2021), and para- or post-infectious effects such as induction of inflammation and autoimmune responses (Kumar et al., 2020; Zubair et al., 2020). These effects of SARS-CoV-2 on the CNS have potential implications for the development of long-term neurological disease, including neurodegeneration.
SARS-CoV-2 neurotropism and entry to the CNS are debated topics. Given that SARS-CoV-2 is a possible cause of synucleinopathies (Albornoz et al., 2023; Iravanpour et al., 2024; Wang et al., 2023) and taupathies (Di Primio et al., 2023; Eberle et al., 2023; Käufer et al., 2022) and has the potential to worsen existing neuropathologies, potential ways of viral entry to the brain tissue should be thoroughly examined. Current research does not give an indisputable answer to why some COVID-19 patients have neurological symptoms, often lasting longer than coronaviral infection. Microglia and astrocyte activation could explain the long COVID syndrome and progression of neurodegenerative diseases (Stein et al., 2023), however, whether the activation occurs as a direct or indirect response to SARS-CoV-2 infection is still a puzzle to solve. Given that human brain tissue is not widely available, more in vitro and in vivo research is needed to better understand these highly significant issues.
In conclusion, it is worth adding that COVID-19 is the first pandemic to occur in the context of an aging population (Adesse et al., 2022; Mitra et al., 2022; Strong, 2023). Its survivors are at a greater risk of developing neurodegenerative diseases as they age. The potential long-term effects on the nervous system could be a lasting legacy of an even greater global health challenge than acute infection.
Author contributions
WK, MB, and JC conceived the work and wrote the manuscript. All authors contributed to the article and approved the submitted version.
Funding
The author(s) declare that no financial support was received for the research, authorship, and/or publication of this article.
Conflict of interest
The authors declare that the research was conducted in the absence of any commercial or financial relationships that could be construed as a potential conflict of interest.
Generative AI statement
The author(s) declare that no Generative AI was used in the creation of this manuscript.
References
Adesse, D., Gladulich, L., Alvarez-Rosa, L., Siqueira, M., Marcos, A. C., Heider, M., et al. (2022). Role of aging in blood–brain barrier dysfunction and susceptibility to SARS-CoV-2 infection: Impacts on neurological symptoms of COVID-19. Fluids Barriers CNS 19 (1), 63. doi:10.1186/S12987-022-00357-5
Albornoz, E. A., Amarilla, A. A., Modhiran, N., Parker, S., Li, X. X., Wijesundara, D. K., et al. (2023). SARS-CoV-2 drives NLRP3 inflammasome activation in human microglia through spike protein. Mol. Psychiatry 28 (7), 2878–2893. doi:10.1038/s41380-022-01831-0
Andrews, M. G., Mukhtar, T., Eze, U. C., Simoneau, C. R., Ross, J., Parikshak, N., et al. (2022). Tropism of SARS-CoV-2 for human cortical astrocytes. Proc. Natl. Acad. Sci. U. S. A. 119 (30), e2122236119. doi:10.1073/pnas.2122236119
Asaba, C. N., Ekabe, C. J., Ayuk, H. S., Gwanyama, B. N., Bitazar, R., and Bukong, T. N. (2024). Interplay of TLR4 and SARS-CoV-2: unveiling the complex mechanisms of inflammation and Severity in COVID-19 infections. J. Inflamm. Res. 17, 5077–5091. doi:10.2147/JIR.S474707
Beckman, D., Bonillas, A., Diniz, G. B., Ott, S., Roh, J. W., Elizaldi, S. R., et al. (2022). SARS-CoV-2 infects neurons and induces neuroinflammation in a non-human primate model of COVID-19. Cell Rep. 41 (5), 111573. doi:10.1016/j.celrep.2022.111573
Butowt, R., and Bilinska, K. (2020). SARS-CoV-2: olfaction, brain infection, and the urgent need for clinical samples allowing earlier virus detection. ACS Chem. Neurosci. 11 (9), 1200–1203. doi:10.1021/acschemneuro.0c00172
Butowt, R., and von Bartheld, C. S. (2021). Anosmia in COVID-19: underlying mechanisms and assessment of an olfactory route to brain infection. Neuroscientist 27 (6), 582–603. doi:10.1177/1073858420956905
Buzhdygan, T. P., DeOre, B. J., Baldwin-Leclair, A., Bullock, T. A., McGary, H. M., Khan, J. A., et al. (2020). The SARS-CoV-2 spike protein alters barrier function in 2D static and 3D microfluidic in-vitro models of the human blood–brain barrier. Neurobiol. Dis. 146, 105131. doi:10.1016/j.nbd.2020.105131
Charnley, M., Islam, S., Bindra, G. K., Engwirda, J., Ratcliffe, J., Zhou, J., et al. (2022). Neurotoxic amyloidogenic peptides in the proteome of SARS-COV2: Potential implications for neurological symptoms in COVID-19. Nat. Commun. 13 (1), 3387. doi:10.1038/s41467-022-30932-1
Chen, M., Pekosz, A., Villano, J. S., Shen, W., Zhou, R., Kulaga, H., et al. (2024). Evolution of nasal and olfactory infection characteristics of SARS-CoV-2 variants. J. Clin. Investigation 134 (8), e174439. doi:10.1172/JCI174439
Ciaccio, M., Lo Sasso, B., Scazzone, C., Gambino, C. M., Ciaccio, A. M., Bivona, G., et al. (2021). COVID-19 and Alzheimer’s disease. Brain Sci. 11 (3), 305. doi:10.3390/brainsci11030305
Constant, O., Barthelemy, J., Bolloré, K., Tuaillon, E., Gosselet, F., Chable-Bessia, C., et al. (2021). SARS-CoV-2 poorly replicates in cells of the human blood-brain barrier without associated deleterious effects. Front. Immunol. 12, 697329. doi:10.3389/fimmu.2021.697329
Crunfli, F., Carregari, V. C., Veras, F. P., Silva, L. S., Nogueira, M. H., Antunes, A. S. L. M., et al. (2022). Morphological, cellular, and molecular basis of brain infection in COVID-19 patients. Proc. Natl. Acad. Sci. U. S. A. 119 (35), e2200960119. doi:10.1073/pnas.2200960119
de Melo, G. D., Lazarini, F., Levallois, S., Hautefort, C., Michel, V., Larrous, F., et al. (2021). COVID-19-related anosmia is associated with viral persistence and inflammation in human olfactory epithelium and brain infection in hamsters. Sci. Transl. Med. 13 (596), eabf8396. doi:10.1126/scitranslmed.abf8396
Di Primio, C., Quaranta, P., Mignanelli, M., Siano, G., Bimbati, M., Scarlatti, A., et al. (2023). Severe acute respiratory syndrome coronavirus 2 infection leads to Tau pathological signature in neurons. PNAS Nexus 2 (9), pgad282. doi:10.1093/pnasnexus/pgad282
Douaud, G., Lee, S., Alfaro-Almagro, F., Arthofer, C., Wang, C., McCarthy, P., et al. (2022). SARS-CoV-2 is associated with changes in brain structure in UK Biobank. Nature 604 (7907), 697–707. doi:10.1038/s41586-022-04569-5
Eberle, R. J., Coronado, M. A., Gering, I., Sommerhage, S., Korostov, K., Stefanski, A., et al. (2023). Tau protein aggregation associated with SARS-CoV-2 main protease. PLoS ONE 18, e0288138. doi:10.1371/journal.pone.0288138
Emmi, A., Rizzo, S., Barzon, L., Sandre, M., Carturan, E., Sinigaglia, A., et al. (2023). Detection of SARS-CoV-2 viral proteins and genomic sequences in human brainstem nuclei. Npj Parkinson’s Dis. 9 (1), 25. doi:10.1038/s41531-023-00467-3
Fernández-Castañeda, A., Lu, P., Geraghty, A. C., Song, E., Lee, M. H., Wood, J., et al. (2022). Mild respiratory COVID can cause multi-lineage neural cell and myelin dysregulation. Cell 185 (14), 2452–2468.e16. doi:10.1016/j.cell.2022.06.008
Fontes-Dantas, F. L., Fernandes, G. G., Gutman, E. G., De Lima, E. V., Antonio, L. S., Hammerle, M. B., et al. (2023). SARS-CoV-2 spike protein induces TLR4-mediated long-term cognitive dysfunction recapitulating post-COVID-19 syndrome in mice. Cell Rep. 42 (3), 112189. doi:10.1016/j.celrep.2023.112189
Fullard, J. F., Lee, H. C., Voloudakis, G., Suo, S., Javidfar, B., Shao, Z., et al. (2021). Single-nucleus transcriptome analysis of human brain immune response in patients with severe COVID-19. Genome Med. 13 (1), 118. doi:10.1186/s13073-021-00933-8
Hamming, I., Timens, W., Bulthuis, M. L. C., Lely, A. T., Navis, G. J., and van Goor, H. (2004). Tissue distribution of ACE2 protein, the functional receptor for SARS coronavirus. A first step in understanding SARS pathogenesis. J. Pathology 203 (2), 631–637. doi:10.1002/path.1570
Hu, B., Huang, S., and Yin, L. (2021). The cytokine storm and COVID-19. J. Med. Virology 93 (1), 250–256. doi:10.1002/jmv.26232
Huang, X., Hussain, B., and Chang, J. (2021). Peripheral inflammation and blood–brain barrier disruption: effects and mechanisms. CNS Neurosci. Ther. 27 (1), 36–47. doi:10.1111/cns.13569
Iravanpour, F., Farrokhi, R., Jafarinia, M., and Oliaee, R. T. (2024). The effect of SARS-CoV-2 on the development of Parkinson’s disease: the role of α-synuclein. Hum. Cell 37, 1–8. doi:10.1007/s13577-023-00988-2
Jacob, F., Pather, S. R., Huang, W. K., Zhang, F., Wong, S. Z. H., Zhou, H., et al. (2020). Human pluripotent stem cell-derived neural cells and brain organoids reveal SARS-CoV-2 neurotropism predominates in choroid plexus epithelium. Cell Stem Cell 27 (6), 937–950.e9. doi:10.1016/j.stem.2020.09.016
Jeong, G. U., Lyu, J., Kim, K.-D., Chung, Y. C., Yoon, G. Y., Lee, S., et al. (2022). SARS-CoV-2 infection of microglia elicits proinflammatory activation and apoptotic cell death. Microbiol. Spectr. 10 (3), e0109122. doi:10.1128/spectrum.01091-22
Käufer, C., Schreiber, C. S., Hartke, A.-S., Denden, I., Stanelle-Bertram, S., Beck, S., et al. (2022). Microgliosis and neuronal proteinopathy in brain persist beyond viral clearance in SARS-CoV-2 hamster model. EBioMedicine 79, 103999. doi:10.1016/j.ebiom.2022.103999
Kettunen, P., Lesnikova, A., Räsänen, N., Ojha, R., Palmunen, L., Laakso, M., et al. (2023). SARS-CoV-2 infection of human neurons is TMPRSS2 independent, requires endosomal cell entry, and can Be blocked by inhibitors of host phosphoinositol-5 kinase. J. Virology 97 (4), e0014423. doi:10.1128/jvi.00144-23
Khan, M., Yoo, S. J., Clijsters, M., Backaert, W., Vanstapel, A., Speleman, K., et al. (2021). Visualizing in deceased COVID-19 patients how SARS-CoV-2 attacks the respiratory and olfactory mucosae but spares the olfactory bulb. Cell 184 (24), 5932–5949.e15. doi:10.1016/j.cell.2021.10.027
Krasemann, S., Haferkamp, U., Pfefferle, S., Woo, M. S., Heinrich, F., Schweizer, M., et al. (2022). The blood-brain barrier is dysregulated in COVID-19 and serves as a CNS entry route for SARS-CoV-2. Stem cell Rep. 17 (2), 307–320. doi:10.1016/j.stemcr.2021.12.011
Kumar, A., Pareek, V., Prasoon, P., Faiq, M. A., Kumar, P., Kumari, C., et al. (2020). Possible routes of SARS-CoV-2 invasion in brain: in context of neurological symptoms in COVID-19 patients. J. Neurosci. Res. 98, 2376–2383. doi:10.1002/jnr.24717
Lee, M.-H., Perl, D. P., Nair, G., Li, W., Maric, D., Murray, H., et al. (2021). Microvascular injury in the brains of patients with covid-19. N. Engl. J. Med. 384 (5), 481–483. doi:10.1056/nejmc2033369
Luczo, J. M., Edwards, S. J., Ardipradja, K., Suen, W. W., Gough, ·, Au, G., et al. (2024). SARS-CoV and SARS-CoV-2 display limited neuronal infection and lack the ability to transmit within synaptically connected axons in stem cell-derived human neurons. J. NeuroVirology 1, 39–51. doi:10.1007/s13365-023-01187-3
Mao, L., Jin, H., Wang, M., Hu, Y., Chen, S., He, Q., et al. (2020). Neurologic manifestations of hospitalized patients with coronavirus disease 2019 in wuhan, China. JAMA Neurol. 77 (6), 683. doi:10.1001/jamaneurol.2020.1127
Matschke, J., Lütgehetmann, M., Hagel, C., Sperhake, J. P., Schröder, A. S., Edler, C., et al. (2020). Neuropathology of patients with COVID-19 in Germany: A post-mortem case series. Lancet Neurology 19 (11), 919–929. doi:10.1016/S1474-4422(20)30308-2
Meinhardt, J., Radke, J., Dittmayer, C., Franz, J., Thomas, C., Mothes, R., et al. (2021). Olfactory transmucosal SARS-CoV-2 invasion as a port of central nervous system entry in individuals with COVID-19. Nat. Neurosci. 24 (2), 168–175. doi:10.1038/s41593-020-00758-5
Meinhardt, J., Streit, S., Dittmayer, C., Manitius, R. v., Radbruch, H., and Heppner, F. L. (2023). The neurobiology of SARS-CoV-2 infection. Nat. Rev. Neurosci. 25 (1), 30–42. doi:10.1038/s41583-023-00769-8
Mitra, J., Kodavati, M., Provasek, V. E., Rao, K. S., Mitra, S., Hamilton, D. J., et al. (2022). SARS-CoV-2 and the central nervous system: emerging insights into hemorrhage-associated neurological consequences and therapeutic considerations. Ageing Res. Rev. 80, 101687. doi:10.1016/J.ARR.2022.101687
Motta, C. S., Torices, S., da Rosa, B. G., Marcos, A. C., Alvarez-Rosa, L., Siqueira, M., et al. (2023). Human brain microvascular endothelial cells exposure to SARS-CoV-2 leads to inflammatory activation through NF-κB non-canonical pathway and mitochondrial remodeling. Viruses 15 (3), 745. doi:10.3390/v15030745
Nyström, S., and Hammarström, P. (2022). Amyloidogenesis of SARS-CoV-2 spike protein. J. Am. Chem. Soc. 144 (20), 8945–8950. doi:10.1021/jacs.2c03925
Paniz-Mondolfi, A., Bryce, C., Grimes, Z., Gordon, R. E., Reidy, J., Lednicky, J., et al. (2020). Central nervous system involvement by severe acute respiratory syndrome coronavirus-2 (SARS-CoV-2). J. Med. Virology 92 (7), 699–702. doi:10.1002/jmv.25915
Ray, S. T. J., Abdel-Mannan, O., Sa, M., Fuller, C., Wood, G. K., Pysden, K., et al. (2021). Neurological manifestations of SARS-CoV-2 infection in hospitalised children and adolescents in the UK: a prospective national cohort study. Lancet Child Adolesc. Health 5 (9), 631–641. doi:10.1016/S2352-4642(21)00193-0
Rea, I. M., Gibson, D. S., McGilligan, V., McNerlan, S. E., Denis Alexander, H., and Ross, O. A. (2018). Age and age-related diseases: role of inflammation triggers and cytokines. Front. Immunol. 9 (586), 586. doi:10.3389/fimmu.2018.00586
Sariol, A., and Perlman, S. (2021). SARS-CoV-2 takes its Toll. Nat. Immunol. 22 (7), 801–802. doi:10.1038/s41590-021-00962-w
Semerdzhiev, S. A., Segers-Nolten, I., Van Der Schoot, P., Blum, C., and Claessens, M. M. A. E. (2023). SARS-CoV-2 N-protein induces the formation of composite α-synuclein/N-protein fibrils that transform into a strain of α-synuclein fibrils. Nanoscale 15 (45), 18337–18346. doi:10.1039/d3nr03556e
Shimizu, S., Nakayama, M., Thanh Nguyen, C., Ishigaki, H., Kitagawa, Y., Yasui, F., et al. (2024). SARS-CoV-2 induces inflammation and intracranial infection through the olfactory epithelium-olfactory bulb pathway in non-human primates. J. Neuroimmunol. 387, 578288. doi:10.1016/j.jneuroim.2024.578288
Song, E., Zhang, C., Israelow, B., Lu-Culligan, A., Prado, A. V., Skriabine, S., et al. (2021). Neuroinvasion of SARS-CoV-2 in human and mouse brain. J. Exp. Med. 218 (3), e20202135. doi:10.1084/JEM.20202135
Stein, J. A., Kaes, M., Smola, S., and Schulz-Schaeffer, W. J. (2023). Neuropathology in COVID-19 autopsies is defined by microglial activation and lesions of the white matter with emphasis in cerebellar and brain stem areas. Front. Neurology 14, 1229641. doi:10.3389/fneur.2023.1229641
Strong, M. J. (2023). SARS-CoV-2, aging, and Post-COVID-19 neurodegeneration. J. Neurochem. 165 (2), 115–130. doi:10.1111/JNC.15736
Szabo, M. P., Iba, M., Nath, A., Masliah, E., and Kim, C. (2022). Does SARS-CoV-2 affect neurodegenerative disorders? TLR2, a potential receptor for SARS-CoV-2 in the CNS. Exp. Mol. Med. 54 (4), 447–454. doi:10.1038/s12276-022-00755-7
Varatharaj, A., Thomas, N., Ellul, M. A., Davies, N. W. S., Pollak, T. A., Tenorio, E. L., et al. (2020). Neurological and neuropsychiatric complications of COVID-19 in 153 patients: a UK-wide surveillance study. Lancet Psychiatry 7 (10), 875–882. doi:10.1016/S2215-0366(20)30287-X
Wang, J., Dai, L., Deng, M., Xiao, T., Zhang, Z., and Zhang, Z. (2023). SARS-CoV-2 spike protein S1 domain accelerates α-synuclein phosphorylation and aggregation in cellular models of synucleinopathy. Mol. Neurobiol. 61 (4), 2446–2458. doi:10.1007/s12035-023-03726-9
Whittaker, A., Anson, M., and Harky, A. (2020). Neurological manifestations of COVID-19: a systematic review and current update. Acta Neurol. Scand. 142 (1), 14–22. doi:10.1111/ane.13266
World Health Organization (2024). WHO COVID-19 dashboard. Available at: https://data.who.int/dashboards/covid19/cases (Accessed January 15, 2024).
Yang, A. C., Kern, F., Losada, P. M., Agam, M. R., Maat, C. A., Schmartz, G. P., et al. (2021). Dysregulation of brain and choroid plexus cell types in severe COVID-19. Nature 595 (7868), 565–571. doi:10.1038/s41586-021-03710-0
Yang, J., Ran, M., Li, H., Lin, Y., Ma, K., Yang, Y., et al. (2022). New insight into neurological degeneration: inflammatory cytokines and blood–brain barrier. Front. Mol. Neurosci. 15, 1013933. doi:10.3389/fnmol.2022.1013933
Yang, L., Han, Y., Nilsson-Payant, B. E., Gupta, V., Wang, P., Duan, X., et al. (2020). A human pluripotent stem cell-based platform to study SARS-CoV-2 tropism and model virus infection in human cells and organoids. Cell Stem Cell 27 (1), 125–136.e7. doi:10.1016/j.stem.2020.06.015
Yang, L., Kim, T. W., Han, Y., Nair, M. S., Harschnitz, O., Zhu, J., et al. (2024). SARS-CoV-2 infection causes dopaminergic neuron senescence. Cell Stem Cell 31 (2). doi:10.1016/J.STEM.2023.12.012
Zhang, L., Zhou, L., Bao, L., Liu, J., Zhu, H., Lv, Q., et al. (2021). SARS-CoV-2 crosses the blood–brain barrier accompanied with basement membrane disruption without tight junctions alteration. Signal Transduct. Target. Ther. 6 (1), 337. doi:10.1038/s41392-021-00719-9
Zhou, P., Yang, X. L., Wang, X. G., Hu, B., Zhang, L., Zhang, W., et al. (2020). A pneumonia outbreak associated with a new coronavirus of probable bat origin. Nature 579 (7798), 270–273. doi:10.1038/S41586-020-2012-7
Zilio, G., Masato, A., Sandre, M., Caregnato, A., Moret, F., Maciola, A. K., et al. (2023). SARS-CoV-2-Mimicking pseudoviral particles accelerate α-synuclein aggregation in vitro. ACS Chem. Neurosci. 15 (2), 215–221. doi:10.1021/acschemneuro.3c00468
Keywords: SARS-CoV-2, long COVID symptoms, neurotropism, neurodegeneration, CNS
Citation: Krahel WD, Bartak M and Cymerys J (2024) Acute and long-term SARS-CoV-2 infection and neurodegeneration processes—circulus vitiosus. Acta Virol. 68:12765. doi: 10.3389/av.2024.12765
Received: 29 January 2024; Accepted: 04 October 2024;
Published: 15 October 2024.
Edited by:
Katarina Polcicova, Slovak Academy of Sciences, SlovakiaReviewed by:
Peter Sabaka, Comenius University, SlovakiaZinaida Klestova, University of Tübingen, Germany
Copyright © 2024 Krahel, Bartak and Cymerys. This is an open-access article distributed under the terms of the Creative Commons Attribution License (CC BY). The use, distribution or reproduction in other forums is permitted, provided the original author(s) and the copyright owner(s) are credited and that the original publication in this journal is cited, in accordance with accepted academic practice. No use, distribution or reproduction is permitted which does not comply with these terms.
*Correspondence: Weronika Daria Krahel, weronika_krahel@sggw.edu.pl; Joanna Cymerys, joanna_cymerys@sggw.edu.pl