- Department of Virology, The Stephan Angeloff Institute of Microbiology, Bulgarian Academy of Sciences, Sofia, Bulgaria
The challenges in combating enteroviruses continue due to their genetic diversity and the rapid development of resistance. Combining antiviral agents offers a promising solution. Our study evaluated the combined effects of pocapavir, a potent capsid-binding inhibitor, with enterovirus-targeting compounds such as pleconaril, 2-(3,4-dichlorophenoxy)-5-nitrobenzonitrile (MDL-860), guanidine hydrochloride, oxoglaucine, and 2-α-hydroxybenzyl-benzimidazole (HBB). The combinations tested demonstrated additive to highly synergistic antiviral effects without observed combined cytotoxicity. These results suggest their potential for further research and clinical trials in combating enterovirus infections.
Introduction
Enteroviruses (EVs) are a group of single, positive-stranded RNA viruses of the Picornaviridae family that include polioviruses, echoviruses, coxsackieviruses and enteroviruses. EVs are associated with several human diseases and affect millions of people of all ages worldwide each year. Clinical manifestations of enteroviral infection differ by viral type. Commonly, infections caused by non-polio enteroviruses are asymptomatic or result only in an undifferentiated febrile illness, sometimes they are associated with severe diseases such as aseptic meningitis, encephalitis, non-polio flaccid paralysis, dilated cardiomyopathy (Rotbart, 2002). Treatment for these infections is mainly supportive. The combat against enteroviruses follows two main directions: the development and implementation of effective vaccines and the discovery of chemotherapeutic agents that selectively inhibit viral replication.
The historical Salk and Sabin vaccines against polio are at present the only ones applied in clinical practice. To date several vaccines against enterovirus 71 are available or under development in China (Li et al., 2014; Liang and Wang, 2014). Candidate vaccines containing B4, B5, C1, C2, and C4 genogroups are in development (Chong et al., 2014; Chia et al., 2018; Tamura et al., 2022). Vaccines against Coxsackie A16, A6 and A10 is being considered with a view to developing combination/multivalent vaccines (Lim et al., 2018; He et al., 2021; Zhang et al., 2023). Other vaccines such as recombinant and other forms of subunit vaccines, vectored vaccines, virus-like particle vaccines are in the early stage of development (Zhang et al., 2010; He et al., 2021).
The fast development of drug resistance is the main problem in the chemotherapy of enteroviral infections, based until now on monotherapy courses (Loddo, 1980). Several capsid-binding agents (such as pleconaril, disoxaril, pirodavir, vapendavir) as well as 3C protease inhibitors (such as rupintrivir), 3A protein Inhibitors (such as enviroxime) failed in several clinical trials of human enterovirus infection due to limited efficacy or side effects (Tammaro et al., 2023). Currently, pocapavir is undergoing Phase2a, and like pleconaril it is available for use in emergency treatment of severe EV-B infections. Thus, there is a need for more potent therapy, as well as for treatment that may decrease the frequency of the emergence of drug-resistant viruses. One way to fight resistance is by applying drug combination therapy of new or already existing substances with different mechanisms of action.
The main aims of using drug combinations are to achieve a synergistic therapeutic effect, reduce dose and toxicity reduction, and to minimize or delay the induction of drug resistance (Chou, 2006). In a synergistic drug combination, the effect is larger than the additive effect of each individual drug, while antagonistic combination has smaller than the additive effect of each individual drug. An experimental alternative strategy based on the synergistic dual combinations of antivirals against the replication of enteroviruses has been established in previous work (Eggers and Tamm, 1963a; Nikolaeva and Galabov, 1999; Nikolaeva and Galabov, 2000; Nikolaeva-Glomb et al., 2011; Thibaut et al., 2011; Wang et al., 2016; Stoyanova and Galabov, 2021a; Lanko et al., 2021; Ianevski et al., 2022; Ianevski et al., 2024).
In the present study, we tested the combined effects of dual combinations involving pocapavir and a panel of picornavirus inhibitors, each with known mechanisms of action. Pocapavir, an orally active capsid inhibitor, falls within the picornavirus antiviral mechanistic class known as capsid inhibitors. It functions by blocking virus uncoating and preventing the release of viral RNA into cells, thus inhibiting virus replication (Wittekind et al., 2017). Pleconaril inhibits virus uncoating by direct insertion into the canyon of enteroviral capsid protein VP1 (Pevear et al., 1999). 2-(3,4-dichlorophenoxy)-5-nitrobenzonitrile (MDL-860) targets the host phosphatidylinositol-4 kinase III beta (PI4KB) (Arita et al., 2017), while oxoglaucine, an enviroxime-like inhibitor, specifically targets the PI4KB (Arita et al., 2015). Guanidine hydrochloride interferes with the function of the enteroviral 2C protein, thereby preventing the initiation of negative RNA strand synthesis (Loddo et al., 1962; Barton and Flanegan, 1997). 2-α-hydroxybenzyl-benzimidazole (HBB) inhibits the activity of the virus-specific RNA-dependent RNA polymerase, resulting in the suppression of single-stranded RNA synthesis (Eggers and Tamm, 1961; Dmitrieva and Agol, 1974).
Materials and methods
Cells and viruses
Human epithelial type 2 (HEp-2) cells (National Bank for Industrial Microorganisms and Cell Cultures, Sofia, Bulgaria) were grown in Dulbecco’s Modified Eagle’s Medium (DMEM) supplemented with 10% (v/v) Fetal Bovine Serum (FBS) and antibiotics (penicillin: 100 IU/mL; streptomycin: 100 lg/ml). The cells were maintained in a 37°C incubator with 5% CO2 and high humidity. The viruses used in this study included Poliovirus type 1 (PV-1), Coxsackievirus A9 (CV-A9) and Coxsackievirus B4 (CV-B4) (obtained from the collection of The Stephan Angeloff Institute of Microbiology, Bulgarian Academy of Sciences, Sofia). They were produced in HEp-2 cells in maintenance medium consisting of DMEM, supplemented with 0.5% FBS and antibiotics. All pathogens were handled in the BSL-2 laboratory.
Antiviral compounds
Pocapavir (SCH-48973; V-073) was purchased from MedKoo Biosciences (Morrisville, United States); pleconaril (WIN 63843) was synthesized and kindly provided by Dr. Vadim Makarov (State Research Center for Antibiotics, Moscow, Russia); MDL-860 [2-(3,4-dichlorophenoxy)-5-nitrobenzonitrile] was synthesized by Dr. Gerhard Purstinger (University of Innsbruck, Austria); oxoglaucine (1,2,9,10-tetramethoxy-7h-dibenzo [de,g]quinolin-7-one) an aporphinoid alkaloid from Glaucium flavum Cranz, was provided by Dr. Stefan Philipov (Institute of Organic Chemistry, BAS); guanidine hydrochloride was obtained from Eastman Organic Chemicals (New York, United States); HBB (2-α-hydroxybenzyl-benzimidazole) was received by Dr. T. Dmitrieva (Moscow State University, Moscow, Russia). The compounds were prepared as 10 mmol stock solutions dissolved as follows: pleconaril, MDL-860, and HBB in polyethylene glycol (PEG) 400, pocapavir and oxoglaucine in a 1:9 v/v dimethyl sulfoxide (DMSO)/saline solution, guanidine hydrochloride in a saline solution. These stock solutions were then serially diluted to the desired concentrations in DMEM with 0.5% FBS.
Cell culture assays
The assays were performed using 96-well microplates, infected with approximately 100 CCID50 per 0.1 mL of virus. The antiviral effects of the compounds were determined by a cytopathic effect (CPE) inhibition assay. After 1 h of virus adsorption, the compounds were added in various concentrations, and cells were incubated for 48 h at 37°C with 5% CO2 and high humidity. Inhibition of cytopathic effect was determined using a neutral red uptake assay, based on the initial protocol described by Borenfreund and Puerner (1984). The 50% inhibitory concentration (IC50) of the compounds was identified as the concentration that inhibited the development of CPE by 50%. Drug-drug interactions for three replicate assays were analyzed using the three-dimensional model of Prichard and Shipman (1990), using the MacSynergy™ II software program (Prichard et al., 1992) at 95% confidence limits. The program is an analytical tool which describes and quantitates drug interactions, performs all the required calculations necessary to plot dose-response surface, calculate the volume of synergism or antagonism produced and statically evaluates the interactions for significance. The cytotoxicity of each compound and each combination was assessed in parallel by using the same assay without the addition of the virus.
Statistical analysis
Drug-drug interactions were analyzed by the three dimensional method of Prichard and Shipman (1990), and the analysis was conducted with the MacSynergy™ II (version 1.0) software program. MacSynergy™ II graphically plots three dimension interactions that fall above or below a neutral surface (baseline). Interpretation of drug-drug interactions is based on a volume of synergy or antagonism at 95% confidence interval and calculations, which can be positive (synergy), negative (antagonistic), or neutral (no or minimal interactions, additive). Descriptions of additive, synergistic, and antagonistic interactions in this computer model are based on the Bliss independent model. The synergy/antagonism volumes (μM2%) of drug combinations at 95% confidence level were defined as follows: values between −25 and +25 indicate additivity; values between ±25 and ±50 indicate minor but significant antagonism or synergy, respectively; values between ±50 and ±100 are interpreted as moderate synergism or antagonism, respectively; values greater than +100 or less than −100 indicate strong synergy or antagonism, respectively; values over ±1,000 are considered to be the result of experimental error or occasional phenomena and are statistically insignificant (Prichard et al., 1992; Prichard et al., 1993).
Results
Initial, a 50% inhibitory concentrations (IC50) of each compound were determined from the dose-response curves obtained by the cytopathic effect (CPE) inhibition assay. The range of obtained mean values of IC50 for each compound alone against each tested virus is as follows: pocapavir 0.09–0.5 μM, pleconaril 10–25 μM, MDL-860 0.2–4 μM, oxoglaucine 0.05–0.2 μM, guanidine.HCl 350–590 μM and HBB 25–125 μM.
As the next step, we assessed the antiviral activities of various compound pairs, each containing pocapavir, in a checkerboard format using a CPE assay. To determine whether the antiviral activities of the drug combinations were synergistic, antagonistic, or additive, we analyzed the experimental data with the MacSynergy™ II program. This program calculated the theoretical additive interactions based on the Bliss independence theory and represented each dose combination synergy and antagonism as peaks above or below a predicted additive plane (baseline) in a three-dimensional graph. The corresponding mean volumes of synergy and antagonism at the 95% confidence level are summarized in Table 1.
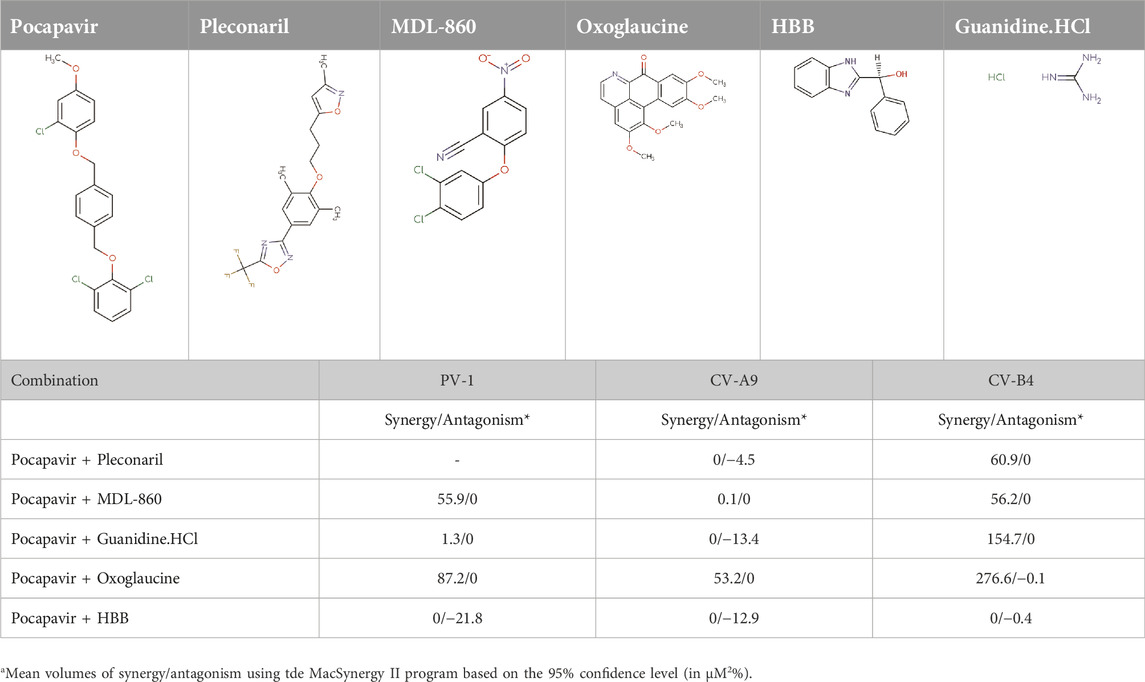
Table 1. Chemical structure of used antivirals and interactions of drug-drug combinations against PV-1, CV-A9 and CV-B4 viruses.
Effect of double combinations against PV-1 virus
The data for the effect of double combinations against PV-1 virus are presented in Table 1 and Figure 1. When pocapavir was individually combined with MDL-860, or oxoglaucine, it resulted in high volumes of synergy (55.9 and 87.2 μM2%, respectively), indicating a moderate synergistic effect. When combined with guanidine.HCl, an additive effect was observed. The combination of pocapavir and HBB showed an additive interaction with increased but not significantly antagonistic (−21.7 μM2%) results. Pleconaril did not exhibit anti-PV-1 activity at doses under 100 μM and was therefore not included in the combination.
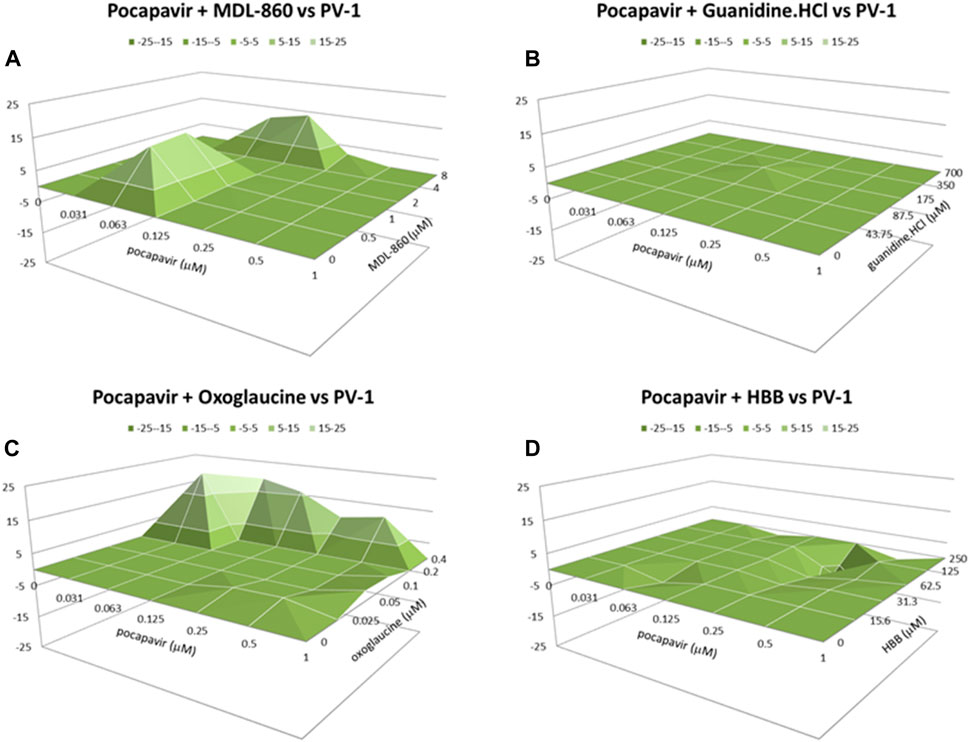
Figure 1. Combined inhibitory effect of pocapavir and MDL-860 (A), guanidine.HCl (B), oxoglaucine (C) and HBB (D) on replication of Polio virus type 1.
Effect of double combinations against CV-A9 virus
Figure 2 present the data obtained for drug-drug combinations against CV-A9 virus replication. In contrast to the combination of pocapavir and oxoglaucine, which yielded a moderate synergistic effect (53.2 μM2%), the other combinations of pocapavir with pleconaril, MDL-860, guanidine.HCl, or HBB resulted in an additive effect with no significant antagonism.
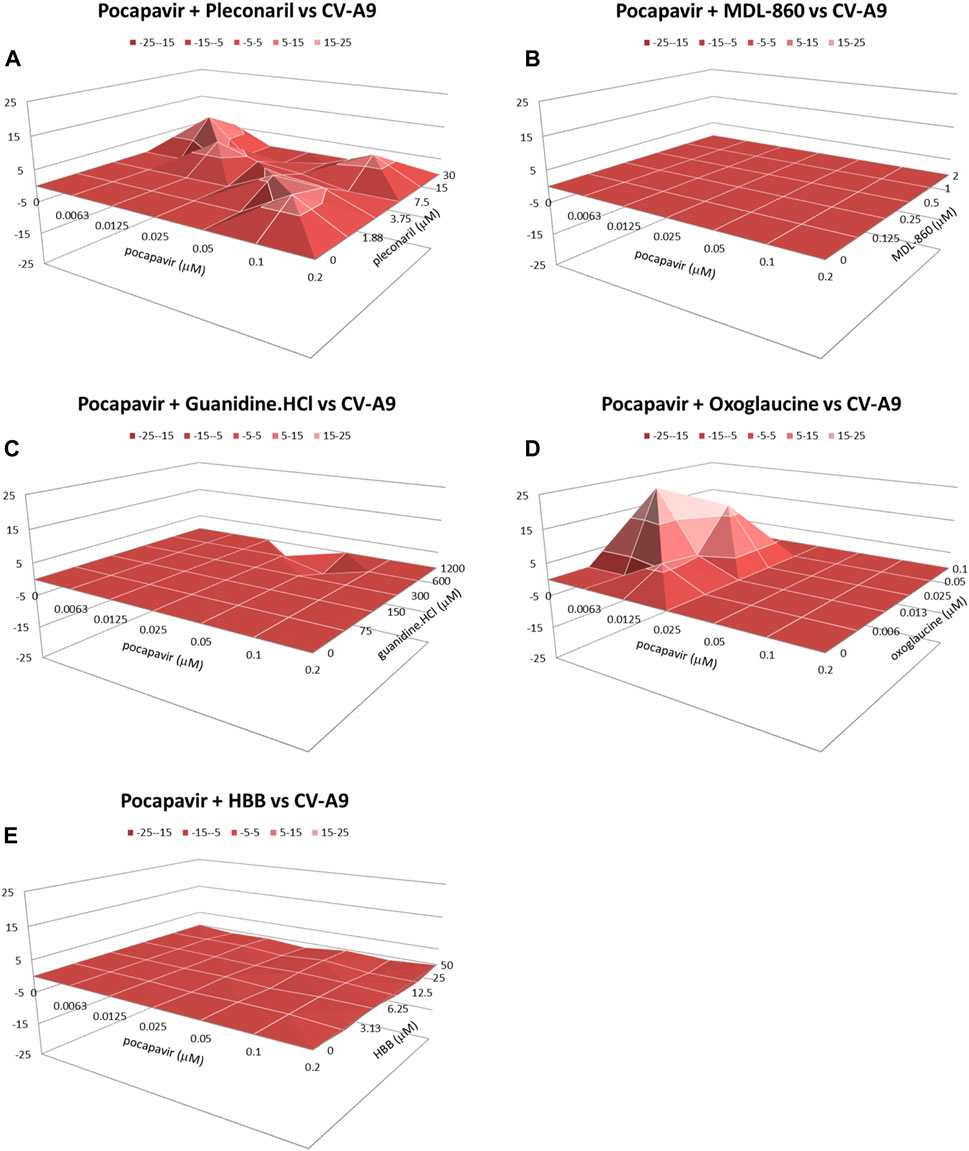
Figure 2. Combined inhibitory effect of pocapavir and pleconaril (A), MDL-860 (B), guanidine.HCl (C), oxoglaucine (D) and HBB (E) on replication of Coxsackievirus A9.
Effect of double combinations against CV-B4 virus
The combinations of pocapavir and oxoglaucine or guanidine.HCl against CV-B4 showed strong synergy (276.6 and 154.7 μM2%, respectively) and a low level of antagonism (Figure 3). The combinations of pocapavir and pleconaril or MDL-860 showed moderate synergy (60.9 and 56.2 μM2%, respectively) with no antagonism present.
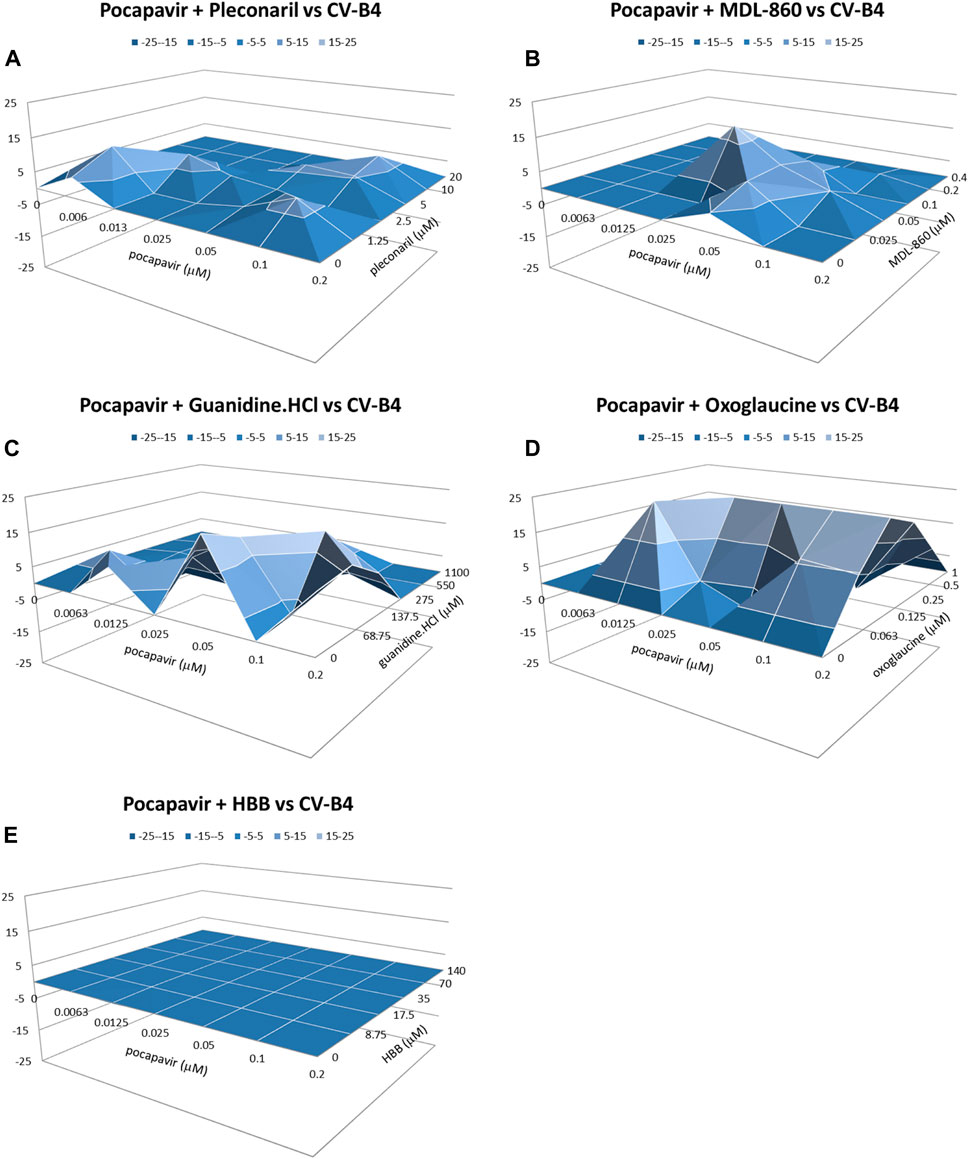
Figure 3. Combined inhibitory effect of pocapavir and pleconaril (A), MDL-860 (B), guanidine.HCl (C), oxoglaucine (D) and HBB (E) on replication of Coxsackievirus B4.
In summary, these experiments revealed a range of moderate to strong synergistic interactions, especially in the combination of pocapavir and oxoglaucine against all included viruses, particularly in CV-B4-induced CPE inhibition. The degrees of synergistic interactions between pocapavir and pleconaril or MDL-860 were essentially the same for PV-1 and CV-A9 inhibition based on the volume of synergy values. Interactions between pocapavir and guanidine.HCl were strong synergistic for CV-B4 CPE inhibition, and additive for PV-1 and CV-A9. The combined effects of pocapavir and HBB were additive.
All combination studies included evaluations of cell viability, and none of the synergistic and additive combinations showed cytotoxicity within the range of drug concentrations examined (Figure 4).
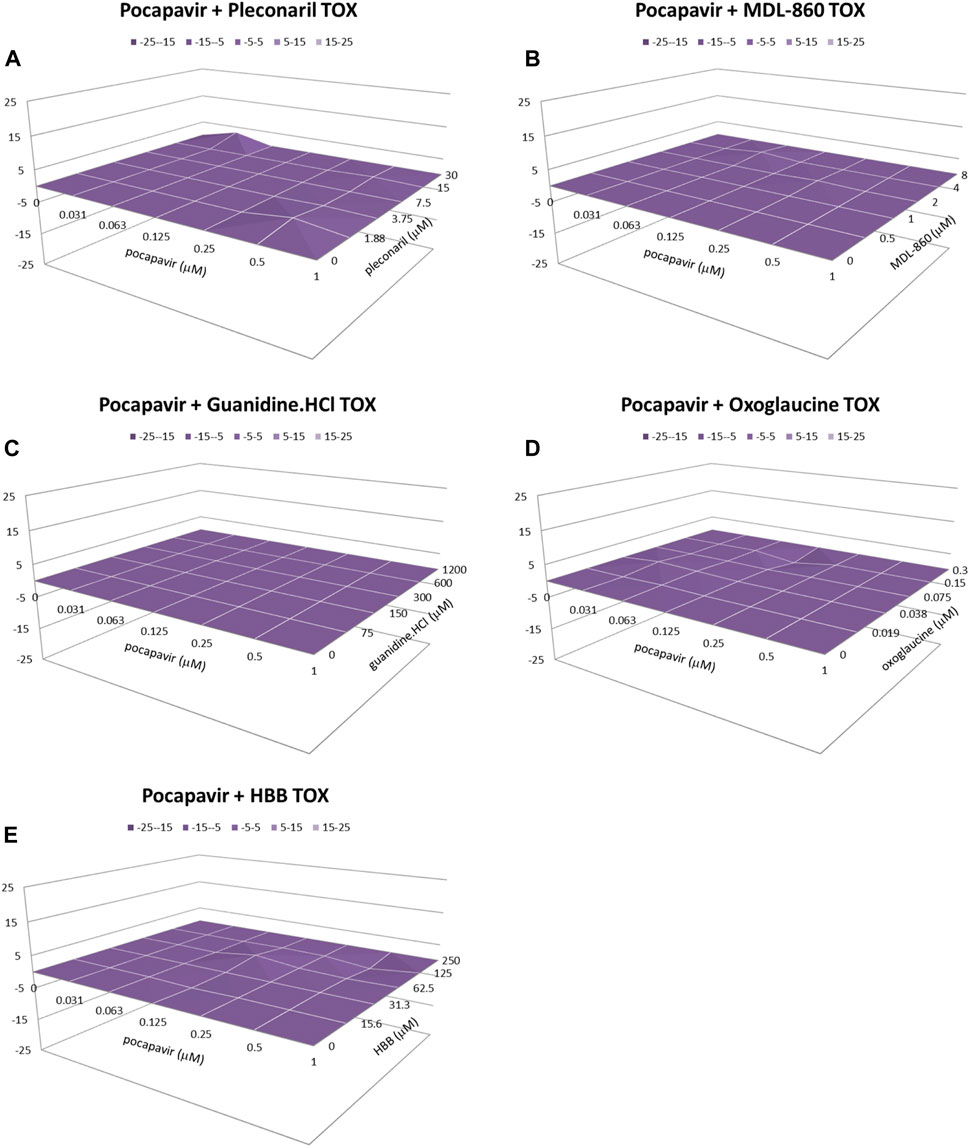
Figure 4. Cytotoxicity of pocapavir and pleconaril (A), MDL-860 (B), guanidine.HCl (C), oxoglaucine (D) and HBB (E) on HEp-2 cells. *Analysis of drug combinations using the MacSynergy II program. Data shown were obtained at the 95% confidence level. Values in the zero plane indicate additive activity, values under the zero plane indicate antagonistic activity, and values above the zero plane indicate synergistic activity. All data points are averages of four measurements from at least three independent experiments.
Discussion
Enteroviruses are widespread and economically significant, making them a significant challenge for researchers. Their genetic diversity and rapid development of resistance make them particularly hard to combat. To address this, our team is exploring various methods, such as the use of drug combinations, which can reduce the likelihood of drug-resistant mutants emerging (Stoyanova and Galabov, 2020; Stoyanova and Galabov, 2021a; Stoyanova and Galabov, 2021b). The use of drug combinations reduces the possibility of selecting drug-resistant mutants (Mouton, 1999). Additionally, drug combinations that leverage synergistic interactions can enhance antiviral efficacy while minimizing cytotoxicity by reducing the required therapeutic doses. Combining antiviral agents with different resistance profiles and modes of action creates a higher genetic barrier to resistance development, suppressing its emergence.
Our study aimed to evaluate the combined effects of pocapavir and compounds with anti-enterovirus activity and a known mode of action against different enteroviruses. Pocapavir is a potent capsid-binding inhibitor that blocks virus uncoating and viral RNA release, thus preventing virus replication. It had previously shown promise in in vitro/vivo activities and had undergone phase II clinical trials for poliomyelitis treatment, but no recent reports on its development were identified (Collett et al., 2017). In this study, we selected five other compounds based on their mechanisms of action for combination studies and conducted two-drug combination experiments. Pleconaril, like pocapavir, inhibits virus uncoating by binding to the viral particle in the pocket of enteroviral structural protein VP1 (Pevear et al., 1999), which stabilizes the capsid, prevents conformational changes, and inhibits viral genome release. The rapid development of resistance to capsid binders is a major problem in the clinical development of this class of compounds. MDL-860 is a broad-spectrum antipicornavirus compound, it targets the host PI4KB and causes covalent modification and irreversible inactivation of the PI4KB (Arita et al., 2017). Oxoglaucine is an inhibitor of the PI4KB/oxysterol-binding protein (OSBP) pathway in EV replication, directly targeting the PI4KB (Arita et al., 2015). Guanidine hydrochloride and HBB suggest similar but not identical mechanisms of antiviral action, by blocking viral RNA synthesis (Eggers and Tamm, 1963b). Guanidine hydrochloride interferes with the function of the 2C protein, one of the most conserved polyproteins of the EVs, thereby preventing the initiation of negative RNA strand synthesis (Loddo et al., 1962; Barton and Flanegan, 1997). HBB blocks viral RNA synthesis by inhibiting the activity of the enteroviral RNA-dependent RNA polymerase (Eggers and Tamm, 1961; Dmitrieva and Agol, 1974).
The combinations of pocapavir and any of the five other compounds produced at least additive antiviral effects on virus-induced CPE inhibition. This suggests that pocapavir’s mode of action is not affected by the mechanisms of the other compounds, based on the absence of significant antagonism. The highest synergistic effect for each of the used viruses was observe with the combination of pocapavir and oxoglaucine. A similar, albeit more modest, result was observed in the combination of pocapavir and MDL-860. Oxoglaucine and MDL-860 have similar modes of antiviral action. The combined effect of pocapavir and MDL-860 was moderately synergistic against PV-1 and CV-B4, while for CV-A9, the combined effect was additive. Based on these results, we can speculate that, the combination of capsid-binding inhibitor and compounds blocking host proteins, providing key stages of viral RNA replication, has a moderate to strong inhibitory effect on viral progeny, depending on the virus. On the other hand, in this case, combining an entry inhibitor with inhibitors of a specific viral RNA synthesis, does not result in an increased inhibitory effect, except in combination with guanidine hydrochloride against CV-A9. The combined effect of pocapavir and HBB was additive against each of the three viruses, while the effect of combination with guanidine hydrochloride resulted in an additive effect against PV-1 and CV-A9, and a synergistic effect against CV-B4. Similar results were obtained in previous studies involving other capsid-binding inhibitors (Nikolaeva and Galabov, 1999; Nikolaeva-Glomb and Galabov, 2004; Stoyanova and Galabov, 2021a). Pocapavir combined with oxoglaucine demonstrated a synergistic effect, while the combination of pleconaril or disoxaril and oxoglaucine had an additive effect. The effect of combination of pocapavir and pleconaril resulted in moderate synergism against CV-B4 replication, and an additive effect on CV-A9. Based on the obtained results, it is noticed that CV-B4 has a higher sensitivity to the examined combinations.
The differences in observed effects among individual virus strains can be attributed to variations in sensitivity to tested compounds. This suggests that since the mechanism of action of the tested compounds is known and enteroviruses share a similar replicative cycle, differences in combined effects may stem from individual variances in target region and thus the compound’s impact on specific viral strains. However, additional studies are needed to clarify this statement.
In summary, four of the analyzed two-drug combinations (i.e., pocapavir plus pleconaril, pocapavir plus MDL-860, pocapavir plus oxoglaucine and pocapavir plus guanidine.HCl) demonstrate moderate to strong synergistic antiviral effects, without cytotoxicity, in HEp-2 cell culture. These results suggest the potential for further in vivo research and provide a basis for combining anti-enteroviral agents in clinical trials to combat enteroviral infections.
Data availability statement
The raw data supporting the conclusion of this article will be made available by the authors, without undue reservation.
Ethics statement
Ethical approval was not required for the studies on humans in accordance with the local legislation and institutional requirements because only commercially available established cell lines were used.
Author contributions
Conceptualization, AS and AG; methodology, AS and SG; formal analysis, AS and SG; investigation, AS and SG; data curation, AS; writing—original draft preparation, AS, AG, and SG; project administration, AS; funding acquisition, AS. All authors contributed to the article and approved the submitted version.
Funding
This work was funded by National Science Fund of Bulgaria (Project KП-06-М51.5/2021).
Conflict of interest
The authors declare that the research was conducted in the absence of any commercial or financial relationships that could be construed as a potential conflict of interest.
References
Arita, M., Dobrikov, G., Pürstinger, G., and Galabov, A. S. (2017). Allosteric regulation of phosphatidylinositol 4-kinase III beta by an anti-picornavirus compound MDL-860. ACS Infect. Dis. 3 (8), 585–594. doi:10.1021/acsinfecdis.7b00053
Arita, M., Philipov, S., and Galabov, A. S. (2015). Phosphatidylinositol 4-kinase III beta is the target of oxoglaucine and pachypodol (Ro 09-0179) for their anti-poliovirus activities, and is located at upstream of the target step of brefeldin A. Microbiol. Immunol. 59, 338–347. doi:10.1111/1348-0421.12261
Barton, D. J., and Flanegan, J. B. (1997). Synchronous replication of poliovirus RNA: Initiation of negative-strand RNA synthesis requires the guanidine-inhibited activity of protein 2C. J. Virol. 71, 8482–8489. doi:10.1128/jvi.71.11.8482-8489.1997
Borenfreund, E., and Puerner, J. A. (1984). A simple quantitative procedure using monolayer cultures for cytotoxicity assays (HTD/NR-90). J. Tissue Cult. Meth. 9, 7–9. doi:10.1007/BF01666038
Chia, M. Y., Chung, W. Y., Wang, C. H., Chang, W. H., and Lee, M. S. (2018). Development of a high-growth enterovirus 71 vaccine candidate inducing cross-reactive neutralizing antibody responses. Vaccine 36 (9), 1167–1173. doi:10.1016/j.vaccine.2018.01.041
Chong, P., Liu, C. C., Chow, Y. H., Chou, A. H., and Klein, M. (2014). Review of enterovirus 71 vaccines. Clin. Infect. Dis. official Publ. Infect. Dis. Soc. Am. 60, 797–803. doi:10.1093/cid/ciu852
Chou, T. C. (2006). Theoretical basis, experimental design, and computerized simulation of synergism and antagonism in drug combination studies. Pharmacol. Rev. 68, 621–681. doi:10.1124/pr.58.3.10
Collett, M. S., Hincks, J. R., Benschop, K., Duizer, E., van der Avoort, H., Rhoden, E., et al. (2017). Antiviral activity of pocapavir in a randomized, blinded, placebo-controlled human oral poliovirus vaccine challenge model. J. Infect. Dis. 215, 335–343. doi:10.1093/infdis/jiw542
Dmitrieva, T. M., and Agol, V. (1974). Selective inhibition of the synthesis of single-stranded RNA of encephalomyocarditis virus by 2-(a-Hydroxybenzyl)-benzimidazole in cell free systems. V. I. Arch. Ges. Virusforsch 45, 17–26. doi:10.1007/BF01240538
Eggers, H., and Tamm, I. (1961). Spectrum and characteristics of the virus inhibitory action of HBB. J. Exp. Med. 111, 657. doi:10.1084/jem.113.4.657
Eggers, H., and Tamm, I. (1963a). Drug dependence of enteroviruses: variants of Coxsackie A9 and ECHO 13 viruses that require 2-(a-Hydroxybenzyl)-benzimidazole for growth. Virology 20, 62–74. doi:10.1016/0042-6822(63)90141-7
Eggers, H. J., and Tamm, I. (1963b). Inhibition of enterovirus ribonucleic acid synthesis by 2-(α-hydroxybenzyl)-benzimidazole. Nature 197, 1327–1328. doi:10.1038/1971327a0
He, X., Zhang, M., Zhao, C., Zheng, P., and Xu, J. (2021). From monovalent to multivalent vaccines, the exploration for potential preventive strategies against hand, foot, and mouth disease (HFMD). Virol. Sin. 36, 167–175. doi:10.1007/s12250-020-00294-3
Ianevski, A., Frøysa, A. T., Lysvand, H., Calitz, C., Smura, T., Nilsen, H. J. S., et al. (2024). The combination of pleconaril, rupintrivir, and remdesivir efficiently inhibits enterovirus infections in vitro, delaying the development of drug-resistant virus variants. Antivir. Res. 224, 105842. doi:10.1016/j.antiviral.2024.105842
Ianevski, A., Zusinaite, E., Tenson, T., Oksenych, V., Wang, W., Afset, J. E., et al. (2022). Novel synergistic anti-enteroviral drug combinations. Viruses 14 (9), 1866. doi:10.3390/v14091866
Lanko, K., Shi, C., Patil, S., Delang, L., Matthijnssens, J., Mirabelli, C., et al. (2021). Assessing in vitro resistance development in enterovirus A71 in the context of combination antiviral treatment. ACS Infect. Dis. 7 (10), 2801–2806. doi:10.1021/acsinfecdis.0c00872
Li, R., Liu, L., Mo, Z., Wang, X., Xia, J., Liang, Z., et al. (2014). An inactivated enterovirus 71 vaccine in healthy children. N. Engl. J. Med. 370, 829–837. doi:10.1056/NEJMoa1303224
Liang, Z., and Wang, J. (2014). EV71 vaccine, an invaluable gift for children. Clin. Transl. Immunol. 3 (10), e28. doi:10.1038/cti.2014.24
Lim, H., In, H. J., Lee, J. A., Sik, Y. J., Lee, S. W., Chung, G. T., et al. (2018). The immunogenicity and protection effect of an inactivated coxsackievirus A6, A10, and A16 vaccine against hand, foot, and mouth disease. Vaccine 36 (24), 3445–3452. doi:10.1016/j.vaccine.2018.05.005
Loddo, B. (1980). Development of drug resistance and dependence in viruses. Pharm. Ther. 10, 431–460. doi:10.1016/0163-7258(80)90026-1
Loddo, B., Ferraru, W., Spanneda, A., and Brotzu, G. (1962). In vitro guanidino-resistance and guanidino-dependence of poliovirus. Experientia 18, 518–519. doi:10.1007/BF02151608
Mouton, J. W. (1999). Combination therapy as a tool to prevent emergence of bacterial resistance. Infction. 27 (2), S24–S28. doi:10.1007/BF02561666
Nikolaeva, L., and Galabov, A. S. (1999). In vitro inhibitory effects of dual combinations of picornavirus replication inhibitors. Acta virol. 43, 303–311. PMID: 10757231.
Nikolaeva, L., and Galabov, A. S. (2000). Antiviral effect of the combination of enviroxime and disoxaril on coxsackievirus B1 infection. Acta virol. 44, 73–78. PMID: 10989697.
Nikolaeva-Glomb, L., and Galabov, A. S. (2004). Synergistic drug combinations against the in vitro replication of Coxackie B1 virus. Antivir. Res. 16, 41–46. doi:10.1016/j.antiviral.2003.11.001
Nikolaeva-Glomb, L., Stoyanova, A., Meodieva, A., and Galabov, A. S. (2011). “Antiviral effect of oxoglaucine in combination with some enterovirus replication inhibitors,” in Proceedings of the Twenty-fourth International Conference on Antiviral Research, Sofia, Bulgaria, 2011 May 8-11 (Antiviral Research) 90, A61. abstract 133. doi:10.1016/j.antiviral.2011.03.119
Pevear, D. C., Tull, T. M., Seipel, M. E., and Groarke, J. M. (1999). Activity of pleconaril against enteroviruses. Antimicrob. Agents. Chem. 43 (9), 2109–2115. doi:10.1128/aac.43.9.2109
Prichard, M. N., Aseltine, K. R., and Shipman, C. (1993). MacSynergyTM II (version 1.0) User’s Manual. Ann Arbor, MI: University of Michigan.
Prichard, M. N., Aseltine, K. R., and Shipman, Jr.C. (1992). MacSynergyTM II. Ann Arbor, MI: University of Michigan.
Prichard, M. N., and Shipman, C. (1990). A three-dimensional model to analyze drug-drug interaction. Antivir. Res. 14, 181–206. doi:10.1016/0166-3542(90)90001-n
Rotbart, H. A. (2002). Treatment of picornavirus infections. Antivir. Res. 53, 83–98. doi:10.1016/S0166-3542(01)00206-6
Stoyanova, A., and Galabov, A. S. (2020). Effect of consecutive alternating administration of a triple combination of anti-enteroviral compounds in mice infected with coxsackievirus B3. Pathogens Dis. 78, ftaa065. doi:10.1093/femspd/ftaa065
Stoyanova, A., and Galabov, A. S. (2021a). Effects of double combinations of enterovirus replication inhibitors against Coxsackie B viruses. Acta virol. 65, 411–419. doi:10.4149/av_2021_410
Stoyanova, A., and Galabov, A. S. (2021b). Consecutive alternating administration as an effective anti-coxsackievirus B3 in vivo treatment scheme. Archives Virology 166, 1869–1875. doi:10.1007/s00705-021-05057-3
Tammaro, C., Guida, M., Appetecchia, F., Biava, M., Consalvi, S., and Poce, G. (2023). Direct-acting antivirals and host-targeting approaches against enterovirus B infections: recent advances. Pharm. (Basel) 16 (2), 203. doi:10.3390/ph16020203
Tamura, K., Kohnoe, M., Takashino, A., Kobayashi, K., Koike, S., Karwal, L., et al. (2022). TAK − 021, an inactivated Enterovirus 71 vaccine candidate, provides cross-protection against heterologous sub-genogroups in human scavenger receptor B2 transgenic mice. Vaccine 40 (24), 3330–3337. doi:10.1016/j.vaccine.2022.04.064
Thibaut, H. J., Leyssen, P., Puerstinger, G., Muigg, A., Neyts, J., and De Palma, A. M. (2011). Towards the design of combination therapy for the treatment of enterovirus infections. Antivir. Res. 90 (3), 213–217. doi:10.1016/j.antiviral.2011.03.187
Wang, Y., Li, G., Yan, S., Gao, Q., Lan, K., Altmeyer, R., et al. (2016). In vitro assessment of combinations of enterovirus inhibitors against enterovirus 71. Antimicrob. Agents Chemother. 60 (9), 5357–5367. doi:10.1128/AAC.01073-16
Wittekind, S. G., Allen, C. C., Jefferies, J. L., Rattan, M. S., Madueme, P. C., Taylor, B. N., et al. (2017). Neonatal enterovirus myocarditis with severe dystrophic calcification: novel treatment with pocapavir. J. Investig. Med. High. Impact. Case. Rep. 14 (3), 232470961772939. doi:10.1177/2324709617729393
Zhang, D., Lu, J., and Lu, J. (2010). Enterovirus 71 vaccine: Close but still far. Int. J. Infect. Dis. 14 (9), e739–e743. doi:10.1016/j.ijid.2009.12.002
Keywords: pocapavir, double combination, CV-B4, CV-A9, PV-1
Citation: Stoyanova A, Galabov S and Galabov AS (2024) Antiviral activity in vitro of double combinations of enteroviral inhibitors. Acta Virol. 68:12361. doi: 10.3389/av.2024.12361
Received: 02 November 2023; Accepted: 26 April 2024;
Published: 15 May 2024.
Edited by:
Daniel Ruzek, Masaryk University, CzechiaReviewed by:
Ludek Eyer, Veterinary Research Institute (VRI), CzechiaJan Weber, Academy of Sciences of the Czech Republic (ASCR), Czechia
Copyright © 2024 Stoyanova, Galabov and Galabov. This is an open-access article distributed under the terms of the Creative Commons Attribution License (CC BY). The use, distribution or reproduction in other forums is permitted, provided the original author(s) and the copyright owner(s) are credited and that the original publication in this journal is cited, in accordance with accepted academic practice. No use, distribution or reproduction is permitted which does not comply with these terms.
*Correspondence: Adelina Stoyanova, YWRlbGluYXN0b3lhbm92YUBhYnYuYmc=; Angel S. Galabov, Z2FsYWJvdkBtaWNyb2Jpby5iYXMuYmc=