- 1Center for Transplantation Sciences, Department of Surgery, Massachusetts General Hospital, Harvard Medical School, Boston, MA, United States
- 2The Transplantation Institute at the Second Affiliated Hospital of Hainan Medical University, Haikou, Hainan, China
An overview is provided of the evolution of strategies towards xenotransplantation during the past almost 40 years, focusing on advances in gene-editing of the organ-source pigs, pre-transplant treatment of the recipient, immunosuppressive protocols, and adjunctive therapy. Despite initial challenges, including hyperacute rejection resulting from natural (preformed) antibody binding and complement activation, significant progress has been made through gene editing of the organ-source pigs and refinement of immunosuppressive regimens. Major steps were the identification and deletion of expression of the three known glycan xenoantigens on pig vascular endothelial cells, the transgenic expression of human “protective” proteins, e.g., complement-regulatory, coagulation-regulatory, and anti-inflammatory proteins, and the administration of an immunosuppressive regimen based on blockade of the CD40/CD154 T cell co-stimulation pathway. Efforts to address systemic inflammation followed. The synergy between gene editing and judicious immunomodulation appears to largely prevent graft rejection and is associated with a relatively good safety profile. Though there remains an incidence of severe or persistent proteinuria (nephrotic syndrome) in a minority of cases. This progress offers renewed hope for patients in need of life-saving organ transplants.
Introduction
Despite the great progress that has been made in the gene-editing of pigs that are the sources of organs or cells for xenotransplantation in nonhuman primate (NHP) or human recipients, there remains a need for the administration of exogenous immunosuppressive therapy to the recipient of a pig xenograft [1]. Increased gene-editing of the organ-source pigs [2, 3] and the introduction of new agents that are more effective in suppressing the human immune response are both key factors that have allowed changes to be made to the immunosuppressive regimen to prevent rejection.
We here briefly review the changes in pre-transplant treatment, immunosuppressive protocols, and adjunctive therapy that have been made during the past almost 40 years of pig-to-NHP heart or kidney transplantation with some based on concomitant in vitro studies [4]. These observations are made largely through the experience of one group but that of several other groups has also been reviewed.
The “Conventional” Immunosuppressive Therapy Era
By the 1980s, it was known that natural (preformed) antibodies, when bound to antigens on a discordant animal organ graft, could activate complement, resulting in hyperacute rejection (defined as rejection occurring within 24 h) [5–10]. There was evidence that natural antibodies developed as a defense mechanism when the gastrointestinal tract of infants became colonized by microorganisms that expressed carbohydrate antigens, e.g., galactose-α1,3-galactose (Gal), that were also expressed on pig cells (Table 1) [11, 12].
Antibody Depletion
When xenotransplantation was first explored in wild-type (i.e., genetically-unmodified) pig-to-NHP models in the 1980s [13, 14], cyclosporine had become available, but tacrolimus was not yet accessible to most groups. Initial studies were therefore based on the regimens used in clinical allotransplantation, i.e., cyclosporine with added steroids with or without azathioprine or mycophenolate mofetil (MMF) (Table 2) [14]. The results were extremely disappointing and, with graft survival still measured in minutes, hours, or a few days, the administration of a cyclosporine-based regimen made little difference to the outcome. The innate immune response was clearly very strong and the effect of cyclosporine in suppressing the adaptive immune response was very modest (in contrast to its effect in allotransplantation).
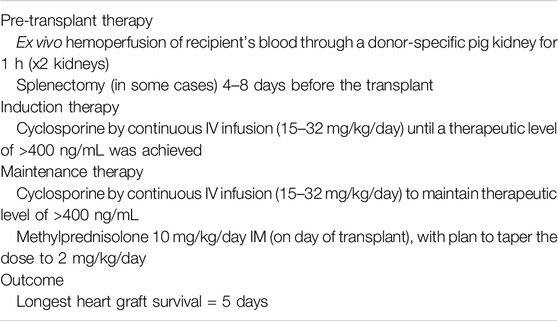
Table 2. Representative immunosuppressive regimen administered in the wild-type pig-to-NHP heterotopic heart Tx model [14].
As pre-transplant splenectomy was thought to be beneficial in allotransplantation across the ABO blood group barrier [15, 16], it was believed that it might also reduce the immune response to a pig graft, probably by removing a major source of B cells in the host as well as by decreasing the number of lymphocytes and their proliferative responses [17, 18]. With time, however, evidence for this was lacking and, possibly after the addition of rituximab to the protocol, it was eventually omitted from the regimen.
An effort was made to deplete the potential recipient of anti-pig antibodies either by 1) plasmapheresis [17], which extended graft survival to a maximum of 23 days, though usually for a shorter period of time, or by 2) preliminary perfusion of the recipient blood through a donor-specific second organ, e.g., the kidney, before donor-specific heart transplantation [13, 14], but graft survival remained very limited.
When Gal was clearly identified as the major target for human preformed anti-pig antibodies (Table 1) [19–23], techniques of antibody depletion were explored in vitro [24, 25] and refined to allow removal or “neutralization” of only anti-Gal antibodies, thus not depleting the NHP of antibodies that might be important in protecting from infectious complications. Again based on experience in overcoming the barrier of ABO-incompatibility, anti-pig antibody immunoadsorption was achieved by 1) perfusion of the recipient’s blood through an immunoaffinity column of synthetic Gal oligosaccharides [20, 26–33] or 2) the continuous intravenous infusion of soluble synthetic Gal oligosaccharides [33–36].
In this latter approach, the infused synthetic Gal oligosaccharides would be bound by the circulating anti-Gal antibodies and therefore “neutralize” them by preventing them from binding to the pig graft. This approach had proven to be successful in preventing rejection of ABO-incompatible cardiac allografts in baboons [14]. Subsequently, modifications were made, e.g., synthetic Gal oligosaccharides were attached to bovine serum albumin which was then infused intravenously [37, 38]. All of these approaches prevented hyperacute rejection of the graft, but the return of antibody within days inevitably resulted in graft loss [39, 40].
In retrospect, the removal or neutralization of anti-Gal antibodies alone was probably misguided because, in addition to the early return of anti-Gal antibodies, there was already evidence of the presence of antibodies to non-Gal antigens [41–43] (identified as N-glycolylneuraminic acid [44] and Sda [45] [Table 1]). However, at the time, it was hoped that “accommodation” would develop [46] (i.e., when the return of antibody is no longer associated with rejection) as occurs in many patients receiving an ABO-incompatible allograft [47], but this proved not to be the case. The exact mechanism by which accommodation occurs remains uncertain. The additional differences in complement and coagulation factors between pig and human (see below) probably contributed to the difference in outcome between allograft and xenograft.
Protection From Complement Injury
Complement depletion or inhibition in the potential NHP recipient extended graft survival to a maximum of 25 days [48–52], but it was suspected that patients with no complement activity would be at risk for infectious complications and would not do well long-term and so this approach was not pursued (although prolonged complement inhibition has been adopted again by some groups recently [53]).
The introduction of the first gene-edited pigs by White and his colleagues at the British biotechnology company, Imutran, was a milestone in xenotransplantation research and enabled progress to be made [54]. These pigs expressed a single human complement-regulatory protein, CD55 (decay accelerating factor, DAF), and this alone extended kidney or heart graft survival in some immunosuppressed recipient NHPs for several weeks – in one case for up to 3 months [55]. However, the transplantation of hCD55 transgenic pig grafts proved successful only if intensive cyclosporine-based immunosuppressive regimens were employed (Table 3) [56]. For example, induction therapy with cyclophosphamide was found to be beneficial or even essential. It was later confirmed that the expression of a human complement-regulatory protein helps protect the graft from systemic complement activation in the host [57].
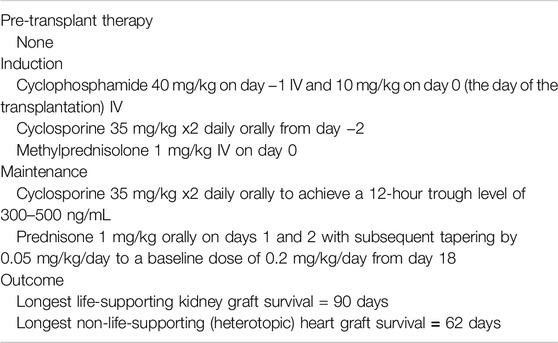
Table 3. Representative immunosuppressive regimen administered in the hDAF (CD55) transgenic pig-to-NHP heterotopic heart and kidney Tx models [55].
Depletion of complement for a prolonged period of time was, and still is, considered to put the patient at increased risk of infectious complications. Once the transgenic expression of human complement-regulatory proteins could be induced in the organ-source pig, systemic complement inhibition was avoided by most groups. However, transient systemic complement inhibition at the time of pig organ transplantation, when there is complement activation and inflammation, may be beneficial [58]. When introduced by Langin et al [59], the administration of a C1-esterase inhibitor on just 2 days appears to be safe and beneficial, even though it has not been conclusively demonstrated to be essential. Long-term complement inhibition, e.g., with a C5 inhibitor, has been incorporated into the immunosuppressive regimen by some groups [53], but its necessity remains controversial.
The Introduction of CD40/CD154 T Cell Co-Stimulation Pathway Blockade
Based on encouraging studies in models of allotransplantation, in 2,000 Buhler et al. carried out pig hematopoietic cell transplantation in an attempt to induce chimerism as a basis for achieving immunological tolerance to a pig organ in an immunosuppressed NHP [60, 61]. When immunosuppressive therapy was based on cyclosporine, an elicited antibody response to the pig cells was clearly detected within the first 14 post-transplant days. In contrast, treatment with an anti-CD154 monoclonal antibody (mAb) prevented this antibody response [60] (Blockade of the B7/CD28 pathway did not prove equally successful) [62–65]. This proved a major step forward. Since then, almost all groups have employed an anti-CD154 or anti-CD40mAb as the basis of their immunosuppressive regimen [53, 66–77].
Yamamoto et al. subsequently demonstrated prolonged survival of kidney grafts from α1,3-galactosyltransferase gene-knockout (GTKO) pigs (in which the most important xenoantigen, galactose-α1,3-galactose [Gal] against which humans and NHPs have natural antibodies had been deleted [78–80] in NHPs receiving a CD40/CD154 co-stimulation-based inhibitory regimen compared to NHPs receiving a conventional (tacrolimus-based) immunosuppressive regimen (Figure 1) [81]. The administration of an anti-CD154mAb has been associated with better results when compared with an anti-CD40mAb [74]. In summary, by modulating the immune response between T cells and antigen-presenting cells, inhibiting co-stimulatory pathways improves long-term post-transplant outcomes.
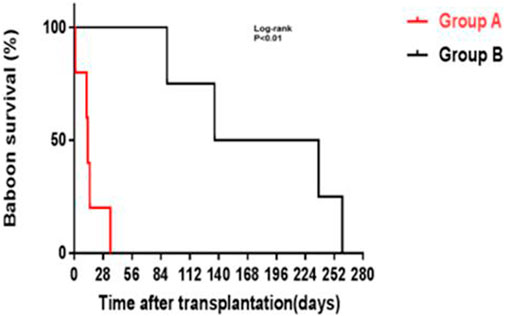
Figure 1. GTKO pig kidney survival in baboons receiving US FDA-approved immunosuppressive agents (Group A, in red) was much shorter than in those receiving an anti-CD40mAb-based regimen (Group B, in black) outlined in Table 6 (Reproduced with permission from Yamamoto T, et al. [81]).
When attempting to induce tolerance to an allotransplant, at that time the potential recipient was pre-treated with whole body and thymic irradiation. However, in the pig-to-NHP model, survival of the graft rather than tolerance induction was the major aim, and so whole body irradiation was deemed unnecessary, although thymic irradiation was still carried out for a period of time (Table 4).
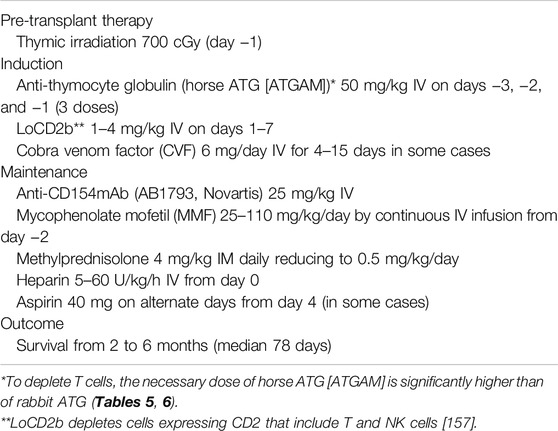
Table 4. Representative immunosuppressive regimen administered in the GTKO pig-to-NHP heterotopic heart Tx model [82].
Nevertheless, induction therapy was still considered essential. Initially, this consisted of large doses of anti-thymocyte globulin and other agents that depleted T cells (Table 4). With the transplantation of organs from pigs expressing fewer xenoantigens and a greater number of human protective transgenes it was determined that the dose of ATG could be reduced. The effect of the ATG can be determined simply by following the total lymphocyte count.
However, additional B cell depletion was considered beneficial and so the administration of an anti-CD20mAb (in the form of Rituximab) was included, initially by McGregor et al [83]. Whether this is essential remains uncertain but a significant reduction in B cells in the blood for several weeks (Figure 2) may possibly result in a subsequent reduction in plasma cells, though this has not been proven.
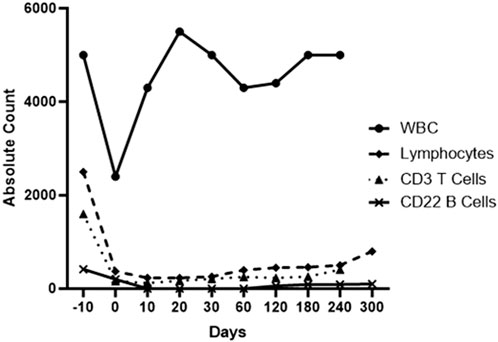
Figure 2. Total white blood cell, lymphocyte, T cell, and B cell counts in the blood of a baboon with a life-supporting pig kidney that received the immunosuppressive regimen outlined in Table 6.
The initial anti-CD154mAbs were tested in vitro and in vivo [75, 84–86]. When transplants were still being carried out with organs from wild-type pigs, the results remained disappointing because the innate response remained strong. When GTKO pigs became available, however, the results improved markedly. The first series that combined the transplantation of heterotopically-placed hearts (i.e., not life-supporting) from GTKO pigs into baboons with immunosuppression based on CD40/CD154 co-stimulation pathway blockade demonstrated greatly improved graft survival, extending to a maximum of 6 months (Table 4) [82, 87]. However, the recipient baboons were selected on the basis of their low anti-pig antibody levels.
Using an identical immunosuppressive regimen, life-supporting GTKO kidney grafts survived approximately only half as long [88], possibly because 1) kidneys may be more immunogenic than hearts, or 2) the kidneys were life-supporting whereas the hearts were not. When GTKO pig kidneys were transplanted into NHPs immunosuppressed with a tacrolimus-based regimen, the results were markedly inferior [89].
The transplantation of GTKO hearts that expressed a different human complement-regulatory protein, CD46, with the same or very similar immunosuppressive regimen reduced early graft failure but did not extend maximum graft survival [90, 91].
The withdrawal of the original anti-CD154mAbs because of their thrombogenic effect [92–94] necessitated the use of anti-CD40mAbs, first introduced into xenotransplantation by Mohiuddin et al [68–70]. However, increasing data indicate that anti-CD154 agents are superior to anti-CD40 agents in preventing both the adaptive immune response and some aspects of the innate response [75, 95]. Once Fc-modified anti-CD154 agents (that do not result in platelet activation) were introduced [74, 75, 96–98], these soon became the treatment of choice [74, 76, 77].
One important observation made in regard to anti-CD154mAb therapy was that in infant baboons in which natural antibodies had not yet developed, treatment with an anti-CD154mAb prevented the development of natural anti-Gal and anti-AB antibodies, suggesting that natural antibodies may be, at least in part, T cell-dependent [99]. This has considerable relevance to the treatment of neonates with complex, life-threatening congenital heart disease, e.g., single ventricle physiology, by pig heart xenotransplantation [100, 101]. For example, by inhibiting both natural and elicited antibody production, treatment with an anti-CD154mAb during the first week of life (at the time of pig heart transplantation) might possibly facilitate the development of immunological tolerance to the graft. Once the graft has been established, it may be possible to discontinue all immunosuppressive therapy.
Despite the suppressive effect of agents that block the CD40/CD154 co-stimulation pathway, the transplantation of kidneys or hearts from GTKO pigs was not consistently successful, with some grafts failing from antibody-mediated rejection [66, 82, 88, 102–105], coagulation dysfunction, or graft vasculopathy (chronic rejection) (Figure 3) [106].
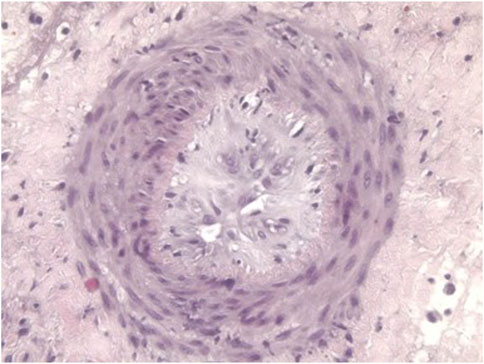
Figure 3. Histopathological features of graft vasculopathy (chronic rejection) In a GTKO pig heart transplanted heterotopically (in the abdomen) 3 months previously in a baboon that received the immunosuppressive regimen outlined in Table 4.
Protection From Coagulation Dysfunction
Predictions of significant differences in the coagulation-anticoagulation systems between pig and human had been discussed for some time [107, 108], but evidence in the important pig-to-NHP model was first reported in the late 1990s [84, 109–111] (Figure 4). Although the presence of porcine cytomegalovirus (CMV) in the graft was identified as playing a role in coagulation dysfunction [112], later confirmed by Yamada [113], this problem stimulated the need to introduce human coagulation-regulatory genes into the pig. Thrombomodulin, endothelial cell protein C receptor (EPCR), and/or tissue factor pathway inhibitor (TFPI) were expressed in the pig.
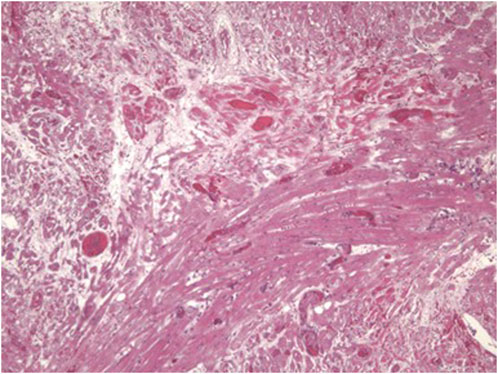
Figure 4. Histopathological features of a pig cardiac graft demonstrating multiple vascular thromboses with surrounding ischemic changes (fibrosis) in a baboon that received the immunosuppressive regimen outlined in Table 4.
When the problems relating to coagulation dysfunction between pig and human were confirmed, the introduction of GTKO. hCD46 pigs that additionally expressed human thrombomodulin reduced the incidence of thrombotic microangiopathy in the pig graft and of consumptive coagulopathy in the recipient NHPs (Table 5; Figure 5) [70]. This prolonged life-supporting kidney graft survival to 7–8 months, with termination of the experiments from infectious complications rather than from rejection [70].
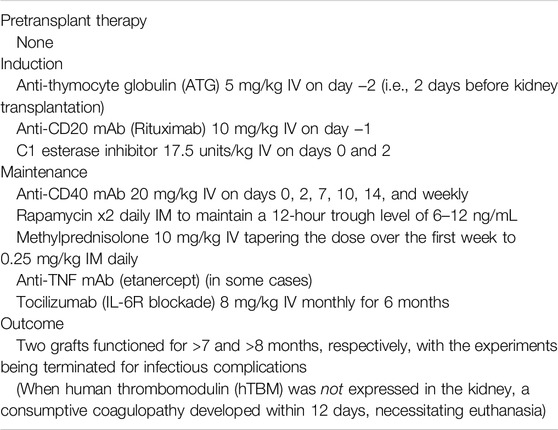
Table 5. Representative immunosuppressive regimen used in the GTKO/CD46/hTBM pig-to-NHP life-supporting kidney Tx model [70].
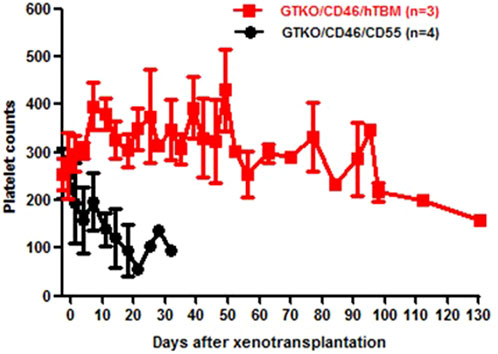
Figure 5. Platelet counts in baboons with hearts from GTKO.CD45.TBM (red) or GTKO.CD46.CD55 (black) pigs. The baboons received the immunosuppressive regimen outlined in Table 5.
Protection From Systemic Inflammation
Xenotransplantation was found to be accompanied by a systemic inflammatory response that could be detrimental to the survival of the graft by augmenting the immune response and/or coagulation dysfunction [62, 114–118]. Corticosteroids appeared to have no effect in suppressing this response.
However, in the period 2015-2020, the beneficial effects of interleukin-6 receptor (IL-6R) blockade using tocilizumab (which blocks IL-6 binding to the receptors on NHP cells but not on pig cells) were investigated [119–121]. Although the initial results were encouraging, the accompanying rise in IL-6 in the blood engendered some caution in the use of tocilizumab [120, 121]. With additional experience, we have tentatively concluded that this agent has a positive effect on graft survival [77]. It may be particularly beneficial when orthotopic pig heart transplantation is carried out because it may protect the recipient’s tissues, e.g., the lungs, from inflammatory injury associated in part to the need for cardiopulmonary bypass [118]. However, the combination of two inhibitors of IL-6 proved fatal from profound thrombocytopenia [122].
There is some evidence that the introduction of a human “anti-inflammatory” transgene into the pig, e.g., hemeoxygenase-1, A20, has a protective effect on the graft [3, 121, 123].
Low-dose corticosteroids have been used in almost all regimens (probably because they are included in the regimens of most patients with organ allografts) but there is little evidence that they are essential when co-stimulation blockade is employed, particularly if tocilizumab is being administered. As long ago as 2005, Yamada carried out one GTKO pig kidney transplant in a baboon without maintenance steroids and found no significant detrimental effect on graft survival [88]. Our suspicion is that steroids add little to the efficacy of the regimen, particularly if it includes tocilizumab.
Although not fully recognized in the early days of xenotransplantation research, inhibition of complement activity also has beneficial effects on coagulation dysfunction and the inflammatory response (see below) [58].
Additional Immunosuppressive Therapy and Adjunctive Therapy
Although blockade of the CD40/CD154 co-stimulation pathway has formed the basis of all effective regimens for the past two decades [60], its dosage is important. For example, dosing of the Tonix-1500 anti-CD154mAb at 20 mg/kg weekly, although effective in preventing rejection of allografts, was not entirely successful in regard to grafts from triple-knockout (TKO) pigs, in which expression of all three of the known pig xenoantigens against which humans have natural antibodies has been deleted (Table 1) [74]. A higher dosage, however, appears to be consistently successful without significant infectious complications [77] and Kinoshital et al. (unpublished data).
It must be remembered that all Old World NHPs have natural antibodies to TKO pig cells, thus increasing the hurdle that has to be overcome, i.e., the hurdle of “sensitization” (that will not be the case in many human patients receiving a pig xenograft) [124–127]. The results of TKO pig organ transplantation in NHPs are inferior to those of GTKO pig organ transplantation [71, 128], but remarkably CD40/CD154 co-stimulation pathway blockade appears to overcome this hurdle if recipient NHPs are selected with low anti-pig antibody levels (Table 6) [72, 77, 129].
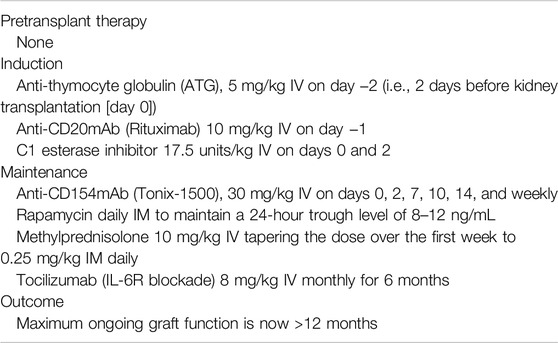
Table 6. Representative immunosuppressive regimen used in the TKO (+added transgenes) pig-to-NHP life-supporting kidney Tx model [77].
Whether other agents, if any, need to be combined with co-stimulation blockade remains uncertain. Pierson and Kawai and their respective colleagues have clearly demonstrated that in allotransplantation no other agents (either for induction or maintenance) may be necessary because anti-CD154mAb alone (in the form of Tonix-1500) prevents rejection almost consistently for at least 6 months (at which time the studies have been electively concluded) [97, 98]. Rejection develops only some weeks or months after cessation of treatment. There is some evidence, however, that the addition of low-dose rapamycin or tacrolimus to the regimen ensures an absence of rejection.
However, these agents alone are not so effective in xenotransplantation. For example, Tonix-1500 alone (with no induction therapy or additional maintenance therapy) was associated with antibody-mediated rejection of a kidney graft from a pig with 10 gene-edits on post-transplant day 4 [77]. This indicated to us that some form of induction therapy and additional maintenance therapy is required (Table 6).
When both T and B cells are depleted by this induction therapy (Table 6), the anti-CD40 or anti-CD154mAb maintenance therapy appears to maintain low lymphocyte counts throughout the first 6 months of the post-transplant period (Figure 2), which we suspect contributes to preventing an immune response to the graft [70, 77, 130].
To augment the effect of anti-CD40 or anti-CD154mAbs, we have selected rapamycin, in part because it can be administered intramuscularly, which is an advantage when managing NHPs that do not consistently take oral medications [131]. Mammalian target of rapamycin (mTOR) inhibitors have several properties that may be especially beneficial in xenotransplantation, e.g., suppression of T cell proliferation, increases in the number of T regulatory cells, inhibition of pig graft growth, and anti-inflammatory, anti-viral, and anti-cancer effects [132].
However, rapamycin is not tolerated by some patients (largely from gastrointestinal disturbances or oral ulcers) and so other pharmacologic immunosuppressive agents have been incorporated in several regimens. MMF has perhaps been the most commonly used agent [74, 76] but its value, as with rapamycin and tacrolimus, has not been proven. No group has yet had the courage to maintain immunosuppression with co-stimulation blockade alone. However, preliminary evidence that this may be possible was reported in one baboon when all immunosuppressive therapy, except anti-CD40mAb, was discontinued 2 months after pig kidney transplantation. During follow-up for a further 2 months, no clinical or histopathological features of rejection were observed [133]. Nevertheless, we have seen antibody-mediated rejection on occasions when the rapamycin level fell to subtherapeutic levels.
One important observation made recently is that, when proteinuria is present (which may be an early sign of antibody-mediated rejection), therapeutic mAbs may be lost in the urine, thus exposing the xenograft to rejection [77]. Furthermore, there is some evidence that an infectious microorganism in a xenograft (e.g., pyelonephritis) may induce an immune response, resulting in rapid rejection as has been well-documented in ABO-incompatible kidney allotransplantation [134, 135].
Comment
Throughout the early years covered by this brief report, researchers searched for other agents that might suppress the production of anti-pig antibodies. These agents included 1) various known immunosuppressive agents [30], 2) drugs used in other conditions but thought to have immunosuppressive properties [136–142], 3) new monoclonal antibodies directed towards depletion of plasma cells [143], 4) anti-idiotypic antibodies [144, 145], and 5) agents that influence expansion of T regulatory cells, but the majority proved unworthy or unnecessary of inclusion in the immunosuppressive regimen. An important observation was that prolonged treatment with bortezomib (a proteosome inhibitor) to patients who were highly sensitized to HLA had only a minimal effect in reducing anti-pig antibody levels [142].
Two agents that might well be valuable when xenotransplantation is introduced into the clinic are 1) atorvastatin [140, 141], whose anti-inflammatory effect could be of value. (As only tablets were available to us, we found it difficult to administer it successfully to NHPs.) and 2) alemtuzumab - but administering it to NHPs has several major limitations [146].
With the aim of protecting their cells from the adaptive immune response, gene-editing of the organ-source pigs has been explored. This included producing pigs that secreted CTLA4-Ig [147, 148] and pigs in which Major Histocompatibility Complex (MHC) Class II expression had been downregulated [149].
The level of CTLA4-Ig in the blood in pigs expressing CTLA4-Ig was approximately 10-fold higher than the therapeutic level in humans being treated with the agent [147]. Although this demonstrated the success of the gene-editing, the pigs were rendered immunocompromised and developed infections at a relatively young age for which they required euthanasia. This clearly precluded them from acting as sources of organs and from breeding. Furthermore, we concluded that maintaining the correct level of immunosuppression after organ transplantation in NHPs would also be difficult. However, others have successfully expressed CTLA4-Ig in the pancreatic islets [150, 151] although this limited expression may not be sufficient to protect against T cell-mediated rejection. MHC-Class II-knockdown was successful in reducing the T cell response and is worthy of further exploration [65, 149].
The currently available gene-edited pigs that are TKO and also express multiple human proteins [3, 76] would appear to be sufficient for clinical trials to be undertaken today. Future gene-editing may include the introduction of HLA-E and G [151], PD-L1 [152, 153], and MHC Class 1 knockout [154] or MHC Class II modification [155, 156].
Although gene-editing of pigs has been the major factor that has enabled progress to be made in pig organ xenotransplantation in NHPs, the introduction of immunosuppressive agents directed towards blockade of the CD40/CD154 co-stimulation pathway must not be underestimated. Successful clinical pig organ transplantation will require a combination of judicious gene-editing and the administration of an effective, but not excessive, immunosuppressive regimen. We suggest that selecting recipients with low levels of anti-pig IgM and preferably no IgG will contribute to success.
Author Contributions
The topic was suggested by DC and SS. All authors contributed to gathering relevant evidence and drafting the manuscript.
Funding
The author(s) declare financial support was received for the research, authorship, and/or publication of this article. Work on xenotransplantation in the authors’ laboratory is supported in part by NIH NIAID U19 grant AI090959 and in part by a Kidney X Prize from the US Department of Health and Human Services and the American Society of Nephrology.
Conflict of Interest
DC is a consultant to eGenesis Bio of Cambridge, MA, but the opinions expressed in this article are those of the authors, and do not necessarily reflect those of eGenesis.
The remaining authors declare that the research was conducted in the absence of any commercial or financial relationships that could be construed as a potential conflict of interest.
Generative AI Statement
The author(s) declare that no Generative AI was used in the creation of this manuscript.
Acknowledgments
The authors thank their many collaborators and research fellows (many of the Europeans) who contributed to this work over the past 40 years.
Abbreviations
ATG, anti-thymocyte globulin; Gal, galactose-α1,3-galactose; GTKO, α1,3-galactosyltransferase gene-knockout; mAb, monoclonal antibody; MMF, mycophenolate mofetil; NHP, nonhuman primate; TKO, triple-knockout (i.e., with deletion of expression of the three known pig carbohydrate xenoantigens against which humans have natural antibodies.).
References
1. Cooper, DKC, and Pierson, RN. Milestones on the Path to Clinical Pig Organ Xenotransplantation. Am J Transpl (2023) 23:326–35. doi:10.1016/j.ajt.2022.12.023
2. Cooper, DKC, Ezzelarab, M, Iwase, H, and Hara, H. Perspectives on the Optimal Genetically Engineered Pig in 2018 for Initial Clinical Trials of Kidney or Heart Xenotransplantation. Transplantation (2018) 102:1974–82. doi:10.1097/TP.0000000000002443
3. Cooper, DKC, Hara, H, Iwase, H, Yamamoto, T, Li, Q, Ezzelarab, M, et al. Justification of Specific Genetic Modifications in Pigs for Clinical Organ Xenotransplantation. Xenotransplantation (2019) 26:e12516. doi:10.1111/xen.12516
4. Hara, H, Yamamoto, T, Wei, H-J, and Cooper, DKC. What Have We Learned From In Vitro Studies About Pig-to-Primate Organ Transplantation? Transplantation (2023) 107:1265–77. doi:10.1097/TP.0000000000004458
5. Perper, RJ, and Najarian, JS. Experimental Renal Heterotransplantation: I. In Widely Divergent Species. Transplantation (1966) 4(4):377–88. doi:10.1097/00007890-196607000-00002
6. Mozes, MF, Shons, AR, Harris, NS, Merino, GE, Moberg, AW, Campos, RA, et al. Specificity of the Heteroantibody in Xenograft Rejection. In: Surg Forum (1971). p. 244–6.
7. Moberg, AW, Shons, AR, Gewurz, H, Mozes, M, and Najarian, JS. Prolongation of Renal Xenografts by the Simultaneous Sequestration of Preformed Antibody, Inhibition of Complement, Coagulation and Antibody Synthesis. Transpl Proc (1971) 3:538–41.
8. hons, AR, Kromrey, C, and Najarian, JS. Heterophile Antibodies in Man. Eur Surg Res (1973) 5:26–36. doi:10.1159/000127637
9. Shons, AR, and Najarian, JS. Modification of Xenograft Rejection by Aspirin, Dextran, and Cinanserin: The Importance of Platelets in Hyperacute Rejection. Transpl Proc (1974) 6:435–40.
11. Damian, RT. Molecular Mimicry: Antigen Sharing by Parasite and Host and Its Consequences. Am Nat (1964) 98:129–49. doi:10.1086/282313
12. Galili, U, Mandrell, RE, Hamadeh, RM, Shohet, SB, and Griffiss, JM. Interaction between Human Natural Anti-Alpha-Galactosyl Immunoglobulin G and Bacteria of the Human Flora. Infect Immun (1988) 56:1730–7. doi:10.1128/iai.56.7.1730-1737.1988
13. Lexer, G, Cooper, DKC, Rose, AG, Wicomb, WN, Rees, J, Keraan, M, et al. Hyperacute Rejection in a Discordant (Pig to Baboon) Cardiac Xenograft Model. J Heart Transpl (1986) 5:411–8.
14. Cooper, DKC, Human, PA, Lexer, G, Rose, AG, Rees, J, Keraan, M, et al. Effects of Cyclosporine and Antibody Adsorption on Pig Cardiac Xenograft Survival in the Baboon. J Heart Transpl (1988) 7:238–46.
15. Alexander, GPJ, De Bruyere, M, Squifflet, JP, Moriau, M, Latinne, D, and Pirson, Y. Human ABO-Incompatible Living Donor Renal Homografts. Neth J Med (1985) 28:231–4.
16. Alexander, GPJ, Alexandre, GP, Squifflet, JP, De Bruyère, M, Latinne, D, Reding, R, et al. Present Experiences in a Series of 26 ABO-Incompatible Living Donor Renal Allografts. Transpl Proc (1987) 19:4538–42.
17. Alexandre, GPJ, Gianello, P, Latinne, D, Carlier, M, Dewaele, A, Van Obbergh, L, et al. Plasmapheresis and Splenectomy in Experimental Renal Xenotransplantation. Xenograft (1989) 25:259.
18. Eibl, M. Immunological Consequences of Splenectomy. In: Gastro-Esophageal Reflux in Childhood Problems of Splenic Surgery in Childhood (Berlin, Heidelberg: Springer) (1985). p. 139–45.
19. Good, AH, Cooper, DKC, Malcolm, AJ, Ippolito, RM, Koren, E, Neethling, FA, et al. Identification of Carbohydrate Structures That Bind Human Antiporcine Antibodies: Implications for Discordant Xenografting in Humans. Transpl Proc (1992) 24(2):559–62.
20. Cooper, DKC. Depletion of Natural Antibodies in Non-Human Primates--A Step Towards Successful Discordant Xenografting in Humans. Clin Transpl (1992) 6:178–83. doi:10.1111/j.1399-0012.1992.tb00614.x
21. Cooper, DKC, Good, AH, Koren, E, Oriol, R, Malcolm, AJ, Ippolito, RM, et al. Identification of Alpha-Galactosyl and Other Carbohydrate Epitopes That Are Bound by Human Anti-Pig Antibodies: Relevance to Discordant Xenografting in Man. Transpl Immunol (1993) 1:198–205. doi:10.1016/0966-3274(93)90047-c
22. Cooper, DKC, Koren, E, and Oriol, R. Oligosaccharides and Discordant Xenotransplantation. Immunol Rev (1994) 141:31–58. doi:10.1111/j.1600-065x.1994.tb00871.x
23. Cooper, DKC. Modifying the Sugar Icing on the Transplantation Cake. Glycobiology (2016) 26:571–81. doi:10.1093/glycob/cww028
24. Neethling, FA, Joziasse, D, Bovin, N, Cooper, DKC, and Oriol, R. The Reducing End of Alpha Gal Oligosaccharides Contributes to Their Efficiency in Blocking Natural Antibodies of Human and Baboon Sera. Transpl Int (1996) 9:98–101. doi:10.1007/BF00336385
25. Neethling, FA, Koren, E, Ye, Y, Richards, SV, Kujundzic, M, Oriol, R, et al. Protection of Pig Kidney (PK15) Cells From the Cytotoxic Effect of Anti-Pig Antibodies by Alpha-Galactosyl Oligosaccharides. Transplantation (1994) 57:959–63. doi:10.1097/00007890-199403270-00032
26. Rieben, R, von Allmen, E, Korchagina, EY, Nydegger, UE, Neethling, FA, Kujundzic, M, et al. Detection, Immunoabsorption, and Inhibition of Cytotoxic Activity of Anti-αGal Antibodies Using Newly Developed Substances With Synthetic Gal α1–3Gal Disaccharide Epitopes. Xenotransplantation (1995) 2:98–106. doi:10.1111/j.1399-3089.1995.tb00072.x
27. Oriol, R, Neethling, F, Joziasse, D, Bovin, N, and Cooper, DKC. Efficiency of Different Synthetic Oligosaccharides for Blocking the Natural Anti-g Gal Antibodies Found in Human and Baboon Sera. Transpl Proc (1996) 28(2):562.
28. Taniguchi, S, Neethling, FA, Korchagina, EY, Bovin, N, Ye, Y, Kobayashi, T, et al. In Vivo Immunoadsorption of Antipig Antibodies in Baboons Using a Specific Galα1-3Gal Column. Transplantation (1996) 62:1379–84. doi:10.1097/00007890-199611270-00001
29. Kozlowski, T, Ierino, FL, Lambrigts, D, Foley, A, Andrews, D, Awwad, M, et al. Depletion of Anti-Galα1–3Gal Antibody in Baboons by Specific α-Gal Immunoaffinity Columns. Xenotransplantation (1998) 5:122–31. doi:10.1111/j.1399-3089.1998.tb00018.x
30. Lambrigts, D, Van Calster, P, Xu, Y, Awwad, M, Neethling, FA, Kozlowski, T, et al. Pharmacologic Immunosuppressive Therapy and Extracorporeal Immunoadsorption in the Suppression of anti-αGal Antibody in the Baboon. Xenotransplantation (1998) 5:274–83. doi:10.1111/j.1399-3089.1998.tb00038.x
31. Xu, Y, Lorf, T, Sablinski, T, Gianello, P, Bailin, M, Monroy, R, et al. Removal of Anti-Porcine Natural Antibodies From Human and Nonhuman Primate Plasma In Vitro and In Vivo by a Galα1-3Galβ1-4βGlc-X Immunoaffinity Column. Transplantation (1998) 65:172–9. doi:10.1097/00007890-199801270-00005
32. Watts, A, Foley, A, Awwad, M, Treter, S, Oravec, G, Buhler, L, et al. Plasma Perfusion by Apheresis Through a Gal Immunoaffinity Column Successfully Depletes Anti-Gal Antibody: Experience With 320 Aphereses in Baboons. Xenotransplantation (2000) 7:181–5. doi:10.1034/j.1399-3089.2000.00068.x
33. Teranishi, K, Alwayn, IPJ, Bühler, L, Gollackner, B, Knosalla, C, Huck, J, et al. Depletion of Anti-Gal Antibodies by the Intravenous Infusion of Gal Type 2 and 6 Glycoconjugates in Baboons. Xenotransplantation (2003) 10:357–67. doi:10.1034/j.1399-3089.2003.02078.x
34. Ye, Y, Neethling, FA, Niekrasz, M, Koren, E, Richards, SV, Martin, M, et al. Evidence That Intravenously Administered α-Galactosyl Carbohydrates Reduce Baboon Serum Cytotoxicity to Pig Kidney Cells (PK15) and Transplanted Pig Hearts. Transplantation (1994) 58:330–6. doi:10.1097/00007890-199408000-00014
35. Simon, PM, Neethling, FA, Taniguchi, S, Goode, PL, Zopf, D, Hancock, WW, et al. Intravenous Infusion of Galα1-3Gal Oligosaccharides in Baboons Delays Hyperacute Rejection of Porcine Heart Xenografts. Transplantation (1998) 65:346–53.
36. Kuwaki, K, Knosalla, C, Moran, K, Alt, A, Katopodis, AG, Duthaler, RO, et al. Reduction of Anti-Galα1, 3Gal Antibodies by Infusion of Types 2 and 6 Gal Trisaccharides Conjugated to Poly-l-Lysine. Xenotransplantation (2004) 11:210–5. doi:10.1046/j.1399-3089.2003.00096.x
37. Teranishi, K, Gollackner, B, Bühler, L, Knosalla, C, Correa, L, Down, JD, et al. Depletion of Anti-Gal Antibodies in Baboons by Intravenous Therapy With Bovine Serum Albumin Conjugated to Gal Oligosaccharides. Transplantation (2002) 73:129–39. doi:10.1097/00007890-200201150-00024
38. Gollackner, B, Knosalla, C, Houser, S, Mauiyyedi, S, Buhler, L, Kawai, T, et al. Pig Kidney Transplantation in Baboons Treated Intravenously With a Bovine Serum Albumin-Galα1-3Gal Conjugate. Xenotransplantation (2003) 10:606–14. doi:10.1034/j.1399-3089.2003.00065.x
39. Rose, AG, and Cooper, DKC. A Histopathologic Grading System of Hyperacute (Humoral, Antibody-Mediated) Cardiac Xenograft and Allograft Rejection. J Heart Lung Transpl (1996) 15:804–17.
40. Rose, AG, Cooper, DKC, Human, PA, Reichenspurner, H, and Reichart, B. Histopathology of Hyperacute Rejection of the Heart: Experimental and Clinical Observations in Allografts and Xenografts. J Heart Lung Transpl (1991) 10:223–34.
41. Rood, PPM, Hara, H, Ezzelarab, M, Busch, J, Zhu, X, Ibrahim, Z, et al. Preformed Antibodies to α1, 3-Galactosyltransferase Gene-Knockout (GT-KO) Pig Cells in Humans, Baboons, and Monkeys: Implications for Xenotransplantation. Transpl Proc (2005) 8:3514–5. doi:10.1016/j.transproceed.2005.09.082
42. Ezzelarab, M, Hara, H, Busch, J, Rood, PPM, Zhu, X, Ibrahim, Z, et al. Antibodies Directed to Pig Non-Gal Antigens in Naive and Sensitized Baboons. Xenotransplantation (2006) 13:400–7. doi:10.1111/j.1399-3089.2006.00320.x
43. Hara, H, Long, C, Lin, YJ, Tai, H, Ezzelarab, M, Ayares, D, et al. In Vitro Investigation of Pig Cells for Resistance to Human Antibody-Mediated Rejection. Transpl Int (2008) 21:1163–74. doi:10.1111/j.1432-2277.2008.00736.x
44. Bouhours, D, Pourcel, C, and Bouhours, J-F. Simultaneous Expression by Porcine Aorta Endothelial Cells of Glycosphingolipids Bearing the Major Epitope for Human Xenoreactive Antibodies (Gal Alpha 1-3Gal), Blood Group H Determinant and N-Glycolylneuraminic Acid. Glycoconj J (1996) 13:947–53. doi:10.1007/BF01053190
45. Byrne, GW, Du, Z, Stalboerger, P, Kogelberg, H, and McGregor, CGA. Cloning and Expression of Porcine β1,4 N-Acetylgalactosaminyl Transferase Encoding a New Xenoreactive Antigen. Xenotransplantation (2014) 21:543–54. doi:10.1111/xen.12124
46. Lynch, RJ, and Platt, JL. Accommodation in Organ Transplantation. Curr Opin Organ Transpl (2008) 13:165–70. doi:10.1097/MOT.0b013e3282f6391e
47. Urschel, S, and West, LJ. ABO-Incompatible Heart Transplantation. Curr Opin Pediatr (2016) 28:613–9. doi:10.1097/MOP.0000000000000398
48. Leventhal, JR, Dalmasso, AP, Cromwell, JW, Manivel, CJ, Bolman, RM, and Matas, AJ. Complement Depletion Prolongs Discordant Cardiac Xenograft Survival in Rodents and Non-Human Primates. Transpl Proc (1993) 25(1):398–9.
49. Leventhal, JR, Dalmasso, AP, Cromwell, JW, Platt, JL, Manivel, C, Bolman, RM, et al. Prolongation of Cardiac Xenograft Survival by Depletion of Complement. Transplantation (1993) 55:857–65. doi:10.1097/00007890-199304000-00033
50. Taniguchi, S, Kobayashi, T, Neethling, FA, Ye, Y, Niekrasz, M, White, DJG, et al. Cobra Venom Factor Stimulates Anti-α-Galactose Antibody Production in Baboons: Implications for Pig-To-Human Xenotransplantation. Transplantation (1996) 62:678–1. doi:10.1097/00007890-199609150-00024
51. Kobayashi, T, Neethling, FA, Taniguchi, S, Ye, Y, Niekrasz, M, Koren, E, et al. Investigation of the Anti-Complement Agents, FUT-175 and K76COOH, in Discordant Xenotransplantation. Xenotransplantation (1996) 3:237–45. doi:10.1111/j.1399-3089.1996.tb00143.x
52. Kobayashi, T, Taniguchi, S, Neethling, FA, Rose, AG, Hancock, WW, Ye, Y, et al. Delayed Xenograft Rejection of Pig-To-Babbon Cardiac Transplants After Cobra Venom Factor Therapy. Transplantation (1997) 64:1255–61. doi:10.1097/00007890-199711150-00005
53. Adams, AB, Lovasik, BP, Faber, DA, Burlak, C, Breeden, C, Estrada, JL, et al. Anti-C5 Antibody Tesidolumab Reduces Early Antibody-Mediated Rejection and Prolongs Survival in Renal Xenotransplantation. Ann Surg (2021) 274(3):473–80. doi:10.1097/SLA.0000000000004996
54. Cozzi, E, and White, DJG. The Generation of Transgenic Pigs as Potential Organ Donors for Humans. Nat Med (1995) 1:964–6. doi:10.1038/nm0995-964
55. Baldan, N, Rigotti, P, Calabrese, F, Cadrobbi, R, Dedja, A, Iacopetti, I, et al. Ureteral Stenosis in HDAF Pig-To-Primate Renal Xenotransplantation: A Phenomenon Related to Immunological Events? Am J Transpl (2004) 4:475–81. doi:10.1111/j.1600-6143.2004.00407.x
56. Lambrigts, D, Sachs, DH, and Cooper, DKC. Discordant Organ Xenotransplantation in Primates: World Experience and Current Status. Transplantation (1998) 66:547–61. doi:10.1097/00007890-199809150-00001
57. Jagdale, A, Nguyen, H, Li, J, Burnette, K, Ayares, D, Cooper, DKC, et al. Does Expression of a Human Complement-Regulatory Protein on Xenograft Cells Protect Them From Systemic Complement Activation? Int J Surg (2020) 83:184–8. doi:10.1016/j.ijsu.2020.09.034
58. Zhou, H, Hara, H, and Cooper, DKC. The Complex Functioning of the Complement System in Xenotransplantation. Xenotransplantation (2019) 26:e12517. doi:10.1111/xen.12517
59. Längin, M, Mayr, T, Reichart, B, Michel, S, Buchholz, S, Guethoff, S, et al. Consistent Success in Life-Supporting Porcine Cardiac Xenotransplantation. Nature (2018) 564:430–3. doi:10.1038/s41586-018-0765-z
60. Bühler, L, Awwad, M, Basker, M, Gojo, S, Watts, A, Treter, S, et al. High-Dose Porcine Hematopoietic Cell Transplantation Combined With Cd40 Ligand Blockade in Baboons Prevents an Induced Anti-Pig Humoral Response. Transplantation (2000) 69(11):2296–304. doi:10.1097/00007890-200006150-00013
61. Tseng, YL, Sachs, DH, and Cooper, DKC. Porcine Hematopoietic Progenitor Cell Transplantation in Nonhuman Primates: A Review of Progress. Transplantation (2005) 79:1–9. doi:10.1097/01.tp.0000146504.73727.13
62. Li, J, Hara, H, Wang, Y, Esmon, C, Cooper, DKC, and Iwase, H. Evidence for the Important Role of Inflammation in Xenotransplantation. J Inflamm (2019) 16:10–6. doi:10.1186/s12950-019-0213-3
63. Samy, KP, Butler, JR, Li, P, Cooper, DKC, and Ekser, B. The Role of Costimulation Blockade in Solid Organ and Islet Xenotransplantation. J Immunol Res (2017) 2017:8415205. doi:10.1155/2017/8415205
64. Iwase, H, Ekser, B, Satyananda, V, Bhama, J, Hara, H, Ezzelarab, M, et al. Pig-to-Baboon Heterotopic Heart Transplantation–Exploratory Preliminary Experience With Pigs Transgenic for Human Thrombomodulin and Comparison of Three Costimulation Blockade-Based Regimens. Xenotransplantation (2015) 22(3):211–20. doi:10.1111/xen.12167
65. Iwase, H, Ekser, B, Satyananda, V, Zhou, H, Hara, H, Bajona, P, et al. Initial In Vivo Experience of Pig Artery Patch Transplantation in Baboons Using Mutant MHC (CIITA-DN) Pigs. Transpl Immunol (2015) 32:99–108. doi:10.1016/j.trim.2015.02.003
66. Ezzelarab, M, Garcia, B, Azimzadeh, A, Sun, H, Lin, CC, Hara, H, et al. The Innate Immune Response and Activation of Coagulation in α1, 3-Galactosyltransferase Gene-Knockout Xenograft Recipients. Transplantation (2009) 87:805–12. doi:10.1097/TP.0b013e318199c34f
67. Ezzelarab, MB, Ekser, B, Echeverri, G, Hara, H, Ezzelarab, C, Long, C, et al. Costimulation Blockade in Pig Artery Patch Xenotransplantation–A Simple Model to Monitor the Adaptive Immune Response in Nonhuman Primates. Xenotransplantation (2012) 19:221–32. doi:10.1111/j.1399-3089.2012.00711.x
68. Mohiuddin, MM, Singh, AK, Corcoran, PC, Hoyt, RF, Thomas, ML, Lewis, BGT, et al. Role of Anti-CD40 Antibody-Mediated Costimulation Blockade on Non-Gal Antibody Production and Heterotopic Cardiac Xenograft Survival in a GTKO. hCD46Tg Pig-to-Baboon Model. Xenotransplantation (2014) 21:35–45. doi:10.1111/xen.12066
69. Iwase, H, Liu, H, Wijkstrom, M, Zhou, H, Singh, J, Hara, H, et al. Pig Kidney Graft Survival in a Baboon for 136 Days: Longest Life-Supporting Organ Graft Survival to Date. Xenotransplantation (2015) 22:302–9. doi:10.1111/xen.12174
70. Iwase, H, Hara, H, Ezzelarab, M, Li, T, Zhang, Z, Gao, B, et al. Immunological and Physiological Observations in Baboons With Life-Supporting Genetically Engineered Pig Kidney Grafts. Xenotransplantation (2017) 24:e12293. doi:10.1111/xen.12293
71. Iwase, H, Jagdale, A, Yamamoto, T, Bikhet, MH, Nguyen, HQ, Ezzelarab, M, et al. Evidence Suggesting that Deletion of Expression of N-Glycolylneuraminic Acid (Neu5Gc) in the Organ-Source Pig Is Associated With Increased Antibody-Mediated Rejection of Kidney Transplants in Baboons. Xenotransplantation (2021) 28:e12700. doi:10.1111/xen.12700
72. Higginbotham, L, Mathews, D, Breeden, CA, Song, M, Farris, AB, Larsen, CP, et al. Pre-Transplant Antibody Screening and Anti-CD154 Costimulation Blockade Promote Long-Term Xenograft Survival in a Pig-to-Primate Kidney Transplant Model. Xenotransplantation (2015) 22:221–30. doi:10.1111/xen.12166
73. Kim, SC, Mathews, DV, Breeden, CP, Higginbotham, LB, Ladowski, J, Martens, G, et al. Long-Term Survival of Pig-To-Rhesus Macaque Renal Xenografts Is Dependent on CD4 T Cell Depletion. Am J Transpl (2019) 19:2174–85. doi:10.1111/ajt.15329
74. Ma, D, Hirose, T, Lassiter, G, Sasaki, H, Rosales, I, Coe, TM, et al. Kidney Transplantation From Triple-Knockout Pigs Expressing Multiple Human Proteins in Cynomolgus Macaques. Am J Transpl (2022) 22:46–57. doi:10.1111/ajt.16780
75. Perrin, S, and Magill, M. The Inhibition of CD40/CD154 Costimulatory Signaling in the Prevention of Renal Transplant Rejection in Nonhuman Primates: A Systematic Review and Meta Analysis. Front Immunol (2022) 13:861471. doi:10.3389/fimmu.2022.861471
76. Anand, RP, Layer, JV, Heja, D, Hirose, T, Lassiter, G, Firl, DJ, et al. Design and Testing of a Humanized Porcine Donor for Xenotransplantation. Nature (2023) 622:393–401. doi:10.1038/s41586-023-06594-4
77. Kinoshita, K, Maenaka, A, Rosales, IA, Karadagi, A, Tomosugi, T, Ayares, D, et al. Novel Factors Potentially Initiating Acute Antibody-Mediated Rejection in Pig Kidney Xenografts Despite an Efficient Immunosuppressive Regimen. Xenotransplantation (2024) 31:e12859. doi:10.1111/xen.12859
78. Cooper, DKC, Koren, E, and Oriol, R. Genetically Engineered Pigs. Lancet (1993) 342:682–3. doi:10.1016/0140-6736(93)91791-j
79. Phelps, CJ, Koike, C, Vaught, TD, Boone, J, Wells, KD, Chen, SH, et al. Production of α1, 3-Galactosyltransferase-Deficient Pigs. Science (2003) 299:411–4. doi:10.1126/science.1078942
80. Kolber-Simonds, D, Lai, L, Watt, SR, Denaro, M, Arn, S, Augenstein, ML, et al. Production of α-1, 3-Galactosyltransferase Null Pigs by Means of Nuclear Transfer With Fibroblasts Bearing Loss of Heterozygosity Mutations. Proc Natl Acad Sci U S A (2004) 101:7335–40. doi:10.1073/pnas.0307819101
81. Yamamoto, T, Hara, H, Foote, J, Wang, L, Li, Q, Klein, EC, et al. Life-Supporting Kidney Xenotransplantation From Genetically Engineered Pigs in Baboons: A Comparison of Two Immunosuppressive Regimens. Transplantation (2019) 103:2090–104. doi:10.1097/TP.0000000000002796
82. Kuwaki, K, Tseng, YL, Dor, FJMF, Shimizu, A, Houser, SL, Sanderson, TM, et al. Heart Transplantation in Baboons Using Alpha1,3-Galactosyltransferase Gene-Knockout Pigs as Donors: Initial Experience. Nat Med (2005) 11:29–31. doi:10.1038/nm1171
83. McGregor, CGA, Davies, WR, Oi, K, Teotia, SS, Schirmer, JM, Risdahl, JM, et al. Cardiac Xenotransplantation: Recent Preclinical Progress With 3-Month Median Survival. J Thorac Cardiovasc Surg (2005) 130:844–51. doi:10.1016/j.jtcvs.2005.04.017
84. Bühler, L, Basker, M, Alwayn, IPJ, Goepfert, C, Kitamura, H, Kawai, T, et al. Coagulation and Thrombotic Disorders Associated With Pig Organ and Hematopoietic Cell Transplantation in Nonhuman Primates. Transplantation (2000) 70(9):1323–31. doi:10.1097/00007890-200011150-00010
85. Buhler, L, Yamada, K, Kitamura, H, Alwayn, IPJ, Basker, M, Appel, JZIII, et al. Pig Kidney Transplantation in Baboons: Anti-gal(alpha)1-3Gal IgM Alone Is Associated With Acute Humoral Xenograft Rejection and Disseminated Intravascular Coagulation. Transplantation (2001) 72(11):1743–52. doi:10.1097/00007890-200112150-00007
86. Kuwaki, K, Knosalla, C, Dor, FJMF, Gollackner, B, Tseng, YL, Houser, S, et al. Suppression of Natural and Elicited Antibodies in Pig-To-Baboon Heart Transplantation Using a Human Anti-Human CD154 mAb-Based Regimen. Am J Transpl (2004) 4:363–72. doi:10.1111/j.1600-6143.2004.00353.x
87. seng, YL, Kuwaki, K, Dor, FJMF, Shimizu, A, Houser, S, Hisashi, Y, et al. Alpha1,3-Galactosyltransferase Gene-Knockout Pig Heart Transplantation in Baboons With Survival Approaching 6 Months. Transplantation (2005) 80:1493–500. doi:10.1097/01.tp.0000181397.41143.fa
88. Yamada, K, Yazawa, K, Shimizu, A, Iwanaga, T, Hisashi, Y, Nuhn, M, et al. Marked Prolongation of Porcine Renal Xenograft Survival in Baboons Through the Use of α1, 3-Galactosyltransferase Gene-Knockout Donors and the Cotransplantation of Vascularized Thymic Tissue. Nat Med (2005) 11:32–4. doi:10.1038/nm1172
89. Chen, G, Qian, H, Starzl, T, Sun, H, Garcia, B, Wang, X, et al. Acute Rejection Is Associated With Antibodies to Non-gal Antigens in Baboons Using Gal-Knockout Pig Kidneys. Nat Med (2005) 11:1295–8. doi:10.1038/nm1330
90. McGregor, CGA, Ricci, D, Miyagi, N, Stalboerger, PG, Du, Z, Oehler, EA, et al. Human CD55 Expression Blocks Hyperacute Rejection and Restricts Complement Activation in Gal Knockout Cardiac Xenografts. Transplantation (2012) 93:686–92. doi:10.1097/TP.0b013e3182472850
91. Azimzadeh, AM, Kelishadi, SS, Ezzelarab, MB, Singh, AK, Stoddard, T, Iwase, H, et al. Early Graft Failure of GalTKO Pig Organs in Baboons Is Reduced by Expression of a Human Complement Pathway-Regulatory Protein. Xenotransplantation (2015) 22:310–6. doi:10.1111/xen.12176
92. Kawai, T, Andrews, D, Colvin, RB, Sachs, DH, and Cosimi, AB. Thromboembolic Complications After Treatment With Monoclonal Antibody Against CD40 Ligand. Nat Med (2000) 6:114. doi:10.1038/72162
93. Kirk, AD, Knechtle, SJ, Sollinger, HW, Vincenti, FG, Stecher, S, and Nadeau, K. Preliminary Results of the Use of Humanized Anti-CD154 in Human Renal Allotransplantation. Am J Transpl (2001) 1:191.
94. Knosalla, C, Gollackner, B, and Cooper, DKC. Anti-CD154 Monoclonal Antibody and Thromboembolism Revisted. Transplantation (2002) 74:416–7. doi:10.1097/00007890-200208150-00024
95. Liu, D, and Ford, ML. CD11b Is a Novel Alternate Receptor for CD154 During Alloimmunity. Am J Transpl (2020) 20:2216–25. doi:10.1111/ajt.15835
96. Shock, A, Burkly, L, Wakefield, I, Peters, C, Garber, E, Ferrant, J, et al. CDP7657, an Anti-CD40L Antibody Lacking an Fc Domain, Inhibits CD40L-Dependent Immune Responses Without Thrombotic Complications: An In Vivo Study. Arthritis Res Ther (2015) 17:234–12. doi:10.1186/s13075-015-0757-4
97. Miura, S, Habibabady, ZA, Pollok, F, Ma, M, Rosales, IA, Kinoshita, K, et al. TNX-1500, a Crystallizable Fragment–Modified Anti-cd154 Antibody, Prolongs Nonhuman Primate Cardiac Allograft Survival. Am J Transpl (2023) 23:1182–93. doi:10.1016/j.ajt.2023.03.025
98. Lassiter, G, Otsuka, R, Hirose, T, Rosales, IA, Karadagi, A, Tomosugi, T, et al. TNX-1500, a Crystallizable Fragment–Modified Anti-cd154 Antibody, Prolongs Nonhuman Primate Renal Allograft Survival. Am J Transpl (2023) 23:1171–81. doi:10.1016/j.ajt.2023.03.022
99. Dons, EM, Montoya, C, Long, CE, Hara, H, Echeverri, GJ, Ekser, B, et al. T-Cell-Based Immunosuppressive Therapy Inhibits the Development of Natural Antibodies in Infant Baboons. Transplantation (2012) 93:769–76. doi:10.1097/TP.0b013e3182481168
100. Li, Q, Hara, H, Banks, CA, Yamamoto, T, Ayares, D, Mauchley, DC, et al. Anti-Pig Antibody in Infants: Can a Genetically Engineered Pig Heart Bridge to Allotransplantation? Ann Thorac Surg (2020) 109:1268–73. doi:10.1016/j.athoracsur.2019.08.061
101. Cleveland, DC, Jagdale, A, Carlo, WF, Iwase, H, Crawford, J, Walcott, GP, et al. The Genetically Engineered Heart as a Bridge to Allotransplantation in Infants Just Around the Corner? Ann Thorac Surg (2022) 114:536–44. doi:10.1016/j.athoracsur.2021.05.025
102. Hisashi, Y, Yamada, K, Kuwaki, K, Tseng, YL, Dor, F, Houser, SL, et al. Rejection of Cardiac Xenografts Transplanted From α1, 3-Galactosyltransferase Gene-Knockout (GalT-KO) Pigs to Baboons. Am J Transpl (2008) 8:2516–26. doi:10.1111/j.1600-6143.2008.02444.x
103. Shimizu, A, Meehan, SM, Kozlowski, T, Sablinski, T, Ierino, FL, Cooper, DKC, et al. Acute Humoral Xenograft Rejection: Destruction of the Microvascular Capillary Endothelium in Pig-To-Nonhuman Primate Renal Grafts. Lab Invest (2000) 80:815–30. doi:10.1038/labinvest.3780086
104. Shimizu, A, Hisashi, Y, Kuwaki, K, Tseng, YL, Dor, FJMF, Houser, SL, et al. Thrombotic Microangiopathy Associated With Humoral Rejection of Cardiac Xenografts From α1, 3-Galactosyltransferase Gene-Knockout Pigs in Baboons. Am J Pathol (2008) 172:1471–81. doi:10.2353/ajpath.2008.070672
105. Rosales, IA, Kinoshita, K, Maenaka, A, How, IDAL, Selig, MK, Laguerre, CM, et al. De Novo Membranous Nephropathy in a Pig-To-Baboon Kidney Xenograft: A New Xenograft Glomerulopathy. Am J Transpl (2024) 24:30–6. doi:10.1016/j.ajt.2023.08.016
106. Houser, SL, Kuwaki, K, Knosalla, C, Dor, FJMF, Gollackner, B, Cheng, J, et al. Thrombotic Microangiopathy and Graft Arteriopathy in Pig Hearts Following Transplantation into Baboons. Xenotransplantation (2004) 11:416–25. doi:10.1111/j.1399-3089.2004.00155.x
107. Robson, SC, Cooper, DKC, and d’Apice, AJF. Disordered Regulation of Coagulation and Platelet Activation in Xenotransplantation. Xenotransplantation (2000) 7:166–76. doi:10.1034/j.1399-3089.2000.00067.x
108. d’Apice, AJF, and Cowan, PJ. Profound Coagulopathy Associated With Pig-To-Primate Xenotransplants: How Many Transgenes Will Be Required to Overcome This New Barrier? Transplantation (2000) 70:1273–4. doi:10.1097/00007890-200011150-00003
109. Ierino, FL, Kozlowski, T, Siegel, JB, Shimizu, A, Colvin, RB, Banerjee, PT, et al. Disseminated Intravascular Coagulation in Association With the Delayed Rejection of Pig-To-Baboon Renal Xenografts. Transplantation (1998) 66:1439–50. doi:10.1097/00007890-199812150-00006
110. Kozlowski, T, Shimizu, A, Lambrigts, D, Yamada, K, Fuchimoto, Y, Glaser, R, et al. Porcine Kidney and Heart Transplantation in Baboons Undergoing a Tolerance Induction Regimen and Antibody Adsorption. Transplantation (1999) 67:18–30. doi:10.1097/00007890-199901150-00004
111. Lin, CC, Cooper, DKC, and Dorling, A. Coagulation Dysregulation as a Barrier to Xenotransplantation in the Primate. Transpl Immunol (2009) 21:75–80. doi:10.1016/j.trim.2008.10.008
112. Mueller, NJ, Kuwaki, K, Dor, FJMF, Knosalla, C, Gollackner, B, Wilkinson, RA, et al. Reduction of Consumptive Coagulopathy Using Porcine Cytomegalovirus-Free Cardiac Porcine Grafts in Pig-To-Primate Xenotransplantation. Transplantation (2004) 78:1449–53. doi:10.1097/01.tp.0000141361.68446.1f
113. Yamada, K, Tasaki, M, Sekijima, M, Wilkinson, RA, Villani, V, Moran, SG, et al. Porcine Cytomegalovirus Infection Is Associated With Early Rejection of Kidney Grafts in a Pig to Baboon Xenotransplantation Model. Transplantation (2014) 98:411–8. doi:10.1097/TP.0000000000000232
114. Ezzelarab, MB, and Cooper, DKC. Systemic Inflammation in Xenograft Recipients (SIXR): A New Paradigm in Pig-To-Primate Xenotransplantation? Int J Surg (2015) 23:301–5. doi:10.1016/j.ijsu.2015.07.643
115. Ezzelarab, MB, Ekser, B, Azimzadeh, A, Lin, CC, Zhao, Y, Rodriguez, R, et al. Systemic Inflammation in Xenograft Recipients Precedes Activation of Coagulation. Xenotransplantation (2015) 22:32–47. doi:10.1111/xen.12133
116. Iwase, H, Ekser, B, Zhou, H, Liu, H, Satyananda, V, Humar, R, et al. Further Evidence for Sustained Systemic Inflammation in Xenograft Recipients (SIXR). Xenotransplantation (2015) 22:399–405. doi:10.1111/xen.12182
117. Iwase, H, Liu, H, Li, T, Zhang, Z, Gao, B, Hara, H, et al. Therapeutic Regulation of Systemic Inflammation in Xenograft Recipients. Xenotransplantation (2017) 24:e12296. doi:10.1111/xen.12296
118. hompson, CP, Jagdale, A, Walcott, G, Iwase, H, Foote, JB, Cron, RQ, et al. A Perspective on the Potential Detrimental Role of Inflammation in Pig Orthotopic Heart Xenotransplantation. Xenotransplantation (2021) 28:e12687. doi:10.1111/xen.12687
119. Zhao, Y, Cooper, DKC, Wang, H, Chen, P, He, C, Cai, Z, et al. Potential Pathological Role of Pro-Inflammatory Cytokines (IL-6, TNF-α, and IL-17) in Xenotransplantation. Xenotransplantation (2019) 26:e12502. doi:10.1111/xen.12502
120. Zhang, G, Iwase, H, Wang, L, Yamamoto, T, Jagdale, A, Ayares, D, et al. Is Interleukin-6 Receptor Blockade (Tocilizumab) Beneficial or Detrimental to Pig-To-Baboon Organ Xenotransplantation? Am J Transpl (2020) 20:999–1013. doi:10.1111/ajt.15712
121. Zhang, G, Iwase, H, Li, Q, Yamamoto, T, Jagdale, A, Ezzelarab, MB, et al. The Role of Interleukin-6 (IL-6) in the Systemic Inflammatory Response in Xenograft Recipients and in Pig Kidney Xenograft Failure. Front Immunol (2021) 12:788949. doi:10.3389/fimmu.2021.788949
122. Bikhet, MH, Hansen-Estruch, C, Javed, M, Collins, DE, Foote, JB, Ayares, D, et al. Profound Thrombocytopenia Associated With Administration of Multiple Anti-Inflammatory Agents in Baboons. Immun Inflamm Dis (2022) 10(3):e588. doi:10.1002/iid3.588
123. Oropeza, M, Petersen, B, Carnwath, JW, Lucas-Hahn, A, Lemme, E, Hassel, P, et al. Transgenic Expression of the Human A20 Gene in Cloned Pigs Provides Protection Against Apoptotic and Inflammatory Stimuli. Xenotransplantation (2009) 16:522–34. doi:10.1111/j.1399-3089.2009.00556.x
124. Yamamoto, T, Hara, H, Iwase, H, Jagdale, A, Bikhet, MH, Morsi, MA, et al. The Final Obstacle to Successful Pre-Clinical Xenotransplantation? Xenotransplantation (2020) 27:e12596. doi:10.1111/xen.12596
125. Yamamoto, T, Iwase, H, Patel, D, Jagdale, A, Ayares, D, Anderson, D, et al. Old World Monkeys Are Less Than Ideal Transplantation Models for Testing Pig Organs Lacking Three Carbohydrate Antigens (Triple-Knockout). Sci Rep (2020) 10:9771. doi:10.1038/s41598-020-66311-3
126. Yamamoto, T, Hara, H, Ayares, D, and Cooper, DKC. The Problem of the “4th Xenoantigen” After Pig Organ Transplantation in Non-Human Primates May Be Overcome by Expression of Human “Protective” Proteins. Xenotransplantation (2021) 28(2):e12658. doi:10.1111/xen.12658
127. Cui, Y, Yamamoto, T, Raza, SS, Morsi, M, Nguyen, HQ, Ayares, D, et al. Evidence for GTKO/β4GalNT2KO Pigs as the Preferred Organ-Source for Old World Nonhuman Primates as a Preclinical Model of Xenotransplantation. Transpl Direct (2020) 6:e590. doi:10.1097/TXD.0000000000001038
128. Foote, JB, Jagdale, A, Yamamoto, T, Hara, H, Bikhet, MH, Schuurman, H, et al. Histopathology of Pig Kidney Grafts With/Without Expression of the Carbohydrate Neu5Gc in Immunosuppressed Baboons. Xenotransplantation (2021) 28:e12715. doi:10.1111/xen.12715
129. Kim, SC, Wakwe, W, Higginbotham, LB, Mathews, DV, Breeden, CP, Stephenson, AC, et al. Fc-Silent Anti-CD154 Domain Antibody Effectively Prevents Nonhuman Primate Renal Allograft Rejection. Am J Transpl (2017) 17:1182–92. doi:10.1111/ajt.14197
130. Jagdale, A, Nguyen, H, Iwase, H, Foote, JB, Yamamoto, T, Javed, M, et al. T and B Lymphocyte Dynamics After Genetically-Modified Pig-To-Baboon Kidney Xenotransplantation With an Anti-CD40mAb-Based Immunosuppressive Regimen. Transpl Immunol (2022) 71:101545. doi:10.1016/j.trim.2022.101545
131. Bikhet, M, Iwase, H, Yamamoto, T, Jagdale, A, Foote, JB, Ezzelarab, M, et al. What Therapeutic Regimen Will Be Optimal for Initial Clinical Trials of Pig Organ Transplantation? Transplantation (2021) 105:1143–55. doi:10.1097/TP.0000000000003622
132. Maenaka, A, Kinoshita, K, Hara, H, and Cooper, DKC. The Case for the Therapeutic Use of Mechanistic/Mammalian Target of Rapamycin (mTOR) Inhibitors in Xenotransplantation. Xenotransplantation (2023) 30:e12802. doi:10.1111/xen.12802
133. Cooper, DKC, Foote, JB, Javed, M, Nguyen, HQ, Bikhet, MH, Hansen-Estruch, C, et al. Initial Evidence That Blockade of the CD40/CD154 Costimulation Pathway Alone Is Sufficient as Maintenance Therapy in Xenotransplantation. Xenotransplantation (2021) 28:e12721. doi:10.1111/xen.12721
134. Takahashi, K. Recent Findings in ABO-Incompatible Kidney Transplantation: Classification and Therapeutic Strategy for Acute Antibody-Mediated Rejection Due to ABO-Blood-Group-Related Antigens During the Critical Period Preceding the Establishment of Accommodation. Clin Exp Nephrol (2007) 11:128–41. doi:10.1007/s10157-007-0461-z
135. Fiorentino, M, Pesce, F, Schena, A, Simone, S, Castellano, G, and Gesualdo, L. Updates on Urinary Tract Infections in Kidney Transplantation. J Nephrol (2019) 32:751–61. doi:10.1007/s40620-019-00585-3
136. Teranishi, K, Treter, S, Alwayn, IPJ, Buhler, L, Awwad, M, and Cooper, DKC. Assessment of Methotrexate as a Potential Immunosuppressive Agent in Baboons. J Heart Lung Transpl (2001) 20:1335–9. doi:10.1016/s1053-2498(01)00317-5
137. Gollackner, B, Ryan, D, Knosalla, C, Basker, M, Alwayn, IPJ, Harper, D, et al. An Exploratory Investigation of the Effect of Arsenic Trioxide on Anti-Gal Antibody Production in Baboons. Xenotransplantation (2003) 10:80–7. doi:10.1034/j.1399-3089.2003.01136.x
138. Knosalla, C, Teranishi, K, Gollackner, B, Dor, FJ, and Cooper, DKC. Neurotoxicity of Leflunomide in Baboons. Xenotransplantation (2003) 10:187–8. doi:10.1034/j.1399-3089.2003.02110.x
139. Yamamoto, S, and Cooper, DKC. An Investigation of the Effect of Thalidomide on Anti-Gal Antibody Production in Baboons. Xenotransplantation (2003) 10:470–4. doi:10.1034/j.1399-3089.2003.00064.x
140. Ezzelarab, M, and Cooper, DKC. The Potential of Statins in Xenotransplantation. Xenotransplantation (2007) 14(2):100–3. doi:10.1111/j.1399-3089.2007.00389.x
141. Ezzelarab, M, Welchons, D, Torres, C, Hara, H, Long, C, Yeh, P, et al. Atorvastatin Down-Regulates the Primate Cellular Response to Porcine Aortic Endothelial Cells In Vitro. Transplantation (2008) 86:733–7. doi:10.1097/TP.0b013e3181821cad
142. Hara, H, Bentall, A, Long, C, Fang, J, Andreyev, O, Lunz, J, et al. Minimal Effect of Bortezomib in Reducing Anti-Pig Antibodies in Human Leukocyte Antigen-Sensitized Patients: A Pilot Study. Xenotransplantation (2013) 20:429–37. doi:10.1111/xen.12052
143. Harper, D, Gollackner, B, Xu, Y, Calderhead, D, Ryan, D, Li, W, et al. In Vitro and In Vivo Investigation of a Novel Monoclonal Antibody to Plasma Cells (W5 mAb). Xenotransplantation (2004) 11:78–90. doi:10.1111/j.1399-3089.2004.00086.x
144. Koren, E, Milotic, F, Neethling, FA, Koscec, M, Fei, D, Kobayashi, T, et al. Monoclonal Antiidiotypic Antibodies Neutralize Cytotoxic Effects of Anti-αGal Antibodies. Transplantation (1996) 62:837–43. doi:10.1097/00007890-199609270-00023
145. McMorrow, IM, Buhler, L, Treter, S, Neethling, FA, Alwayn, IPJ, Comrack, CA, et al. Modulation of the In Vivo Primate Anti-Gal Response Through Administration of Anti-Idiotypic Antibodies. Xenotransplantation (2002) 9:106–14. doi:10.1034/j.1399-3089.2002.1o028.x
146. Van Der Windt, DJ, Smetanka, C, Macedo, C, He, J, Lakomy, R, Bottino, R, et al. Investigation of Lymphocyte Depletion and Repopulation Using Alemtuzumab (Campath-1H) in Cynomolgus Monkeys. Am J Transpl (2010) 10:773–83. doi:10.1111/j.1600-6143.2010.03050.x
147. Phelps, CJ, Ball, SF, Vaught, TD, Vance, AM, Mendicino, M, Monahan, JA, et al. Production and Characterization of Transgenic Pigs Expressing Porcine CTLA4-Ig. Xenotransplantation (2009) 16:477–85. doi:10.1111/j.1399-3089.2009.00533.x
148. Koshika, T, Phelps, C, Fang, J, Lee, SE, Fujita, M, Ayares, D, et al. Relative Efficiency of Porcine and Human Cytotoxic T-Lymphocyte Antigen 4 Immunoglobulin in Inhibiting Human CD4+ T-Cell Responses Co-Stimulated by Porcine and Human B7 Molecules. Immunology (2011) 134:386–97. doi:10.1111/j.1365-2567.2011.03496.x
149. Hara, H, Witt, W, Crossley, T, Long, C, Isse, K, Fan, L, et al. Human Dominant-Negative Class II Transactivator Transgenic Pigs–Effect on the Human Anti-Pig T-Cell Immune Response and Immune Status. Immunology (2013) 140:39–46. doi:10.1111/imm.12107
150. Klymiuk, N, Van Buerck, L, Bähr, A, Offers, M, Kessler, B, Wuensch, A, et al. Xenografted Islet Cell Clusters From INS LEA29Y Transgenic Pigs Rescue Diabetes and Prevent Immune Rejection in Humanized Mice. Diabetes (2012) 61:1527–32. doi:10.2337/db11-1325
151. Cross-Najafi, AA, Farag, K, Isidan, A, Li, W, Zhang, W, Lin, Z, et al. Co-Expression of HLA-E and HLA-G on Genetically Modified Porcine Endothelial Cells Attenuates Human NK Cell-Mediated Degranulation. Front Immunol (2023) 14:1217809. doi:10.3389/fimmu.2023.1217809
152. Plege, A, Borns, K, Beer, L, Baars, W, Klempnauer, J, and Schwinzer, R. Downregulation of Cytolytic Activity of Human Effector Cells by Transgenic Expression of Human PD-Ligand-1 on Porcine Target Cells. Transpl Int (2010) 23:1293–300. doi:10.1111/j.1432-2277.2010.01130.x
153. Buermann, A, Petkov, S, Petersen, B, Hein, R, Lucas-Hahn, A, Baars, W, et al. Pigs Expressing the Human Inhibitory Ligand PD-L1 (CD 274) Provide a New Source of Xenogeneic Cells and Tissues With Low Immunogenic Properties. Xenotransplantation (2018) 25:e12387. doi:10.1111/xen.12387
154. Reyes, LM, Estrada, JL, Wang, ZY, Blosser, RJ, Smith, RF, Sidner, RA, et al. Creating Class I MHC–Null Pigs Using Guide RNA and the Cas9 Endonuclease. J Immunol (2014) 193:5751–7. doi:10.4049/jimmunol.1402059
155. Ladowski, JM, Reyes, LM, Martens, GR, Butler, JR, Wang, ZY, Eckhoff, DE, et al. Swine Leukocyte Antigen Class II Is a Xenoantigen. Transplantation (2018) 102:249–54. doi:10.1097/TP.0000000000001924
156. Ladowski, JM, Hara, H, and Cooper, DKC. The Role of SLAs in Xenotransplantation. Transplantation (2021) 105:300–7. doi:10.1097/TP.0000000000003303
157. Nishimura, H, Scalea, J, Wang, Z, Shimizu, A, Moran, S, Gillon, B, et al. First Experience With the Use of a Recombinant CD3 Immunotoxin as Induction Therapy in Pig-To-Primate Xenotransplantation: The Effect of T-Cell Depletion on Outcome. Transplantation (2011) 92:641–7. doi:10.1097/TP.0b013e31822b92a5
Keywords: immunosuppression, xenotransplantation, transplantation immunology, swine, non-human primate
Citation: Sanatkar SA, Kinoshita K, Maenaka A, Hara H and Cooper DKC (2025) The Evolution of Immunosuppressive Therapy in Pig-to-Nonhuman Primate Organ Transplantation. Transpl Int 37:13942. doi: 10.3389/ti.2024.13942
Received: 15 October 2024; Accepted: 27 November 2024;
Published: 13 January 2025.
Copyright © 2025 Sanatkar, Kinoshita, Maenaka, Hara and Cooper. This is an open-access article distributed under the terms of the Creative Commons Attribution License (CC BY). The use, distribution or reproduction in other forums is permitted, provided the original author(s) and the copyright owner(s) are credited and that the original publication in this journal is cited, in accordance with accepted academic practice. No use, distribution or reproduction is permitted which does not comply with these terms.
*Correspondence: S. A. Sanatkar, c3NhbmF0a2FyQG1naC5oYXJ2YXJkLmVkdQ==